In vivo maltase and sucrase inhibitory activities of five underutilized Nigerian edible fruits
Abstract
BACKGROUND: Inhibition of intestinal maltase and sucrase prevents postprandial blood glucose excursions which is beneficial in ameliorating diabetes-associated complications.
OBJECTIVE: In this study, the inhibitory effects of fruit extracts of Parinari macrophylla, Detarium microcarpum, Ziziphus spina-christi, Z. mairei and Parkia biglobosa were investigated against intestinal maltase and sucrase.
METHODS: Rats were given co-administration of the fruit extracts with maltose or sucrose and blood glucose levels were measured at 0, 30, 90 and 120 min.
RESULTS: The glucose-time curves indicated that all the fruits had the most potent inhibitory effects on both maltase and sucrase within the first 30 min. The computed Area Under the Curves (AUC0–120) for all the fruits indicated more potent inhibitory effects against intestinal maltase than sucrase. The ED50 range for the fruits extract against maltase and sucrase were 647.15–1118.35 and 942.44–1851.94 mg/kg bw respectively.
CONCLUSION: The data suggests that the fruits could prevent postprandial hyperglycemia via inhibition of intestinal maltase and sucrase.
1Introduction
Diabetes mellitus is a heterogeneous group of metabolic disorder characterized by high blood glucose levels (hyperglycemia) with alterations in carbohydrate, lipid and protein metabolism resulting from defects in insulin secretion and/or action [1]. According to the International Diabetes Federation (IDF), about 382 million people are living with diabetes, a figure projected to increase to 592 million by the year 2035 [2]. Diabetes mellitus is primarily classified in to type 1 diabetes (insulin dependent) and type 2 diabetes (insulin independent) with the latter accounting for more than 90% of all diabetic cases in the world [2]. Type 2 diabetes (T2D) is a heterogeneous disorder characterized by a progressive decline in insulin action (insulin resistance), followed by the inability of pancreatic β-cells to compensate for insulin resistance (β-cell dysfunction) which leads to hyperglycemia [3].
Hyperglycemia is the hallmark of diabetes mellitus that plays vital roles in all aspects of the T2D pathology including macrovascular and microvascular complications associated with the disease [4, 5]. Also, postprandial hyperglycemia is known to immensely contribute to the overall glycemic control in T2D patients [6]. Therefore, the control of postprandial hyperglycemia is considered to be important in the treatment of T2D and prevention of its associated complications. Currently, the major therapeutic strategy for the management of postprandial hyperglycemia is through delaying the release of glucose by inhibiting carbohydrate hydrolyzing enzyme α-glucosidase (EC 3.2.1.20) in the digestive tract. The α-glucosidase is a multifunctional enzyme with distinct maltase and sucrase activities [6]. Inhibition of these enzymatic activities would retard gastrointestinal absorption of dietary carbohydrates by restricting the breakdown of linear or branched oligosaccharide units (like α-limit dextrins, maltose, sucrose, maltotriose) to produce glucose thereby preventing glucose absorption into the blood stream [7]. Consequently, this will ultimately reduce the flow of glucose from dietary carbohydrates into the bloodstream, thereby diminishing the postprandial hyperglycemia. Unfortunately, the leading α-glucosidase inhibitors, acarbose and miglitol, are often reported to produce diarrhea and other intestinal disturbances, with bloating, flatulence, cramping and abdominal pain occurring concurrently [8]. Randomized controlled trials with glucosidase inhibitors report these gastrointestinal side effects as the most common reason for non-compliance and early subject withdrawal [9]. More importantly, these drugs, and indeed all other anti-diabetic drugs, are expensive and not readily available to the majority of the T2D patients living in rural communities. This is also in spite of the high prevalence of the disease in such areas. Luckily, these communities are also naturally endowed with tremendous plant foods, including fruits pulps, which could be exploited for the management of postprandial hyperglycemia.
