The effects of reduced rpd3 levels on fly physiology
Abstract
BACKGROUND: Rpd3 is a conserved histone deacetylase that removes acetyl groups from lysine residues within histones and other proteins. Reduction or inhibition of Rpd3 extends longevity in yeast, worms, and flies. Previous studies in flies suggest an overlap with the mechanism of lifespan extension by dietary restriction. However, the mechanism of rpd3’s effects on longevity remains unclear.
OBJECTIVES: In this study we investigated how rpd3 reduction affects fly spontaneous physical activity, fecundity, and stress resistance.
METHODS: We examined the effects of rpd3 reduction on fly spontaneous physical activity by using population monitors, we determined female fecundity by counting daily egg laying, and we determined fly survivorship in response to starvation and paraquat.
RESULTS: In flies, rpd3 reduction increases peak spontaneous physical activity of rpd3def male flies at a young age but does not affect total 24 hour activity. Male and female rpd3def mutants are more resistant to starvation on low and high calorie diets. In addition, increased resistance to paraquat was observed in females of one allele. A decrease in rpd3 levels does not affect female fecundity.
CONCLUSIONS: A decrease in rpd3 levels mirrors some but not all changes associated with calorie restriction, illustrated by an increased peak of spontaneous activity in rpd3def/+ heterozygous male flies but no effect on total spontaneous activity and fecundity.
1Introduction
Aging is characterized with progressive decline of physiological responses. Dietary restriction (DR) without malnutrition delays age-related pathophysiology and increases mean and maximal lifespan in a number of species [1, 2]. DR affects many physiological processes, including mitochondrial function, preserved protein homeostasis, enhanced genomic stability, increased insulin sensitivity, and nutrient metabolism. DR improves organismal health, and it delays the development of Type 2 diabetes, cardiovascular disease, neurodegeneration, and cancer. Similar health benefits have been observed in humans on a strict DR regimen without malnutrition, as well as in short-term, randomized clinical trials [3]. The effects of DR depend on the timing and particular nutrients in diet [2]. Some of the possible mediators of beneficial effects of DR include the nutrient-sensing insulin/insulin-like signaling (IIS), the Tor signaling pathway, AMPK, HSF, and sirtuins [2].
Members of class I of the zinc-dependent histone deacetylase (HDAC) family are vital regulators of chromatin structure and gene expression [4–6]. Class I HDACs have a role in development, as well as in pathophysiology of human disorders. Mutations that affect the normal function of HDAC1 have been implicated in development of human cancers, Alzheimer’s, Huntington’s, and Parkinson’s diseases. Thus HDAC inhibitors have the potential to be used in therapy for cancer, neurodegenerative disorders, muscular atrophy, and heart disease [6–9]. Underexpression of Rpd3, the Drosophila HDAC1 homologue, is associated with a longer lifespan in yeast and fruit flies [10–15]. Males heterozygous for either the hypomorphic or null rpd3 mutations live 33% and 41% longer compared to controls, respectively [11]. Female flies heterozygous for the hypomorphic allele have a 52% longer lifespan, while females carrying the null mutation have a longer maximum but not median lifespan [11]. Longer lifespan was also observed in flies fed 4-phenylbutyrate (PBA), a histone deacetylase inhibitor [16]. Others and our lab have shown that longevity extension associated with reduced rpd3 overlaps with longevity effects of a dietary restricted diet [11, 13–15, 17, 18]. This hypothesis was supported by findings that DR and rpd3 under-expression are not additive in their lifespan effects [11]. They also cause a similar change in transcription levels of dSir2, takeout, 4E-BP, and members of the insulin/insulin-like signaling (IIS) pathway such as InR, chico, and dFoxo [11, 13, 15, 17]. It was also reported that levels of rpd3 mRNA are decreased and dSir2 mRNA levels are increased in starved flies, while the opposite was found in flies when re-fed; they have increased levels of rpd3 and reduced dSir2 mRNA levels [19]. Flies mutant for both rpd3 and dSir2 live shorter compared to rpd3 mutant flies, suggesting that dSir2 mediates some effects of rpd3’s effects on fly physiology and longevity [13]. However, when longevity studies were done on diets with varying caloric content, rpd3 mutant flies live longer on some DR diet levels suggesting that rpd3 and DR have distinct but interacting effects on fly longevity [14]. This suggests that the relationship between the mechanism of longevity observed in rpd3 flies and DR is more complex than previously thought.
2Materials and methods
2.1Fly strains and maintenance
rpd3-deficient (rpd3def24/TM6,Sb) and rpd3–hypomorphic (rpd3P-UTR/TM3,Sb,Ser) flies and their genetic controls, were used in the experiments. Heterozygous rpd3P-UTR/+ and rpd3P-1.8/+ flies were F1 generation from the crosses between male rpd3P-UTR/TM3,Sb,Ser or rpd3P-1.8/TM6,Tb and CS virgin females. Male rpd3def24/TM6,Sb flies were crossed to yw females. Genetic controls for rpd3def were progeny generated by crossing F1 rpd3def/yw littermates. CS and yw flies are wild type for the rpd3 gene, thus flies heterozygous for rpd3def24/yw, rpd3P-UTR/CS and rpd3P-1.8/CS are referred as rpd3def/+, rpd3P-UTR/+, and rpd3P-1.8/+ in the text. The hypomorphic rpd3P-UTR allele has a P-element inserted in the 5’UTR region of the rpd3 gene, which affects expression throughout the fly’s body. The control rpd3P-1.8 (rpd3P-1.8/TM6,Tb) allele has a P-element inserted 1.8 Kb upstream from the transcriptional start site, which only decreases expression in the eye [20]. Canton S and yw were kindly provided by the Bloomington Stock center. Flies were collected within 24 hours of eclosion and maintained using standard corn culture media, low calorie (0.5 N, 0.7 N) or high calorie (1.5 N) diet in plastic vials. The caloric content of 0.5 N food is 50% that of the 1.0 N food. Flies were kept at 25C in a humidified incubator with a 12 hour light-dark cycle. 25 males and 25 females are kept together in each vial, and they are passed to a fresh vial every Monday, Wednesday, and Friday. Fly diets were prepared as previouslyreported [21].
2.2Spontaneous physical activity
Flies were aged until 10 or 40 days of age on a corn diet. 10 males or 10 females were placed in separate glass vials containing culture media. 3 vials per rpd3def/+ and their controls, and 4 vials per rpd3P-UTR/+ and rpd3P-1.8/+ control were used. These vials were placed in mobility monitors that counted every time a fly passed the top, middle, or bottom of the vial. Data were taken at the start of the following light cycle to assure the flies had acclimated to the new environment [21].
2.3Fecundity
Male rpd3P-UTR/TM3,Sb,Ser or rpd3P-1.8/TM6,Tb were crossed to CS virgin females. 1 female virgin of the same genotype (CS, rpd3P-UTR/CS or rpd3P - 1 .8/CS) was collected on CO2 and placed in individual plastic vials containing 0.5 N or 1.5 N agar medium [22, 23]. The flies were passed to new vials daily and the number of eggs was counted. 10 vials were used for each genotypes and each diet.
