Cocoa improves age-associated health and extends lifespan in C. elegans
Abstract
BACKGROUND:
Cocoa, one of the richest dietary sources of polyphenols has been studied for its health promoting effects, but how long-term consumption of cocoa affects age-associated health and lifespan is not well defined.
OBJECTIVE:
The objective of this study was to determine the effects of long-term cocoa consumption on age-associated health and lifespan in C. elegans
METHODS:
The standard E. coli OP50 diet of wild type C. elegans was supplemented with cocoa powder starting from L1 stage until they die. Body length and area were measured as indicators of worm nutrition. Age associated health was determined at different stages of life as day 4, day 8 and day 12 using worm locomotion, thermotolerance, cognition and mitochondrial function. In addition, lifespan was evaluated.
RESULTS:
Cocoa improved age-associated decline in neuromuscular function. Both mean and median lifespan were extended by cocoa supplementation. However, maximum lifespan was not affected. Cocoa showed beneficial effects on thermotolerance at all ages (more prominent effects at young (day 4) and middle (day 8) age). Further, consumption of cocoa improved age-related learning deficits, short-term memory loss and mitochondrial dysfunction.
CONCLUSIONS:
Long-term cocoa consumption seemed to improve age-associated health and extends lifespan in C. elegans
1Introduction
Aging is the primary risk factor for many chronic diseases including cancer, diabetes, cardiovascular and neurodegenerative diseases [1]. Mitochondrial dysfunction which is characterized by the accumulation of mitochondrial DNA (mtDNA) mutations and the increased production of reactive oxygen species (ROS) is considered to be one of the key hallmarks in aging [2]. The free radical theory of aging suggests that increased ROS production in mitochondria with age causes oxidative damage to cellular macromolecules thereby, further increasing the production of ROS and the accumulation of molecular damage [3]. The imbalance between excessive ROS production and limited anti-oxidant defences leads to oxidative stress and ultimately aging and related diseases [4].
Diet-derived antioxidants have recently gained the popularity for their protective/preventive roles aga-inst chronic diseases [5, 6]. Epidemiological studies suggest that polyphenols are the major source of antioxidants that exert the preventive/protective role [7–9]. Cocoa and its products are known to be rich sources of polyphenols which significantly contri-butes to the total antioxidant capacity of the diet [10, 11]. Consumption of cocoa or related products including chocolates has been reported to have age-related health promoting effects including improvements in cognitive function [7, 12, 13], cardiovascular aging [14], oxidative stress [15], physical performance and quality of life [16]. However, most of these studies are either short-term interventions or acute effects of cocoa supplementation. Effects of long-term consumption of cocoa starting from early life on healthspan and lifespan are not well-defined.
Caenorhabditis elegans (C. elegans) has been extensively used in aging research due to its attractive features including short lifespan, availability of mutants and many readily observable and quantifiable aging associated changes including tissue degeneration, decreased movement, accumulation of fluorescent material and reduced pharyngeal pumping [17]. Particularly, its short lifespan of approximately 17 days at 20 °C makes C. elegans an attractive model to study long-term effects of dietary interventions. Therefore, the aim of this study was to determine the effects of long-term cocoa consumption on age-associated health and lifespan using C. elegans as the model organism.
2Materials and methods
2.1Strains and culture conditions
Wild type (N2, Bristol) C. elegans and Escherichia coli (E. coli) OP50 were obtained from Caenorhabditis Genetics Center (Minneapolis, MN, USA). Worms were maintained on nematode growth medium (NGM) plates carrying a lawn of E. coli OP50. Except for thermotolerance assay, all the experiments were carried out at 20 °C.
2.2Cocoa treatment
In this study, we used commercially available cocoa powder which was an unsweetened dry powder processed with alkali. A cocoa suspension was made to the desired final concentration in M9 buffer in order to make it spreadable. For all the experiments except lifespan, a single dose of cocoa which is 5 mg of cocoa powder suspended in 1 ml of M9 was used. For the lifespan experiment, five different doses of cocoa were prepared by suspending 1 mg, 2 mg, 3 mg, 4 mg and 5 mg cocoa in 1 ml of M9 buffer. Concentrated E. coli OP50 was prepared by suspending 1 g of E. coli pellet in 16 ml of M9 buffer. Concentrated E. coli was first added to NGM plates and was grown overnight at 20 °C. Cocoa suspension was added on the top of E. coli lawn at a ratio of 1:2 volume, E. coli: cocoa suspension (for small plates: 100μl of E.coli and 200μl of cocoa, for medium plates: 200μl of E.coli and 400μl of cocoa). Worms received cocoa starting from L1 stage.
2.3Obtaining synchronous cultures
Age-synchronized worms were obtained by blea-ching gravid adults. Briefly, gravid hermaphrodites were washed off from an NGM plate with M9 buffer and made the final volume to 3.5 ml. Bleaching solution (bleach: 5N NaOH = 2:1) was added to the worm suspension at a volume of 1.5 ml. The suspension was mixed by vortexing the tube five seconds and keeping further 30 seconds on rest until all the worm bodies got dissolved. The suspension was centrifuged (2 min at 1300 g) to pellet the released eggs and the supernatant was poured off. M9 buffer was added to a final volume of 5 ml, centrifuged again and the supernatant was removed. This step was repeated at least three times to completely get rid of the bleaching solution. The egg pellet was resuspended in 3 ml of M9 and kept for 48 hours on a shaker for hatching.
2.4Body length, area and locomotion
Age synchronized worms were washed off from both treatment and control plates (at day 4, day 8 and day 12) and collected in a 15 ml falcon tube. Once worms settled to the bottom, supernatant was removed and 5 ml of M9 was added to wash off the food and repeated twice. Thereafter, 100μl of worm suspension was placed on plain agar plates in the absence of food. Plates were left in the laminar flow cabinet for 5 minutes to dry out the liquid. Then, they were placed under a stereo microscope (SMZ745T; Nikon, Japan equipped with G-AL 1.0x objective) and 30 seconds videos were captured using NIS Elements imaging software (version 5.01, Japan) and a digital camera (DS-Fi2; Nikon, Japan) with settings at 50 frames per second, 30 second duration per video and resolution of 640×480 pixels. The scale bar (μm) in the imaging software was used to convert pixels into μm. Worms were tracked and analysed via WormLab 3.1 software (MBF Bioscience, Williston, VT USA). Worms tracked over a minimum of 10 seconds were included in the final analysis. Mean body length (μm), mean area (μm2), mean speed (μm/s), maximum speed (μm/s), peristaltic speed (μm/s) and maximum amplitude (μm) were reported. According to the WormLab software, speed is defined as the distance per second travelled by the worm along its central axis. Peristaltic speed is defined as the peristaltic track length (length of forward motion minus length of reverse motion) divided by time. Maximum amplitude is the maximum centroid displacement over an entire track.
2.5Lifespan assay
Lifespan assay was performed as described in reference [18]. Floxuridine (FUdR) was not used to avoid progeny production and instead worms were transferred everyday onto fresh plates until they stop laying eggs (until day 9). Thereafter, worms were transferred every other day. Worms that crawled off from the plates were excluded from the analysis. Survival curves were plotted. Mean, median and maximum lifespan were reported.
2.6Thermotolerance
Worms were grown on NGM plates until they reached the required age (day 4 or day 8 or day 12). Approximately 75 worms per treatment were distributed among two NGM plates seeded with E. coli and maintained at 35 °C. Survival was measured every hour by touch provocation. Survival curves were plotted. Mean and median survival time were reported.
2.7Learning and short-term associative memory
Naive chemotaxis, learning and short-term associative memory were determined based on C. elegans positive butanone learning assay [19].
Age-synchronized worms (approximately 1000) were grown on high growth media plates with food until they reached the desired age. Chemotaxis assay (Fig. 1) was performed with approximately 200 worms. Rest were starved for 1 hour and at the end of starvation period, worms were associated with food and chemo-attractant butanone for 1 hour. Che-motaxis assay was repeated to test 1x (massed) associative learning immediately after conditioning (t = 0 time point). Rest of the worms were transferred equally to two NGM plates seeded with E. coli and incubated at 20 °C. After 1 hour, and 2 hours from food-butanone association, chemotaxis assay was performed with the worms on two plates (t = 1 hr and t = 2 hrs time points). Chemotaxis index (CI) was calculated as, CI = [(Number of worms at butanone) –(Number of worms at ethanol)]/ (Total number of worms). The learning index (LI) at different time points was calculated as, LI = Chemotaxis indext –Chemotaxis indexNaive. Dementia index (short-term associative memory loss) was calculated after 1 hour and 2 hours from the food-butanone association (Dementia indext = Learning index0 - Learning indext)
Fig. 1
Chemotaxis assay setup, adapted from the reference (19). (A) Chemotaxis assay plate with spots marked for the origin of worms (bottom), butanone and EtOH (left and right respectively). (B) 1μl of 2M NaN3 is added to the butanone and EtOH spots. (C) 1μl of 10%Butanone in 95%EtOH and 95%EtOH are added to the butanone and EtOH spots (left and right) (D) Worms (200) in M9 buffer are added to the origin. (E) KimWipe absorbs M9 and releases worms (F) Worms move to either butanone or EtOH spots and get paralysed.
