Skin aging as a mechanical phenomenon: The main weak links
Abstract
From a mechanical point of view, human skin appears as a layered composite containing the stiff thin cover layer presented by the stratum corneum, below which are the more compliant layers of viable epidermis and dermis and further below the much more compliant adjacent layer of subcutaneous white adipose tissue (sWAT). Upon exposure to a strain, such a multi-layer system demonstrates structural instabilities in its stiffer layers, which in its simplest form is the wrinkling. These instabilities appear hierarchically when the mechanical strain in the skin exceeds some critical values. Their appearance is mainly dependent on the mismatch in mechanical properties between adjacent skin layers or between the skin and sWAT, on the adhesive strength and thickness ratios between the layers, on their bending and tensile stiffness as well as on the value of the stress existing in single layers. Gradual reduction of elastic fibers in aging significantly reduces the skin’s ability to bend, prompting an up to 4-fold reduction of its stability against wrinkling, thereby explaining the role of these fibers in skin aging. While chronological and extrinsic aging differently modify these parameters, they lead to the same end result, reducing the critical strain required for the onset of instabilities. Comparing of mechanical properties of the skin presented as a bi-, tri- or tetra-layer structure demonstrates the particular importance of the papillary dermis in skin aging and provides the arguments to consider the undulations on the dermal-epidermal and dermal-sWAT interfaces as the result of mechanical bifurcation, leading to structural instabilities inside of the skin. According to this model, anti-aging strategies should focus not as much on the reinforcement of the dermis, but rather aim to treat the elastic mismatch between different adjacent layers in the skin and sWAT as well as the adhesion between these layers.
1Introduction
Skin wrinkling is one of the most important and prominent signs of the aging skin. Appearance of epidermal wrinkles in human skin was connected with elastic properties of a thin epidermal layer, including a very stiff stratum corneum (SC) sub-layer placed on a much more compliant and thick dermis [1, 2]. From the physics of failure mechanics, it is known that a stiff thin film adhered to a thick compliant pre-stressed substrate can undergo mechanical deformations leading to production of different structural instabilities [3, 4]. This model generally correlates with the human skin structure: skin is pre-stressed, the Young’s modulus describing the strain response of materials to the uniaxial stress is much bigger in the SC than in the dermis [5], and the typical thickness of the SC is 50–100 times smaller than the thickness of the dermis.
It is well known that the appearance of structural instabilities in thin films placed on the top of the laminate-like structure is dependent on several dimensionless parameters, such as the elastic mismatch describing the relative difference between the Young’s moduli in adjacent layers, relative thicknesses of the substrate and the film, as well as the ratios of tensile and bending stiffness between these layers. An increase of elastic mismatch between the layers can quickly reduce the critical strain in the film required for the induction of instabilities [4]. From here, any standalone mechanical reinforcement of the dermis increasing its Young’s modulus should reduce the elastic mismatch between this layer and the epidermis and thus increase the structural stability of the skin. Variation of the film thickness should also significantly influence its mechanical stability [6]. Additionally, an increase of the SC thickness by the same thickness of the dermis should increase the critical strain in the skin required for the appearance of the wrinkles.
Contrarily to this model, topical long-term application of retinoic acid, which is one of the best investigated substances claimed to be effective in anti-aging, was reported not to increase but decrease the stiffness of the dermis [7]. Similar effects were observed for the local temperature increase in the skin, used in different anti-aging procedures [8]. For example, application of supra-physiological temperatures reduces the Young’s modulus of the skin by about 30% at 45°C and even by more than 50% at 50°C [9]. Moreover, the thickness of the facial SC [10] and its Young’s modulus [5] demonstrate only weak correlations with aging. In contrast, the thickness of the viable epidermis is reduced and the thickness of the dermis remains almost unchanged in aging skin. These results contradict the main postulate of anti-aging, which states that the reinforcement and thickening of dermis should be the main target for the improvement of the skin appearance in aging.
An examination of the literature of the age-dependency of the dermal Young’s modulus provides contradictory information: some authors found this modulus to be increased in the aged skin [11–15], others reported it to be decreased [16–19]. This could mean that the skin aging is not implicitly connected with the loss of the skin stiffness, as it is traditionally assumed in skin aging theories. Additionally, skin architecture, which is geometrically connected with the epidermis, is also strongly modified during aging [16, 18], and it was even proposed that this architecture hampers the appearance of large wrinkles, thus making the skin surface more stable against structural instabilities [20].
Human skin cannot be considered as a simple mechanical bi-layer: stiffness of the deep skin containing the reticular dermis was reported to be much bigger than the corresponding mechanical modulus of the upper skin containing the viable epidermis and papillary dermis, but much lower than the stiffness of the SC layer [21–23]. Accordingly, human skin must be described as a tri- or even tetra-layer composite, and different authors considered such multi-layer models which were solved numerically for some given Young’s moduli and thicknesses of different skin layers. Absolute values of the Young’s moduli in various skin layers can vary by orders of magnitude depending on the measurement procedure. Values can also demonstrate high inter-subject variations, hence explaining the many contradictive opinions. For example, analyzing a tetra-layer based mechanical model of the skin, Kuwazuru et al. came to conclusion that the appearance of skin wrinkles should be mainly caused by “epidermal aging” connected with stiffening and thinning of this skin layer [22, 23]. This result was generally based on the model assumption that the Young’s modulus of the SC increases twice in the old skin, which is, however, not supported by direct measurements provided in [5].
Recently, it was theoretically shown that the appearance of a compliant intermediate layer under the stiff film can significantly contribute to the onset of film instabilities [24]. Since the reticular dermis is much stiffer than the papillary dermis, induced instabilities cannot propagate through the total skin depth and should be mainly restricted to the papillary dermis which, in the facial skin, has a thickness of about 20–30μm [25]. Contrary to this, a closer microscopic examination of cutaneous wrinkles reveals that not only the SC/epidermis/dermis layers, but also the adjacent subcutaneous white adipose tissue (sWAT) should be involved in formation of deep wrinkles. As shown in [26, 27], appearance of shallow wrinkles having the depth of up to 150μm (thus restricted to the papillary dermis) does not influence the dermal thickness and does not lead to any deformation of the interface between the dermis and sWAT. On the other hand, the appearance of deep wrinkles correlates with downward buckling of this interface and with sufficient reduction of the dermal thickness at the wrinkle point (Fig. 1).
Structure of the sWAT layer is also modified in aging. This modification is mainly connected with the loss of collagen network which significantly influences the Young’s modulus in the sWAT [28, 29]. Recently, we have proposed that sWAT, and especially its superficial layer known as dermal white adipose tissue (dWAT), can mechanically interact with the dermis and thus should be involved in skin aging [29, 30]. Indeed, it was reported that skin stiffness in mice is inversely dependent on the dWAT thickness [31], which is significantly reduced in aging. Different groups reported the reduction of dWAT also after chronic UV-B irradiation, which was accompanied by increased deposition of insoluble collagen substituting for adipose tissue and causing an increase of the thickness of the dermis [32, 33]. Very recently, we have proposed that UV radiation can induce an “adipocyte-myofibroblast transition” (AMT) in the superficial layer of the sWAT and that these myofibroblasts can produce fibrotic structures, locally substituting adipose tissue [29, 34]. All these biological processes can additionally change the mechanical properties of aging skin.
Fig. 1
Typical wrinkles in human skin: a – superficial wrinkles; b – deep wrinkles (modified after [26]).
![Typical wrinkles in human skin: a – superficial wrinkles; b – deep wrinkles (modified after [26]).](https://content.iospress.com:443/media/nha/2018/4-4/nha-4-4-nha170037/nha-4-nha170037-g001.jpg)
The role of sWAT in skin aging was considered in several theoretical models. The multi-layer skin model described in [22, 23] assumed that the sWAT layer does not change its geometrical and mechanical characteristics in aging, which accordingly led in numerical calculations to the conclusion that this layer does not influence skin wrinkling. In contrast, the tri-layer model epidermis/dermis/sWAT, described in [35, 36], proposed that the mechanical properties of the sWAT layer can indeed affect the wrinkle formation. At the same time, this model revealed that an increase of the dermal collagen density should result in enhanced severity of appearing wrinkles. This effect evidently contradicts the main paradigm of dermal reinforcement prevailing in the anti-aging field.
While the Young’s moduli measured in different skin layers demonstrate high variations even among samples taken from the same body location and investigated with the same methodology, only the relative ratios of these moduli obtained in the same study can be considered as reliable.
Here we present the analytical results concerning the onset of structural instabilities in the skin described as a multi-layer composite. For this, we will consider the critical strain required for the appearance of instabilities in such multi-layer systems as a main parameter and will analyze how the thicknesses, stiffness and adhesive properties of single layers influence this strain. Comparing critical strains in composites of various structures allows to determine the weak links in such a system, which are mainly responsible for the onset of instabilities. Results obtained will be further applied to discuss the process of skin wrinkling in aging.