2.4Starvation and paraquat resistance
Flies were collected as described above and aged until 10 or 40 days of age. They were separated into vials of 20 males or 20 females and transferred into new vials containing 2 filter papers with 300μl of DI H2O for starvation studies. For paraquat resistance 20 flies were transfer into a vial containing filter paper soaked with 300μL of 20 mM paraquat following initial starvation for 6 hours [24]. The number of dead flies was counted hourly during the day and twice overnight. Stress resistance data were analyzed by log-rank tests using the JMP 12 program. The total number of flies per experiment is listed in Table 3 and Table 4.
Table 3
Starvation resistance of rpd3def/+ heterozygous flies and genetic controls
Gender | Genotype | N | Age | Food | Mean LS (% change) | X2 | p |
M | rpd3def/+ | 115 | 10 | 0.7 N | 25 | 66.054 | <0.0001* |
M | Control | 41 | 10 | 0.7 N | 19(–31.5) | ||
F | rpd3def/+ | 104 | 10 | 0.7 N | 45 | 15.075 | <0.0001* |
F | Control | 8 | 10 | 0.7 N | 38(–18.4) | ||
M | rpd3def/+ | 126 | 10 | 1.5 N | 28 | 124.6 | <0.0001* |
M | Control | 69 | 10 | 1.5 N | 20 (–40) | ||
F | rpd3def/+ | 117 | 10 | 1.5 N | 75 | 95.77 | <0.0001* |
F | Control | 100 | 10 | 1.5 N | 46 (–63) | ||
M | rpd3def/+ | 82 | 40 | 0.7 N | 20 | 23.267 | <0.0001* |
M | Control | 19 | 40 | 0.7 N | 15 (–33) | ||
F | rpd3def/+ | 89 | 40 | 0.7 N | 26 | 44.512 | <0.0001* |
F | Control | 63 | 40 | 0.7 N | 17 (–52) | ||
M | rpd3def/+ | 137 | 40 | 1.5 N | 21 | 23.01 | <0.0001* |
M | Control | 23 | 40 | 1.5 N | 14 (–50) | ||
F | rpd3def/+ | 136 | 40 | 1.5 N | 45 | 261.77 | <0.0001* |
F | Control | 106 | 40 | 1.5 N | 15 (–200) |
The mean lifespan of rpd3def/+ and genetic control (+/+) heterozygous male (M) and female (F) flies after exposure to starvation at 10 or 40 days of age. The flies were aged on 0.7 N or 1.5 N food levels before being exposed to starvation as indicated. Control values are compared to either male or female rpd3def/+ groups to determine the percent change in mean lifespan. Mean lifespans are in hours. Log-rank analyses were performed using the JMP 12 program. M = Males, F = Females, N = number of flies in the experiment. *= a statistical significant result.
Table 4
rpd3P-UTR but not rpd3def heterozygous female flies have higher paraquat resistance compared to the control flies in the same genetic backgrounds
Gender | Genotype | N | Age | Mean | X2 | p | Maximal lifespan |
(% change) | (% change) | ||||||
M | rpd3def/+ | 71 | 10 | 24 | 8.7859 | <0.0030* | 43 |
M | Control | 51 | 10 | 20 (–20) | 25 (–72) | ||
F | rpd3def/+ | 70 | 10 | 31 | 9.0113 | <0.0027* | 67 |
F | Controls | 94 | 10 | 39 (21) | 70 (4) | ||
M | rpd3def/+ | 49 | 40 | 11 | 0.5829 | 0.4633 | 17 |
M | Control | 17 | 40 | 12 (0.8) | 17 (0) | ||
F | rpd3def/+ | 28 | 40 | 16 | 0.0192 | 0.8898 | 24 |
F | Controls | 55 | 40 | 17 (0.5) | 25 (0) | ||
M | rpd3P-UTR/+ | 172 | 10 | 32 | 7.0464 | 0.0079* | 54 |
M | rpd3P-1.8/+ | 107 | 10 | 36 (11) | 66 (18) | ||
F | rpd3P-UTR/+ | 172 | 10 | 52 | 29.548 | <0.0001* | 84 |
F | rpd3P-1.8/+ | 121 | 10 | 42 (–24) | 66 (–27) | ||
M | rpd3P-UTR/+ | 300 | 40 | 16 | 7.757 | <0.0053* | 18 |
M | rpd3P-1.8/+ | 306 | 40 | 17 (0.5) | 19 (0.5) | ||
F | rpd3P-UTR/+ | 160 | 40 | 24 | 94.416 | <0.001* | 42 |
F | rpd3P-1.8/+ | 169 | 40 | 17 (–41) | 24 (–75) |
The mean and maximal lifespan of rpd3def/+ and genetic control (+/+), and rpd3P-UTR/+ and their genetic control (rpd3 P-1.8/+) heterozygous male (M) and female (F) flies after exposure to paraquat at 10 or 40 days of age. Control values are compared to either male or female rpd3def/+ groups or rpd3P-UTR to determine the percent change in mean and maximal lifespan. Mean and maximal lifespan are in days. N = number of flies in the experiment. Log-rank analyses were performed using the JMP 12 program. *= a statistical significant result.
3Results
3.1The effect of rpd3 reduction on fly spontaneous locomotor activity
To test if the mechanism of rpd3 reduction on fly longevity is similar to DR we investigated various aspects of Drosophila physiology in rpd3 mutant flies and their genetic controls. We used two different heterozygous rpd3 alleles due to embryonic lethality of homozygous rpd3 mutant flies. We used rpd3 deficient, an rpd3 null line, (rpd3def/+) and their genetic control, F1 progeny of rpd3def/+ littermates. The levels of rpd3 mRNA in rpd3def/+ is 50% lower compared to controls. We also used rpd3P-UTR/+ flies, an rpd3 hypomorph, and rpd3P-1.8/+, which are their genetic controls and have rpd3 reduction only in the eyes [20]. The presence of one copy of a P-element in rpd3P-UTR/+ flies results in 60% of rpd3 mRNA levels compared to heterozygous rpd3P-1.8/+ control flies [11]. Locomotor activity reflects the functional activity of the nervous system. There is an age-related decline in the locomotor activity of flies [21, 22]. One of the hallmarks of DR is increased spontaneous locomotor activity, and it is observed in multiple species [21, 22, 25, 26]. This is thought to be due to an increased scavenging for food. In mice and flies Sirt1 and dSir2 mediate the increased locomotor activity associated DR, respectively [25–27]. To test if there is an overlap in the mechanism of DR and rpd3 mutation, we examined the spontaneous physical activity of rpd3 mutants and their controls. We used population monitors, which allow continuous monitoring of spontaneous physical activity of the population of 10 flies during longer time periods [21]. At 10 days of age, we found an increase in peak activity in rpd3def/+ male flies (Fig. 1A, Table 1). However, at an older age the peak activity in male rpd3def/+ flies was not different compared to controls (Fig. 1B, Table 1). Total 24 hour activity was not different at age 10 or 40 (Fig. 1C). Females rpd3def/+ flies showed little difference compared to their controls at 10 days of age (data not shown). A similar tendency toward increased peaks of spontaneous activity but no significant effect on the peak or on total 24 hour activity was observed in rpd3P-UTR/+ male and female flies when compared to controls at 10 days of age (Fig. 1D-F).
