Quantitative ultrasound of submental and masticatory muscles in children with cerebral palsy
Abstract
PURPOSE:
Quantitative muscle ultrasound (QMUS) is potentially valuable as a diagnostic tool in central neurological disorders, as it provides information about changes in muscle architecture. This study aimed to investigate whether ultrasound images of the submental and masticatory muscles in children with spastic cerebral palsy (CP) differ from those obtained in a reference group, and whether observed ultrasound abnormalities differ between subgroups of children with different Eating and Drinking Ability Classification System (EDACS) levels to support its construct validity.
METHODS:
A prospective cohort study was conducted in 25 children with spastic CP aged 3-18 years. QMUS of selected muscles was performed. Muscle thickness and echogenicity in the CP group were compared to previously collected reference values, and between different EDACS levels within the CP group.
RESULTS:
Median echogenicity of all muscles was significantly higher in children with CP than in healthy controls. The temporalis muscle was significantly thinner in the CP group. There were no differences in muscle thickness or echogenicity between EDACS levels.
CONCLUSION:
QMUS is able to detect abnormal architecture of submental and masticatory muscles in children with spastic CP, but the interpretation of abnormalities in relation to the severity of mastication and swallowing problems needs further investigation.
Abbreviations
CP | Cerebral Palsy |
EDACS | Eating and Drinking Ability Classification System |
GMFCS | Gross Motor Function Classification System |
QMUS | Quantitative Muscle Ultrasound |
1Introduction
Cerebral palsy (CP) is a neurodevelopmental condition of early childhood with lifelong consequences. CP often leads to limitations in daily activities and participation because of disturbances of posture, movement, sensation, perception, cognition, communication, and/or behavior [1]. The prevalence of CP is approximately 2 : 1000 live births, of which spastic CP is the most common subtype [2, 3].
Disturbed movement and sensation in children with CP can lead to limited oral motor skills that negatively affect speech, eating, drinking, swallowing, and salivary control. As a consequence, dysphagia is reported in 21-99% of children with CP [4, 5]. Both the volitional oral phase of food intake (including mastication) and the reflexive pharyngeal phase of swallowing may be disturbed, depending on the affected brain regions [6]. Dysphagia can have profound consequences resulting in a reduced quality of life: inadequate food intake, a failure to thrive, aspiration, the need for tube feeding [7]. To classify the daily feeding and swallowing abilities of children with CP, the Eating and Drinking Ability Classification System (EDACS) has been developed [8]. Previous research has shown that the severity of dysphagia in children with CP is related to gross motor function as assessed with the Gross Motor Function Classification System (GMFCS) [9]. Generally, the most common way to identify feeding and swallowing problems in children with CP is a routine clinical assessment.
In children with neuromuscular disorders, it was shown that ultrasound of submental and masticatory muscles involved in feeding and swallowing has an added value in the diagnostic process of mastication and swallowing difficulties [10, 11]. Quantitative muscle ultrasound (QMUS) provides measures of muscle echogenicity (determined by the degree to which sound is reflected back to the transducer) and muscle thickness [11]. Muscle ultrasound is a quick, non-invasive, point-of-care technique that could augment the clinical speech-language therapist examination by providing information about the structural integrity of muscles involved in the oral phase of feeding [11]. Previous studies using QMUS have shown that the structure of skeletal muscles in patients with central neurological disorders may be changed [12] and that these changes may involve both paretic and nonparetic muscles [13]. In various age groups of patients with spastic CP, several imaging studies have shown that the thickness of the upper and lower limb muscles significantly differed from reference values obtained in a healthy population [14–17]. Another study found that the amount of intramuscular fat of lower limb muscles was abnormally high in patients with spastic CP, dependent on GMFCS level [18]. Yet, the thickness and structure of submental and masticatory muscles have not previously been assessed with ultrasound in patients with CP. If the thickness and echogenicity of submental and masticatory muscles in children with CP are abnormal, this would confirm structural muscle changes that could help describe some of the mechanisms underlying mastication and swallowing difficulties in these children.