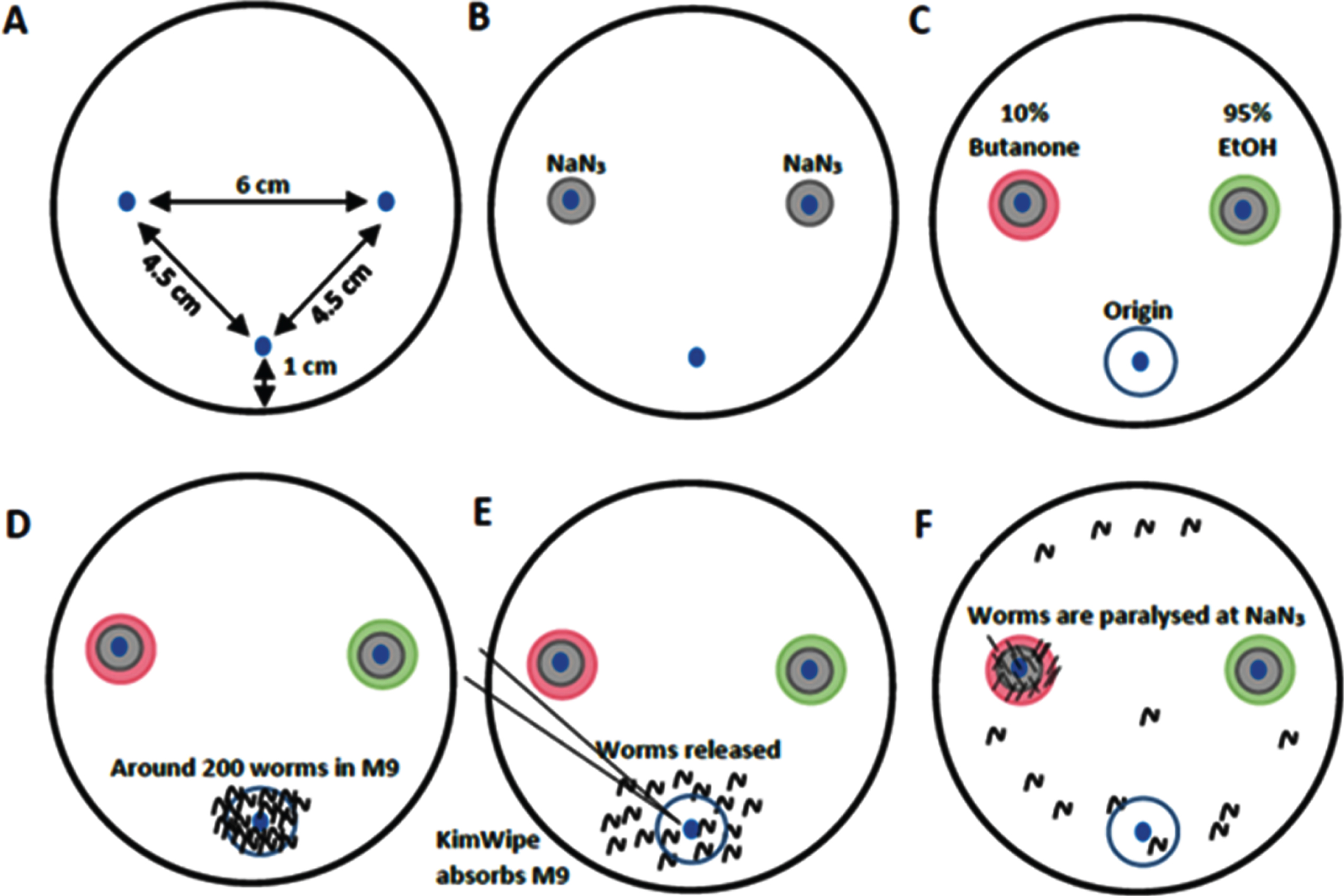
2.8Mitochondrial respiration
Oxygen consumption rate (OCR) was used to investigate the mitochondrial function of C. elegans. To measure OCR, XFe24 Extracellular Flux Analyzer (Seahorse Bioscience) was used as described in reference [20] and [21] with some modifications. Briefly, age-synchronized worms were washed off from plates and allowed to settle in a falcon tube. The process was repeated twice with M9 buffer and kept on an orbital shaker for 20 minutes to ensure the excretion of live E. coli in the gut. OCR was measured at day 4, day 8 and day 12 with 50, 35 and 35 worms in each well respectively followed by the addition of M9 to make the final volume of 500μl per well. Sodium azide (an inhibitor of oxidative phosphorylation via inhibition of cytochrome oxidase) was used at a final well concentration of 20mM. Measurement cycles were set up as 8 cycles for both basal and drug measurements. Mix, wait and measure cycles were set as 3, 2 and 3 minutes respectively. OCR readings were normalized based on the number of worms added per well. Basal respiration (OCR in the absence of sodium azide), non-mitochondrial respiration (OCR in the presence of sodium azide) and mitochondrial respiration (basal-non-mitochondrial) were calculated.
2.9Analysis of cocoa powder
Cocoa powder was analysed for total phenolic (TP) and total flavonoid (TF) contents and for antioxidant activity. Prior to analysis, cocoa powder was defatted by extracting four times with hexane [22]. Total phenolics were determined using the spectrometric analysis with Folin-Ciocalteu’s phenol reagent [23]. Following the same procedure, a standard curve for TP was prepared using gallic acid standard solution (0–100 mg/l). TP in cocoa extract were expressed as milligrams of gallic acid equivalents (GAE) per gram cocoa powder. Sample was analysed in six replicates. TF content was determined according to a colorimetric assay [24]. Quantification of TF was done using catechin as the standard compound. TF were expressed as milligrams of catechin equivalents (CE) per gram cocoa powder. Sample was analysed in six replicates. Antioxidant activity of the cocoa extract was determined according to the ABTS [2,2′-azino-di-(3-ethylbenzthiazoline sulfonic acid)] assay [25].
2.10Statistical analysis
All statistical analyses were performed in IBM SPSS® statistics software (version 24). Data were expressed as mean±standard error of mean (SEM). Differences between groups for body length, mean area, locomotion, chemotaxis and OCR were analysed using general linear model (multivariate test). Survival function was estimated using Kaplan-Meier curves. Survival curves were compared using log rank (Mantel-Cox) test. Maximum lifespan was the average lifespan of top 10 longest-lived worms. Differences between groups for maximum lifespan were determined using one-way ANOVA with post-tests (Tukey’s test). P < 0.05 was considered significant.
3Results
3.1Effect of cocoa-supplementation on body length and area
We measured the body length and area at two time points as day 4 (1st day of adulthood) to represent young age and day 8 (5th day of adulthood), the middle age at which that they have fully grown. Analysis of body length showed that cocoa supplemented worms were significantly longer (Fig. 2A) than control worms at both day 4 and day 8 (P < 0.05). In addition, cocoa-supplemented worms were significantly thicker (Fig. 2B) than control worms at day 8 as indicated by the mean area (P < 0.05).
Fig. 2
Body length (μm) and area (μm2) of control and cocoa-supplemented wild type (N2) C. elegans. Body length (μm) and area (μm2) were measured at two different time points as day 4 (1st day of adulthood) and day 8 (5th day of adulthood). Experiments were performed in triplicate. Values are expressed in mean±SEM (n = 52 for control at both day 4 and 8 and n = 53 for cocoa at both day 4 and 8). *P < 0.05 for the indicated comparison (calculated using multivariate test, general linear model). (A) Cocoa supplemented worms were significantly longer than control worms at both day 4 and day 8 (P < 0.05). (B) Cocoa-supplemented worms were significantly thicker than control worms at day 8 as indicated by the mean area (P < 0.05).
