Dioscorea esculenta increase cytochrome c oxidase 1 expression and adenosine triphosphate in diabetic rats
Abstract
BACKGROUND:
The low cytochrome c oxidase (COX)-1 activity was associated with reduction of adenosin triphosphate (ATP) production in diabetes mellitus (DM) individuals. Interestingly, the secondary metabolite of Dioscorea esculenta (lesser yam) fermentation in gut can increase ATP production.
OBJECTIVES:
The aim of this study was to evaluate the effect of Lesser yam diet on ATP level and COX-1 expression in type 2 diabetic rats.
METHODS:
Thirty Wistar rats were divided into 5 groups: (1) normal rats (N), (2) diabetic rats (DM), (3) diabetic rats with lesser yam 200 mg/kg BW (DMT1), (4) diabetic rats with lesser yam 400 mg/kg BW (DMT2), (5) diabetic rats with lesser yam 800 mg/kg BW (DMT3). The diabetic rats were induced by nicotinamide and streptozotocin and had plasma glucose more than 126 mg/dL. ATP was measured before and after 4 weeks of intervention. COX-1-was determined at skeletal muscle, heart, liver, brown adipose tissue and kidney after intervention using immuno-histochemistry (IHC).
RESULTS:
Fasting blood glucose was reduced in all intervention groups compared to DM group (p = 0.016). ATP level was significantly increased in DMT1 group and slightly higher in DMT2 and DMT3 compared with the negative control (p > 0.05). After the intervention, COX-1 protein expression was higher in kidney, liver and skeletal muscle in diabetic rats received lesser yam compared to DM group (p < 0.05).
CONCLUSION:
In this study we found that lesser yam reduced fasting blood glucose, increase plasma ATP and expression of COX-1 protein.
1Background
Impairment in energy metabolism due to decreased mitochondrial activity has been observed in type 2 diabetes mellitus [1, 2]. Several studies have found a reduction in ATP synthesis both ininsulin-resistant and type 2 diabetes mellitus individuals [3–5]. The ATP reduction was caused by reduced activity of mitochondria enzyme for oxidative phosphorylation; particularly cytochrome C-oxidase (COX) [6]. COX is a key mitochondria enzyme, which is important for ATP generation through redox-linked proton pump [7, 8]. In human, COX consists of 13 subunits with subunit 1 and 2 act as catalytic region of COX enzyme [8]. Reduced activity of COX enzyme has been reported by Akude et al. [9] and Morino et al. [10] indicating the impairment in energy metabolism in diabetes condition.
Lesser yam (Dioscorea esculenta) is a traditional food and ubiquitous in Java, Indonesia. It is reported that resistant starch in local Indonesian lesser yam is 10.4 mg/dry weight [11]. Resistant starch is component in diet which has the highest yield of butyrate by colonic fermentation compared with non-starch polysaccharides [12]. Butyric acid is secondary metabolite product of gut fermentation which has an activity as histone deacetylase (HDAC) inhibitor [13]. Inhibiton of HDAC by butyrate has been shown in improving energy metabolism through activation of several transcription factors important in mitochondrial biogenesis, which will ameliorate insulin sensitivity in diabetic condition [13–15]. Therefore, in this study we want to investigate whether lesser yam has potential for improving energy metabolism in diabetic condition.
2Methods
2.1Animals and induction of diabetes
Male Wistar rats aged 2 months old with body weight between 180–200 gram were purchased from Unit Pengembangan Hewan Percobaan (UPHP), Institut Pertanian Bogor, Indonesia. Thirty rats were placed inside individual plastic cages with 12 hours light cycle. Rats were adapted 1 week prior to study. After adaptation, rats were fasted overnight before induction of type 2 diabetes mellitus according to Rabbani et al. [16]. In brief, rats were injected with nicotinamide (230 mg/kg body weight) and after 15 minutes, streptozotocin were introduced to rats intraperitoneally (65 mg/kg body weight). Rats with glucose serum level reached more than 126 mg/dL were selected for the study. Streptozotocin and nicotinamide were purchased from Sigma (USA). This study was approved by the Ethics Committee of Faculty of Medicine, Universitas Gadjah Mada, Indonesia with number of etichal clearence Ref: KE/FK/641/EC.
2.2Preparation of lesser yam flour
Lesser yam flour was prepared according to Richana and Sunarti [17]. In brief, lesser yam was purchased from local market and peeled, washed and cutted in small pieces. Lesser yam then dried in the oven (50° C for 24 hour), milled,sifted (80 mesh), and stored in vacuum plastic bag. Resistant starch, soluble fiber and insoluble fiber were analyzed in lesser yam flour using a method conducted by Goñi et al. [18].