Therefore, the aim of this study was to assess whether the thickness and echogenicity of submental and masticatory muscles in a cohort of children with spastic CP differed from previously collected reference values of typically-developing children [19, 20]. The secondary aim was to investigate whether observed ultrasound abnormalities were different for subgroups of children with different EDACS levels to support its construct validity.
2Methods
2.1Participants
Children from the age of three to 18 years diagnosed with spastic CP (or a mixed type of CP in which spasticity was clinically the dominant feature) were included in this prospective clinical cohort study. All visited a speech-language therapist at the interdisciplinary pediatric outpatient clinic for swallowing and salivary control symptoms between April 2018 and June 2021. The study was approved by the regional medical ethics committee. Written informed consent was given by all parents and, in the case of participants 12 years or older, assent was also provided.
2.2Procedure
The usual assessment for children with CP was performed during their visit to the outpatient clinic and could vary depending on the nature of the problem voiced by patients and parents. Age, height, weight, GMFCS level, and the pattern of spastic CP (unilateral, bilateral) were noted. The clinical assessment included classifying the individual child’s EDACS level with input from the parents. The EDACS consists of five levels: level I, the patient eats and drinks safely and efficiently; level II, eats and drinks safely but with some limitations to efficiency; level III, eats and drinks with some limitations to safety; level IV, eats and drinks with significant limitations to safety; level V, the patient is unable to eat or drink [8, 21]. Furthermore, in all children, bilateral QMUS of selected submental and masticatory muscles was added to the standard assessment.
2.3QMUS exams
All ultrasound examinations were performed using the Z.One Ultra Convertible ultrasound system (Zonare Medical Systems, Mountain View, California, USA) with a broadband linear 10–5 MHz transducer. Ultrasound settings were standardized as described previously [19, 20]. A generous amount of contact gel was applied to the probe, and the probe was placed on the skin of the participant according to a predefined protocol [19, 20]. Figure 1 shows the probe placement per muscle. Standardized muscle ultrasound images were obtained from the bilateral submental and masticatory muscles to assess the following variables: echogenicity and thickness of the digastric muscles, masseter muscles and temporalis muscles; the echogenicity of the geniohyoid muscles; and thickness of the tongue. The intrinsic tongue muscles themselves (which would have required the probe to be put inside the oral cavity) [11] were not measured in order to avoid a pathological bite reflex. Measurements were performed while the participants were sitting in a regular chair or in their wheelchair. Three images were taken from every muscle and stored as DICOM files. The mean echogenicity of selected regions of interest was calculated using the grayscale histogram function of a custom software program for quantitative muscle image analysis, resulting in a mean grayscale level ranging from zero (black) to 255 (white) [22]. Muscle thickness was measured by placing electronic calipers in the ultrasound images within the fascial outline of the muscle at the level of the maximum muscle bulk, following a standardized protocol [19, 20, 23]. All ultrasound images were checked for technical errors (i.e., sub-optimal scan angle). Ultrasound images showing fatty degeneration of a muscle were noted, as this lowers muscle echogenicity, leading to “false negative” outcomes.
Fig. 1
Probe placement and ultrasound image per muscle.