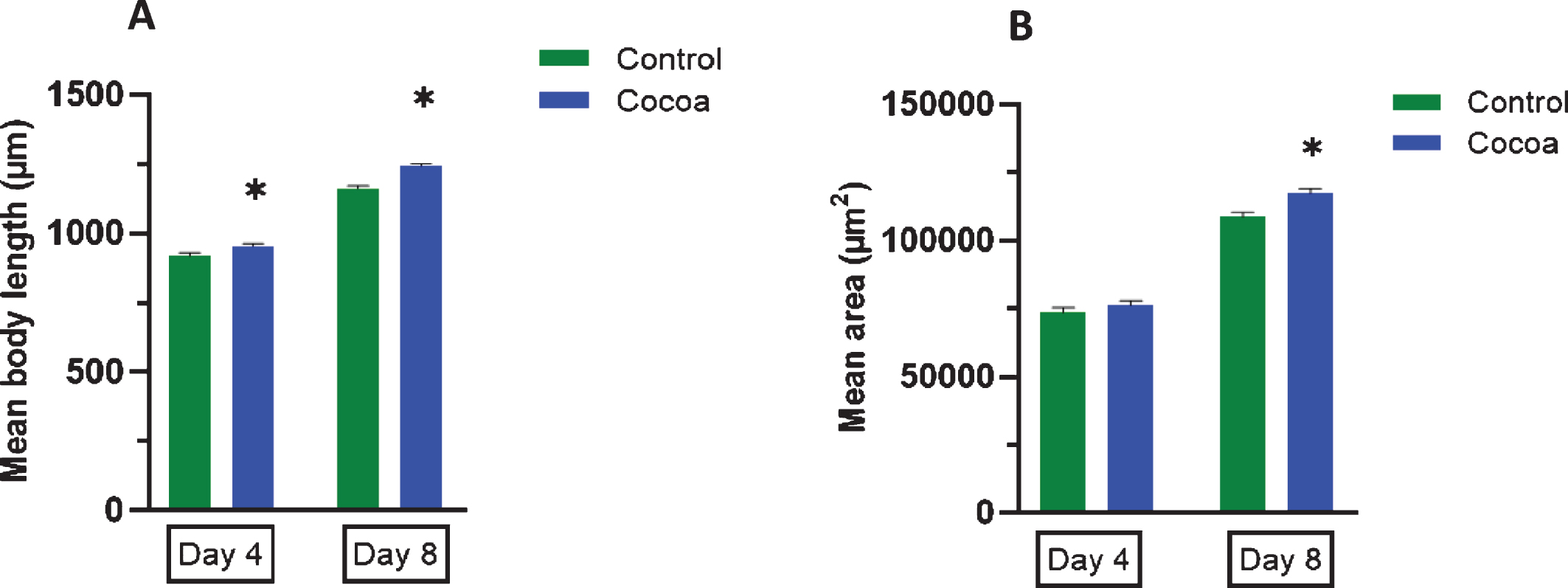
3.2Effect of cocoa-supplementation on locomotion
C. elegans showed a reduction in mean speed over time (9.0%at day 8 and 29.0%at day 12 compared to day 4, Fig. 3A). Even though cocoa-supplementation significantly reduced the mean speed at day 8 (P < 0.05, 9.8%), it did not affect the mean speed at day 4 and day 12. At day 12, worms showed a reduction in maximum speed compared to both day 4 and day 8 (31.4%, 37.0%respectively, Fig. 3B). Cocoa-supplementation increased the maximum speed of worms at later stage of the life (day 12) by 12%however, it was not statistically significant. Peristaltic speed of worms was decreased over time (47.3%at day 8 and 79.4%at day 12 compared to day 4, Fig. 3C). There was an improvement in peristaltic speed with cocoa-supplementation (20%) in later life (at day 12). Worms showed an increase in maximum amplitude (Fig. 3D) at day 8 compared to day 4 (36.6%), but it was decreased at day 12 compared to day 8 (20.6%). Interestingly, cocoa-supplementation significantly increased the maximum amplitude of worms at day 12 (P < 0.05, 8.8%).
Fig. 3
Locomotion parameters of control and cocoa-supplemented wild type (N2) C. elegans. Mean speed (μm/s), maximum speed (μm/s), peristaltic speed (μm/s) and maximum amplitude (μm) were measured at three different time points as day 4 (1st day of adulthood), day 8 (5th day of adulthood) and day 12 (9th day of adulthood). Experiments were performed in triplicate. Values are expressed in mean±SEM (n = 52 for control and n = 53 for cocoa at all 3 time points). *P < 0.05 for the indicated comparison (calculated using multivariate test, general linear model). (A) Worms showed a reduction in mean speed over time. Cocoa-supplemented worms showed a significantly reduced mean speed at day 8 (P < 0.05), but there was no effect significant at day 4 and day 12. (B) Worms showed a reduction in maximum speed at day 12 compared to both day 4 and day 8. Cocoa-supplementation increased the maximum speed of worms at later stage of the life (day 12). (C) Peristaltic speed of worms was decreased over time. There was an improvement in peristaltic speed with cocoa-supplementation in later life (at day 12). (D) Worms showed an increase in maximum amplitude at day 8 compared to day 4, but it was decreased at day 12 compared to day 8. Cocoa-supplementation significantly increased the maximum amplitude of worms at day 12 (P < 0.05).
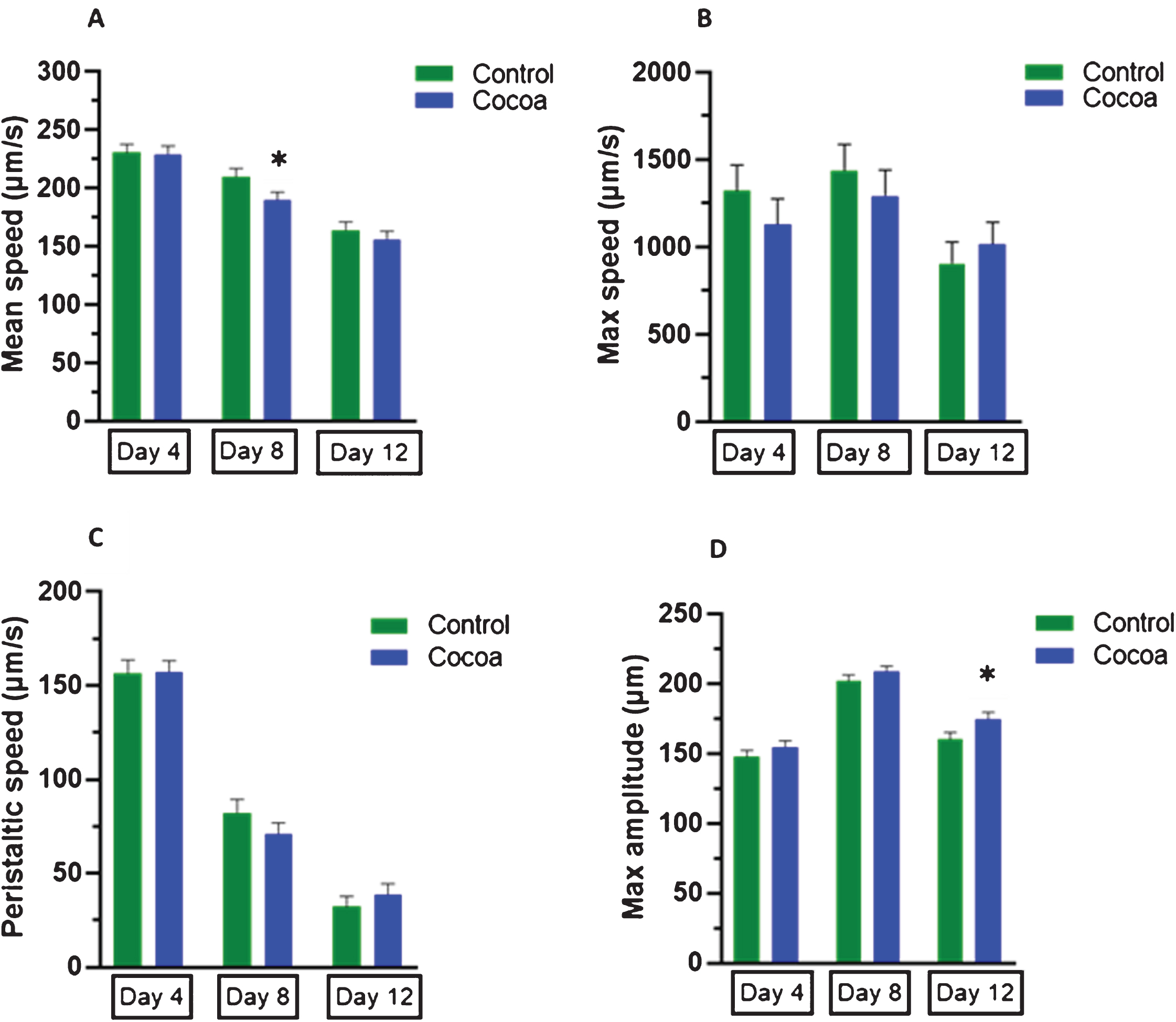
3.3Effect of cocoa-supplementation on lifespan
We performed a dose-response experiment for the lifespan where the worm diet was supplemented with cocoa at 1 mg, 2 mg, 3 mg, 4 mg and 5 mg concentrations. The wild type (N2) worms grown under our laboratory conditions at 20 °C had a mean lifespan of 13.5 days, a median lifespan of 13 days and a maximum lifespan of 16.7 days (Fig. 4, Table 1). The mean lifespan of worms was significantly increased by cocoa-supplementation at 3 mg/ml and 5 mg/ml doses by 8.3%and 5.9%respectively (P < 0.05). The median lifespan was increased by cocoa at 3 mg/ml, 4 mg/ml and 5 mg/ml doses (P < 0.05, all 3 doses by 7.8%). The maximum lifespan was not affected by any of the cocoa doses.
Fig. 4
Survival curves for control and cocoa-supplemented wild type (N2) C. elegans. Experiments were performed in triplicate (n = 122 for control, n = 116 for 1 mg/ml, n = 135 for 2 mg/ml, n = 112 for 3 mg/ml, n = 135 for 4 mg/ml and n = 118 for 5 mg/ml). Differences between groups for mean and median lifespans were calculated using log rank (Mantel-Cox) test and maximum lifespan using one-way ANOVA (Tukey’s test). Cocoa supplementation at a dose of 3 mg/ ml and 5 mg/ml significantly increased both mean (P < 0.05, 8.3%and 5.9%) and median (P < 0.05, 7.8%) lifespan. Cocoa at 4mg/ml dose also significantly increased the median lifespan (P < 0.05, 7.8%). However, maximum lifespan was not affected by any cocoa dose.