2.3Experimental study
Rats then divided into 5 groups including a non-diabetic control rats (N), diabetic control rats (DM), diabetic rats with lesser yam 200 mg/kg body weight (DMT1), diabetic rats with lesser yam 400 mg/kg body weight (DMT2), diabetic rats with lesser yam 800 mg/kg body weight (DMT3). The dose of 400 mg/kg body weight was used based on recommended daily consumption of fiber in normal weight human for 25 gram a day/62.5 kg of adult weight. We used 200 mg/kg body weight was used a the half dosage of normal dose and 800 mg/kg body weight was used as the doubled dose of normal fiber dosage. Those additional two dosages were used in order to evaluate whether this intervention was dose dependent. The flour was incoorperated in the diet of rats. The composition of the animal diet were presented in Table 1. Body weight and food intake were recorded.
2.4Laboratorium analysis
After 4 weeks of intervention, rats were euthanized with cervical dislocation under anesthesia. Skeletal muscle, heart, liver, brown adiposed tissue, and kidney were taken and stored immediately in 10% (v/v) of liquid formalin buffer. Tissue from skeletal muscle, heart, liver, brown adipose tissue and kidney were paraformaldehyde-fixed prior to immunohistochemistry analysis. The tissue then embedded and sectioned by microtom (3μm), and incubated at 50°C overnight. Paraformaldehyde was removed from the tissue by addition of xylol I, xylol II, and xylol III then rehydrated in alcohol with different concentration (absolute, 95% and 75% v/v), 3 minutes each.
After the sections were cooled down, the sections were blocked with universal tracking antibody and incubated with COX-1 antibody for 1 hour. Secondary antibody streptavidin peroxydase and S-(2-aminoethyl)-L-cysteine (AEC) were added as a substrate. Diaminobenzydine (DAB) was used as chromogen. The hematoxylin meyer was used as counterstain, and mounted with E-Z mount. COX-1 antibody was purchased from Cusabio (China). Expression of COX-1 was calculated based on the amount of cells expressing the protein stained using microscope. Blood was drawned from orbital cynus of rats at day 5 of induction and after 4 weeks of intervention. Blood was centrifused immediately to collect serum. Glucose was measured using enzymatic reaction of GOD-PAP (Dyasis, Germany). ATP was measured using Enzyme Linked Immunosorbant Assay (ELISA) method (Bluegene).
2.5Statistical analysis
Statistical analysis was done using GraphPad Prism 6 for Windows (GraphPad Software La Jolla, California USA). Analysis of variance (ANOVA) was used to compare the effect of each interventions (DMT1, DMT2, DMT3 and DM) followed by Tukey HSD. Minimum significance value was set at α= 0.05.
3Results
Lesser yam starch contains 14.29±0.16% of resistant starch, 15.10±0.06% of soluble fiber, and 19.69±0.21% of insoluble fiber per 100 gram of dry weight. As seen in Fig. 1, rats in DMT2 and DMT3 groups have gained less weight compared to DM control (p = 0.033 and p = 0.031 respectively). In addition, rats in DMT2 and DMT3 groups have lower food intake compared to control and diabetic control groups (Fig. 1). Fasting serum glucose was significantly lower in all lesser yam treated groups compared with DM control (p < 0.0001). There is no significant difference (p > 0.05) in fasting serum glucose level between DMT1, DMT2 and DMT3, indicating that all lesser yam intervention groups are effective in lowering serum glucose level in diabetic condition.
Plasma ATP level was not significantly different in the pre test condition (Fig. 1). However, after 4 weeks of intervention, DM group had significantly lower ATP level (p = 0.0025) compared to Control group and slightly lower in all lesser yam groups (Fig. 1). Furthermore, DMT1 group has the highest ATP level compared with other lesser yam group (DMT2 and DMT3).
An Immunohistochemistry was done to analyze the effect of lesser yam intervention to protein expression of COX-1 (Fig. 2). Five organs including liver, heart, brown fat, skeletal muscle and kidney were collected from rats after interventions. From those organs, only liver, skeletal muscle and kidney that were affected by lesser yam intervention. After 4 weeks of intervention, DMT1 increased COX 1 expression in kidney (p = 0,016); DMT2 increased COX 1 expression in liver (p = 0.001) and kidney (p = 0.009); DMT3 increased COX1 expression in liver (p = 0.037), kidney (p = 0.001) and skeletal muscle (p = 0.032).
4Discussion
Impairment in energy metabolism due to reduction in mitochondrial function was observed in T2DM and insulin resistance [19]. The disturbance of mitochondrial function leads to a reduction in oxidative capacity of mitochondria thus lowering the production of ATP [20]. This process is also followed by decreased COX1 expression in mitochondria [9, 10], which lead to the low level of ATP due to impairment in several enzymes important in phosphorylative oxidation activity [4, 20].