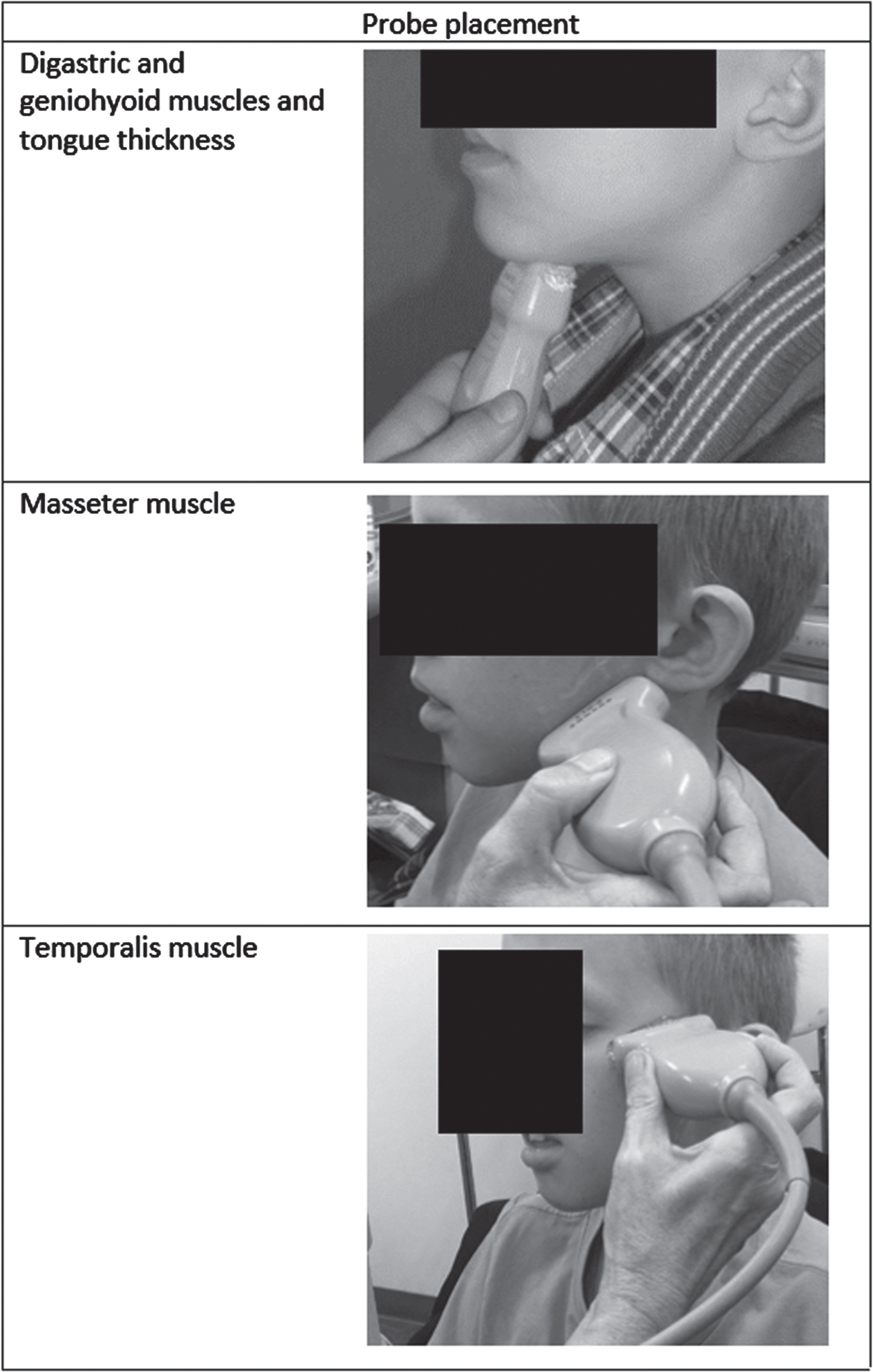
2.4Statistical analysis
The average grayscale level and diameter obtained from the three measurements per muscle was taken for analysis. Z-scores (i.e., the number of standard deviations from the mean) for the average echogenicity and thickness were calculated for all individual muscles. Reference values for healthy subjects and the equations to correct for height, weight, and age to calculate z-scores were previously reported [19, 20, 23]. Because of the small sample size, non-parametric statistical tests were performed. Z-scores were considered abnormal for echogenicity if they exceeded 2.0. For muscle thickness, z-scores were considered abnormal if they were lower than -2.0 or exceeded 2.0. Wilcoxon signed rank tests for paired data were performed to determine whether the median z-scores differed between the left and right body side.