2Skin and sWAT as multi-layer mechanical composites with adherence between the layers
2.1Layered structure of the composite skin/sWAT
In a general case, human skin must be considered as a multi-layer composite containing the SC/viable epidermis/papillary dermis/reticular dermis layers with different geometrical characteristics and mechanical properties (Fig. 2). Further, we are describing the single layers i of such composite by their thicknesses, hi, Young’s moduli, Ei, and Poisson’s ratios, νi. Such a description limits further consideration of mechanical loadings with low strains and low strain rates and neglects the anisotropic and viscoelastic properties of the skin.
Typical average thicknesses of the facial SC layer, hsc, epidermis, he, papillary dermis, hpd, and reticular dermis, hrd, are about 15–20μm, 100–150μm, 20–30μm, and 1–1.5 mm, respectively [37]; the thickness of the adjacent sWAT layer, hf, can be several fold bigger than the thickness of the dermis, which is hd = hpd + hrd. Typical thickness ratios ηesc = he/hsc, ηde = hd/he, and ηfd = hf/hd in the facial skin are about 10–15, 10–15, and 2-3, respectively.
Fig. 2
Layered composite skin/sWAT with adherences between single layers.
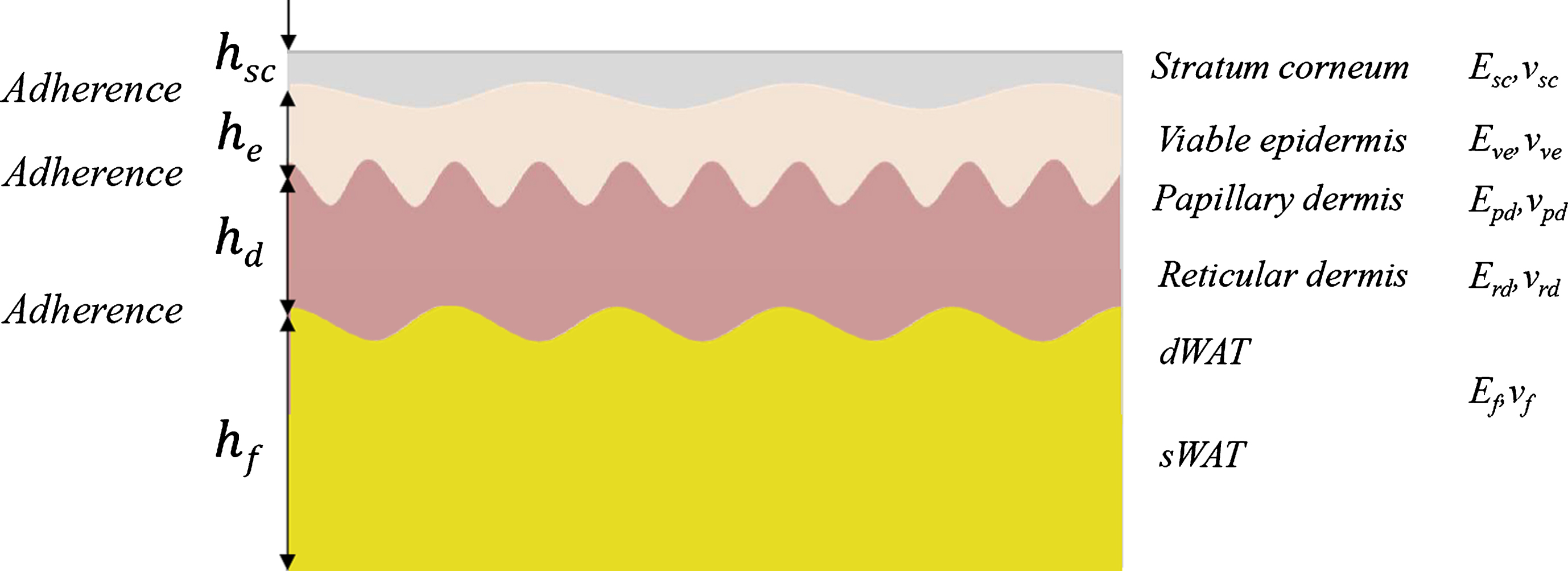
The thickness of single layers demonstrate significant variations even within a specific site. Application of reflectance confocal microscopy revealed the within-site variations of SC and epidermis thicknesses of 59% and 49%, respectively [38]. This should be connected with the fact that the interfaces between the single layers in the composite skin/sWAT are not flat and demonstrate typical undulations. These undulations as well as their reduction and smoothening in aging are well-known for the dermal-epidermal interface where they are named rete ridges or papillae. Such deformations of the interfacial area are strongly connected with mechanical properties of the papillary dermis which appears to be much more compliant than the adjacent epidermal layer. Similar undulations were also described on the dermal-sWAT interface and were connected with existence of the special dWAT sub-layer [29], which is supposed to be much more compliant than the adjacent reticular dermis. Whereas such undulations were not directly described for the SC-viable epidermis interface, their existence should be also strongly assumed based on the high within-site variations of SC thickness observed in [38].
Adherence between adjacent layers does not allow them to slide past each other under mechanical deformation, thus causing the separate layers to have very different mechanical properties to a single laminated composite. When the adherence between the layers is weak, they fail to exchange the mechanical energy with each other and thus cannot produce the single composite with some effective mechanical properties. Adhesive strength was shown to be an important parameter defining the appearance and propagation of structural instabilities in laminate structures [3], which makes it especially important for the description of the mechanical properties of the skin in aging.
The Young’s modulus of the SC layer, Esc, is three to four orders of magnitude bigger than the corresponding modulus of the dermis, which, in turn, is much bigger than the Young’s modulus of the adjacent sWAT layer. At low strain rates, it can generally be assumed that Ef << Erd << Esc. Typical reported values for the Young’s moduli are 1–20 kPa in the sWAT [39–41], and 30–100 kPa in the dermis [42, 43], whereas the absolute values are strongly dependent on the measurement procedure. For example, the Young’s moduli of SC and dermis obtained with suction methods were reported to be about 2 MPa and 66 kPa, respectively [5].
The ratio between the Young’s moduli in adjacent skin layers strongly depends on the strain rates applied to the composite. At the strain rates of 0–10 s–1, this modulus in porcine skin was reported to be about 1000-fold bigger than in the sWAT, at the strain rates over 1,000 s–1 it was just about 7-fold bigger [41]. Additionally, mechanical pre-loading can significantly influence the Young’s modulus; this effect is especially pronounced in tissues having high content of fibrotic structures [39].
2.2Pre-stress conditions in the skin
Normally, both the skin and the sWAT are subject to different types of external thermic and mechanical stresses, and the composite SC/epidermis/dermis/sWAT can demonstrate different reactions depending on the stress type [44]. At the same time, these layers are pre-stressed, i.e. they are under stress even without application of external mechanical forces. Human skin in vivo is normally in a state of tension [35, 36], whereas the absolute values of this tension are spatially heterogenous. Loss of tension in aging skin was connected with reduction of underlying sWAT layer [45] and it was supposed that this process can influence the wrinkle appearance in the skin. Skin can be additionally stressed, for example, through local inflammation or through UV irradiation, which can modify its mechanical properties in a dose- and time-dependent manner [46, 47].
Stress conditions in the sWAT layer are connected with its expansion or reduction. Expansion of the sWAT is generally caused by such processes as local inflammation or application of cold or heat, whereas its reduction is typical, for example, in involution and in development of local fibrosis [48–50].
Expansion or reduction of the sWAT layer can produce additional stress in the skin. Such effect should be especially pronounced in sWAT depots having the compartmental and thus spatially limited structure, which is the case for the facial sWAT [51]. Boundaries between different facial fat compartments consist of fibrous septae. These fibrotic structures not only separate distinct compartments, but also clamp the fat tissue at the compartments’ edges, thus producing additional mechanical stress in sWAT.
Different facial fat compartments have distinct structures, whereas generally two main types of them can be described according to [52]. Fat compartments of the type 1 are characterized by a loosed interface and relatively weak adherence between the skin and underlying sWAT, which can be anatomically easily separated from each other. Such type of adherence was found in the medial and lateral midface, in some parts of the periorbital area, in the temple, forehead, and neck [51]. SWAT in these compartments was classified as “structural” adipose tissue. Fat compartments of the type 2 are characterized by a strong adherence between the skin and the sWAT and were found in the perioral and nasal areas as well as in the proximity of the eyebrows. SWAT in these compartments was classified as “fibrous” adipose tissue. Thus, different facial fat compartments demonstrate various mechanical properties and must produce different mechanical stress in a covering skin. Less is known about the “vertical” correlation of collagen content and of corresponding Young’s moduli in adjacent areas of reticular dermis and sWAT, whereas it can be strongly assumed that such correlations exist.