In the present study, we showed the potential effect of lessser yam (Dioscorea esculenta) in the regulation of energy metabolism, body weight and blood glucose in diabetic condition. Intervention of lesser yam can suppress appetite and halted body weight gain. Additionally, we showed that ATP production is increased significantly in diabetic group treated with low dose of lesser yam. COX 1 expression was also affected by the lesser yam intervention in kidney, liver and skeletal muscle.
Previous study has reported the beneficial effect of lesser yam in improving fasting plasma glucose of diabetic mice [21]. This effect was particularly caused by the presence of fiber, resistant starch and inulin in lesser yam [11, 22]. Inulin and other dietary fiber component in food have been reported to increase insulin sensitivity and improvement in glucose homeostasis in animal model with diabetes [23–26]. In addition, inulin and other dietary fibers can resist human enzyme dygestion and escape to the colon where it will be fermented by the colonic bacteria to produce short chain fatty acids (SCFAs) including acetate, butyrate, and propionate [27]. After being absorbed, those fatty acids are circulated in the blood and contacted with their receptors; free fatty acid 2 and 3 (FFAR2 and FFAR3), in the cells [28–30]. Activation of FFAR2 and FFAR3 in the targeted cells have been reported to induce several function such as anti-inflammation, regulation of appetite through the release of leptin secretion and regulation of symphatetic nervous system [30]. In addition, stimulation of both FFAR2 and FFAR3 by SCFAs in the small intestine have been linked to the elevated production of glucagon like peptide (GLP)-1 and PYY, which has been correlated with decreased appetite, elevation of insulin secretion and improvement of insulin sensitivity [30–35].
In addition, butyrate has gained attention as this small molecule can inhibit histone deacetylase (HDAc); an important enzyme for down regulating gene expression through histone modification [13]. It is previously demonstrated that inhibition of HDAc by butyrate can increase the expression of transcription coactivators that are important in mitochondria biogenesis such as PGC-1α [14]. Increasing PGC-1α expression is correlated with elevated fatty acid oxidation and ATP production [36, 37].
It is well established that diabetes is associated with decreased production of cellular ATP synthesis [3, 5]. Indeed, in this study, we observed marked decrease, approximately 70% ,of plasma or extracellular ATP level in diabetic rats compared to normal rats, which is followed by the reduction of COX-1 expression in liver, kidney, heart, and skeletal muscle. Although we didn’t measure the intracellular level of ATP, however, extracellular ATP level reflects the cellular production of ATP [38, 39]. Extracellular ATP is an extracellular adenine compound, which is released and transported out from most of the cells under normal or in response to stress or certain stimuli in the body [40–42]. Under basal condition, only 1% of intracellular ATP that would be released into extracellular compartment although there is a favorable gradient concentration (around 106 fold) to induce ATP efflux from the cells [38]. This concentration is sufficient to induce certain physiological effect through activation of purinoreceptor such as apoptosis [43], regulation of glucose uptake [44–46], cell survival [42],vasoconstriction [47] and vasodilation [48].
Extracellular ATP level in the blood is regulated by two factors; i.e., the ATP release from the cells and ATP degradation by the ectoATPase in the plasma membrane of the cells [49, 50]. Impairment in ATP release and ATP catabolism can cause low level of ATP level in extracellular compartment as previously demonstrated in diabetic rats condition [51]. Diet also has an important role in increasing extracellular ATP level. For instance, ketogenic diet (very low carbohydrate diet) has been reported to induce ATP synthesis in the cells and increase the ATP level in the extracellular compartment of the cells [52]. The ability of ketogenic diet in increasing extracellular ATP level was caused by the increase activity of mitochondria in generating ATP through ketone bodies oxidation [52–54]. In this study, we showed that low dose of lesser yam from the diet is able to increase extracellular ATP level whereas high dose of lesser yam has an inverse impact on extracellular ATP level. Interestingly, low dose of lesser yam slightly increase the COX-1 expression in several organ. Why low dose of lesser yam can increase extracellular ATP level despite modest increase in the COX-1 expression is currently unclear; however,we hypothesize that the high level of extracellular ATP in this group is independent to mitochondria function. Further study is needed to address the mechanism behind this issue.
In conclusion, we showed that lesser yam intervention in diabetic rats has ability to ameliorate fasting plasma glucose,increase extracellular ATP level and COX-1 expression. Further study is needed to evaluate the effect lesser yam on mitochondrial dysfunction in human.
Conflict of interest
The authors declare that there is no conflict of interest regarding the publication of this manuscript.