To test whether the median z-scores of the participants differed from reference values, the Wilcoxon signed rank test (with hypothesized median value
3Results
3.1Participants
Twenty-five children with spastic CP were included. Patient characteristics are presented in Table 1.
Table 1
Participant characteristics (n = 25)
Age (yrs), median (IQR) | 9.3 (5.9) |
Sex (n) | |
Male | 14 |
Female | 11 |
Height (cm), median (IQR) | 135.0 (31.5) |
Weight (kg), median (IQR) | 26.0 (27.0) |
Clinical pattern (n) | |
Bilateral | 23 |
Unilateral (right side) | 2 |
GMFCS level (n) | |
I | 5 |
II | 3 |
III | 5 |
IV | 6 |
V | 6 |
EDACS level (n) | |
I | 3 |
II | 5 |
III | 8 |
IV | 6 |
V | 3 |
Yrs, Years; IQR, Interquartile range; GMFCS, Gross Motor Function Classification System; Level I: The child can walk at home, school, outdoors, and in the community and can climb stairs without the use of a railing; Level II: The child can walk in most settings and climb stairs holding onto a railing; Level III: The child can walk using a hand-held mobility device in most indoor settings and may climb stairs holding onto a railing with supervision or assistance; Level IV: The child uses methods of mobility that require physical assistance or powered mobility in most settings; Level V: The child is transported in a manual wheelchair in all settings. EDACS, Eating and Drinking Ability Classification System; Level I: Eats and drinks safely and efficiently; Level II: Eats and drinks safely but with some limitations to efficiency; Level III: Eats and drinks with some limitations to safety and there may be limitations to efficiency; Level IV: Eats and drinks with significant limitations to safety; Level V: Unable to eat or drink safely – tube feeding may be considered to provide nutrition.
3.2Muscle thickness
There was no significant difference in thickness between the left and right side for any muscle (Table 2). The temporalis showed either a normal or decreased thickness (Table 2) and was bilaterally significantly thinner in these participants as a group compared to reference values (Table 3). The digastric, masseter, and the tongue muscles showed a decreased, normal, and increased thickness. In the two participants with unilateral CP (participants 1 and 15), differences were found in the thickness of the masseter and the temporalis. In the total group, seven children showed normal thickness of all muscles. All other children showed abnormal thickness of one or more muscles.
Table 2
Muscle thickness and echogenicity per participant and per muscle
EDACS | Participant | Digastric | Digastric | Geniohyoid | Masseter | Masseter | Temporalis | Temporalis | Tongue | ||||||
left | right | left | right | left | right | ||||||||||
MT | EG | MT | EG | EG | MT | EG | MT | EG | MT | EG | MT | EG | MT | ||
1 | 1 | + | + | + | + | + | + | + | > | + | < | - | + | - | + |
9 | + | + | + | + | - | > | + | + | + | + | + | + | + | + | |
21 | < | - | < | - | - | + | - | + | + | < | - | < | - | + | |
2 | 2 | + | + | + | + | + | + | - | > | + | < | - | + | + | + |
16 | + | + | > | + | + | + | + | + | + | + | + | + | + | > | |
20 | > | + | + | + | + | + | + | + | + | + | + | + | + | + | |
22 | + | + | + | + | + | + | + | + | - | + | + | + | + | + | |
24 | + | + | + | - | - | + | - | + | - | + | - | + | - | < | |
3 | 3 | + | - | + | - | + | + | - | + | - | + | + | + | + | + |
4 | < | + | < | - | - | < | - | + | - | < | - | + | - | + | |