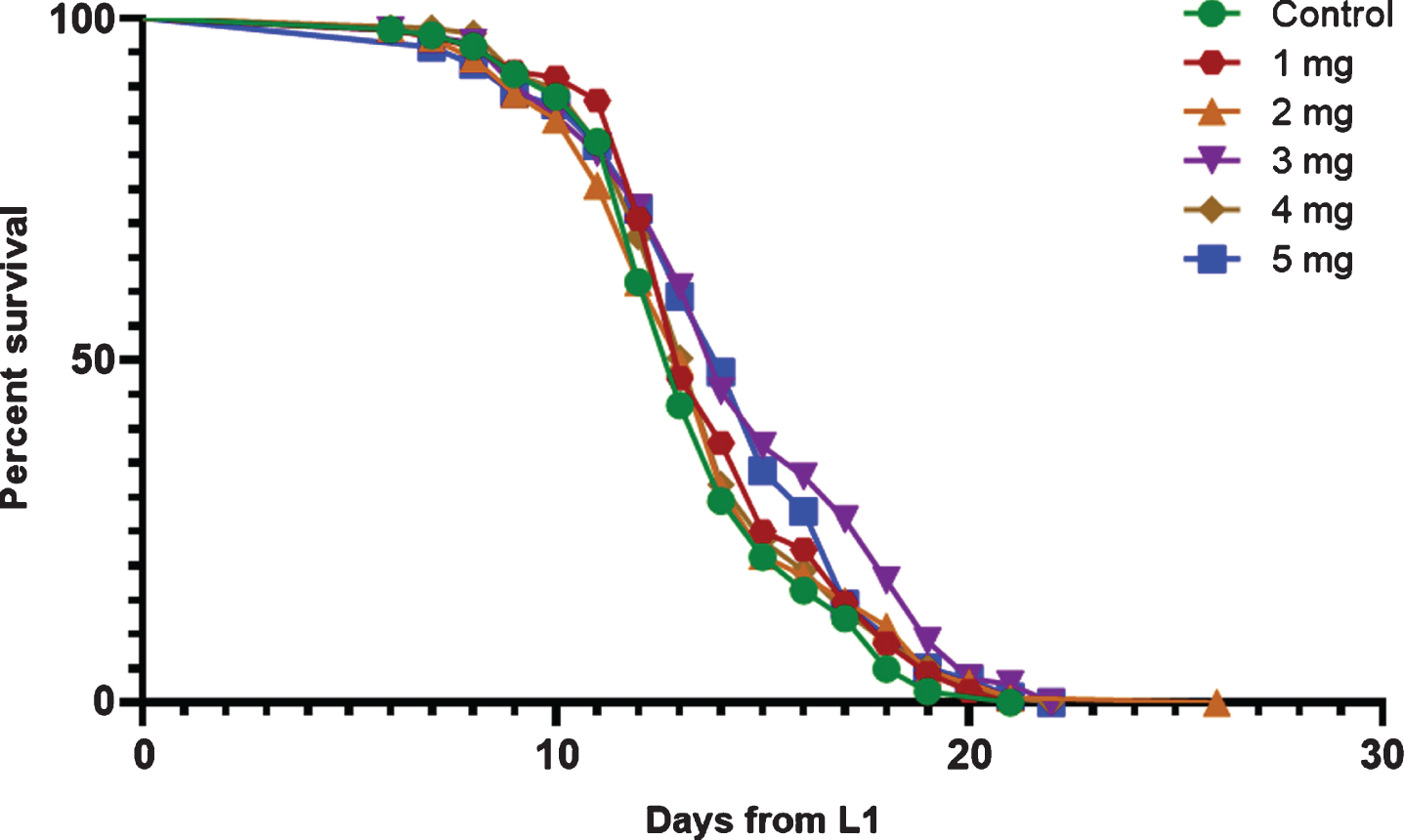
Table 1
Mean, median and maximum lifespans of different doses of cocoa-supplemented worms
Cocoa dose (mg/ml) | Mean lifespan | %extension compared to control | Median lifespan | %extension compared to control | Maximum lifespan |
0 | 13.5±0.26 | 13±0.25 | 16.7±0.38 | ||
1 | 14.0±0.28 | 13±0.28 | 16.9±0.46 | ||
2 | 13.6±0.29 | 13±0.28 | 17.3±0.53 | ||
3 | 14.6±0.35* | 8.3 | 14±0.41* | 7.8 | 18.2±0.46 |
4 | 13.8±0.26 | 14±0.22* | 7.8 | 17.4±0.43 | |
5 | 14.3±0.32* | 5.9 | 14±0.36* | 7.8 | 18.0±0.40 |
*Statistically significant (P < 0.05) compared to the control group.
3.4Effect of cocoa-supplementation on thermotolerance
We measured the survival of control and cocoa treated worms at 35 °C. At both day 4 and day 8, control worms showed a mean survival time of 8.2 hours and a median survival time of 8 hours (Fig. 5, Table 2). At day 12, the mean and median survival time for control worms were 7.1 hours and 7 hours respectively. Both mean and median survival times of control worms were significantly reduced at day 12 compared to both day 4 and day 8 (P < 0.05). Cocoa supplementation significantly increased the mean survival time by 15.0%at day 4, 11.1%at day 8 and 3.3%at day 12 (P < 0.05). Moreover, cocoa-supplementation significantly increased the median survival time by 12.5%at both day 4 and day 8 (P < 0.05). Results revealed that the cocoa effect is more prominent at early stages of life than latter stages.
Fig. 5
Survival curves at 35 °C for control and cocoa-supplemented wild type (N2) C. elegans at day 4, day 8 and day 12. Experiments were performed in triplicate [n = 204 (day 4); n = 224 (day 8); n = 252 (day 12) for control and n = 178 (day 4); n = 218 (day 8); n = 194 (day 12) for cocoa]. Differences between survival curves were calculated using log rank (Mantel-Cox) test. Both mean and median survival times of control worms were significantly reduced at day 12 compared to both day 4 and day 8 (P < 0.05). Cocoa supplementation significantly increased the mean survival time at day 4, day 8 and day 12 (P < 0.05, 15.0%, 11.1%and 3.3%respectively). Cocoa supplementation significantly increased the median survival time at both day 4 and day 8 (P < 0.05, 12.5%).
![Survival curves at 35 °C for control and cocoa-supplemented wild type (N2) C. elegans at day 4, day 8 and day 12. Experiments were performed in triplicate [n = 204 (day 4); n = 224 (day 8); n = 252 (day 12) for control and n = 178 (day 4); n = 218 (day 8); n = 194 (day 12) for cocoa]. Differences between survival curves were calculated using log rank (Mantel-Cox) test. Both mean and median survival times of control worms were significantly reduced at day 12 compared to both day 4 and day 8 (P < 0.05). Cocoa supplementation significantly increased the mean survival time at day 4, day 8 and day 12 (P < 0.05, 15.0%, 11.1%and 3.3%respectively). Cocoa supplementation significantly increased the median survival time at both day 4 and day 8 (P < 0.05, 12.5%).](https://content.iospress.com:443/media/nha/2021/6-1/nha-6-1-nha200100/nha-6-nha200100-g005.jpg)
Table 2
Mean and median survival times for cocoa-supplemented worms
Day | Treatment | Mean survival time | %extension compared to control | Median survival time | %extension compared to control |
4 | Control | 8.2±0.09 | 8±0.10 | ||
Cocoa | 9.5±0.08* | 15.0 | 9±0.09* | 12.5 | |
8 | Control | 8.2±0.11 | 8±0.14 | ||
Cocoa | 9.1±0.12* | 11.1 | 9±0.14* | 12.5 | |
12 | Control | 7.1±0.10 | 7±0.09 | ||
Cocoa | 7.4±0.10* | 3.3 | 7±0.09 |
*Statistically significant (P < 0.05) compared to the control group.
3.5Effect of cocoa-supplementation on learning and short-term associative memory
At day 8, worms showed a significant reduction in chemotaxis towards butanone even after conditioning (87.2%reduction in naive chemotaxis and 86.0%reduction in learning index). Therefore, we determined chemotaxis behaviour only at day 4 and day 8 as it was not detectable at day 12. There was no significant effect of cocoa-supplementation on naive chemotaxis at both day 4 and day 8 (Fig. 6A). However, cocoa-supplementation significantly increased the learning index of worms at day 8 (P < 0.05, Fig. 6B). As learning index of control worms at day 8 was lower, we did not measure the short-term memory loss (dementia index) at day 8. However, cocoa-supplemented worms showed a reduction (27%) in dementia index 2 hours after conditioning at day 4 which was not statistically significant (Fig. 6C).
Fig. 6
Naive chemotaxis index, learning index and dementia index (short-term memory loss) of control and cocoa-supplemented wild type (N2) C. elegans as measured at day 4 (1st day of adulthood) and day 8 (5th day of adulthood) using C. elegans positive butanone learning and short-term memory assay. Chemotaxis assay was performed for well-fed, synchronized worms and naive chemotaxis index was calculated; chemotaxis index (CI) = [(number of worms at butanone) –(number of worms at ethanol)]/ [total number of worms on the plate]. Well-fed, synchronized worms were starved for 1 hour, then fed in the presence of 10%butanone for 1 hour. Worms were tested immediately after food-butanone association for 1 hour for their learning; learning Index (LI) = chemotaxis indext –chemotaxis indexNaive. Dementia index (short-term associative memory loss) was measured after 1 hour and 2 hours from the food-butanone association without exposure to butanone again (Dementia indext = Learning index0 - Learning indext). Four independent experiments were performed (n≥50 worms per group). Values are expressed as mean±SEM. *P < 0.05 for the indicated comparison (calculated using multivariate test, general linear model). (A) There was no significant effect of cocoa supplementation on naive chemotaxis index at both day 4 and 8. (B) Cocoa supplementation significantly increased the learning index at day 8 (P < 0.05). (C) Cocoa-supplemented worms showed no significant difference in dementia index at day 4.