3Structural instabilities in different multi-layer skin models
3.1A thin stiff film on a thick compliant substrate
Different structural instabilities can appear in a stiff thin film adhered to a thick compliant pre-strained substrate (further, SFCS model) after this substrate was released. Such instabilities were described for the substrates with elastic [53] or viscoelastic [6] properties, having infinite or finite thickness [54, 55], and appearing as a single layer or a bi-layer structure [56]. Structural instabilities occur when the strain in the film exceeds some critical value, and they demonstrate a hierarchy in their appearance [3, 57]. The occurrence of these structures and their propagation reduces the strain energy in the stiff film, which is transferred to a more compliant substrate to minimize the total energy of the composite. Of note, the type of appearing instability is strongly dependent on the type of mechanical stress – tensile or compressive – applied to the film. Recently, this model was extended to the case of SFCS containing the fiber-reinforced layers [58] as well as to the case of a soft interfacial layer located between the film and the substrate [24], which are both the typical features of the composite SC/epidermis/dermis/sWAT.
Fig. 3
Bi-layer composite epidermis/dermis.
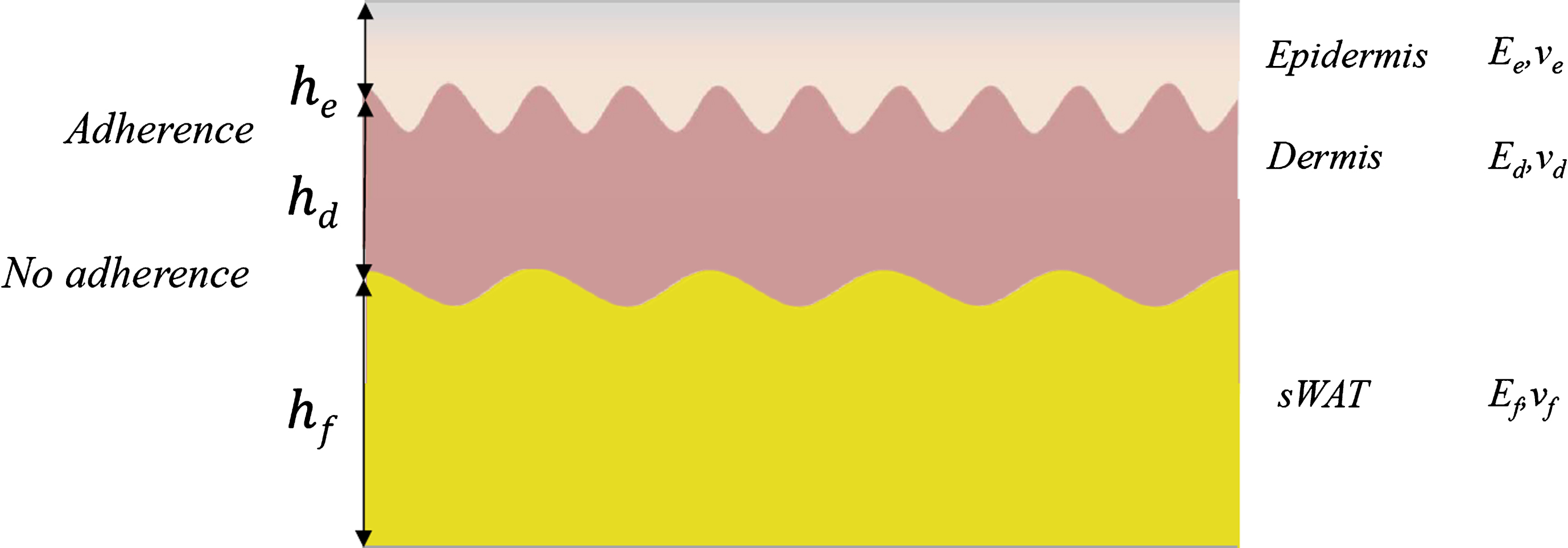
Mechanical stability of the film in the SFCS model is dependent on different dimensionless parameters. For such instability as wrinkles which appear under application of tensile stress to the film, these are the ratios of stiffness,
Generally, every system can be destroyed by deformations which are strong enough. Because of this, critical strains required for the loss of structural stability is the most important parameter providing the possibility to compare different systems under various loading conditions. Further, we are concentrating our discussion on the critical strain to compare the influence of different structural units of the layered skin on its stability.
3.2Epidermal instabilities appearing in a bi-layer model epidermis/dermis with and without bending
The SFCS model allows a simple analytical solution for the critical strain,
This description corresponds to the skin/sWAT model presented in Fig. 2 where the layers 1 and 2 are combined into an effective stiff “epidermal” layer, and the layers 3 and 4 produce an effective compliant “dermis” layer (Fig. 3). In this model, sWAT layer is considered to have no adherence to the dermis, which excludes this layer from a mechanical description. The critical strain required for the appearance of epidermal wrinkles
(1)
Expression (1) provides the critical strain required for the skin wrinkling of
Generalization of the bi-layer SFCS model to the case of the substrate of a finite thickness was provided in [54, 55]. One important prediction from these models is that the behavior of a thin film on a finite thickness substrate is mainly dependent on the bending stiffness ratio between the layers, which for the bi-layer epidermis/dermis is
(2)
Fig. 4
Wrinkling in the bi-layer skin model with bending: a – bi-layer composite epidermis/dermis; b – bending of this composite; c – wrinkling of the bended composite (modified after [55]).
![Wrinkling in the bi-layer skin model with bending: a – bi-layer composite epidermis/dermis; b – bending of this composite; c – wrinkling of the bended composite (modified after [55]).](https://content.iospress.com:443/media/nha/2018/4-4/nha-4-4-nha170037/nha-4-nha170037-g004.jpg)
Critical strain in epidermis required for its wrinkling can be found from the minimization of the potential energy of the system epidermis/dermis including the bending energy and the strain energy in the epidermis and the dermis with respect to the strain. The result is strongly dependent on the bending ability of the skin. If skin bending is not allowed, after straightforward calculations similar to [55], the critical strain can be presented in the form
(3)
From (3), the critical strain quickly reduces with increasing thickness ratio ηde = hd/he and reaches the value of
If the skin is able to undergo arbitrary bending deformation, the critical strain for wrinkling will be much higher. Its theoretically maximum value is
(4)
Expression (4) shows an interesting property of such layered skin composite: bending can increase the critical strain up to 4-fold, making the appearance of epidermal wrinkles under physiological conditions almost impossible. The reason for this is that bending of the film and substrate is energetically more favorable than their wrinkling; consequently, skin bending must take place before the onset of its wrinkling (Fig. 4). Thus, when such a layered system as human skin can bend, it will first pump the energy into the bending modes and does not produce the structural instabilities on its surface. From the physical point of view, bending is the first and wrinkling is the second order bifurcation taking place in such layered composite.
Fig. 5
Tri-layer skin model containing an intermediate compliant layer.