The fruit of Parinari macrophylla Sabine (chrysobalanaceae), commonly called gingerbread plum, is indigenous to northern Nigeria and used in a variety of ways. It is eaten either fresh, boiled or after pounded with water to create a colorful red lemonades-like juice [10]. Detarium microcarpum Guill. & Perr. (Leguminosae) fruit pulp is edible and very rich in crude fibre, vitamins C and E, folic acid, mineral elements and carbohydrates [11]. Also, the D. microcarpum fruit pulp was reported to affect some hematological indices in rats [12]. Ziziphus spina-christi (L.) Desf. And Ziziphus mairei Lam. belong to the Rhamnaceae family and are virtually cosmopolitan in warm-temperate and subtropical region. The plants have nutritiously edible fruits with various applications in folk medicine. The fruits are used locally to treat skin cuts and pulmonary ailments [13]. Furthermore, the leaves of Z. spina-christi have been shown to possess blood glucose lowering effect in diabetic rats [14]. Parkia biglobosa (Jacq.) G. Don (Leguminosae) is widely distributed in West Africa and utilized for both nutritional and medicinal purposes.The fruits of the plant are used as routine flouring and seasoning agent for traditional dishes [15]. Moreover, the seeds of the plant have been shown to possess ameliorative effects on alloxan induced diabetes in experimental animals [16].
All the above mentioned fruits are indigenous to northern Nigeria and other semi arid sub-Saharan Africa which are mostly consumed during period of grain shortage as dietary supplements among rural dwellers [10]. Unfortunately, the health benefits of these fruits, especially with respect to diabetes management, have not been documented. In this study, we report the inhibitory effects of these selected fruits on intestinal maltase and sucrase activities in non-diabetic animals. This is with a view to determine, whether or not, consumption of these fruits would be beneficial to diabetic patients in controlling postprandial hyperglycemia which would go a long way to provide a scientific basis to encourage diabetic patients, especially in rural communities, to supplement their carbohydrate rich meals with these fruits. It is also hoped that this study would serve as a basis for the development of a standardised anti-diabetic supplements or nutraceutical from these fruits.
2Materials and methods
2.1Fruit samples
Fresh riped fruit samples of P. macrophylla, D. microcarpum, Z. spina-christi, Z. mairei and P. biglobosa were purchased at a local market at Wudil local government area of Kano state, Nigeria in September-October, 2014. Subsequently, the fruit samples were immediately washed and shade-dried for three weeks to constant weights. Thereafter, the pulps of the dried fruit samples were carefully excised and pounded to fine powder using laboratory mortar and pestle. The samples were stored in air-tight dry containers until needed.
2.2Preparation of aqueous extracts
Thirty grams of each of the fine powdered fruit samples were dissolved in 500 ml of distilled water and allowed to stand for 24 h, after which the samples were filtered through cheese cloth. The filtrates were further filtered through Whatmann filter paper (No. 1) and the resultant extracts were evaporated in a water bath at 50°C to obtain the crude aqueous extracts.
2.3Experimental animals
The protocol employed met the guidelines of the Good Laboratory Practice (GLP) regulations of World Health Organization. Also, the rules and regulations of Ahmadu Bello University, Zaria Ethics Committee were duly followed. Apparently healthy albino rats (Wistar strain) weighing 140–200 g were procured from the animal house of the Nigerian Institute of Trypanosomiasis and Onchorcerciasis Research, Kaduna, Nigeria. The animals were maintained in well ventilated polycarbonated laboratory cages (25 ± 2°C, 12 h light–dark cycle) and fed on a standard rat pellet diet (Vital Feeds, Jos, Nigeria) with drinking water ad libitum during the entire experimental period.
2.4Animal grouping
After one week adaptation period, the rats were randomly divided in to twelve groups of five animals each namely; NC: Normal Control, PM100: treated with a low dose (100 mg/kg bw) of P. macrophylla fruit extract, PM200: treated with a high dose (200 mg/kg bw) of P. macrophylla fruit extract, DM100: treated with a low dose (100 mg/kg bw) of D. microcarpum fruit extract, DM200: treated with a high dose (200 mg/kg bw) of D. microcarpum fruit extract, ZS100: treated with a low dose (100 mg/kg bw) of Z. spinachristi fruit extract, ZS200: treated with a high dose (200 mg/kg bw) of Z. spinachristi fruit extract, ZM100: treated with a low dose (100 mg/kg bw) of Z. mairei fruit extract, ZM200: treated with a high dose (200 mg/kg bw) of Z. mairei fruit extract, PB100: treated with a low dose (100 mg/kg bw) of P. biglobosa fruit extract, PB200: treated with a high dose (200 mg/kg bw) of P. biglobosa fruit extract, Acarbose: treated with 100 mg/kg bw of a standard drug, acarbose.