![Naive chemotaxis index, learning index and dementia index (short-term memory loss) of control and cocoa-supplemented wild type (N2) C. elegans as measured at day 4 (1st day of adulthood) and day 8 (5th day of adulthood) using C. elegans positive butanone learning and short-term memory assay. Chemotaxis assay was performed for well-fed, synchronized worms and naive chemotaxis index was calculated; chemotaxis index (CI) = [(number of worms at butanone) –(number of worms at ethanol)]/ [total number of worms on the plate]. Well-fed, synchronized worms were starved for 1 hour, then fed in the presence of 10%butanone for 1 hour. Worms were tested immediately after food-butanone association for 1 hour for their learning; learning Index (LI) = chemotaxis indext –chemotaxis indexNaive. Dementia index (short-term associative memory loss) was measured after 1 hour and 2 hours from the food-butanone association without exposure to butanone again (Dementia indext = Learning index0 - Learning indext). Four independent experiments were performed (n≥50 worms per group). Values are expressed as mean±SEM. *P < 0.05 for the indicated comparison (calculated using multivariate test, general linear model). (A) There was no significant effect of cocoa supplementation on naive chemotaxis index at both day 4 and 8. (B) Cocoa supplementation significantly increased the learning index at day 8 (P < 0.05). (C) Cocoa-supplemented worms showed no significant difference in dementia index at day 4.](https://content.iospress.com:443/media/nha/2021/6-1/nha-6-1-nha200100/nha-6-nha200100-g006.jpg)
3.6Effect of cocoa-supplementation on mitochondrial respiration
Both basal (Fig. 7A) and mitochondrial respiration (Fig. 7B) were significantly decreased in control worms at day 12 compared to day 4 (P < 0.05, 15.0%and 26.5%respectively). Cocoa-supplementation significantly increased both basal and mitochondrial respiration at both day 4 (P < 0.05, 38.0%and 35.4%respectively) and day 12 (P < 0.05, 19.4%and 41.4%respectively). However, cocoa supplementation significantly decreased the mitochondrial respiration at day 8 (P < 0.05). Non-mitochondrial respiration (Fig. 7C) in control worms was signifi-cantly higher at day 12 compared to day 4 (P < 0.05, 48.4%). Cocoa-supplementation significantly red-uced the non-mitochondrial respiration at day 12 to reach similar levels to day 4 (P < 0.05, 40.2%).
Fig. 7
(A) Basal respiration (B) mitochondrial respiration (basal-sodium azide) and (C) non-mitochondrial respiration of control and cocoa-supplemented wild type (N2) C. elegans at day 4, day 8 and day 12. Values are expressed in mean±SEM (n = 50 per well at day 4 and 8, n = 35 per well at day 12; 4 wells/treatment at all 3 time points). *P < 0.05 for the indicated comparison compared to control at each time point (calculated using multivariate test, general linear model). Both basal (A) and mitochondrial respiration (B) were significantly decreased in control worms at day 12 compared to day 4 (P < 0.05). Cocoa-supplementation significantly increased both basal and mitochondr-ial respiration at both day 4 and day 12 (P < 0.05). However, cocoa supplementation significantly decreased the mitochondrial respiration at day 8 (P < 0.05). Non-mitochondrial respiration (C) in control worms was significantly higher at day 12 compared to day 4 (P < 0.05). Cocoa-supplementation significantly reduced the non-mitochondrial respiration at day 12 to reach similar levels to day 4 (P < 0.05).
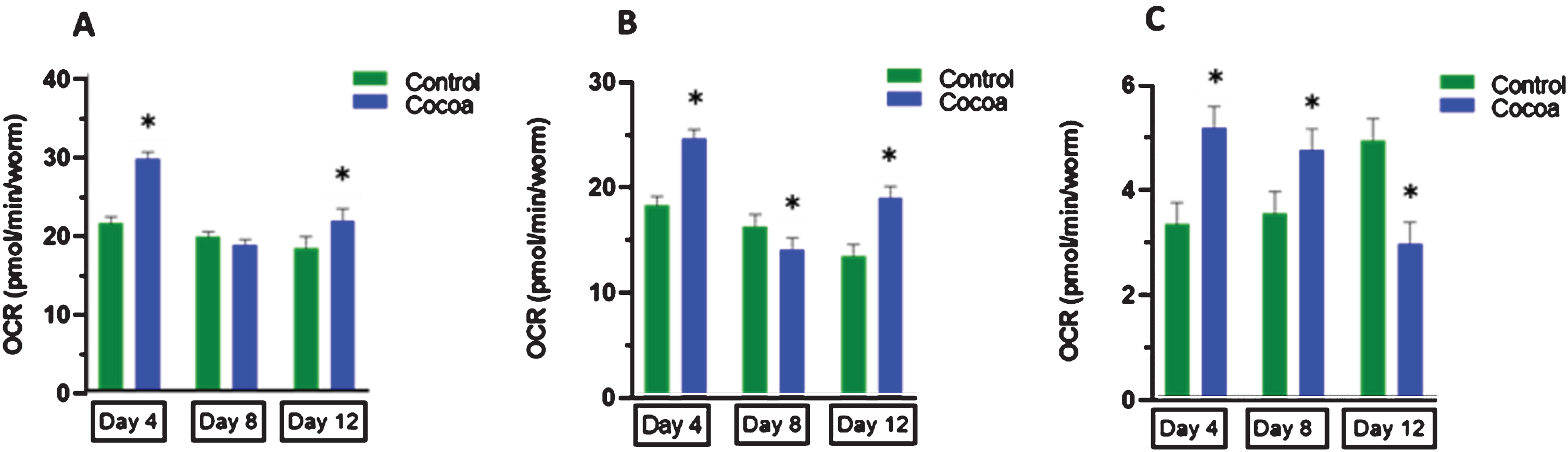
3.7Total phenolics content, total flavonoids content and the antioxidant activity of cocoa powder
The TP content of defatted cocoa powder was 27.01 mg GAE/g. The TF content was 10.13 mg CE/g and the antioxidant activity was 7.84 mg GAE/g.
4Discussion
Effect of long-term cocoa supplementation on age-associated health and lifespan was evaluated in the model organism C. elegans. Worm diet was supplemented with cocoa powder starting from L1 stage until they die. Body length and area were measured as indicators of growth and development. Moreover, a reduced body size could also indicate dietary restriction in C. elegans. Results indicated that cocoa provided a better nutrition to worms as cocoa-supplemented worms were longer and thicker than control worms. It has been widely reported that aging in C. elegans is accompanied by sarcopenia which is the progressive deterioration in muscle mass and strength [27]. In clinical settings, sarcopenia in humans is diagnosed with reduced gait speed, grip strength and muscle mass [28]. In C. elegans, the speed of movement is analogous to the gait speed of humans. Moreover, maximum speed is believed to be one of the powerful metrics of C. elegans health as it is highly correlated with longevity [29]. Apart from movement speed, maximum amplitude has been identified as a marker of C. elegans muscle strength. The mutants showing deficiencies in components of sarcomeres which transmits muscle contraction into locomotion have differences in bending amplitude, but not in locomotion [30]. Therefore, we measured mean speed, maximum speed and maximum amplitude as well-known aging-dependent markers of C. elegans [31]. In addition, we observed an age-dependent reduction in peristaltic speed which is the speed of forward movement. Hence, we used it as another marker of aging. All four parameters showed an age dependent decline. Even though it was not statistically significant, cocoa supplemented worms showed an increase in maximum speed (12%) and peristaltic speed (20%) at day 12 (old age). Cocoa-supplementation improved the maximum amplitude which is an indicator of muscle strength at old age (day 12). The improvements in locomotion are consistent with previous results as epicatechin which is the most abundant flavonoid in cocoa has been reported to improve the muscle performance in mice and humans by stimulating mitochondrial biogenesis [32], enhancing biosynthesis of capillaries [33], enhancing myogenic differentiation [34] and reversing the age-related degeneration in the skeletal muscle [35]. In addition, catechin which is another flavanol found in cocoa has also been reported to have similar effects on skeletal muscle [36]. Flavanol procyanidin in cocoa has also shown to improve skeletal muscle function [37]. Interestingly, cocoa-supplementation seemed to be beneficial on locomotion only at later stages of life. We assume that this beneficial effect of cocoa supplementation at old age was achieved by reversing/reducing the age-related degeneration mechanisms in the skeletal muscle. The degenerative processes reversed/reduced by cocoa may include mitochondrial dysfunction [38] and increased ROS production [39] which are known to cause sarcopenia.