Acknowledgments
This research was supported by a grant from the Community Fund, Faculty of Medicine, Universitas Gadjah Mada.
References
1 | Abdul-Ghani MA , DeFronzo RA . Mitochondrial dysfunction, insulin resistance, and type 2 diabetes mellitus. Curr Diab Rep. (2008) ;8: :173–8. doi: 10.1007/s11892-008-0030-1. |
2 | Shen W , Hao J , Tian C , et al. A combination of nutriments improves mitochondrial biogenesis and function in skeletal muscle of type 2 diabetic goto-kakizaki rats. PLoS One. (2008) ;3: . doi: 10.1371/journal.pone.0002328. |
3 | Petersen KF , Dufour S , Shulman GI . Decreased insulin-stimulated ATP synthesis and phosphate transport in muscle of insulin-resistant offspring of type 2 diabetic parents. PLoS Med. (2005) ;2: :0879–84 . doi: 10.1371/journal.pmed.0020233. |
4 | Petersen KF , Dufour S , Befroy D , Garcia R , Shulman GI . Impaired mitochondrial activity in the insulin-resistant offspring of patients with type 2 diabetes. N Engl J Med. (2004) ;350: :664–71. doi: 10.1056/NEJMoa031314. |
5 | Szendroedi J , Schmid AI , Chmelik M , et al. Muscle mitochondrial ATP synthesis and glucose transport/phosphorylation in type 2 diabetes. PLoS Med. (2007) ;4: :0858–67. doi: 10.1371/journal.pmed.0040154. |
6 | Mogensen M , Sahlin K , Fernström M , et al. Mitochondrial respiration is decreased in skeletal muscle of patients with type 2 diabetes. Diabetes. (2007) ;56: :1592–9. doi: 10.2337/db06-0981. |
7 | Munusamy S , MacMillan-Crow LA . Mitochondrial superoxide plays a crucial role in the development of mitochondrial dysfunction during high glucose exposure in rat renal proximal tubular cells. Free Radic Biol Med. (2009) ;46: :1149–57. doi: 10.1016/j.freeradbiomed.2009.01.022. |
8 | Richter O-MH , Ludwig B . Cytochrome c oxidase–structure, function, and physiology of a redox-driven molecular machine. Rev Physiol Biochem Pharmacol. (2003) ;147: :47–74. doi: 10.1007/s10254-003-0006-0. |
9 | Akude E , Zherebitskaya E , Chowdhury SKR , Smith DR , Dobrowsky RT , Fernyhough P . Diminished superoxide generation is associated with respiratory chain dysfunction and changes in the mitochondrial proteome of sensory neurons from diabetic rats. Diabetes. (2011) ;60: 288–97. doi: 10.2337/db10-0818. |
10 | Morino K , Petersen KF , Dufour S , et al. Reduced mitochondrial density and increased IRS-1 serine phosphorylation in muscle of insulin-resistant offspring of type 2 diabetic parents. Journal of Clinical Investigation. (2005) ;115: (12):3587–93. doi: 10.1172/JCI25151. |
11 | Marsono . Perubahankadar resistant starch (RS) dan komposisi kimia beberapa bahan pangan kaya karbohidrat dalam pengolahan. Agritech. (1998) ;(3):124–7. |
12 | Topping DL , Clifton PM . Short-chain fatty acids and human colonic function: Roles of resistant starch and nonstarch polysaccharides. Physiol Rev. (2001) ;81: :1031–64. doi: 10.1002/(SICI)1096-8644(199706)103:2 <157::AID-AJPA2 >3.0.CO;2-R. |
13 | McKay JA , Mathers JC . Diet induced epigenetic changes and their implications for health. Acta Physiol (Oxf). (2011) ;202: :103–18. doi: 10.1111/j.1748-1716.2011.02278.x. |
14 | Gao Z , Yin J , Zhang J , Ward RE , Martin RJ , et al. Butyrate improves insulin sensitivity and increases energy expenditure in mice. Diabetes. (2009) ;58: (7):1509–17. |
15 | Lagouge M , Argmann C , Gerhart-Hines Z , et al. Resveratrol Improves Mitochondrial Function and Protects against Metabolic Disease by Activating SIRT1 and PGC-1α. Cell. (2006) ;127: :1109–22. doi: 10.1016/j.cell.2006.11.013. |
16 | Rabbani SI , Devi K , Khanam S . Protective role of glibenclamide against nicotinamide-streptozotocin induced nuclear damage in diabetic Wistar rats. J Pharmacol Pharmacother. (2010) ;1: :18–23. doi: 10.4103/0976-500X.64531. |
17 | Richana N , Sunarti TC . Karakterisasi sifat fisikokimia tepung umbi dan tepung pati dari umbi ganyong, suweg, ubikelapadangembili. J Pascapanen. (2004) ;1: (1):29–37. |