Fig.1
Effects of rpd3 mutations on fly spontaneous physical activity. (A-C) Average (A, B) and total (C) 24 hours spontaneous physical activity of rpd3def/+ male flies and their genetic controls (+/+) at 10 (A) and 40 (B) days of age. (A-C) rpd3def/+ male flies have increased peak spontaneous physical activity compared to their genetic controls but the total locomotor activity of rpd3def/+ male flies over the course of 24 hours is simialr to their genetic controls at age 10 and 40 days. (D,E) Average spontaneous physical activity of rpd3P-UTR/+ male (D) and female (E) flies and their genetic controls rpd3P-1.8/+ at 10 days of age. (F) The total spontaneous physical activity of rpdP-UTR/+male and female flies and their genetic controls rpd3P-1.8/+ over the course of 24 hours at age 10 days. (A, B, D, E) The data are collected in 10 minutes bins and represent an average of sponteneous locomotor activity of three biological replicates of 10 female or 10 male flies (each replicate) of experimental (rpd3def /+ (A-C); rpd3P-UTR (D-F)) or control (+/+(A-C); rpd3P-1.8 (D-F)) flies. An asterix on the x-axis marks the time when the light was switched off and the transition to dark cycle (6 : 00PM or 720 minutes).
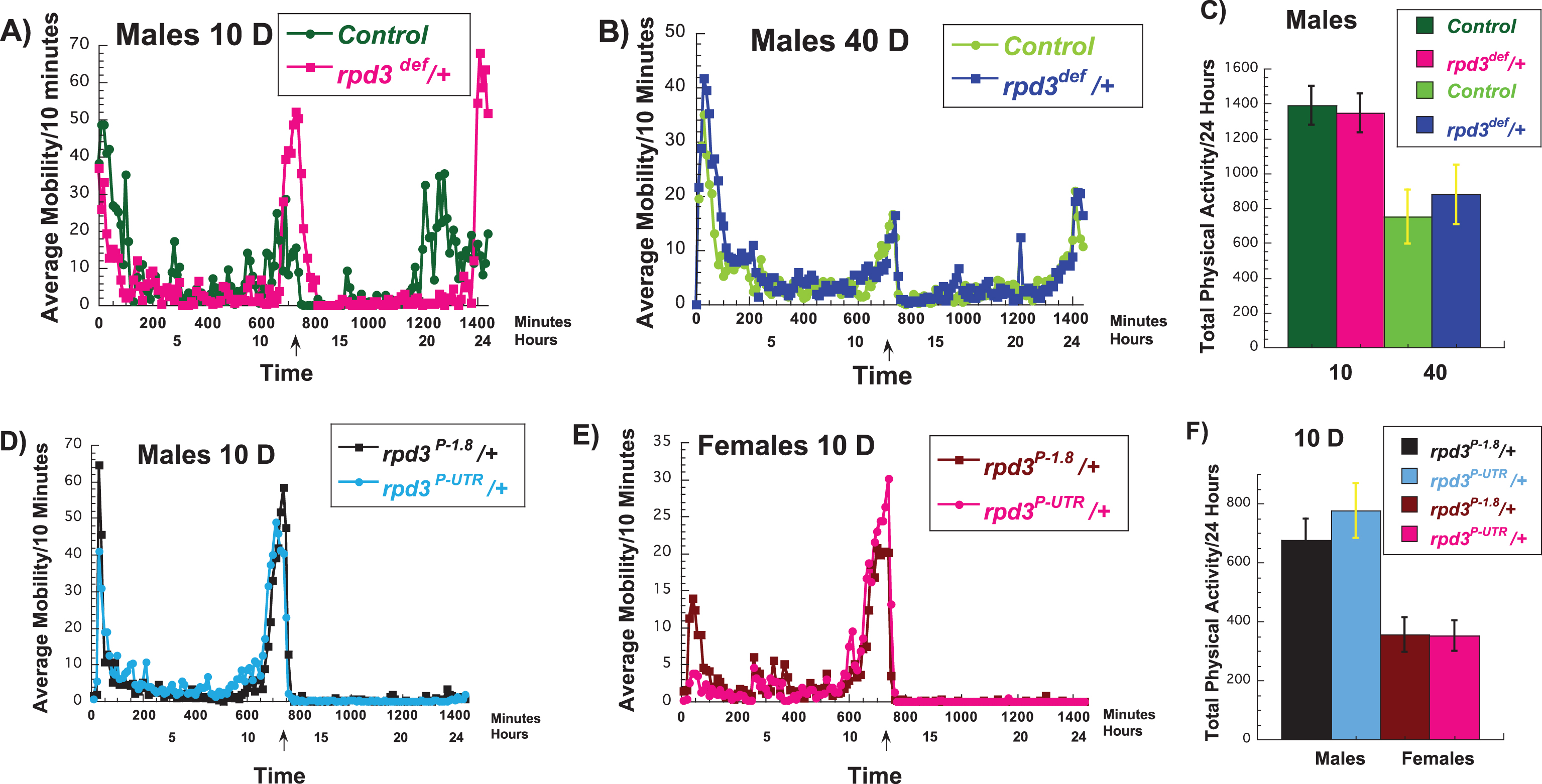
Table 1
Spontaneous physical activity of rpd3def and rpd3P-UTR heterozygous flies and controls
Gender | Genotype | Age | Peak activity | Total activity | (SE) | |||||
1 | p | 2 | p | 3 | p | |||||
M | rpd3def/+ | 10 | 141 | 388 | 192 | 1347 | 112 | |||
M | Control | 10 | 223 | 0.3 | 171 | 0.004* | 90 | 0.18 | 1390 | 305 |
M | rpd3def/+ | 40 | 193 | 85 | 93 | 879 | 172 | |||
M | Control | 40 | 154 | 0.28 | 98 | 0.57 | 76 | 0.58 | 749 | 156 |
M | rpd3P-UTR/+ | 10 | 116 | 328 | 5 | 775 | 93 | |||
M | rpd3P-1.8/+ | 10 | 137 | 0.6 | 331 | 0.95 | 6 | 0.08 | 677 | 79 |
F | rpd3P-UTR/+ | 10 | 14 | 199 | 0 | 351 | 51 | |||
F | rpd3P-1.8/+ | 10 | 49 | 0.08 | 153 | 0.5 | 0 | 1.0 | 355 | 59 |
The peaks and the total spontaneous physical activity of rpd3def/+ and their genetic controls+/+, rpdP-UTR/+ and their genetic controls rpd3P-1.8/+. The peak 1 is sum of the total activity between 6AM–7AM, peak 2 between 5PM–7PM, and 3 between 5AM–6AM. The total spontenaous activity is sum of the activity over the course of 24 hours at age 10 or 40 days. The data were collected for at least three biological replicates of 10 female or 10 male flies (each replicate) of experimental or control flies. Student T test analysis was used to determine the difference in the peaks of activity. *= a statistical significant result.