Cocoa-supplementation improved both mean and median lifespan of C. elegans, but the improvement was not dose-dependent. Interestingly, antioxidants including N-acetylcysteine, vitamin C and resveratrol induce an inverted U-shaped dose-response relationship between ROS levels and lifespan [40]. No significant effect was found on the maximum lifespan. A previous lifespan study has shown cocoa-supplementation to increase the average lifespan of Drosophila melanogaster under normoxia [41]. In the same study, cocoa increased average lifespan of D. melanogaster under hyperoxia or in a Cu/Zn-superoxide dismutase-deficient background, suggesting that it has strong antioxidant properties. Moreover, it has been previously reported that polyphenol-rich extracts of cocoa powder increased the lifespan of rats [42], Saccharomyces cerevisiae [43] and Oryzias latipes [44]. Conversely, catechin and epicatechin (two major flavanols in cocoa) showed no improvement in mean lifespan of C. elegans in another study [45]. However, epicatechin increased the maximum lifespan of C. elegans in the same study.
Survival under heat stress (thermotolerance) is dependent on the mechanisms contributing to proteostasis [46]. Aging alters proteostasis (1) thereby, declining the ability to cope with heat stress. Our study demonstrated that worms at old age (day 12) to have a significantly reduced survival rate compared to young age (day 4) and middle age (day 8). Exposure to cocoa powder significantly increased survival rate at all three ages, but the effect was less prominent with old worms (day 12). Therefore, results indicate that cocoa may improve the ability of C. elegans to deal with poor living environments at young and middle age better than the old age. Even though there are no previous studies to determine the effect of cocoa on thermotolerance of C. elegans, there are few studies which show the effect of some polyphenol compounds in cocoa. In one such study, the effects of epicatechin and catechin (two major flavanols in cocoa) on the thermal stress of C. elegans was evaluated [45]. Results indicate both epicatechin and catechin to improve survival in early adult stages (worms at 1st and 6th day of adulthood), with relatively greater protective effects in older (6th day of adulthood) than in young worms. However, our study showed a better protection at early stage (1st day of adulthood) which was gradually declined with age. In another study, the effect of epicatechin in aged worms (10th and 17th day of adulthood) was evaluated [47]. Epicatechin significantly increased the survival of worms, but the protective effect of epicatechin against heat stress was not increased in more aged worms (at 17th day of adulthood).
Loss of cognitive function is one of the most dev-astating age-related declines in humans. C. elegans chemotaxis behaviour, a neuronally-controlled process has been widely used to study the functional changes in neurons [48, 49]. Here, we used C. eleg-ans positive butanone learning and short-term memory assay [19] to determine age-related changes in neuronal function and the effects of cocoa-sup-plementation. Naive chemotaxis index which is the natural response of C. elegans towards butanone and the learning index which is an indicator of the extent that worms learn food-butanone association were measured. Moreover, we measured the short-term memory loss/dementia index (memory loss within 2 hours after conditioning). There was no significant effect of cocoa-supplementation at young (day 4) and middle age (day 8) on naive chemotaxis index. However, Cocoa supplementation significantly improved the learning ability of C. elegans at middle age (day 8). As cocoa-supplementation had no effect on motility parameters at day 8, the improvement in learning can be independent from locomotory improvements. Even though the improvement of dementia index at young age (day 4) with cocoa was not statistically significant, there was a 28%reduction after 2 hours from conditioning which suggest that cocoa can improve age-induced memory loss. No previous studies were found on the effect of cocoa on age-related decline of cognition in C. elegans. However, several animal models and human trials have examined the effects of cocoa on the onset of age-related cognitive deficits. Administration of a cocoa polyphenolic extract improved the cognitive impairments in rats caused by heat exposure [50]. In a similar study, high-flavanol cocoa has improved the cognitive performance in aged rats [42]. Consumption of cocoa-related products like chocolate has reduced the cognitive decline in adults who were not cognitively impaired [51]. Further, it has been reported that consumption of a drink containing cocoa flavanols to improve cognition in older people with mild cognitive impairment [52].
It has been reported that the decline in mitocho-ndrial function is related to many aging-related diseases [53, 54]. Accumulation of mitochondrial DNA (mtDNA) mutations and increased reactive oxygen species (ROS) production in aging is known to ca-use oxidative damage to cellular macromolecules which progressively results in mitochondrial dysfunction [2]. In this study, we used basal respiration, non-mitochondrial respiration (measured directly in worms) and the mitochondrial respiration (derived indirectly by deducting non mitochondrial respiration from basal respiration) to assess the age-related decline in mitochondrial function and the effects of cocoa-supplementation. We have shown that both basal and mitochondrial respiration of C. elegans declines with age. Conversely, non-mitochondrial respiration which is an index of oxygen consuming processes which are not associated with mitocho-ndria increased with age. A previous study showed non-mitochondrial respiration to increase in the presence of stressors, including ROS [55]. Our resu-lts revealed that cocoa supplementation increased both basal and mitochondrial respiration and decr-eased non-mitochondrial respiration at old age sug-gesting that cocoa improves the age-associated decline in mitochondrial function. In a previous study, a cocoa bean extract was shown to improve mitochondrial biogenesis via peroxisome prolifer-ator-activated receptor gamma (PPARγ)/PPARγ co-ativator-1a (PGC-1α) dependent signalling pathway in 1-methyl-4-phenyl-1,2,3,6- pyridine (MPP+) intoxicated human neuroblastoma cells (SHSY5Y) and decreased the ROS formation [56]. In another study, monomeric cocoa catechins significantly increased the mitochondrial respiratory function in INS-1 832/13 rat β-cells through the increased expression of components of the electron transport chain [57]. Another study showed that consumption of epicatechin rich chocolate and a beverage improved the structural alterations in skeletal muscle mitochondria in patients with stage II and III heart failure and type 2 diabetes mellitus [58].
Even though the processing with alkali in commercial cocoa powder manufacturing is known to reduce the phenolics substantially compared to unprocessed natural cocoa powder [59–62], there are some evidence that show about 40%of the natural level of flavanols is retained on average for lightly Dutched powders, and an average of 22%is retained in even moderately alkali processed cocoa powders [63]. Therefore, we thought to determine the TP, TF and the antioxidant activity of our cocoa powder as we attributed these beneficial effects of cocoa to cocoa polyphenols. We found our cocoa powder to have 27.01 mg GAE/g TP which is about 56%compared to natural cocoa powder which contains TP around 48.84 mg GAE/g [64]. In natural cocoa powder, the TF content is around 25.55 mg CE/g [65]. Our cocoa powder contained 10.13 mg CE/g of TF which was around 40%of the TF in natural cocoa powder. Moreover, our cocoa powder showed an antioxidant activity of 7.84 (mg GAE/g). Therefore, these results confirm that the cocoa powder we used in this experiment was not devoid in beneficial flavonoids and other polyphenols.
Flavanols, the major group of polyphenols in cocoa are composed of monomeric forms, (+)-catechin and (–)-epicatechin, and their oligomeric and polymeric forms, procyanidins. In humans, both monomeric flavanols and procyanidins are stable in the gastric environment and reach the small intestine with no changes. Around 20%of monomeric flavanols are rapidly absorbed in the small intestine, undergo rapid and extensive metabolization and circulate in the blood stream. The majority of unabsorbed flavanols reach the colon where they get bio-transformed to secondary metabolites by gut microbiota [66]. Catechin and epicatechin have been shown to cross the blood-brain barrier and accumulate in the brain suggesting their direct positive action on the brain which may include cognitive improvements and neuroprotective effects [67, 68].
As we added cocoa powder with E. coli, there is a possibility that E. coli to modify cocoa powder and produce different metabolites that are different from those present in cocoa powder. In humans, cocoa polyphenols are known to poorly absorbed in the intestine and most of them cannot reach the systemic circulation in their natural forms [66]. Microbial degradation of polyphenols in the colon is considered as the most effective way to produce secondary metabolites from these polyphenols which are bioavailable to enter circulation, reach the target organs and exhibit their activities [69]. Therefore, microbial modification doesn’t seem to affect the beneficial effects of polyphenols.
5Conclusion
In summary, cocoa supplementation of the standard E. coli diet of C. elegans protected age-associated decline in neuromuscular function, as indicated by improved locomotor function. Moreover, cocoa improved the thermotolerance of C. elegans at all stages of life, showing a more prominent effect at young and middle age. Cocoa showed beneficial effects on age-related learning deficits and short-term memory loss. Further, cocoa enhanced the mitochondrial function, especially in late life by significantly reducing the non-mitochondrial respiration. These beneficial effects can be attributed to the antioxidants in cocoa as evident by previous studies. However, further studies are required to identify the active compounds as well as mechanisms of action.
Conflict of interest
There are no conflicts to declare.
Funding
This study was supported by La Trobe University Postgraduate Research Scholarship (LTUPRS) and La Trobe University Full Fee Research Scholarship (LTUFFRS).
Acknowledgements
We would like to thank Dr. Barry Kitchen, Dr. Matthew Flavel and Avon Zhu from The Product Makers (Australia) Pty LTD (TPM) for their help with conducting the compositional analysis of cocoa powder. We also would like to thank Prof. Paul Fisher and Oana Sanislav from Department of Physiology, Anatomy and Microbiology, School of Life sciences, La Trobe University, Australia for allowing us to use the Seahorse XF Analyzer facility.