18 | Goñi I , García-Diz L , Mañas E , Saura-Calixto F . Analysis of resistant starch: A method for foods and food products. Food Chem. (1996) ;56: :445–9. doi: 10.1016/0308-8146(95)00222-7. |
19 | Kim J-A , Wei Y , Sowers JR . Role of mitochondrial dysfunction in insulin resistance. Circ Res. (2008) ;102: :401–14. doi: 10.1161/CIRCRESAHA.107.165472. |
20 | Ritov VB , Menshikova EV , He J , Ferrell RE , Goodpaster BH , Kelley DE . Deficiency of subsarcolemmal mitochondria in obesity and type 2 diabetes. Diabetes. (2005) ;54: :8–14. doi: 10.2337/diabetes.54.1.8. |
21 | Harijono , Estiasih T , Sunarharum WB , Hartono M . Hypoglycemic effect of biscuits containing water-soluble polysaccharides from wild yam (DioscoreahispidaDennts) or lesser yam (Dioscoreaesculenta) lessers and alginate. Int Food Res J. (2013) ;20: (3):2279–85. |
22 | Zubaidah E , Akhadiana W . comparative study of inulin extracts from dahlia, yam, and gembili lessers as prebiotic. Food and Nutrition Science. (2013) ;4: :8–12. doi: 10.4236/fns.2013.411A002. |
23 | Cani PD , Knauf C , Iglesias MA , Drucker DJ , Delzenne NM , Burcelin R . Improvement of glucose tolerance and hepatic insulin sensitivity by oligofructose requires a functional glucagon-like peptide 1 receptor. Diabetes. (2006) ;55: :1484–90. doi: 10.2337/db05-1360. |
24 | Busserolles J , Gueux E , Rock E , Demigné C , Mazur A , Rayssiguier Y . Oligofructose protects against the hypertriglyceridemic and pro-oxidative effects of a high fructose diet in rats. J Nutr. (2003) ;133: :1903–8. |
25 | Cani PD , Possemiers S , Van de Wiele T , et al. Changes in gut microbiota control inflammation in obese mice through a mechanism involving GLP-2-driven improvement of gut permeability. Gut. (1091) ;58: :1091–103. doi: 10.1136/gut.2008.165886. |
26 | Cani PD , Daubioul CA , Reusens B , Remacle C , Catillon G , Delzenne NM . Involvement of endogenous glucagon-like peptide-1(7-36) amide on glycaemia-lowering effect of oligofructose in streptozotocin-treated rats. J Endocrinol. (2005) ;185: :457–65. doi: 10.1677/joe.1.06100. |
27 | Lunn J , Buttriss J . Carbohydrates and dietary fibre. Br Nutr Found. (2007) ;32: :21–64. |
28 | Le Poul E , Loison C , Struyf S , et al. Functional characterization of human receptors for short chain fatty acids and their role in polymorphonuclear cell activation. J Biol Chem. (2003) ;278: :25481–9. doi: 10.1074/jbc.M301403200. |
29 | Brown AJ , Goldsworthy SM , Barnes AA , et al. The Orphan G protein-coupled receptors GPR41 and GPR43 are activated by propionate and other short chain carboxylic acids. J Biol Chem. (2003) ;278: :11312–9. doi: 10.1074/jbc.M211609200. |
30 | Layden BT , Angueira AR , Brodsky M , Durai V , Lowe WL . Short chain fatty acids and their receptors: New metabolic targets. Transl Res. (2013) ;161: :131–40. doi: 10.1016/j.trsl.2012.10.007. |
31 | Byrne CS , Chambers ES , Morrison DJ , Frost G . The role of short chain fatty acids in appetite regulation and energy homeostasis. Int J Obesity. (2015) ;39: (9):1331–8. doi: 10.1038/ijo.2015.84. |
32 | Tolhurst G , Heffron H , Lam YS , Parker HE , Habib AM , et al. Short-chain fatty acids stimulate glucagon-like peptide-1 secretion via the G-protein-coupled receptor FFAR2. Diabetes. (2012) ;61: (2):364–71. doi: 10.2337/db11-1019. |
33 | Kimura I , Ozawa K , Inoue D , Imamura T , Kimura K , et al. The gut microbiota suppresses insulin-mediated fat accumulation via the short-chain fatty acid receptor GPR43. Nature Comm. (2013) ;4: :1829. doi: 10.1038/ncomms2852. |
34 | Batterham RL , Cowley MA , Small CJ , Herzog H , Cohen MA , et al. Gut hormone PYY(3-36) physiologically inhibits food intake. Nature. (2002) ;418: :650–4. |
35 | Van den Hoek AM , Heijboer AC , Corssmit EPM , Voshol PJ , Romijn JA , et al. PYY3-36 reinforces insulin action on glucose disposal in mice fed high-fat diet. Diabetes. (2004) ;53: :1949–52. |