3.2The effect of reduced rpd3 on fly fecundity
We have previously reported that rpd3 reduction does not affect egg production in virgin rpd3P-UTR /+ compared to rpd3P-1.8 /+ female flies aged on a regular diet [11]. DR reduces fly fecundity due to re-allocation of the resources from reproduction to maintenance and repair [22, 28]. Here we examined if feeding rpd3P-UTR /+ flies a high (1.5 N) or a low (0.5 N) calorie diet would affect their fecundity. We counted the number of eggs laid by flies heterozygous for wild type Canton S (CS) and rpd3P-UTR /+ or rpd3P-1.8/+ (genetic control) daily during the first 10 days of life. CS female flies were included for an additional comparison since they share 50% of the genetic background, and to illustrate the negative effect of 0.5 N diet on female fecundity. If rpd3 reduction mimics DR completely, we would expect rpd3P-UTR/+ females to lay fewer eggs compared to controls on a 1.5 N diet, and even fewer on a 0.5 N diet. However, there was no difference in total egg number laid by rpd3P-UTR/CS females compared to rpd3P-1.8 on 1.5 N diet, and only a minor tendency toward a lower number on 0.5 N diet (p = 0.053) (Fig. 2A, B, Table 2). Furthermore, no differences in fecundity between rpd3P-UTR/CS and CS were observed. Thus rpd3 reduction does not affect female fecundity on either 0.5 N or 1.5 N diet.
Fig.2
Effect of rpd3 reduction on fly fecundity. (A) The average number of eggs per day laid by wild type Canton-S (CS), rpd3P-UTR/CS, and genetic controls rpd3P-1.8/CS on 0.5 N and 1.5 N diets. (B) The total number of eggs per 10 day period laid by CS, rpd3P-UTR/CS, and rpd3P-1.8/CS on 0.5 N and 1.5 N diets. rpd3P-UTR/CS flies laid similar numbers of eggs on 1.5 N but lower numbers on 0.5 N compared to the controls, a difference that did not reach significance (p = 0.053). 10 vials with a single male and a single female were used per genotype and diet.
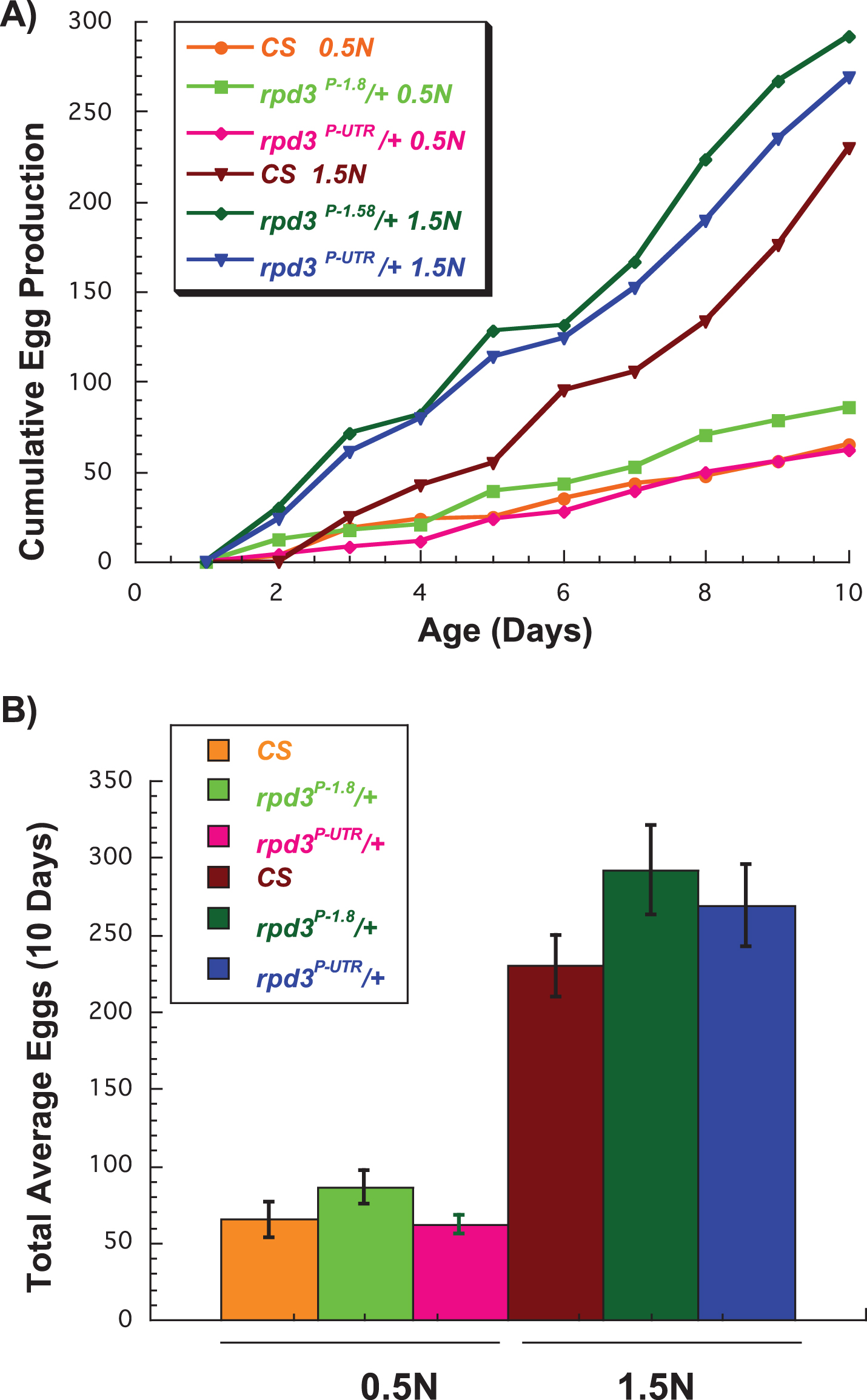
Table 2
Effect of rpd3 reduction on female fecundity
Genotype | Food | Total eggs | SE | |||
rpd3P-UTR/+ | 0.5 N | 62 | 6.2 | |||
rpd3P-1.8/+ | 0.5 N | 86 | 10.9 | |||
CS | 0.5 N | 65 | 11.6 | |||
rpd3P-UTR/+ | 1.5 N | 269 | 26.1 | |||
rpd3P-1.8/+ | 1.5 N | 292 | 28.7 | |||
CS | 1.5 N | 230 | 20.0 |
The total number of eggs per 10 day period laid by CS, rpd3P-UTR/CS, and rpd3P-1.8/CS flies aged on 0.5 N or 1.5 N diets. 10 vials with a single male and a single female were used per genotype and diet.