References
[1] | López-Otín C , Blasco MA , Partridge L , Serrano M , Kroemer G . The hallmarks of aging. Cell. (2013) ;153: (6):1194–217. |
[2] | Srivastava S . The Mitochondrial Basis of Aging and Age-Related Disorders. Genes (Basel). (2017) ;8: (12):398. |
[3] | Harman D . The biologic clock: the mitochondria? J Am Geriatr Soc. (1972) ;20: (4):145–7. |
[4] | Turrens JF . Mitochondrial formation of reactive oxygen species. J Physiol. (2003) ;552: (Pt 2):335–44. |
[5] | Feart C , Samieri C , Barberger-Gateau P . Mediterranean diet and cognitive function in older adults. Current Opinion in Clinical Nutrition and Metabolic Care. (2010) ;13: (1):14–8. |
[6] | Morris MC , Tangney CC , Wang Y , Sacks FM , Bennett DA , Aggarwal NT . MIND diet associated with reduced incidence of Alzheimer’s disease. Alzheimer’s & Dementia: The Journal of the Alzheimer’s Association. (2015) ;11: (9):1007–14. |
[7] | Scholey AB , French SJ , Morris PJ , Kennedy DO , Milne AL , Haskell CF . Consumption of cocoa flavanols results in acute improvements in mood and cognitive performance during sustained mental effort. (2010) ;24: (10):1505–14. |
[8] | Grosso G , Stepaniak U , Micek A , Kozela M , Stefler D , Bobak M , et al. Dietary polyphenol intake and risk of hypertension in the Polish arm of the HAPIEE study. Eur J Nutr. (2018) ;57: (4):1535–44. |
[9] | Cassidy A , Rimm EB , O’Reilly EJ , Logroscino G , Kay C , Chiuve SE , et al. Dietary flavonoids and risk of stroke in women. Stroke. (2012) ;43: (4):946–51. |
[10] | Vinson JA , Proch J , Bose P , Muchler S , Taffera P , Shuta D , et al. Chocolate Is a Powerful ex Vivo and in Vivo Antioxidant, an Antiatherosclerotic Agent in an Animal Model, and a Significant Contributor to Antioxidants in the European and American Diets. Journal of Agricultural and Food Chemistry. (2006) ;54: (21):8071–6. |
[11] | Lee KW , Kim YJ , Lee HJ , Lee CY . Cocoa Has More Phenolic Phytochemicals and a Higher Antioxidant Capacity than Teas and Red Wine. Journal of Agricultural and Food Chemistry. (2003) ;51: (25):7292–5. |
[12] | Field DT , Williams CM , Butler LT . Consumption of cocoa flavanols results in an acute improvement in visual and cognitive functions. Physiology & Behavior. (2011) ;103: (3):255–60. |
[13] | Mastroiacovo D , Kwik-Uribe C , Grassi D , Necozione S , Raffaele A , Pistacchio L , et al. Cocoa flavanol consumption improves cognitive function, blood pressure control, and metabolic profile in elderly subjects: the Cocoa, Cognition, and Aging (CoCoA) Study–a randomized controlled trial. The American Journal of Clinical Nutrition. (2015) ;101: (3):538–48. |
[14] | Gröne M , Sansone R , Höffken P , Horn P , Rodriguez-Mateos A , Schroeter H , et al. Cocoa Flavanols Improve Endothelial Functional Integrity in Healthy Young and Elderly Subjects. Journal of Agricultural and Food Chemistry. (2020) ;68: (7):1871–6. |
[15] | Petyaev IM , Klochkov VA , Chalyk NE , Pristensky DV , Chernyshova MP , Kyle NH , et al. Markers of Hypoxia and Oxidative Stress in Aging Volunteers Ingesting Lycosomal Formulation of Dark Chocolate Containing Astaxanthin. The Journal of Nutrition, Health & Aging. (2018) ;22: (9):1092–8. |
[16] | Munguia L , Rubio-Gayosso I , Ramirez-Sanchez I , Ortiz A , Hidalgo I , Gonzalez C , et al. High Flavonoid Cocoa Supplement Ameliorates Plasma Oxidative Stress and Inflammation Levels While Improving Mobility and Quality of Life in Older Subjects: A Double-Blind Randomized Clinical Trial. The Journals of Gerontology: Series A. (2019) ;74: (10):1620–7. |
[17] | Huang C , Xiong C , Kornfeld K . Measurements of age-related changes of physiological processes that predict lifespan of Caenorhabditis elegans. Proc Natl Acad Sci U S A. (2004) ;101: (21):8084–9. |
[18] | Sutphin GL , Kaeberlein M . Measuring Caenorhabditis elegans life span on solid media. Journal of visualized experiments: JoVE. 2009: (27): 1152. |
[19] | Kauffman A , Parsons L , Stein G , Wills A , Kaletsky R , Murphy C . C. elegans positive butanone learning, short-term, and long-term associative memory assays. J Vis Exp. 2011(49). |
[20] | Koopman M , Michels H , Dancy BM , Kamble R , Mouchiroud L , Auwerx J , et al. A screening-based platform for the assessment of cellular respiration in Caenorhabditis elegans. Nature Protocols. (2016) ;11: (10):1798–816. |
[21] | Luz AL , Smith LL , Rooney JP , Meyer JN . Seahorse Xfe 24 Extracellular Flux Analyzer-Based Analysis of Cellular Respiration in Caenorhabditis elegans. Curr Protoc Toxicol. 2015;66:25.7.1-15. |
[22] | AOAC. AOAC Official Method 963.15 - Fat in Cacao Products. Official Methods of Analysis AOAC International. 16 ed. Washington DC, USA: Association of official analytical chemists; 1995. |
[23] | Kim DO , Chun OK , Kim YJ , Moon HY , Lee CY . Quantification of polyphenolics and their antioxidant capacity in fresh plums. J Agric Food Chem. (2003) ;51: (22):6509–15. |
[24] | Zhishen J , Mengcheng T , Jianming W . The determination of flavonoid contents in mulberry and their scavenging effects on superoxide radicals. Food Chemistry. (1999) ;64: (4):555–9. |
[25] | Re R , Pellegrini N , Proteggente A , Pannala A , Yang M , Rice-Evans C . Antioxidant activity applying an improved ABTS radical cation decolorization assay. Free Radical Biology and Medicine. (1999) ;26: (9):1231–7. |
[26] | Lenaerts I , Walker GA , Van Hoorebeke L , Gems D , Vanfleteren JR . Dietary restriction of Caenorhabditis elegans by axenic culture reflects nutritional requirement for constituents provided by metabolically active microbes. J Gerontol A Biol Sci Med Sci. (2008) ;63: (3):242–52. |
[27] | Glenn CF , Chow DK , David L , Cooke CA , Gami MS , Iser WB , et al. Behavioral deficits during early stages of aging in Caenorhabditis elegans result from locomotory deficits possibly linked to muscle frailty. The Journals of Gerontology Series A, Biological Sciences and Medical Sciences. (2004) ;59: (12):1251–60. |
[28] | Zembron-Lacny A , Dziubek W , Rogowski L , Skorupka E , Dabrowska G . Sarcopenia: monitoring, molecular mechanisms, and physical intervention. Physiol Res. (2014) ;63: (6):683–91. |
[29] | Hahm JH , Kim S , DiLoreto R , Shi C , Lee SJ , Murphy CT , et al. C. elegans maximum velocity correlates with healthspan and is maintained in worms with an insulin receptor mutation. Nat Commun. (2015) ;6: :8919. |
[30] | Nahabedian JF , Qadota H , Stirman JN , Lu H , Benian GM . Bending amplitude - a new quantitative assay of C. elegans locomotion: identification of phenotypes for mutants in genes encoding muscle focal adhesion components. Methods. (2012) ;56: (1):95–102. |
[31] | Rollins JA , Howard AC , Dobbins SK , Washburn EH , Rogers AN . Assessing Health Span in Caenorhabditis elegans: Lessons From Short-Lived Mutants. The Journals of Gerontology: Series A. (2017) ;72: (4):473–80. |
[32] | Moreno-Ulloa A , Miranda-Cervantes A , Licea-Navarro A , Mansour C , Beltrán-Partida E , Donis-Maturano L , et al. (-)-Epicatechin stimulates mitochondrial biogenesis and cell growth in C2C12 myotubes via the G-protein coupled estrogen receptor. Eur J Pharmacol. (2018) ;822: :95–107. |
[33] | Nogueira L , Ramirez-Sanchez I , Perkins GA , Murphy A , Taub PR , Ceballos G , et al. (–)-Epicatechin enhances fatigue resistance and oxidative capacity in mouse muscle. J Physiol. (2011) ;589: (Pt 18):4615–31. |
[34] | Gutierrez-Salmean G , Ciaraldi TP , Nogueira L , Barboza J , Taub PR , Hogan MC , et al. Effects of (–)-epicatechin on molecular modulators of skeletal muscle growth and differentiation. J Nutr Biochem. (2014) ;25: (1):91–4. |
[35] | Si H , Wang X , Zhang L , Parnell LD , Admed B , LeRoith T , et al. Dietary epicatechin improves survival and delays skeletal muscle degeneration in aged mice. FASEB J. (2019) ;33: (1):965–77. |