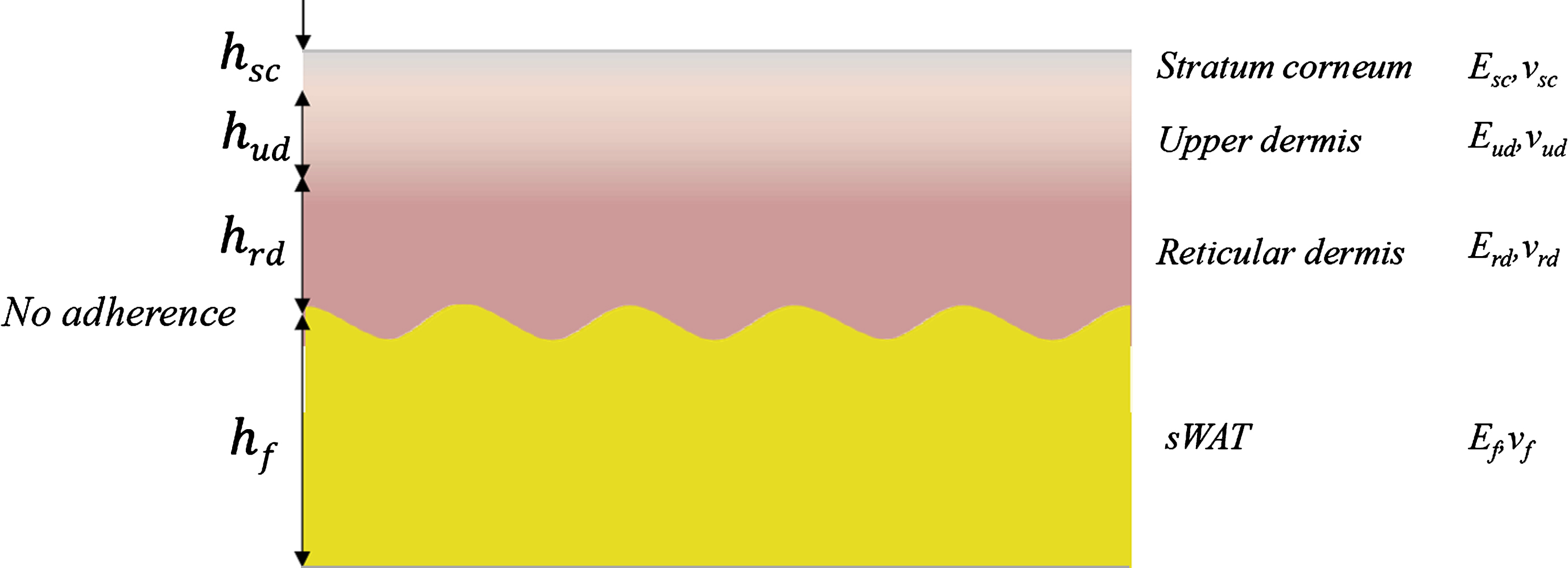
It should be noted that the expression (4) is valid only for the case of the optimal bending, since it was obtained by minimization of the potential energy with respect to the substrate curvature, i.e. it was assumed that the substrate can be deformed to any degree. The optimal curvature leading to the maximal stability of the layered system film/substrate can be calculated from minimization of the total potential energy of the system with respect to the skin curvature, which provides the following result:
(5)
Here we have taken into account the expression (4) and the relationship between the tensile stiffness in two skin layers:
To bend, the dermal layer should contain elastic fibers which support the skin deformation. Gradual loss of these fibers in aging must lead to continuous reduction of the strain threshold required for the onset of structural instabilities. Concentration of elastic fibers in the dermis is indeed gradually reduced in aging skin [59, 60]. This process leads to corresponding gradual reduction of the critical strain from its maximum value described by (4) to the minimum value in the case of no bending described by (3), thus making the aging skin less resistant to wrinkling. This special effect, which to our best knowledge was not considered before, can significantly contribute to the development of structural instabilities in aging skin.
In this context, it should be noted that the increment of the underlaying sWAT layer leads to enlargement of adipocytes, and this effect correlates with increased degradation of dermal elastic fibers [61, 62]. This mechanism demonstrates how the sWAT layer can directly influence the mechanical properties of the skin.
To assess the plausibility of these results, we need the information concerning the natural strains existing in the skin. This parameter was calculated in [13, 27] from the data for the initial stress and Young’s modulus in different age and gender groups and had the average value of about 6% for the group of 30–40 years old females. In this context, it should be noted that the stress and strain fields in the skin can demonstrate gradients near the skin projections of the boundaries between the neighboring facial fat compartments, which together with variable SC thickness can serve as the weak links for the onset of structural instabilities.
Skin aging was reported to be connected with an increase of the effective Young’s modulus of the epidermis,
3.3Epidermal instabilities appearing in a tri-layer model SC/“upper” dermis/“lower” dermis
Next, we consider the tri-layer skin model containing the layers SC/“upper” dermis/“lower” dermis (Fig. 5). The “upper” dermis is an effective skin layer containing the viable epidermis and papillary dermis (layers 2 and 3 in Fig. 2), the “lower” dermis is the reticular dermis layer, and sWAT layer will be further considered to have no adherence to the dermis. The “upper” dermis has the Young’s modulus which is much smaller than both the Young’s moduli of the SC and “lower” dermis [21]. Existence of such intermediate compliant skin layer can significantly change the mechanical properties of the skin and its resistance against wrinkling.
The tri-layer SFCS model containing an additional intermediate soft layer placed between the stiff film and the elastic substrate of the infinite thickness was recently considered in [24]. The critical strain required for the onset of instabilities in SC in the case of very thick reticular dermis can be found by minimization of the total potential energy of the composite with respect to ɛcr in the form
(6)
Here k is the wave number of appearing wrinkles; hud is the thickness of the “upper” dermis layer;
Expression (6) takes the form similar to (1), which in this case describes the composite SC/“effective dermis”, when the intermediate layer is absent
This behavior is dramatically modified if the “upper” dermis is much softer than the “lower” dermis, i.e. if
3.4Structural instabilities appearing on the dermal-epidermal and dermal-subcutaneous interfaces
Till now we have considered the epidermal mechanical instabilities appearing on the top of the bi-layer (epidermis/dermis) and tri-layer (SC/”upper” dermis/”lower” dermis) skin models. Now we consider the question what may respresent the mechanical reason for the structural undulations appearing on the dermal-epidermal interface.
For this, we will consider the tetra-layer skin model SC/viable epidermis/papillary dermis/reticular dermis with flat dermal-epidermal interface and without adherence on the dermal-sWAT interface. To the best of our knowledge, there is no reliable independent measurements for the Young’s moduli of the viable epidermis, papillary dermis and reticular dermis in the same subjects, but it can be strongly assumed that
Morphologically, adherence between epidermis and dermis is mainly determined by properties of the basement membrane, which is structurally connected with both layers [65]. The basement membrane in healthy skin reveals the typical structure known as rete ridges (papillae) – interpenetrations of epidermis and dermis whose amplitude is determined by adhesive strength. Very recently, it was reported that rete ridges are not present in the neonatal skin and appear gradually over the first 3 months of life [66]. It is known that the dermal-epidermal interface is connected with the dermal ECM through a protein network containing COL1, COL4, COL5α1, COL6α3, COL15, and laminin-111 [67].
Adhesive strength on the dermal-epidermal interface is dependent on the effective interfacial contact area which continuously reduces with aging. Increased aging correlates with effacement of rete ridges, decreases of their height and a reduction of dermal papillary projections (Fig. 3) [68]. The number of rete ridges per unit skin surface length in the aged skin decreases to about half of what young skin displays [69]. The expression of collagen along the dermal-epidermal interface can be significantly suppressed by UV irradiation [70]. At the same time, the appearance of epidermal wrinkles as mechanical instabilities is only possible if the adhesive strength between the layers is high. This means that the initial onset of wrinkling should take place in a relatively young skin having a good adherence on the dermal-epidermal interface. This well correlates with observations that the skin wrinkling rate undergoes a step-like increase in early thirties indicating that the wrinkle morphologies in young and old skins must be different [27].
The dermal-sWAT interface also demonstrates undulations, which can reflect mechanical instabilities appearing in this area in an analogous fashion. Deep adipose indentations into the dermis in the thigh area were observed in women with cellulite comparing with women without cellulite or with men, which led to conclusion that such indentations should be the characteristic marker of cellulite [71, 72]. Indeed, it was shown that the architecture of the dermal-sWAT interface correlates with cellulite severity [73] and that this interface can quickly react to mechanical loading with decrease of its surface area [71].
Undulations of the interface with interpenetration of neighboring layers similar to rete ridges are typical for the bimodular substrates having the tension-compression asymmetry, i.e. very different tensile and compressive mechanical moduli [74]. Moreover, it was found that the inward dimples typical for rete ridges appear in the case when the tensile modulus is bigger than the compressive one. This is generally the case for collagen networks, where the Young’s modulus can be an order of magnitude lower under compression than under extension [75].
3.5Influence of the sWAT layer on the onset of epidermal wrinkles
In the above models, the low adhesive strength on the dermal-sWAT interface completely excludes the mechanical involvement of the sWAT layer in modulation of the mechanical properties of the skin. We now assume that the adherence between the dermis and sWAT is strong and discuss how such bonding can affect the critical strain required for the onset of epidermal wrinkling. For this, we consider the system dermis/sWAT as a mechanical bi-layer substrate (Fig. 6). The mechanical behavior of a stiff thin film on a bi-layer substrate with the bottom substrate (in our case, sWAT) having the infinite thickness was discussed in [56].
Fig. 6
Surface instabilities in the film placed on a bi-layer substrate. The adherence between dermis and sWAT is so strong that this bi-layer must be considered as a mechanical composite.