3.3Reduction in rpd3 increases starvation resistance of male and female flies
Increased starvation resistance is a phenotype found in many long-lived animals, yet its modification by DR remains a debatable topic. The first Drosophila DR experiment found that both genders of flies had increased starvation resistance on low-calorie food, yet the increase was not as great in males [28]. Reducing the calorie content in food, especially protein, increases starvation resistance in flies due to lipid accumulation [28, 29, 30]. Nevertheless, extended DR reduces starvation resistance in flies [28]. In addition, some studies indicate that genetic manipulation that mimics DR decreases starvation resistance because of the reduction in energy stores [31]. rpd3def/+ and control (+/+) flies were raised on different food levels until 10 or 40 days of age and then exposed to starvation. We used 0.7 N as a DR diet and 1.5 N as a high calorie diet. We first compared the starvation resistance of aging control flies on 0.7 N to controls aged on 1.5 N food. At 10 days of age male and female control flies aged on 1.5 N food had a higher starvation resistance compared to flies kept on 0.7 N food (Fig. 3A,B, Table 3). However, at 40 days of age, there were no differences in starvation resistance for flies aged on 0.7 N or 1.5 N food (Fig. 3C,D, Table 3). Another interesting observation was that control male flies aged on 0.7 N or 1.5 N had a much lower starvation resistance compared to control females aged on the same food at 10 days of age. However, both male and female control flies had an almost identical starvation resistance at 40 days of age regardless of the content of the food on which they were aged (Fig. 3E). Male and female rpd3def/+ flies were more resistant to starvation than +/+males at 0.7 N and 1.5 N (Fig. 3A-D, Table 3). In addition, starvation resistance in female rpd3def/+ was much higher compared to males at both 10 and 40 days of age. Thus rpd3 reduction increases starvation resistance in rpd3def/+ flies when aged on a standard, a low, or a high calorie diet.
Fig.3
Reduction of rpd3 increases resistance to starvation of male and female flies on a low and a high calorie diet. (A, B) Survival curves of rpd3def/+ and +/+ male (A) and female (B) flies aged on 0.7 N or 1.5 N food levels and exposed to starvation at 10 days of age. (C, D) Survival curves of rpd3def/+ and+/+male (C) and female (D) flies aged on 0.7 N or 1.5 N food levels and exposed to starvation at 40 days of age.
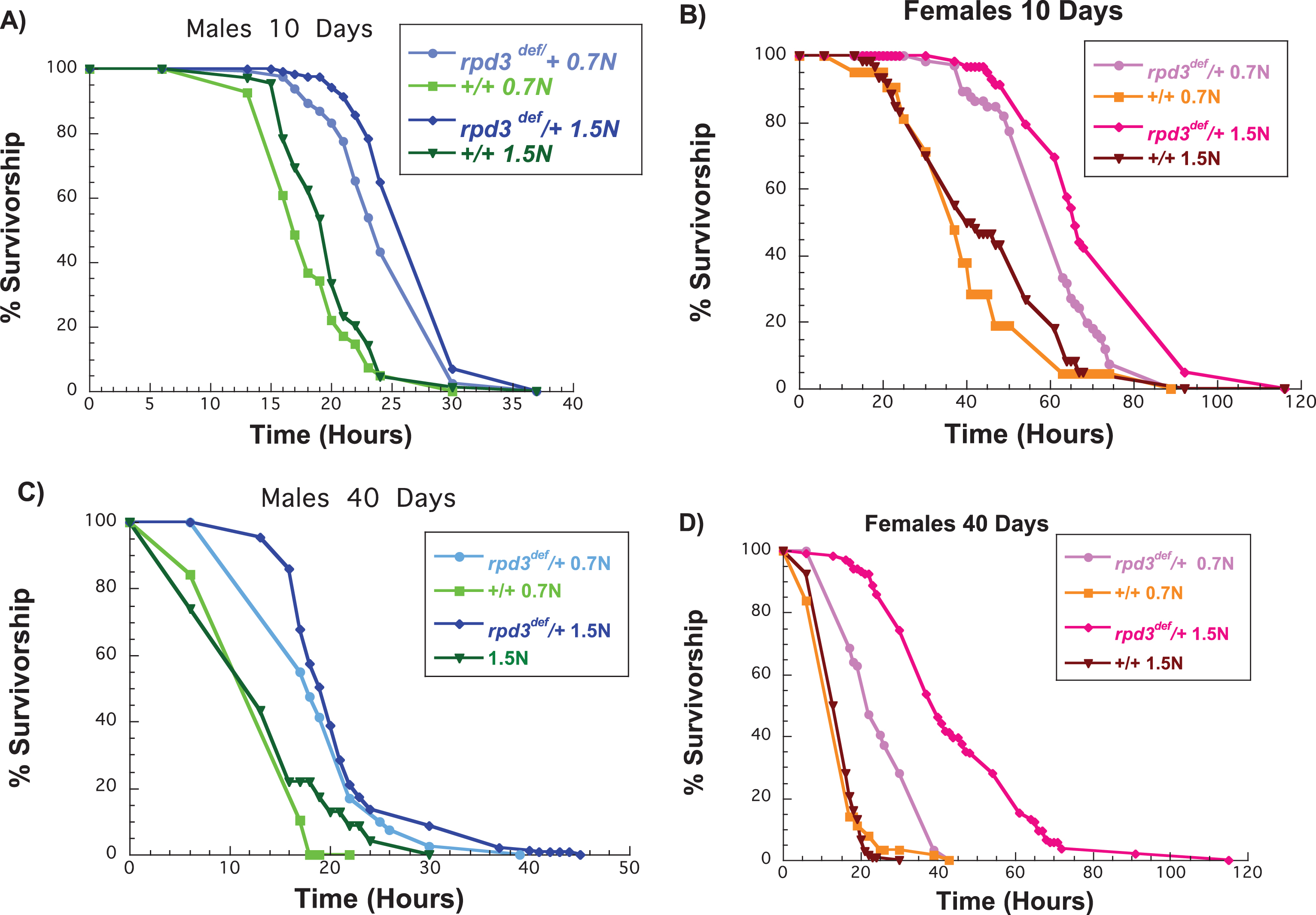
3.4The effect of reduced rpd3 mRNA levels on paraquat resistance
Increased resistance to oxidative stress is often associated with increased longevity and it has been suggested that improved stress response could be one of the contributors to longer life [24]. DR does not affect oxidative stress resistance of young flies but has a negative effect on aged flies [28]. We have reported that rpd3 reduction increases resistance to hydrogen peroxide, but this resistance depends on fly age and sex. Resistance is higher in females compared to males, and more pronounced at older ages [18]. Increased resistance to paraquat is also observed in flies with heart-specific rpd3 reduction, as well as whole body reduction in 2 days old flies [15]. However, the effect of whole body rpd3 reduction on paraquat resistance during aging has not been determined. There is an age-associated decline in resistance to paraquat in wild type flies, thus we examined if rpd3 reduction could postpone the known age-associated decline in resistance [32]. The response of rpd3def/+ and rpd3P-UTR/+ flies to paraquat depends on fly sex, age, and allele. rpd3P-UTR/+ females had increased resistance to paraquat at both 10 and 40 days, while males had no difference compared to controls (Fig. 4A, B). In contrast, rpd3def/+ females were less resistant to paraquat at 10 days while there was no difference at age 40. There was no difference in resistance to paraquat in rpd3def/+ males at both ages (Fig. 4C, D). These data illustrate how genetic background, level of rpd3 reduction, age, and sex affect fly resistance to paraquat.