2.5In vivo maltase and sucrase inhibitory activity of the fruit extracts
The rats in all the experimental groups were fasted overnight for 12 h and after which, the animals were orally administered with maltose or sucrose (2 g/kg bw) which was immediately followed by the oral administration of the respective dose of the fruit extract. However, in addition to the similar maltose or sucrose administrations, the animals in NC and acarbose groups were orally administered with distilled water and acarbose respectively, instead of the fruit extracts. The blood glucose concentrations of all animals were measured in the blood collected from the tail vein by using a portable glucometer (Glucoplus Inc., Saint-Laurent, Quebec, Canada) at 0 (just before maltose or sucrose ingestion), 30, 90 and 120 min after the dose of maltose or sucrose. It is noteworthy to state that a seven day wash out period was given between the two experiments for maltase and sucrase inhibitory effects of the fruit extracts. This was also done to minimize the esophageal as well as overall stress to the animals. The area under the curve (AUC) for all the experimental groups was calculated according to the following formula:
2.6Statistical analysis
All data were presented as the mean ± standard deviation of five animals. Data were analyzed by using a statistical software package (SPSS for Windows, version 18, IBM Corporation, NY, USA) using Tukey’s-HSD multiple range post-hoc test. Values were considered significantly different at P < 0.05.
3Results
The fruit pulps of P. macrophylla, D. microcarpum, Z. spinachristi, Z. mairei and P. biglobosa were found to significantly (P < 0.05) inhibit the activity of intestinal maltase in a dose dependent pattern which was observed as significantly lowered (P < 0.05) blood glucose level (after maltose loading) in the fruit extracts-treated groups (Fig. 1). However, the extracts of P. macrophylla and P. biglobosa fruits at 100 mg/kg bw, did not cause a significant (P > 0.05) inhibitions of the enzyme at 90 and 120 min (Fig. 1A and E). In fact, all the extracts of the fruits demonstrated more potent inhibitory effects against the intestinal maltase within the first 30 min of the experiment. Moreover, the data from the computed area under the maltose tolerance curves revealed that the 200 mg/kg bw of all fruits extract, except D. microcarpum, produced significantly lower AUC values than the normal control and acarbose-treated rats (Table 1). The fruit extracts of Z. mairei and Z. spinachristi showed lower AUC values in the maltose tolerance curves than other extracts when the data was compared for both 100 and 200 mg/kg bw. Interestingly, the computed ED50 of the extracts also demonstrated that the Z. mairei fruit had the least ED50 value of 647.15 mg/kg bw among the fruits, followed by Z. spinachristi fruit with an ED50 value of 661.89 mg/kg bw for inhibiting maltase (Table 3).
In the sucrase inhibition studies, there was a sharp and significant (P < 0.05) rise in blood glucose concentrations in the normal and acarbose-treated rats at 30 min after the sucrose loading (Fig. 2). However, all the fruits extract significantly (P < 0.05) inhibit the intestinal sucrase activities within the first 30 min which manifested as significantly (P < 0.05) lowered blood glucose levels in the extracts-treated groups compared with normal control group within the time period. There were no significant differences (P > 0.05) in the blood glucose concentrations of all experimental groups at 90 and 120 min. The computed area under the sucrose tolerance curves did not show significant (P > 0.05) differences between the normal control and the extracts-treated groups (Table 2). However, the Z. spinachristi had the least ED50 value of 982.44 mg/kg bw followed by Z. mairei with a value of 1512.76 mg/kg bw (Table 3). In fact, the ED50 value for inhibiting sucrase is in the order Z. spinachristi < Z. mairei < P. biglobosa < D. microcarpum < P. macrophylla.