36 | Austin S , St-Pierre J . PGC1α and mitochondrial metabolism – emerging concepts and relevance in ageing and neurodegenerative disorders. J Cell Sci. (2012) ;125: (21):4963–71. |
37 | Holloway GP , Gurd BJ , Snook LA , Lally J , Bonen A . Compensatory increases in nuclear PGC1alpha protein are primarily associated with subsarcolemmal mitochondrial adaptations in ZDF rats. Diabetes. (2010) ;59: (4):819–28. doi: 10.2337/db09-1519. |
38 | Schwiebert EM , Zsembry A . Extracellular ATP as a signaling molecule for epithelial cells. Biochimia et Biophysica Acta. (2003) ;1615: :7–32. |
39 | Mempin R , Tran H , Chen C , Gong H , Ho KK , Lu S . Release of extracellular ATP during growth. BMC Microbiol. (2013) ;13: :301. doi: 10.1186/1471-2180-13-301. |
40 | Bodin P , Burnstock G . Purigenic signalling: ATP release. Neurochem Res. (2001) ;26: :959–69. |
41 | Sadananda P , Kao FLC , Liu L , Mansfield KJ , Burcher E . Acid and stretch, but not capsaicin, are effective stimuli for ATP release in the porcine bladder mucosa: Are ASIC and TRPV1 receptors involved? Eur J Pharmacol (2012) ;683: :252–9. |
42 | Ahmad S , Ahmad A , Ghosh M , Leslie CC , White CW . Extracellular ATP-mediated Signaling for Survival in Hyperoxia-induced Oxidative Stress. J Biol Chem. (2004) ;279: :16317–25. |
43 | Zheng LM , Zychlinsky A , Liu CC , Ojcius DM , Young JD . Extracellular ATP as a trigger for apoptosis or programmed cell death. J Cell Biol. (1991) ;112: (2):279–88. |
44 | Fischer Y , Becker C , Loken C . Purinergic inhibition of glucose transport in cardiomyocytes. J Biol Chem. (1999) ;274: (2):755–61. |
45 | Liu Y , CaoY , Zhan W , Bergmeier S , Qian Y , Akbar H , et al. A small-molecule inhibitor of glucose transporter 1 downregulates glycolysis, induces cell cycle arrest, and inhibits cancer cell growth in vitro and in vivo . Mol Cancer Ther. (2012) ;11: (8):1672–82. |
46 | Osorio-Fuentealba C , Contreras-Ferrat AE , Altamirano F , Espinosa A , Li Q , et al. Electrical stimuli release ATP to increase GLUT4 translocation and glucose uptake via PI3Kγ-Akt-AS160 in skeletal muscle cells. Diabetes. (2013) ;62: :1519–26. |
47 | Inscho EW , Mitchell KD , Navar LG . Extracellular ATP in the regulation of renal microvascular function. FASEB J. (1994) ;8: (3):319–28. |
48 | Liu C , Mather S , Huang Y , Garland CJ , Yao X . Extracellular ATP facilitates flow-induced vasodilatation in rat small mesenteric arteries. Am J Physiol Heart Circ Physiol. (2004) ;286: (5):H1688–95. |
49 | Alvarez CL , Schachter J , de Sá Pinheiro AA , Silva Lde S , Verstraeten SV , Persechini PM , Schwarzbaum PJ . Regulation of extracellular ATP in human erythrocytes infected with Plasmodium falciparum. PLoS One. (2014) ;9: (5):e96216. doi: 10.1371/journal.pone.0096216. |
50 | Zimmermann H . Extracellular metabolism of ATP and other nucleotides. Naunyn Schmiedebergs Arch Pharmacol. (2000) ;362: (4-5):299–309. |
51 | Duarte JMN , Oses JP , Rodrigues RJ , Cunha RA . Modification of purigenic signaling in the hipocampus of streptozotocin-induced diabetic rats. Neuroscience. (2007) ;149: :382–91. |
52 | Masino SA , Kawamura M Jr , Wasser CD , Pomeroy LT , Ruskin DN . Adenosine, Ketogenic Diet and Epilepsy: The Emerging Therapeutic Relationship Between Metabolism and Brain Activity. Curr Neuropharmacol. (2009) ;7: (3):257–68. |
53 | Danial NN , Hartman AL , Stafstrom CE , Thio LL . How does the ketogenic diet work? four potential mechanisms. J Child Neurol. (2013) ;28: (8):1027–33. |
54 | Masino SA , Geiger JD . Are purines mediators of the anticonvulsant/neuroprotective effects of ketogenic diets? Trends Neurosci (2008) ;31: (6):273–8. |
Figures and Tables
Fig.1
Administration oflesser yam (Dioscorea esculenta) in the diet prevent body weight gain (a), decrease food intake (b), reduce fasting serum glucose (c) and increase plasma ATP level (d) of diabetic rats.