Fig.4
rpd3 reduction affects stress resistance in flies. (A, B) Survival curves for male and female rpd3P-UTR/+ (experimental) and rpd3P-1.8/+ (control) flies exposed to 20 mM paraquat at age 10 (A) or 40 (B) days. rpd3P-UTR/+ females are more resistant to paraquat at 10 and 40 days, but no changes in resistance were observed in male rpd3P-UTR/+ flies. (C, D) Survival curves for male and female rpd3def/+ and control flies exposed to 20 mM paraquat at age 10 (C) or 40 (D). Male rpd3def/+ flies are more resistant and female rpd3def/+ are less resistant compared to control flies at age 10. No difference in paraquat resistance was observed between rpd3def/+ male and female and control flies at 40 days.
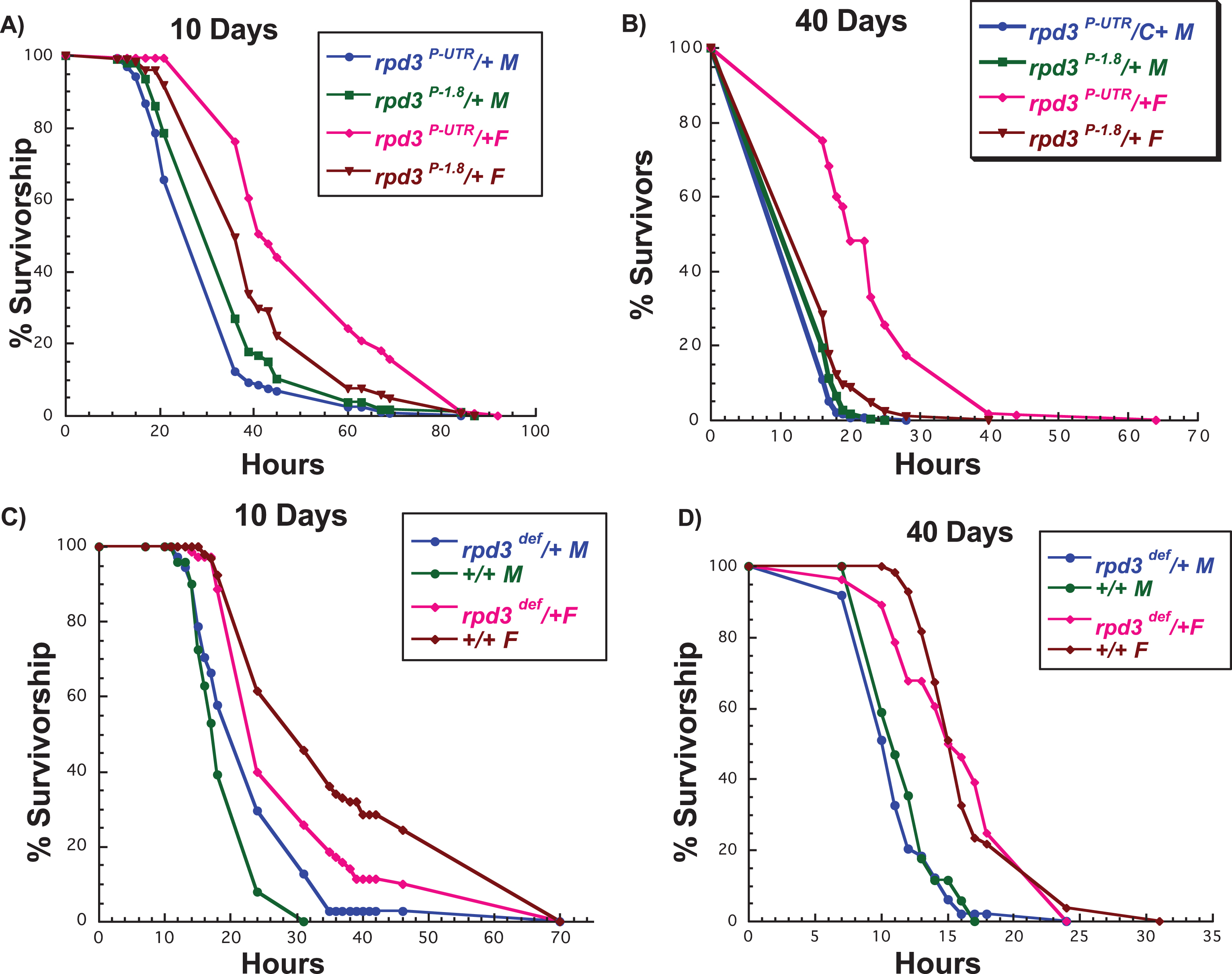
4Discussion
DR has multiple effects on physiology. In flies, DR reduces fecundity, increases fly spontaneous physical activity, increases mitochondrial biogenesis, and slows down age-related mortality [22, 28, 33]. The effects of rpd3 reduction on lifespan in flies appear to at least partially overlap with the effects of DR, as DR and rpd3 whole body under-expression share a key effect on dSir2, takeout, chico, InR, and dFoxo levels of transcription [11, 13–15, 17–19]. Heart-specific rpd3 reduction increases the levels of Sod2, dSir2, dFoxo, Thor, and DptB in 2 day old flies [15]. dSir2 has been thought to mediate longevity extension in DR flies, illustrated by a lack of DR longevity effects in dSir2 mutant flies. Flies double mutant for dSir2 and rpd3 live shorter compared to single rpd3 mutant flies suggesting that dSir2 mediates at least partially the longevity effect in rpd3 flies [13]. In addition, rpd3 reduction and DR are not additive in their effects on lifespan [11]. Therefore, it was hypothesized that the mechanism of longevity extension in DR and rpd3 flies is the same or related [11, 13]. However, rpd3 mutants live longer under conditions close to starvation and during starvation, suggesting that DR and rpd3 may have distinct but interacting or overlapping effects on fly longevity [14, 18]. To gain further insight into the mechanism of lifespan extension observed in rpd3 flies, we determined if similar changes in physiology associated with DR can be observed in flies with reduced rpd3 levels. Particularly, we investigated the effects of decreased levels of rpd3 on spontaneous physical activity, fecundity, and stress resistance. rpd3def/+ male flies had an increased peak in spontaneous activity at a younger age most likely mediated by increased dSir2 levels. Fly spontaneous physical activity decreases with age, and so do the differences in the peak activity. No difference was observed in rpd3def/+ males at age 40. Although peak activity appears to be associated with rpd3 reduction in male rpd3def/+ flies at a younger age, no clear difference in peak or total spontaneous physical activity was associated with rpd3 reduction in rpd3def/+ females or rpd3P-UTR/+ male and female flies. The two rpd3 alleles have different levels of rpd3 mRNA that could explain difference in the peak of spontaneous physical activity or resistance to paraquat. While rpd3 mRNA expression is reduced to 50% in the rpd3def/+ heterozygous flies compared to controls, the level of rpd3 mRNA is reduced by 40% in rpd3P-UTR/+ compared to rpd3P-1.8/+ flies. The levels of rpd3 mRNA are linked to different physiological consequences, as well as longevity effects. For instance, while males of both rpd3 alleles and female rpd3P-UTR/+ flies have dramatically extended longevity, rpd3def/+ females only have extended maximal lifespan but not median lifespan [11]. Similarly, different effects on starvation resistance were observed in flies with whole body rpd3 reduction using RNAi mediated silencing compared to reduction only in fly heart tissue [15]. Likewise, the two rpd3 alleles respond differently to paraquat. However, we show that H2O2 and starvation resistance were similar in both rpd3 alleles (discussed below).