4Discussion
Targeting of postprandial hyperglycemia has been suggested to be an important control strategy especially in preventing cardiovascular mortality in diabetics [17]. The rise in plasma glucose in normoglycemic individuals after consumption of carbohydrate begins at about 10 min and reaches maximum in ∼60 min [18]. In this study, the peak in plasma glucose level is observed within the first 30 min of maltose or sucrose dose, after which a decrease was observed until the level returns to normal. Moreover, the blood glucose lowering effect of the extracts appears to be higher with increasing dose (except D. microcarpum). The observed effects of the fruits extract within the initial 30 min suggest a fast inhibitory activity against intestinal maltase and sucrase. From a therapeutic point of view, this fast action is desirable in preventing postprandial rise in blood glucose for the reason aforementioned. It was also evident from the computed AUC data that all the extracts (at 200 mg/kg bw) have better inhibitory activity on maltase than sucrase. This could possibly be due to the presence of additional phytochemicals in the extracts that specifically target maltase in addition to non-specific α-glucosidase inhibitors. It is possible that the α-glucosidase inhibitors in the fruits have more preference towards inhibiting the hydrolysis of α (1–4) than α (1-2) glycosidic bonds.
On a general note, the fruits of either of the two Ziziphus species have the lowest ED50 values for inhibiting both maltase and sucrase among the fruits. Indeed, various studies have reported on the anti-diabetic properties of different parts of Ziziphus species [14, 19] with the exception of the fruit which is the one commonly consumed. Among the frequently cited phytochemicals responsible for the antihyperglycemic properties are phenolics, alkaloids, flavonoids, terpenoids and glycosides [20]. It is interesting to note that a phloretin derivative has been identified in the fruits of Z. spina-christi as well as other Ziziphus species [21]. Phloretin is a known hexose transport inhibitor across the basolateral membrane of the small intestine which has been employed for experimental purposes [22]. Hence, it is logical to hypothesize the involvement of inhibition of sugar transport in addition to enzyme inhibition in the mechanism of action of these fruits.
5Conclusion
In conclusion, the fruits of Z. spinachristi, Z. mairei, P. biglobosa, D. microcarpum, and P. macrophylla possess intestinal maltase and sucrase inhibitory effects in rats. Because these fruits are edible and safe, frequent consumption would be a rational control measure in preventing postprandial hyperglycemia especially in diabetics. The data also suggested that the development of a standardised anti-hyperglycemic supplements or nutraceuticals from these fruits could be possible. On the other hand, isolation of the specific phytochemicals responsible for the inhibition of sugar hydrolysis and/or transport should be a theme for future research. Moreover, the findings of this study are a preliminary proof of concept that need to be further validated with higher number of animals and eventually in clinically relevant human-based studies. This would be in the interest of the global effort to provide a broad spectrum of blood glucose lowering agents in order to curtail the menace of hyperglycemia-related complications.
Conflict of Interest
Authors declare no conflict of interest for the study conducted.
Acknowledgments
Authors are grateful to Mr. Yakubu Otaru and Abdulkadir Rabiu Salisu of the Department of Microbiology, Bayero University, Kano for their technical assistance. The authorities of Kano University of Science and Technology, Wudil are also acknowledged for awarding a visiting lecturer position to Dr. M. A. Ibrahim.