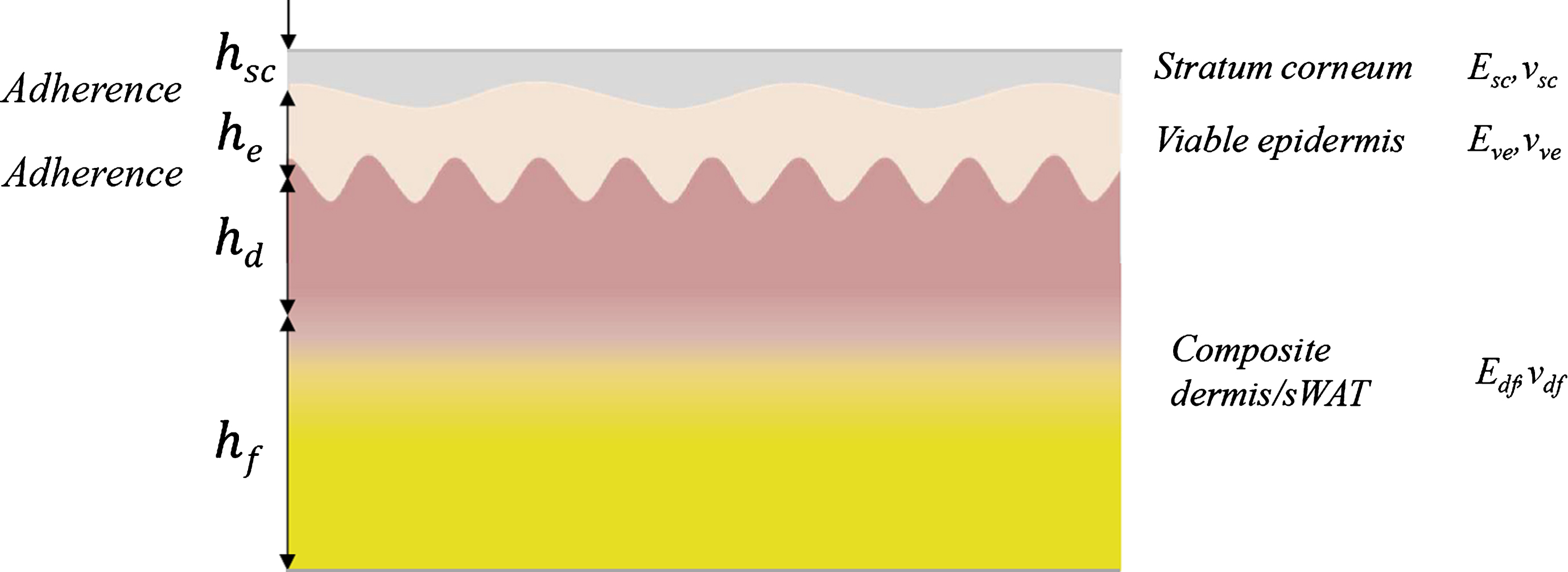
To consider the influence of a bi-layer substrate with single layers of finite thicknesses on the onset of epidermal wrinkling, we will introduce the effective elastic modulus of the composite dermis/sWAT. Let us consider dermis and sWAT to be mutually confined and unable to slide past each other, i.e. we will assume that the dermal-sWAT interface has very high adhesive strength. If the loading producing the stress in the skin is directed in-plane to the skin surface, the effective Young’s modulus of such bi-layer can be approximately presented in the form [44]
(7)
Here ηdf = hd/hf is the thickness ratio between the dermis and sWAT. Thus, the effective Young’s modulus of the mutually confined dermis/sWAT layers is smaller than the corresponding dermal modulus whereas the effective substrate thickness is (1 + ηdf)/ηdf times bigger than hd.
Next, we will compare the critical strains required for the onset of wrinkling in the bi-layer epidermis/dermis described by Eqs. (2)-(3) and in the bi-layer epidermis/”effective dermis”, where the Young’s modulus of the dermis should be replaced by
This consideration is valid for the case of the stress applied in-plane to the skin surface. When mechanical loading is directed normal to the skin, the effective Young’s modulus of the bi-layer dermis/sWAT can be approximately described as [44, 76]
(8)
Whereas the effective Young’s modulus of the bi-layer dermis/sWAT is mainly determined by the Young’s modulus of the dermis in the case of mechanical stress applied in-plane to the skin surface, this modulus is much lower and mainly determined by mechanical properties of the more compliant sWAT layer in the case of the mechanical loading applied perpendicular to the skin. This effect will significantly increase the elastic mismatch between the epidermis and the composite dermis/sWAT and consequently reduce the critical strain required for the onset of structural instabilities in the skin.
At
At ηdf = 0.25, the corresponding values are γed ≈ 0.694, 0.391, and 0.247, and ɛcr = 37.1%, 29.1%, and 24.3% at νf ≈ 0.4, 0.45, and 0.47, respectively. Thus, the critical strain required for the onset of wrinkling decreases with increasing sWAT thickness. In other words, under other equal conditions, the skin must be more susceptible to wrinkling when the sWAT layer is thicker. This effect increases with increasing adhesive strength on the dermis-sWAT interface.
Thus, the adherence on the dermal-sWAT interface must, additionally to the adherence on the dermal-epidermal interface, influence the mechanical behavior of the composite skin/sWAT. While less is known about morphological structures involved in dermal-sWAT adherence, it can be strongly assumed that COL6 [78] and CLMP (Coxsackie virus and adenovirus receptor-like membrane protein) [79, 80] participate on this junction. It was stated that CLMP, also known for adipose tissue as adipocyte adhesion molecule (ACAM), is strongly involved in production of zonula adherence between the cells and significantly influences the integral mechanical strength of the tissue [79]. It can be also supposed that the dermal-sWAT adherence is dependent on the sWAT type. Consequently, different facial fat compartments must demonstrate distinct adhesive properties, which was indeed observed anatomically [51, 52].
Mechanical behavior of the dermal-sWAT interface must be similar to that of the dermal-epidermal interface, since this surface also demonstrates the undulations. Whereas this item was not investigated properly, it can be strongly assumed that these undulations are connected with tension-compression asymmetry existing in sWAT and must be more pronounced in sWAT compartments with high collagen content.
Recapitulating, the sWAT layer of the finite thickness having a strong adherence to the reticular dermis can significantly decrease the critical strain needed for the onset of epidermal wrinkles, whereas this effect must be dependent on the direction of the mechanical loading. Taking into account that different facial fat compartments have various thicknesses of the sWAT layer, different sWAT structures (with correspondingly distinct Young’s moduli and Poisson’s ratios), and demonstrate different adherences on the dermis-sWAT interface, it should be clear that these compartments must differently influence the appearance of skin wrinkling. This effect must be at least partly responsible for the spatial differences in the facial topography of wrinkles in aging skin.
3.6Nutritional effects on fat layers and aging
Both subcutaneous and dermal adipose tissue are affected by nutritional status. At the same time, different depots expand and shrink by different mechanisms. Some depots undergo hyperplasia (i.e. recruitment of additional fat cells), while in other depots, exposure to excess nutrients primarily affects hypertrophy (i.e. an increase in cell size). The subcutaneous fat depots primarily rely on hypertrophic expansion [49]. Contrarily, the dermal fat layer can expand in size and cell number in response to high fat diet, though the underlying mechanisms have not yet been fully explored. Such dietary modulation of the sWAT layer structure must significantly influence the mismatch in mechanical properties between tissue layers. Moreover, it is very likely that nutritional components also influence the adhesion strength between adjacent layers and consequently also modify the aging process. This effect has however not been examined properly.
4Discussion
Critical strain in the skin, required for the onset of wrinkles, depends on such dimensionless parameters as the thickness ratios between the adjacent layers, the tensile and bending stiffness as well as the elastic mismatch in adjacent skin layers. While the ratios and not the absolute values of these parameters appear to be mainly responsible for skin wrinkling, any anti-aging strategy based on the standalone reinforcement of a single skin layer cannot improve skin resistance against appearance of such structural instabilities. This result completely disagrees the main postulate in anti-aging stating that the skin appearance can be improved through standalone reinforcement of the skin, mainly through induction of the neocollagenesis. Accordingly, it can be assumed, that the main target in anti-aging strategies must be the reduction of the mechanical mismatch between the adjacent layers such as epidermis/dermis and dermis/sWAT.
Adherence between adjacent skin layers is of primary importance for the effective mechanical properties of the composite skin/sWAT. Low adhesive strength on such boundaries as dermal-epidermal and dermal-sWAT interfaces reduces the transition of the strain energy from one layer into another and thus can significantly modify the critical conditions required for the onset of skin wrinkles. Adhesive strength on the dermal-epidermal interface is dependent on the structure and functioning of the basement membrane, whereas the corresponding strength on the dermal-sWAT interface should among others be dependent on the fibrotic content in the sWAT, which is different in various facial fat compartments.
Adhesive strength can be influenced by both chronological and external aging, which can be clearly seen in the reduction of such interfacial undulations as rete ridges in aging. Undulations appearing on such inter-layer boundaries as dermal-epidermal and dermal-sWAT interfaces must be connected with strong elastic mismatch existing on the opposite sides of these boundaries as well as with bimodular mechanical properties of at least one layer demonstrating significant tension-compression asymmetry. This is especially the case for the layers containing dense collagen networks.