DR has a negative effect on egg production in female flies due to a shift in use of available resources from reproduction to maintenance [25, 28]. If rpd3 reduction mimics DR we would expect lower fecundity in rpd3 females aged on a DR diet. However, no difference in fecundity was associated with rpd3 reduction. No difference in fecundity could be explained by higher energy storage and/or increased adaptation to DR found in rpd3 flies, as discussed below [18].
The effects on starvation and oxidative resistance caused by DR of wild type flies changes with age [28]. While DR increases starvation resistance early in life, the opposite was found in aged DR flies [28]. Increased resistance to starvation of young flies is associated with increased lipid content. However, this increased lipid storage is not sustained over longer time, which results in lower starvation resistance in older flies [28]. We previously reported that rpd3def/+ and rpd3P-UTR/+ flies have increased starvation resistance [18]. Heart-specific rpd3 reduction also increases starvation resistance in flies at 2 days of age [15]. Nevertheless, the same authors did not find increased starvation resistance in flies when rpd3 was reduced in other tissues. Here we examined how aging rpd3 or control flies on 0.5 N or 1.5 N affect their starvation resistance. Our control flies aged on 1.5 N food had a small increase in starvation resistance compared to flies aged on 0.5 N food at an early age. However, no difference in starvation resistance between control male or female flies was observed at age 40 days, and diet did not have an effect at this age either. We observed increased starvation resistance in both rpd3 alleles, with females showing a larger resistance compared to male rpd3 flies. An increase in lipid storage caused by an increase in the activity of the enzymes associated with lipid biogenesis is often associated with increased starvation resistance [28]. Increased metabolic reserves in the form of both lipids and carbohydrates are associated with increased starvation resistance in an outbred population of Drosophila melanogaster. We recently reported that rpd3 reduction is associated with increased levels of triglycerides before and after starvation, which is at least partially mediated by reduced IIS [18]. Consistently, flies with ablated insulin-producing cells have increased levels of lipids and starvation resistance [35]. Flies with reduced rpd3 levels have also increased levels of glucose, glycogen, and trehalose at older ages, illustrating increased energy storage [18]. It is also possible that increased resistance to starvation observed in rpd3 flies results from metabolic adaptation to nutrient availability. Such changes could be mediated by changes in acetylation of metabolic enzymes, which could affect their enzymatic activity allowing a shift from one metabolic pathway to another [36, 37]. It was shown that histone modifying enzymes link changes in nutrient availability to changes in intermediary metabolism by affecting the activity of the enzymes involved in glycogenesis, glycolysis, gluconeogenesis, and β-oxidation through acetylation [36, 37].
DR has no effect on oxidative stress resistance early in life but decreases resistance to paraquat as flies age [28]. We showed that rpd3 reduction increases resistance to oxidative stress, as measured by increased resistance to H2O2. This resistance is mediated by reduced IIS, illustrated by increased dFoxo mRNA levels found in rpd3 mutant flies, and lack of increased resistance to H2O2 and shorter lifespan observed in flies double mutant for dFoxo and rpd3, compared to single rpd3 mutant flies [18]. This is in agreement with findings that overexpression of nuclear localized dFoxo mediates increased resistance to oxidative stress in flies [38]. Furthermore, flies treated with PBA, a HDAC1 inhibitor, have increased expression of genes that have been implicated in response to oxidative stress such as glutathione S-transferase and SOD [16]. PBA-treated flies have increased climbing activity, increased resistance to starvation and to paraquat, and extended lifespan [16].
Several findings presented here further confirm that rpd3 reduction extends longevity by a mechanism that partially overlaps with DR but has also distinct characteristics. rpd3def male flies have an increased peak of spontaneous physical activity. However, rpd3 flies have no changes in fecundity, total spontaneous physical activity, and are more resistance to starvation and oxidative stress compared to controls. Our data illustrates how complex interactions between reduced rpd3 levels and its downstream targets results in various phenotypic changes at the organismallevel.
Acknowledgments
We would like to thank Suzanne Kowalski, Alexander Pokorski, Bhavin Gupta, and Ryan Rogers for their help in the lab. We thank Dr. Stewart Frankel for critical reading of the manuscript. This work was supported by grant from the NIH (AG023088 to B.R.) and UCONN Health (to B.R.). B. Rogina is a recipient of a Glenn Award for Research in Biological Mechanisms of Aging.