References
[1] | American Diabetes Association. Diagnosis and classification of diabetes mellitus. Diabetes Care. (2010) ;36: (Suppl 1):S67–S74. |
[2] | Guariguata L , Whiting DR , Hambleton I , Beagley J , Linnenkamp U , Shaw JE . Global estimates of diabetes prevalence for 2013 and projections for 2035. Diabetes Res Clin Pract. (2014) ;103: (2):137–149. |
[3] | Henriksen EJ , Diamond-Stanic MK , Marchionne EM . Oxidative stress and the etiology of insulin resistance and type 2 diabetes. Free Radic Biol Med. (2011) ;51: :993–999. |
[4] | Brownlee M . Biochemistry and molecular cell biology of diabetic complications. Nature. (2001) ;414: :813–820. |
[5] | Wright EJ , Scism-Bacon JL , Glass LC . Oxidative stress in type 2 diabetes: The role of fasting and postprandial glycaemia. Int J Clin Pract. (2006) ;60: (3):308–314. |
[6] | Priscilla DH , Roy D , Suresh A , Kumar V , Thirumurugan K . Naringenin inhibits α-glucosidase activity: A promising strategy for the regulation of postprandial hyperglycemia in high fat diet fed streptozotocin induced diabetic rats. Chem Biol Interact. (2014) ;210: :77–85. |
[7] | Lee BH , Eskandari R , Jones K , Reddy KR , Quezada-Calvillo R , Nichols BL , et al. Modulation of starch digestion for slow glucose release through “toggling” of activities of mucosal α-glucosidases. J Biol Chem. (2012) ;287: :31929–31938. |
[8] | Fujisawa T , Ikegami H , Inoue K , Kawabata Y , Ogihara T . Effect of two α-glucosidase inhibitors, voglibose and acarbose, on postprandial hyperglycemia correlates with subjective abdominal symptoms. Metabolism. (2005) ;54: :387–390. |
[9] | Neuser D , Benson A , Bruckner A , Goldberg RB , Hoogwerf BJ , Petzinna D . Safety and tolerability of acarbose in the treatment of type 1 and type 2 diabetes mellitus. Clin Drug Investig. (2005) ;25: (9):579–587. |
[10] | Amza T , Amadou I , Kamara MT , Zhu K , Zhou H . Chemical and nutrient analysis of gingerbread plum (Neocarya macrophylla) seeds. Adv J Food Sci Technol. (2010) ;2: (4):191–195. |
[11] | Oibiokpa FI , Adoga GI , Saidu AN , Shittu KO . Nutritional composition of Detarium microcarpum fruit. Afr J Food Sci. (2014) ;8: (6):342–350. |
[12] | Wahedi JA , David LD . Effect of Detarium microcarpum fruit pulp on the hematological indices of rats in Mubi Adamawa State, Nigeria. Global J Biol Agric Health Sci. (2013) ;2: (3):76–80. |
[13] | Adzu B , Amos S , Wambebe C , Gamaniel K . Antinociceptive activity of Ziziphus spina-christi root bark extract. Fitoterapia. (2001) ;72: :334–350. |
[14] | Abdel-Zaher AO , Salim SY , Assaf MH , Abdel-Hady RH . Antidiabetic activity and toxicity of Zizyphus spina-christi leaves. J Ethnopharmacol. (2005) ;101: :129–138. |
[15] | Omojola MO , Afolayan MO , Adebiyi AB , Orijajogun JO , Thomas SA , Ihegwuagu NE . Further physicochemical characterization of Parkia biglobosa (Jacq). Benth fruit pulp as a mineral supplement. Afr J Biotechnol. (2010) ;10: (75):17285–17264. |
[16] | Odetola AA , Akinloye O , Egunjobi C , Adekunle WA , Ayoola AO . Possible antidiabetic and antihyperlipidaemic effect of fermented Parkia biglobosa (Jacq) extractin alloxan-induced diabetic rats. Clin Exp Pharmacol Physiol. (2006) ;33: (9):808–812. |
[17] | Inzucchi SE . Oral antihyperglycemic therapy for type 2 diabetes: Scientific review. JAMA. (2002) ;287: (3):360–372. |
[18] | American Diabetes Association. Postprandial blood glucose. Diabetes Care. (2001) ;24: (4):775–778. |
[19] | Jarald EE , Joshi SB , Jain DC . Antidiabetic activity of extracts and fraction of Zizyphus mauritiana . Pharm Biol. (2009) ;47: (4):328–334. |
[20] | Niamat R , Khan MA , Khan KY , Ahmed M , Mazari P , Ali B , et al. A review on Zizyphus as antidiabetic. J Appl Pharm Sci. (2012) ;02: (3):177–179. |
[21] | Pawlowska AM , Camangi F , Bader A , Braca A . Flavonoids of Zizyphus jujube L. and Zizyphus spina-christi (L.) Willd (Rhamnaceae) fruits. Food Chem. (2009) ;112: (4):858–862. |
[22] | Csaky TZ , Fischer E . Intestinal sugar transport in experimental diabetes. Diabetes. (1981) ;30: (7):568–574. |
Figures and Tables
Fig.1
In vivo inhibitory effects of P. macrophylla (A), D. microcarpum (B), Z. spinachristi (C), Z. mairei (D) and P. biglobosa (E) fruits on intestinal maltase activity. Data are presented as mean ± SD of five animals. a - cValues with different letters for a given time are significantly different from each other group of animals (Tukey’s-HSD multiple range posthoc test, P < 0.05). NC is normal control; PM, DM, ZS, ZM and PB are group of rats given co-administration of maltose with P. macrophylla, D. microcarpum, Z. spinachristi, Z. mairei and P. biglobosa fruit extract respectively. The 100 and 200 merged with the above-mentioned abbreviations refer to the dose of 100 and 200 mg/kg bw respectively, of the fruit given to the animals.