The collagen network is also involved in important defense mechanisms, reducing the propagation of structural instabilities in the skin. This propagation can be significantly delayed and even arrested through recently discovered redistribution of the collagen fibers in the vicinity of the instability tip in the dermis through mechanisms of fibers straightening, their reorientation towards the tensile direction, elastic stretching and sliding past each other [81]. This adaptation mechanism generally distinguishes the mechanical behavior of the fiber-reinforced from fiber-free materials. Whereas actually not known, it can be assumed that such adaptation of the collagen network to induced mechanical fields should be reduced in aging.
High adhesive strength on the dermal-sWAT interface reduces the effective Young’s modulus of the composite dermis/sWAT and effectively increases its thickness. This can be, for example, the case if the expression of the ACAM molecules involved in this adhesion increases with aging. Whereas, to the best of our knowledge, this question was not investigated properly for adipose tissue, it was shown that at least in some other tissues, CLMP expression indeed increases with aging [82]. Enhanced adhesion can significantly reduce the critical strain required for the onset of the skin wrinkling. For example, as we discussed above, for the sWAT layer which is 2-fold thicker than the dermis, the skin having low adherence to the sWAT should demonstrate the critical strain which is about three times higher than the corresponding critical strain for the composite skin/sWAT with strong adherence between these layers. Remarkably, this effect should be dependent both on the sWAT thickness and Poisson’s ratio in this layer, being stronger for thicker and more incompressible sWAT layers. Thus, stronger adherence between the dermis and sWAT produces more favorable conditions for wrinkling of the epidermal layer. This is a purely mechanical effect, which is not connected with any biological interactions between the cells from different layers. The inter-layer adherence was never considered as an important parameter which can significantly modify the mechanical behavior and development of structural instabilities in the composite skin/sWAT. This important topic must be implicitly implemented in the future research in the field of anti-aging.
Facial fat compartments of the Ghassemi’s types 1 and 2 have different mechanical moduli and adhesive strengths [51] and thus differently transmit the strain energy from the skin to the underlying sWAT. This property must influence the appearance of different structural instabilities in the skin. Facial fat compartments of the type 2 have higher stiffness and thus should demonstrate smaller mismatch in mechanical characteristics between the skin and sWAT. Consequently, the skin covering these compartments should be more resistant against onset of structural instabilities. At the same time, such fat compartments have stronger adherence between the layers, which can facilitate the production of advanced skin instabilities appearing after wrinkles, such as folds or ridges. The end result should be dependent on the relation between these two alternative processes and must be investigated in detail in future research. Contrarily to this, facial fat compartments of the type 1 are more compliant, and their adherence to the overlying skin is weaker; these properties must produce more favorable conditions for the appearance of structural skin instabilities.
Another important phenomenon discussed in this article is the influence of the bending ability of the dermis on the skin stability against wrinkling. Skin bending is energetically more favorable than its wrinkling. Thus, dermis under strain should, if possible, first bend and only by further increase of the applied strain it must produce such surface instabilities as wrinkles. Bending of the dermis is mainly connected with content of elastic fibers in this layer, which is known to be significantly reduced in aging. As it was reported by [13], the unrestored energy ratio (index describing the cutaneous non-elasticity) continuously increases after the age of 30, which should reflect the reduction of cutaneous elasticity with aging. According to above, age-dependent structural changes in the dermis should lead to the reduction of the critical strain required for the onset of structural instabilities in the skin, which can be up to four times smaller in the non-bending skin.
An especially big elastic mismatch exists on the interfaces SC/viable epidermis/papillary dermis/reticular dermis, where the stiffness ratios between the layers can reach three-four orders of magnitude. Existence of such weak link as papillary dermis in the layered skin plays important role in the onset of epidermal wrinkling. Corresponding weak link on the dermis-sWAT interface should be presented by the dWAT tissue filling the undulations penetrating into the dermis. Dermal adipocytes were recently uncovered as special fat cells characterized by high dynamics and able to transdifferentiate into other cell types (for example, into myofibroblasts), thus significantly modulating the geometrical and mechanical properties of the skin composite [44, 47]. These cells are involved in different physiological and pathological processes, among them in wound healing, hypertrophic scarring, hair growth and skin aging [29, 47]. Very recently, we have proposed that these cells should be also involved in psoriasis and androgenetic alopecia [30, 78, 83]. Appearance of internal structural instabilities in the skin, such as undulations on the dermis-sWAT interface was proposed to correlate with other macroscopic mechanical properties of the composite skin/sWAT: existence of such undulations and especially their amplitude were connected with much higher rate of hypertrophic scarring in corresponding body areas [84, 85].
There are different pathways which can lead to the onset of structural instabilities in the skin, among them are:
1) stiffening and/or thickening of the dermis, which can, for example, be observed after UV-irradiation [86, 87] and must lead to increased elastic mismatch between adjacent skin layers;
2) softening and/or reduction of the sWAT layer, which is typical for chronological aging during which sWAT partially loses its collagen network;
3) modulation of the adhesive strength on the dermal-epidermal interface, which takes place both in chronological [68, 69] and in UV-induced aging [70];
4) modification of adhesive strength on the dermis-sWAT interface;
5) strong spatial variations of the SC, epidermal and dermal thicknesses, which can significantly influence the ratios of tensile and bending stiffness, thus producing conditions for the reduction of the critical strain required for the onset of structural instabilities;
6) reduction of the bending ability of the skin through decrease of the elastic fibers content in the dermis.
Consequently, two aging pathways very different in their nature as chronological and extrinsic aging should lead to similar end results concerning the onset of mechanical instabilities in the skin. Both types of aging lead to the impairment of the elastic mismatch between the layers, thus producing more favorable conditions for the appearance of structural instabilities in the skin. This means that the chronological and extrinsic aging should be considered as two sides of the same coin. This can also explain the contradictory reports concerning the modification of the Young’s modulus of the skin in chronological aging in the past.
From above, we can infer that different pathways can lead to qualitatively similar results in improvement of skin resistance against wrinkling, among them are
1) reduction of the elastic mismatch between adjacent skin layers;
2) reinforcement of the sWAT leading to the reduction of the mismatch in the plane tensile moduli on the dermis-sWAT interface;
3) increase of the bending capacity of the skin;
4) modification of the adherence on the dermal-epidermal and dermal-sWAT interfaces.
Despite the fact that these pathways should be realized through the application of different treatment procedures, they lead to one and the same result: increase of the critical strain required for the production of structural instabilities in the skin.
There are very contradictive reports in the literature concerning the absolute values of the Young’s moduli in the skin. The absolute values of these moduli strongly vary not only between different measurement procedures [43], but demonstrate very high dispersions also among the subjects from the same age group [13]. Even more contradictive are the results concerning the age-dependence of these mechanical parameters. This is strange if the modification of the absolute values of the mechanical moduli of the skin is primarily important for its aging. However, this is not contradictory to the theory of structural instabilities whose main parameters are the elastic mismatches between the single layers, the thickness ratios between the layers, as well as some other dimensionless parameters, such as the ratios of tensile stiffness and bending stiffness.
5Conclusions
The multi-layer composite skin/sWAT demonstrates structural instabilities which are similar to those known from the fracture mechanics of mechanical composites containing a thin stiff film adhered to a thick compliant substrate. Appearance of such instabilities, which have the same forms as the typical signs of aging skin, are mainly dependent on the mismatch in mechanical moduli between adjacent layers including sWAT, on the adhesive strengths between these layers, on the thickness ratios between the adjacent layers as well as on the mechanical strains produced in single layers.
Existence of a compliant papillary dermis layer located between the stiffer epidermis and the reticular dermis significantly influences both the critical strain required for the onset of wrinkling in the skin as well as the appearance of rete ridges which should be considered as internal skin instabilities connected with bimodular mechanical properties of the dermis. One of the main parameters controlling the skin wrinkling must be the bending ability of the skin which is dependent on the content of elastic fibers in the skin undergoing strong modification both in intrinsic and extrinsic skin aging. Involvement of different skin layers in wrinkling must be strongly dependent on the adhesive strength existing on the interfaces between these layers; this is especially important for such boundaries as the dermal-epidermal and dermal-sWAT interfaces. Strong adhesion between reticular dermis and sWAT leads to effective involvement of the sWAT layer in skin wrinkling, whereas weak adhesion between these layers reduces the mechanical influence of the sWAT on the structural modifications of the skin in aging.
Such structural instabilities should be more easily induced in the facial skin where the sWAT has the compartmental structure with neighboring compartments having very different mechanical and adherence properties. Consequently, different facial areas should demonstrate also different appearance of the structural skin instabilities. This makes the correlation of mechanical properties and adhesive strengths in different facial compartments with topographical appearance of skin instabilities in aging to important prospective future research field.
Extrinsic (UV-induced) and intrinsic (chronological) aging of the composite skin/sWAT being realized through different pathways should, at the same time, be considered as two sides of a coin: whereas chronological aging should be mainly connected with a loss of the stiffness in the sWAT, extrinsic aging should be realized through an increase of the skin stiffness or through its thickening.