References
[1] | Weindruch RH , Walford RL . The Retardation of Aging and Disease by Dietary Restriction. Springfield, IL, C.C. Thomas. (1988) . |
[2] | Fontana L , Partridge L . Promoting health and longevity through diet: From model organisms to humans. Cell. (2015) ;161: (1):106. |
[3] | Fontana L , Coleman RJ , Holloszy JO , Weindruch R . Calories restriction in non-human and human primates. In Handbook of The Biology of Aging. Eds. Masoro EJ and Austad SN . Academic Press. (2010) , p. 447. |
[4] | Seto E , Yoshida M . Erasers of histone acetylations: The histone deacetyase enzymes Cold Spring Harb. Perspect Biol. (2014) ;6: :a08713. |
[5] | Murphy M , Ahn J , Walker KK , Hoffman WH , Evans RM , Levine AJ , George DL . Transcriptional repression by wild-type p53 utilizes histone deacetylases, mediated by interaction with mSin3a. Gen Devel. (1999) ;13: (19):2490. |
[6] | Juan LJ , Shia WJ , Chen MH , Yang WM , Seto E , Lin YS , Wu CW . Histone deacetylases specifically down-regulate p53-dependent gene activation. J Biol Chem. (2000) ;275: (27):20436. |
[7] | Woods J , Rogina B . The effects of Rpd3 on fly metabolism, health and longevity. Exp Gerontol. (2016) ;8: (11):3028. |
[8] | Marks A , Breslow R . Dimethyl sulfoxide to vorinostat: Development of this histone deacetylase inhibitor as an anticancer drug. Nature Biotechnol. (2007) ;25: (1):84. |
[9] | Ganai SA , Ramadoss M , Mahadevan V . Histone deacetylase (HDAC) inhibitors – emerging roles in neuronal memory, learning, synaptic plasticity and neural regeneration. Curr Neuropharmacol. (2016) ;14: (1):55. |
[10] | Kim S , Benguria CY , Lai SM , Jazwinski M , Modulation of life-span by histone deacetylase genes in Saccharomyces cerevisiae. Mol Biol Cell. (1999) ;10: :3125. |
[11] | Rogina B , Helfand SL , Frankel S . Longevity regulation by Drosophila rpd3 deacetylase and caloric restriction. Science. (2002) ;298: (5599):1745. |
[12] | Pallos J , Bodai L , Lukacsovich T , Purcell JM , Steffan JS , Thompson LM , Marsh JJ . Inhibition of specific HDACs and sirtuins suppresses pathogenesis in a Drosophila model of Huntington’s disease. Hum Mol Genet. (2008) ;17: (23):3767. |
[13] | Rogina B , Helfand SL . Sir2 mediates longevity in the fly through a pathway related to calorie restriction. Proc Natl Acad Sci USA. (2004) ;101: (45):15998. |
[14] | Frankel S , Woods J , Ziafazeli T , Rogina B . RPD3 histone deacetylase and nutrition have distinct but interacting effects on Drosophila longevity. Aging. (2015) ;7: (12):1112. |
[15] | Kopp ZA , Hsieh JL , Li A , Wang W , Bhatt DT , Lee A , Kim SY , Fan D , Shah V , Siddiqui E , Ragam R , Park K , Ardeshna D , Park K , Wu R , Parikh H , Parikh A , Lin YR , Park Y . Heart-specific Rpd3 downregulation enhances cardiac function and longevity. Aging. (2015) ;7: (9):648. |
[16] | Kang H-L , Benzer S , Min K-T . Life extension in Drosophila by feeding a drug. Proc Natl Acad Sci USA. (2002) ;99: (2):838. |
[17] | Bauer J , Antosh M , Chang C , Schorl C , Kolli S , Neretti N , Helfand SL . Comparative transcriptional profiling identifies takeout as a gene that regulates life span. Aging. (2010) ;2: (5):298. |
[18] | Woods J , Ziafazeli T , Rogina B . Rpd3 interacts with insulin signaling in Drosophila longevity extension. Aging. (2016) ;8: (11):3028. |
[19] | Gershman B , Puig O , Hang L , Peitzch RM , Tatar M , Garofalo RS . High-resolution dynamics of the transcriptional response to nutrition in Drosophila: A key role for dFOXO. Physiol Genomics. (2007) ;29: (1):24. |
[20] | Mottus R , Sobel RE , Grigliatti TA . Mutational analysis of a histone deacetylase in Drosophila melanogaster: Missense mutations suppress gene silencing associated with position effect variegation. Genetics. (2000) ;154: (2):657. |
[21] | Woods J , Kowalski S , Rogina B . Determination of the spontaneous locomotor activity in Drosophila melanogaster. J Vis Exp. (2014) ;86: :1–6. doi: 10.3791/51449 |
[22] | Bross TG , Rogina B , Helfand SL . Behavioral, physical, and demographic changes in Drosophila populations through dietary restriction. Aging Cell. (2005) ;4: (6):309. |
[23] | Rogina B , Wolverton T , Bross TG , Chen K , Müller H-G , Carey JR . Distinct biological epochs in the reproductive life od female Drosophila melanogaster. Mech. Aging Dev. (2007) ;128: (9):477. |
[24] | Rogers RP , Rogina B . Increased mitochondrial biogenesis preserves intestinal stem cell homeostasis and contributes to longevity in Indy mutant flies. Aging. (2014) ;6: (4):335. |
[25] | Parashar V , Rogina B . dSir2 mediates the increased spontaneous physical activity in flies on calorie restriction. Aging. (2009) :1: (6):529. |
[26] | Parashar V , Rogina B . dSir2 and fly mobility. Cell Cycle. (2010) ;9: (3):433. |
[27] | Chen D , Steele AD , Lindquist S , Guarente L . Increase in activity during calorie restriction requires Sirt1. Science. (2005) ;310: (5754):1641. |
[28] | Burger JM , Hwangbo DS , Corby-Harris V , Promislow DE . The functional costs ad benefits of dietary restriction in Drosophila. Aging Cell. (2007) ;6: (1):63. |
[29] | Rion S , Kawecki TJ . Evolutionary biology of starvation resistance: What we have learned from Drosophila. J Evol Biol. (2007) ;20: (5):1655. |
[30] | Piper MD , Skorupa D , Partridge L . Diet, metabolism and life span in Drosophila/Exp. Gerontol. (2005) ;40: (11):857. |
[31] | Wang PY , Neretti N , Whitaker R , Hosier S , Chang C , Lu D , Rogina B , Helfand SL . Long-lived Indy and calorie restriction interact to extend life span. Proc Natl Acad Sci USA. (2009) ;106: (23):92629267. |
[32] | Parashar V , Frankel S , Lurie AG , Rogina B . The effects of age on radiation resistance and oxidative stress in adult Drosophila melanogaster . Rad Res. (2008) ;169: (6):707. |
[33] | Skorupa DA , Dervisefendic A , Zwiener J , Pletcher SD . Dietary composition specifies consumption, obesity, and lifespan in Drosophila melanogaster. Aging Cell. (2008) ;7: (4):478. |
[34] | Djawdan M , Chippindale AK , Rose MR , Bradley TJ . Metabolic reserves ad evolved stress resistance in Drosophila melanogaster. Physiol Zool. (1998) ;71: (5):584. |
[35] | Broughton SJ , Piper MD , Ikeya T , Bass TM , Jacobson J , Driege Y , Martinez P , Hafen E , Withers DJ , Leevers SJ , Partridge L . Longer lifespan, altered metabolism, and stress resistance in Drosophila from ablation of cells making insulin-like ligands. Proc Natl Acad Sci USA. (2005) ;102: (8):3105. |
[36] | Guan KL , Xiong Y . Regulation of intermediary metabolism by protein acetylation. Trends Bioch Sci. (2011) ;36: (2):108. |
[37] | Zhao S , Xu W , Jiang W , Yu W , Lin Y , Zhang T , Yao J , Zhou L , Zeng Y , Li H , Li Y , Shi J , An W , Hancock SM , He F , Qin L , Chin J , Yang P , Chen X , Lei Q , Xiong Y , Guan KL . Regulation of cellular metabolism by protein lysine acetylation. Science. (2010) 3275968 1000. |
[38] | Jünger MA , Rintelen F , Stocker H , Wasserman JD , Végh M , Radimerski T , Greenberg ME , Hafen E . The Drosophila Forkhead transcriptional factor FOXO mediates the reduction in cell number associated with reduced insulin signaling. J Biol. (2003) ;2: :20. |