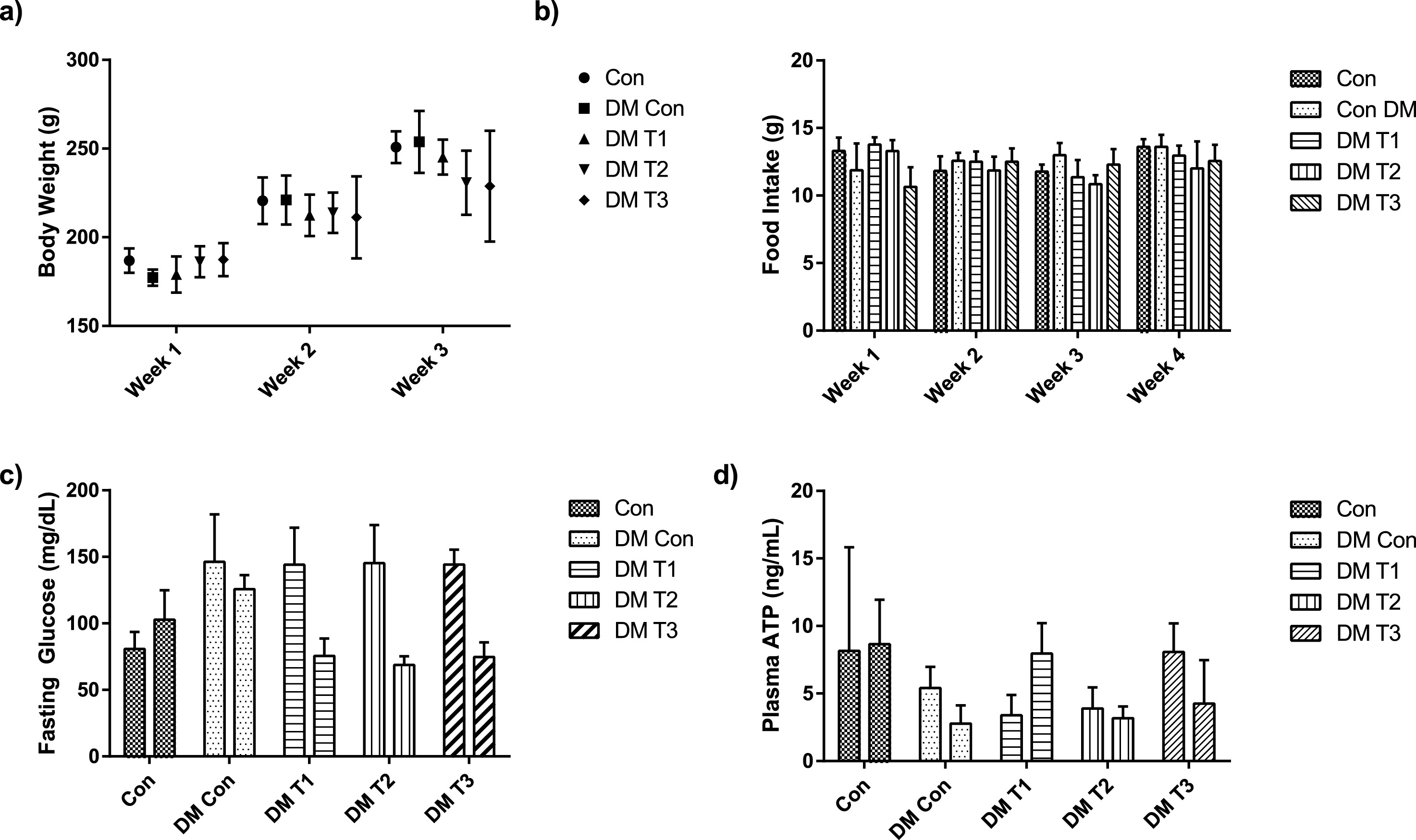
Fig.2
Protein expression of COX-1 in various organs (liver, heart, brown fat, skeletal muscle and kidney) after interventions.