Consequently, any anti-aging strategy should focus not on the standalone skin reinforcement, but target the reduction of the mechanical mismatch between different skin layers as well as between the skin and sWAT.
Funding
PES was supported by US National Institutes of Health (NIH) grants P01-DK088761, R01-DK55758, R01-DK099110 and P01 AG051459.
Conflict of interest
ILK is the managing partner of Wellcomet GmbH. No methods or devices of Wellcomet GmbH were used in this publication. Wellcomet GmbH provided support in the form of salaries for ILK, but did not have any additional role in the study design, data collection and analysis, decision to publish, or preparation of the manuscript. PES reports no conflict of interest.
Acknowledgments
We thank Katja Trende for her generous help with illustrations.
References
[1] | Cerda E , Mahadevan K . Geometry and physics of wrinkling. Phys Rev Lett. (2003) ;90: :074302. |
[2] | Genzer J , Groenewold J . Soft matter with hard skin: From skin wrinkles to templating and material characterization. Soft Matter. (2006) ;2: :310–23. |
[3] | Wang Q , Zhao X . A three-dimensional phase diagram of growth-induced surface instabilities. Sci Rep. (2015) ;5: :8887. |
[4] | Begley MR , Hutchinson JW . The Mechanics and Reliability of Films, Multilayers and Coatings. (Cambridge University Press, (2017) ). |
[5] | Hara Y , Masuda Y , Hirao T , Yoshikawa N . The relationship between the Young’s modulus of the stratum corneum and age: A pilot study. Skin Res Technol. (2013) ;19: :339–45. |
[6] | Huang R . Kinetic wrinkling of an elastic film on a viscoelastic substrate. J Mech Phys Solids. (2005) ;53: :63–9. |
[7] | Diridollou S , et al. Efficacy of topical 0, 05% retinaldehyde in skin aging by ultrasound and rheological techniques. Dermatol. (1999) ;199: :37–41. |
[8] | Zhou B , Xu F , Chen CQ , Lu TJ . Strain rate sensitivity of skin tissue under thermomechanical loading. Philos Trans Royal Soc London A Math Phys Eng Sci. (2010) ;368: :679–90. |
[9] | Lin M , et al. Influences of supra-physiological temperatures on microstructure and mechanical properties of skin tissue. Med Eng Phys. (2012) ;34: :1149–56. |
[10] | Egawa M , Hirao T , Takahashi M . In vivo estimation of stratum corneum thickness from water concentration profiles obtained with Raman spectroscopy. Acta Derm Venereol. (2007) ;87: :4–8. |
[11] | Agache PG , Monneur C , Leveque JL , De Rigal J . Mechanical properties and Young’s modulus of human skin in vivo. Arch Dermatol Res. (1980) ;269: :221–32. |
[12] | Diridollou S , et al. In vivo model of the mechanical properties of the human skin under suction. Skin Res Technol. (2000) ;6: :214–21. |
[13] | Diridollou S , et al. Skin ageing: Changes of physical properties of human skin in vivo. Int J Cosmet Sci. (2001) ;23: :353–62. |
[14] | Alexander H , Cook T . Variations with age in the mechanical properties of human skin in vivo. J Tiss Viabil. (2006) ;16: :6–11. |
[15] | Gerhardt LC , Lenz A , Spencer ND , Münzer T , Derler S . Skin—textile friction and skin elasticity in young and aged persons. Skin Res Technol. (2009) ;15: :288–98. |
[16] | Boyer G , Laquieze L , Le Bot A , Laquièze S , Zahouani H . Dynamic indentation on human skin in vivo: Ageing effects. Skin Res Technol. (2009) ;15: :55–67. |
[17] | Boyer G , et al. Non contact method for in vivo assessment of skin mechanical properties for assessing effect of ageing. Med Eng Phys. (2012) ;34: :172–8. |
[18] | Pailler-Mattei C , Debret R , Vargiolu R , Sommer P , Zahouani H . In vivo skin biophysical behaviour and surface topography as a function of ageing. J Mech Behav Biomed Mater. (2013) ;28: :474–83. |
[19] | Wong R , Geyer S , Weninger W , Guimberteau JC , Wong JK . The dynamic anatomy and patterning of skin. Exp Dermatol. (2016) ;25: :92–8. |
[20] | Shiihara Y , Sato M , Hara Y , Iwai I , Yoshikawa N . Microrelief suppresses large wrinkling appearance: An in silico study. Skin Res Technol. (2015) ;21: :184–91. |
[21] | Hendriks FM , Brokken D , Oomens CWJ , Bader DL , Baaijens FPT . The relative contributions of different skin layers to the mechanical behavior of human skin in vivo using suction experiments. Med Eng Phys. (2006) ;28: :259–66. |
[22] | Kuwazuru O , Saothong J , Yoshikawa N . Evaluation of aging effects on skin wrinkle by finite element method. J Biomech Sci Eng. (2008) ;3: :368–79. |
[23] | Kuwazuru O , Saothong J , Yoshikawa N . Mechanical approach to aging and wrinkling of human facial skin based on the multistage buckling theory. Med Eng Phys. (2008) ;30: :516–22. |
[24] | Lejeune E , Javili A , Linder C . Understanding geometric instabilities in thin films via a multi-layer model. Soft Matter. (2016) ;12: :806–16. |
[25] | Querleux B , et al. Skin from various ethnic origins and aging: An in vivo cross-sectional multimodality imaging study. Skin Res Technol. (2009) ;15: :306–13. |
[26] | Tsukahara K , Tamatsu Y , Sugawara Y , Shimada K . The relationship between wrinkle depth and dermal thickness in the forehead and lateral canthal region. Arch Dermatol. (2011) ;147: :822–8. |
[27] | Kuwazuru O , Miyamoto K , Yoshikawa N , Imayama S . Skin wrinkling morphology changes suddenly in the early 30s. Skin Res Technol. (2012) ;18: :495–503. |
[28] | Donato AJ , et al. The impact of ageing on adipose structure, function and vasculature in the B6D2F1 mouse: Evidence of significant multisystem dysfunction. J Physiol. (2014) ;592: :4083–96. |
[29] | Kruglikov IL , Scherer PE . Dermal adipocytes: From irrelevance to metabolic targets? Trends Endocrinol Metab. (2016) ;27: :1–10. |
[30] | Kruglikov IL , Scherer PE . Adipocyte-myofibroblast transition as a possible pathophysiological step in androgenetic alopecia. Exp Dermatol. (2017) ;26: :522–3. |
[31] | Wang Y , Marshall K , Baba Y , Gerling GJ , Lumpkin EA . Hyperelastic material properties of mouse skin under compression. PLoS One. (2013) ;8: :67439. |
[32] | Mitani H , Koshiishi I , Toyoda H , Toida T , Imanari T . Alterations of hairless mouse skin exposed to chronic UV irradiation and its prevention by hydrocortisone. Photochem Photobiol. (1999) ;69: :41–6. |
[33] | Sayama, A , Soushin, T , Okada, TK , Nakayama, H . Morphological and biochemical changes during aging and photoaging of the skin of C57BL/6J mice. J Toxicol Pathol. (2010) ;23: :133-9. |
[34] | Marangoni RG , et al. Myofibroblasts in murine cutaneous fibrosis originate from adiponectin-positive intradermal progenitors. Arthritis Rheumatol. (2015) ;67: :1062–73. |
[35] | Flynn C , McCormack BA . Finite element modelling of forearm skin wrinkling. Skin Res Technol. (2008) ;14: :261–9. |
[36] | Flynn C , McCormack BA . Simulating the wrinkling and aging of skin with a multi-layer finite element model. J Biomech. (2010) ;43: :442–8. |
[37] | Kruglikov IL . Influence of the dermis thickness on the results of the skin treatment with monopolar and bipolar radiofrequency currents. BioMed ResInt. (2016) :1953203. |
[38] | Robertson K , Rees JL . Variation in epidermal morphology in human skin at different body sites as measured by reflectance confocal microscopy. Acta Derm Venereol. (2010) ;90: :368–73. |
[39] | Krouskop TA , Wheeler TM , Kallel K , Garra BS , Hall T . Elastic moduli of breast and prostate tissue under compression. Ultrason Imaging. (1998) ;20: :260–74. |
[40] | Samani A , Bishop J , Luginbuhl C , Plewes DB . Measuring the elastic modulus of ex vivo small tissue samples. Phys Med Biol. (2003) ;48: :2183–98. |
[41] | Comley K , Fleck N . The compressive response of porcine adipose tissue from low to high strain rate. Int J Imp Eng. (2012) ;46: :1–10. |