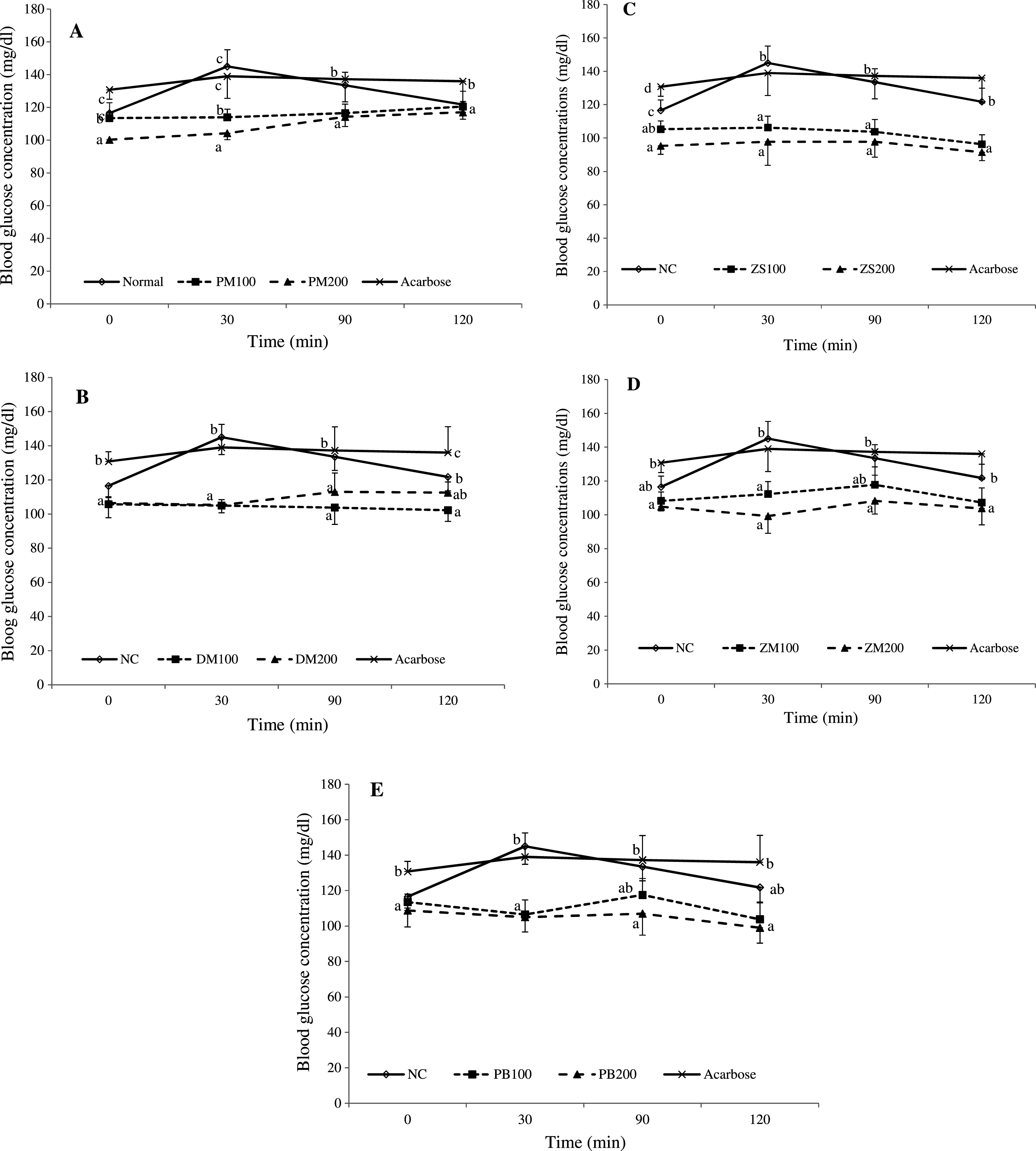
Fig.2
In vivo inhibitory effects of P. macrophylla (A), D. microcarpum (B), Z. spinachristi (C), Z. mairei (D) and P. biglobosa (E) fruits on intestinal sucrase activity. Data are presented as mean ± SD of five animals. a - cValues with different letters for a given time are significantly different from each other group of animals (Tukey’s-HSD multiple range posthoc test, P < 0.05). NC is normal control; PM, DM, ZS, ZM and PB are group of rats given co-administration of sucrose with P. macrophylla, D. microcarpum, Z. spinachristi, Z. mairei and P. biglobosa fruit extract respectively. The 100 and 200 merged with the above-mentioned abbreviations refer to the dose of 100 and 200 mg/kg bw respectively, of the fruit given to the animals.
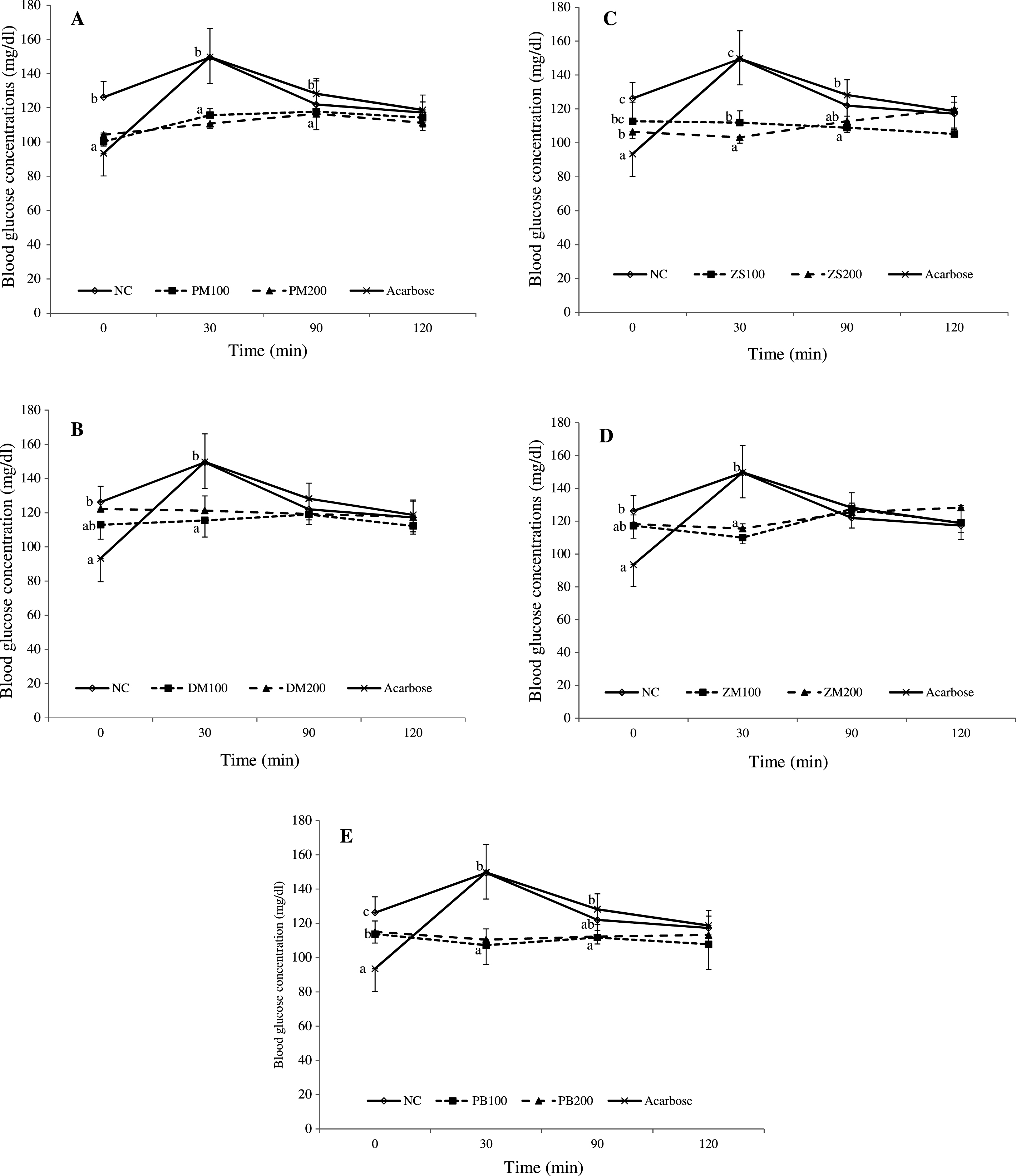
Table 1