[36] | Li P , Liu A , Xiong W , Lin H , Xiao W , Huang J , et al. Catechins enhance skeletal muscle performance. Critical Reviews in Food Science and Nutrition. (2020) ;60: (3):515–28. |
[37] | Xu M , Chen X , Huang Z , Chen D , Chen H , Luo Y , et al. Procyanidin B2 Promotes Skeletal Slow-Twitch Myofiber Gene Expression through the AMPK Signaling Pathway in C2C12 Myotubes. Journal of Agricultural and Food Chemistry. (2020) ;68: (5):1306–14. |
[38] | Short KR , Bigelow ML , Kahl J , Singh R , Coenen-Schimke J , Raghavakaimal S , et al. Decline in skeletal muscle mitochondrial function with aging in humans. Proc Natl Acad Sci U S A. (2005) ;102: (15):5618–23. |
[39] | Broome CS , Kayani AC , Palomero J , Dillmann WH , Mestril R , Jackson MJ , et al. Effect of lifelong overexpression of HSP70 in skeletal muscle on age-related oxidative stress and adaptation after nondamaging contractile activity. FASEB J. (2006) ;20: (9):1549–51. |
[40] | Desjardins D , Cacho-Valadez B , Liu J-L , Wang Y , Yee C , Bernard K , et al. Antioxidants reveal an inverted U-shaped dose-response relationship between reactive oxygen species levels and the rate of aging in Caenorhabditis elegans. Aging cell. (2017) ;16: (1):104–12. |
[41] | Bahadorani S , Hilliker AJ . Cocoa confers life span extension in Drosophila melanogaster. Nutr Res. (2008) ;28: (6):377–82. |
[42] | Bisson J-F , Nejdi A , Rozan P , Hidalgo S , Lalonde R , Messaoudi M . Effects of long-term administration of a cocoa polyphenolic extract (Acticoa powder) on cognitive performances in aged rats. British Journal of Nutrition. (2008) ;100: (1):94–101. |
[43] | Baiges I , Arola L . COCOA (Theobroma cacao) Polyphenol-Rich Extract Increases the Chronological Lifespan of Saccharomyces cerevisiae. J Frailty Aging. (2016) ;5: (3):186–90. |
[44] | Sánchez-Sánchez AV , Leal-Tassias A , Rodríguez-Sánchez N , Piquer-Gil M , Martorell P , Genovés S , et al. Use of Medaka Fish as Vertebrate Model to Study the Effect of Cocoa Polyphenols in the Resistance to Oxidative Stress and Life Span Extension. Rejuvenation Res. (2018) ;21: (4):323–32. |
[45] | Surco-Laos F , Dueñas M , González-Manzano S , Cabello J , Santos-Buelga C , González-Paramás AM . Influence of catechins and their methylated metabolites on lifespan and resistance to oxidative and thermal stress of Caenorhabditis elegans and epicatechin uptake. Food Research International. (2012) ;46: (2):514–21. |
[46] | Balch WE , Morimoto RI , Dillin A , Kelly JW . Adapting proteostasis for disease intervention. Science (New York, NY). (2008) ;319: (5865):916–9. |
[47] | Ayuda-Durán B , González-Manzano S , Miranda-Vizuete A , Dueñas M , Santos-Buelga C , González-Paramás AM . Epicatechin modulates stress-resistance in C. elegans via insulin/IGF-1 signaling pathway. PLoS One. (2019) ;14: (1):e0199483. |
[48] | Kauffman AL , Ashraf JM , Corces-Zimmerman MR , Landis JN , Murphy CT . Insulin signaling and dietary restriction differentially influence the decline of learning and memory with age. PLoS Biol. (2010) ;8: (5):e1000372–e. |
[49] | Stein GM , Murphy CT . C. elegans positive olfactory associative memory is a molecularly conserved behavioral paradigm. Neurobiology of Learning and Memory. (2014) ;115: :86–94. |
[50] | Rozan P , Hidalgo S , Nejdi A , Bisson JF , Lalonde R , Messaoudi M . Preventive antioxidant effects of cocoa polyphenolic extract on free radical production and cognitive performances after heat exposure in Wistar rats. J Food Sci. (2007) ;72: (3):S203–6. |
[51] | Moreira A , Diógenes MJ , de Mendonça A , Lunet N , Barros H . Chocolate Consumption is Associated with a Lower Risk of Cognitive Decline. J Alzheimers Dis. (2016) ;53: (1):85–93. |
[52] | Desideri G , Kwik-Uribe C , Grassi D , Necozione S , Ghiadoni L , Mastroiacovo D , et al. Benefits in cognitive function, blood pressure, and insulin resistance through cocoa flavanol consumption in elderly subjects with mild cognitive impairment: the Cocoa, Cognition, and Aging (CoCoA) study. Hypertension. (2012) ;60: (3):794–801. |
[53] | Chen C , Turnbull DM , Reeve AK . Mitochondrial Dysfunction in Parkinson’s Disease-Cause or Consequence? Biology (Basel). (2019) ;8: (2). |
[54] | Weidling I , Swerdlow RH . Mitochondrial Dysfunction and Stress Responses in Alzheimer’s Disease. Biology. (2019) ;8: (2):39. |
[55] | Dranka BP , Hill BG , Darley-Usmar VM . Mitochondrial reserve capacity in endothelial cells: The impact of nitric oxide and reactive oxygen species. Free Radic Biol Med. (2010) ;48: (7):905–14. |
[56] | Chidambaram SB , Bhat A , Ray B , Sugumar M , Muthukumar SP , Manivasagam T , et al. Cocoa beans improve mitochondrial biogenesis via PPARγ/PGC1α dependent signalling pathway in MPP(+) intoxicated human neuroblastoma cells (SH-SY5Y). Nutr Neurosci. (2018) :1–10. |
[57] | Rowley TJt , Bitner BF , Ray JD , Lathen DR , Smithson AT , Dallon BW , et al. Monomeric cocoa catechins enhance β-cell function by increasing mitochondrial respiration. J Nutr Biochem. (2017) ;49: :30–41. |
[58] | Taub PR , Ramirez-Sanchez I , Ciaraldi TP , Perkins G , Murphy AN , Naviaux R , et al. Alterations in skeletal muscle indicators of mitochondrial structure and biogenesis in patients with type 2 diabetes and heart failure: effects of epicatechin rich cocoa. Clin Transl Sci. (2012) ;5: (1):43–7. |
[59] | Robinson T , Ranalli AW , Phillips AW . Cocoa Polyphenols, Changes in Cocoa Tannins during Processing. Journal of Agricultural and Food Chemistry. (1961) ;9: (4):295–8. |
[60] | Kealey Kirk S , Snyder Rodney M , Romanczyk Leo J Jr , Hammerstone John F Jr , Buck Margaret M , Cipolla Giovanni G , inventors; MARS INC, assignee. Method for producing fat and/or solids from cocoa beans1996 1996/09/06/Application date. |
[61] | Gu L , House SE , Wu X , Ou B , Prior RL . Procyanidin and catechin contents and antioxidant capacity of cocoa and chocolate products. J Agric Food Chem. (2006) ;54: (11):4057–61. |
[62] | Andres-Lacueva C , Monagas M , Khan N , Izquierdo-Pulido M , Urpi-Sarda M , Permanyer J , et al. Flavanol and flavonol contents of cocoa powder products: influence of the manufacturing process. J Agric Food Chem. (2008) ;56: (9):3111–7. |
[63] | Miller KB , Hurst WJ , Payne MJ , Stuart DA , Apgar J , Sweigart DS , et al. Impact of Alkalization on the Antioxidant and Flavanol Content of Commercial Cocoa Powders. Journal of Agricultural and Food Chemistry. (2008) ;56: (18):8527–33. |
[64] | Ünver GD , Baz1ne1rm1 A , Bozplu F , Z1le1s1m Ö , Soyer Y , Didj MC , et al. editors. INVESTIGATION OF NATURAL ANDPROCESSED COCOAANDCOCOACONTAINING PRODUCTS FOR THEIR ANTIOXIDANT CAPACITY AND PHENOLIC COMPOUNDS2015. |
[65] | Todorovic V , Milenkovic M , Vidovic B , Todorovic Z , Sobajic S . Correlation between Antimicrobial, Antioxidant Activity, and Polyphenols of Alkalized/Nonalkalized Cocoa Powders. Journal of Food Science. (2017) ;82: (4):1020–7. |
[66] | Sorrenti V , Ali S , Mancin L , Davinelli S , Paoli A , Scapagnini G . Cocoa Polyphenols and Gut Microbiota Interplay: Bioavailability, Prebiotic Effect, and Impact on Human Health. Nutrients. (2020) ;12: (7):1908. |
[67] | Faria A , Pestana D , Teixeira D , Couraud PO , Romero I , Weksler B , et al. Insights into the putative catechin and epicatechin transport across blood-brain barrier. Food Funct. (2011) ;2: (1):39–44. |
[68] | Abd El Mohsen MM , Kuhnle G , Rechner AR , Schroeter H , Rose S , Jenner P , et al. Uptake and metabolism of epicatechin and its access to the brain after oral ingestion. Free Radic Biol Med. (2002) ;33: (12):1693–702. |
[69] | Rechner AR , Smith MA , Kuhnle G , Gibson GR , Debnam ES , Srai SK , et al. Colonic metabolism of dietary polyphenols: influence of structure on microbial fermentation products. Free Radic Biol Med. (2004) ;36: (2):212–25. |