[42] | Franco W , Kothare A , Ronan SJ , Grekin RC , McCalmont TH . Hyperthermic injury to adipocyte cells by selective heating of subcutaneous fat with a novel radiofrequency device: Feasibility studies. Laser Surg Med. (2010) ;42: :361–70. |
[43] | Kalra A , Lowe A , Al-Jumaily AM . Mechanical behaviour of skin: A review. J Mater Sci Eng. (2016) ;5: :1000254. |
[44] | Kruglikov IL , Scherer PE . General theory of the skin reinforcement. PLos One. (2017) ;12: :e0182865. |
[45] | Quatresooz P , Thirion L , Piérard-Franchimont C , Pierard GE , The riddle of genuine skin microrelief and wrinkles. Int J Cosmet Sci. (2006) ;28: :389–95. |
[46] | Biniek K , Levi K , Dauskardt RH . Solar UV radiation reduces the barrier function of human skin. Proc Natl Acad Sci USA. (2012) ;109: :17111–6. |
[47] | Kruglikov IL , Scherer PE . Skin aging: Are adipocytes the next target? Aging. (2016) ;8: :1457–69. |
[48] | Sun K , Tordjman J , Clément K , Scherer PE . Fibrosis and adipose tissue dysfunction. Cell Metab. (2013) ;18: :470–7. |
[49] | Wang QA , Tao C , Gupta RK , Scherer PE . Tracking adipogenesis during white adipose tissue development, expansion and regeneration. Nat Med. (2013) ;19: :1338–44. |
[50] | Asterholm IW , et al. Adipocyte inflammation is essential for healthy adipose tissue expansion and remodeling. Cell Metab. (2014) ;20: :103–18. |
[51] | Kruglikov IL , et al. The facial adipose tissue: A revision. Facial Plast Surg. (2016) ;32: :671–82. |
[52] | Ghassemi A , Prescher A , Riediger D , Axer H . Anatomy of the SMAS revisited. Aesthetic Plast Surg. (2003) ;27: :258–64. |
[53] | Chen X , Hutchinson JW . Herringbone buckling patterns of compressed thin films on compliant substrates. J Appl Mech. (2004) ;71: :597–603. |
[54] | Huang ZY , Hong W , Suo Z . Nonlinear analyses of wrinkles in a film bonded to a compliant substrate. J Mech Phys Solids. (2005) ;53: :2101–18. |
[55] | Ma Y , et al. Wrinkling of a stiff thin film bonded to a strained, compliant substrate with finite thickness. Proc Math Phys Eng Soc. (2016) ;472: :20160339. |
[56] | Cheng H , Zhang Y , Hwang KC , Rogers JA , Huang Y . Buckling of a stiff thin film on a strained bi-layer substrate. Int J Solid Str. (2014) ;51: :3113–8. |
[57] | Wang Q , Zhao X . Phase diagrams of Instabilities in compressive film-substrate systems. J Appl Mech. (2014) ;81: :051004. |
[58] | Stewart PS , Waters SL , El Sayed T , Vella D , Goriely A . Wrinkling, creasing, and folding in fiber-reinforced soft tissues. Extr Mech Lett. (2016) ;8: :22–9. |
[59] | Sherratt MJ . Tissue elasticity and the ageing elastic fibre. Age. (2009) ;31: :305–25. |
[60] | Naylor EC , Watson RE , Sherratt MJ . Molecular aspects of skin ageing. Maturitas. (2011) ;69: :249–56. |
[61] | Ezure T , Amano S . Influence of subcutaneous adipose tissue mass on dermal elasticity and sagging severity in lower cheek. Skin Res Technol. (2010) ;16: :332–8. |
[62] | Ezure T , Amano S . Increment of subcutaneous adipose tissue is associated with decrease of elastic fibres in the dermal layer. Exp Dermatol. (2015) ;24: :924–9. |
[63] | Mine S , Fortunel NO , Pageon H , Asselineau D . Aging alters functionally human dermal papillary fibroblasts but not reticular fibroblasts: A new view of skin morphogenesis and aging. PLoS One. (2008) ;3: :e4066. |
[64] | Ciarletta P , Amar MB . Papillary networks in the dermal—epidermal junction of skin: A biomechanical model. Mech Res Com. (2012) ;42: :68–76. |
[65] | Amano S . Possible involvement of basement membrane damage in skin photoaging. J Investig Dermatol Symp Proc. (2009) ;14: :2–7. |
[66] | Miyauchi Y , et al. Developmental changes in neonatal and infant skin structures during the first 6 months: In vivo observation. Pediatr Dermatol. (2016) ;33: :289–95. |
[67] | Bonod-Bidaud C , et al. In vivo evidence for a bridging role of a collagen V subtype at the epidermis—dermis interface. J Investig Dermatol. (2012) ;132: :1841–9. |
[68] | Liao YH , et al. Quantitative analysis of intrinsic skin aging in dermal papillae by in vivo harmonic generation microscopy. Biomed Opt Express. (2014) ;5: :3266–79. |
[69] | Sauermann K , et al. Age related changes of human skin investigated with histometric measurements by confocal laser scanning microscopy in vivo. Skin Res Technol. (2002) ;8: :52–6. |
[70] | Hachiya A , et al. Mechanistic effects of long-term ultraviolet B irradiation induce epidermal and dermal changes in human skin xenografts. Am J Pathol. (2009) ;174: :401–13. |
[71] | Lucassen GW , et al. The effectiveness of massage treatment on cellulite as monitored by ultrasound imaging. Skin Res Technol. (1997) ;3: :154–60. |
[72] | Querleux B , Cornillon C , Jolivet O , Bittoun J . Anatomy and physiology of subcutaneous adipose tissue by in vivo magnetic resonance imaging and spectroscopy: Relationships with sex and presence of cellulite. Skin Res Technol. (2002) ;8: :118–24. |
[73] | Smalls LK , et al. Quantitative model of cellulite: Three-dimensional skin surface topography, biophysical characterization, and relationship to human perception. J Cosmet Sci. (2005) ;56: :105–20. |
[74] | Huang X , Li B , Hong W , Cao YP , Feng XQ . Effects of tension—compression asymmetry on the surface wrinkling of film—substrate systems. J Mech Phys Solids. (2016) ;94: :88–104. |
[75] | Van Oosten AS , et al. Uncoupling shear and uniaxial elastic moduli of semiflexible biopolymer networks: Compression-softening and stretch-stiffening. Sci Re. (2016) ;6: :19270. |
[76] | Liu B , Feng X , Zhang SM . The effective Young’s modulus of composites beyond the Voigt estimation due to the Poisson effect. Compos Sci Technol. (2009) ;69: :2198–204. |
[77] | Van Houten EEW , Doyley MM , Kennedy FE , Paulsen KD , Weaver JB . Initial in vivo experience with steady-state subzone-based MR elastography of the human breast. J Magn Reson Imaging. (2003) ;17: :72–85. |
[78] | Kruglikov IL , Wollina U . Local effects of adipose tissue in psoriasis and psoriatic arthritis. Psoriasis Targets Ther. (2017) ;7: :17–25. |
[79] | Murakami K , et al. Antiobesity action of ACAM by modulating the dynamics of cell adhesion and actin polymerization in adipocytes. Diabetes. (2016) ;65: :1255–67. |
[80] | Matthäus C , Langhorst H , Schütz L , Jüttner R , Rathjen FG . Cell-cell communication mediated by the CAR subgroup of immunoglobulin cell adhesion molecules in health and disease. Mol Cell Neurosci. (2016) ;81: :32–40. |
[81] | Yang W , et al. On the tear resistance of skin. Nat Commun. (2015) ;6: :6649. |
[82] | Communal C , et al. Decreased efficiency of adenovirus-mediated gene transfer in aging cardiomyocytes. Circulation. (2003) ;107: :1170–5. |
[83] | Kruglikov IL , Scherer PE , Wollina U . Are dermal adipocytes involved in psoriasis? Exp Dermatol. (2016) ;25: :812–3. |
[84] | Matsumura H , et al. Cones of skin occur where hypertrophic scar occurs. Wound Rep Regen. (2001) ;9: :269–77. |
[85] | Engrav LH , et al. Functional genomics unique to week 20 post wounding in the deep cone/fat dome of the Duroc/Yorkshire porcine model of fibroproliferative scarring. PLoS One. (2011) ;6: :e19024. |
[86] | Takema Y , Yorimoto Y , Kawai M , Imokawa G . Age-related changes in the elastic properties and thickness of human facial skin. Br J Dermatol. (1994) ;131: :641–8. |
[87] | Nishimori Y , et al. Degenerative alterations of dermal collagen fiber bundles in photodamaged human skin and UV-irradiated hairless mouse skin: Possible effect on decreasing skin mechanical properties and appearance of wrinkles. J Invest Dermatol. (2001) ;117: :1458–63. |