Computed Area Under the Curve (AUC) values for the maltose and sucrose tolerance tests over the 2 hour period
Groups | AUC0–120 min (Minutes·mg/dl) | |
Maltose | Sucrose | |
NC | 16106.25 ± 2028.10c | 15870.00 ± 2034.39ab |
PM100 | 13882.50 ± 404.36bc | 13875.00 ± 914.14ab |
PM200 | 13166.25 ± 347.86b | 13458.75 ± 449.50a |
DM100 | 12513.75 ± 1141.04ab | 13931.25 ± 1904.77ab |
DM200 | 13106.25 ± 1377.22abc | 14418.75 ± 1182.69ab |
ZS100 | 12472.50 ± 728.69ab | 13215.00 ± 1555.75ab |
ZS200 | 11576.25 ± 625.01a | 13113.75 ± 956.42ab |
ZM100 | 13582.50 ± 976.38abc | 14231.25 ± 464.60b |
ZM200 | 12565.00 ± 911.02ab | 14542.50 ± 263.13b |
PB100 | 13338.75 ± 1185.19abc | 13177.50 ± 891.25ab |
PB200 | 12656.25 ± 1682.43ab | 13447.50 ± 654.75a |
Acarbose | 16432.50 ± 1358.53c | 15693.75 ± 1316.26ab |
All values are presented as mean ± SD of five animals. a - cValues with different letters along a column are significantly different from each other (Tukey’s-HSD multiple range posthoc test, P < 0.05). NC is normal control; PM, DM, ZS, ZM and PB are group of rats given co-administration of the disaccharide (maltose or sucrose) with P. macrophylla, D. microcarpum, Z. spinachristi, Z. mairei and P. biglobosa fruit extract respectively. The 100 and 200 merged with the above-mentioned abbreviations refer to the dose of 100 and 200 mg/kg bw respectively, of the fruit given to the animals.
Table 2
The ED50 values for inhibiting intestinal maltase and sucrase by the selected Nigerian fruits
ED50 (mg/kg bw) | ||
Maltase | Sucrase | |
PM | 1118.35 | 1851.94 |
DM | 742.55 | 1677.57 |
ZS | 661.89 | 982.44 |
ZM | 647.15 | 1512.76 |
PB | 858.35 | 1643.62 |
PM, DM, ZS, ZM and PB refers to P. macrophylla, D. microcarpum, Z. spinachristi, Z. mairei and P. biglobosa fruit extract respectively.