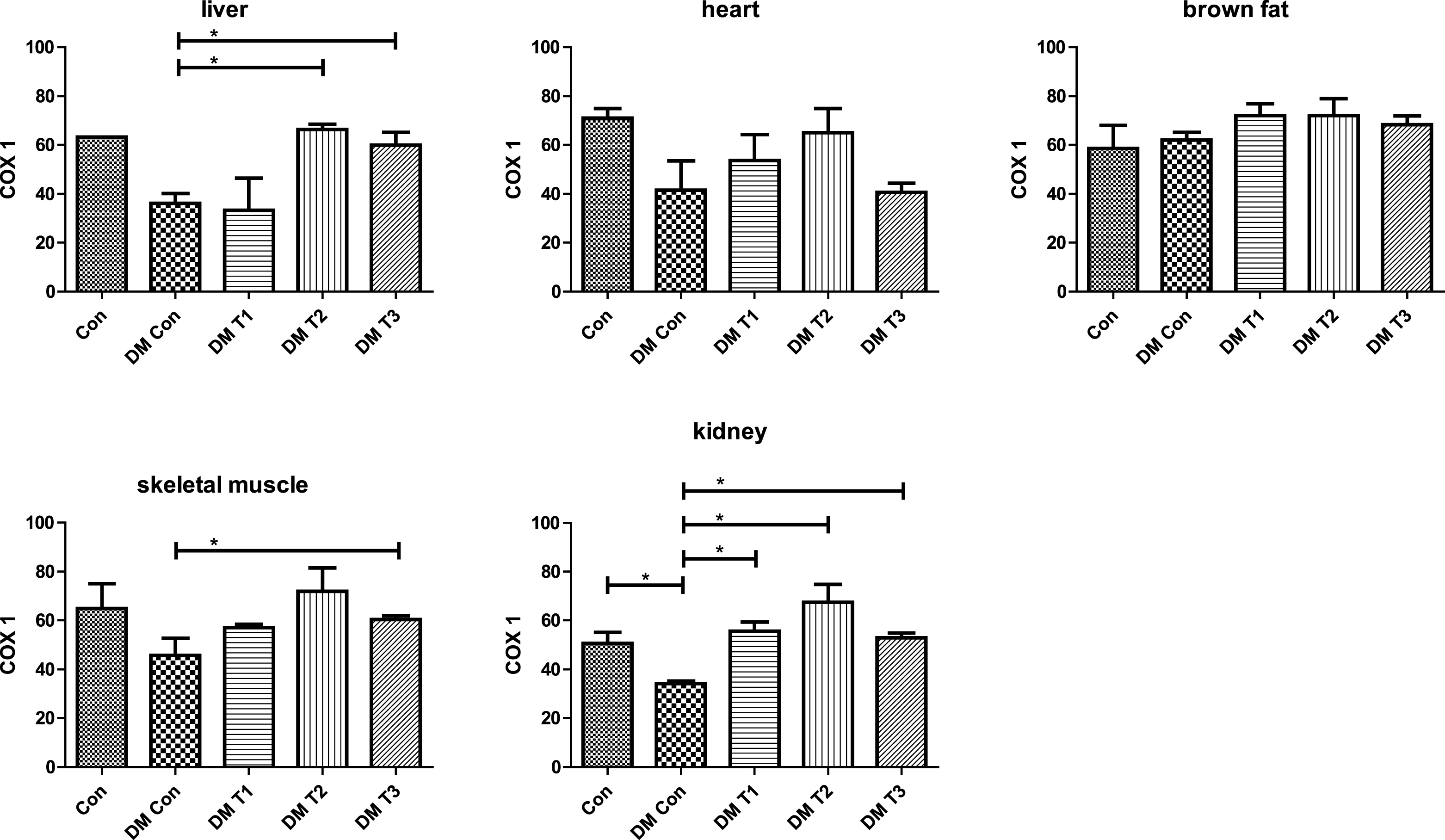
Table 1
Animal diet
Composition | Semi | Lesser yam | Lesser yam | Lesser yam |
Purified Diet | flour 200 mg/kg | flour | flour 800 mg/kg | |
body weight | 400 mg/kg | body weight | ||
(DM T1) | body weight | (DM T3) | ||
(DM T2) | ||||
Casein | 24% | 24% | 24% | 24% |
DL-Metionine | 0.30% | 0.3% | 0.3% | 0.3% |
Cornstarch | 61% | 52.67% | 44.33% | 38% |
Vitamin Mix (AIN 93) | 1% | 1% | 1% | 1% |
Mineral Mix (AIN 93) | 3.5% | 3.5% | 3.5% | 3.5% |
Choline Chloride | 0.20% | 0.2% | 0.2% | 0.2% |
Agar-agar | 5% | 5% | 5% | 5% |
Corn oil | 5% | 5% | 5% | 5% |
Lesser yam | 0% | 9.33% | 16.67% | 23% |