8 | + | + | + | + | - | < | - | < | - | + | - | + | - | + | |
10 | + | - | + | - | + | < | - | < | - | < | - | < | + | < | |
12 | + | + | + | + | + | + | + | + | + | < | - | < | - | na | |
13 | + | - | + | - | - | + | + | > | + | + | - | + | - | + | |
15 | + | - | + | - | + | + | - | + | - | + | + | + | - | + | |
17 | + | - | + | + | - | + | + | + | + | < | - | < | + | + | |
4 | 6 | + | + | + | + | + | + | + | + | + | + | + | + | + | na |
7 | + | + | + | + | + | + | + | < | - | + | + | + | + | + | |
11 | > | - | > | - | - | + | + | + | - | + | + | + | + | + | |
14 | + | + | + | + | - | + | + | + | + | + | + | + | + | + | |
18 | + | - | + | - | + | + | - | + | + | + | - | + | - | + | |
19 | + | - | + | - | + | + | + | + | + | + | - | + | + | + | |
5 | 5 | + | - | + | - | - | + | - | + | + | < | - | + | + | > |
23 | + | - | + | - | - | + | + | + | + | + | + | + | + | + | |
25 | > | + | > | + | - | > | + | > | + | + | - | + | + | < | |
Number of participants with abnormal muscle (% ) | 5 (20) | 11 (44) | 5 (20) | 12 (48) | 12 (48) | 5 (20) | 10 (40) | 7 (28) | 9 (36) | 8 (32) | 14 (56) | 4 (16) | 9 (36) | 5 (20) |
Shaded cells indicate abnormal muscle thickness or echogenicity. EDACS: Eating and Drinking Ability Classification System; MT: muscle thickness; EG: echogenicity; +: normal muscle thickness or echogenicity (based on z-scores); -: abnormal echogenicity; <: z-score for muscle thickness < -2; >: z-score for muscle thickness > 2; na: not applicable.
Table 3
Median muscle thickness and echogenicity z-scores with statistical comparison to healthy controls
Side | Median (IQR) | p-value* | |
Muscle thickness | |||
Digastric | Left | 0.00 (2.35) | 0.58 |
Right | 0.10 (2.40) | 0.60 | |
Masseter | Left | –0.65 (1.60) | 0.13 |
Right | –0.46 (2.42) | 0.70 | |
Temporalis | Left | –0.60 (2.10) | <.01 |
Right | –1.00 (1.90) | <.01 | |
Tongue | – | 0.0 (2.36) | 0.87 |
Echogenicity | |||
Digastric | Left | 1.90 (2.17) | <.01 |
Right | 2.29 (3.20) | <.01 | |
Geniohyoid | – | 2.30 (3.11) | <.01 |
Masseter | Left | 1.34 (2.80) | <.01 |
Right | 1.78 (2.74) | <.01 | |
Temporalis | Left | 2.30 (2.30) | <.01 |
Right | 1.40 (1.40) | <.01 |
IQR: Interquartile range. *test-value
3.3Echogenicity
Only two children showed normal echogenicity of all muscles (Table 2). All others showed increased echogenicity of at least one muscle. Only for the temporalis was there a significant side-to-side difference within the CP group (Z = -2.57, p = .01), in which the echogenicity of the left temporalis muscle was higher. In one of the participants with a diagnosis of unilateral CP, a side-to-side difference in echogenicity of the temporalis muscle was found in which the echogenicity was abnormal on the paretic side. The median echogenicity of all muscles in the CP group was significantly increased compared to healthy controls (Table 3).
3.4Muscle thickness and echogenicity for different EDACS levels
There were no significant differences in muscle thickness or echogenicity between the different EDACS levels, except for the geniohyoid (H(4) = 11.21, p = .02) (Figs. 2 and 3). The Mann Whitney U test for the geniohyoid showed a significant difference between EDACS levels 2 and 5 (U = 0.00, p = .04), but not between the other, sequential levels.
Fig. 2
Boxplots of z-scores for thickness of submental and masticatory muscles per Eating and Drinking Ability Classification System (EDACS) level.
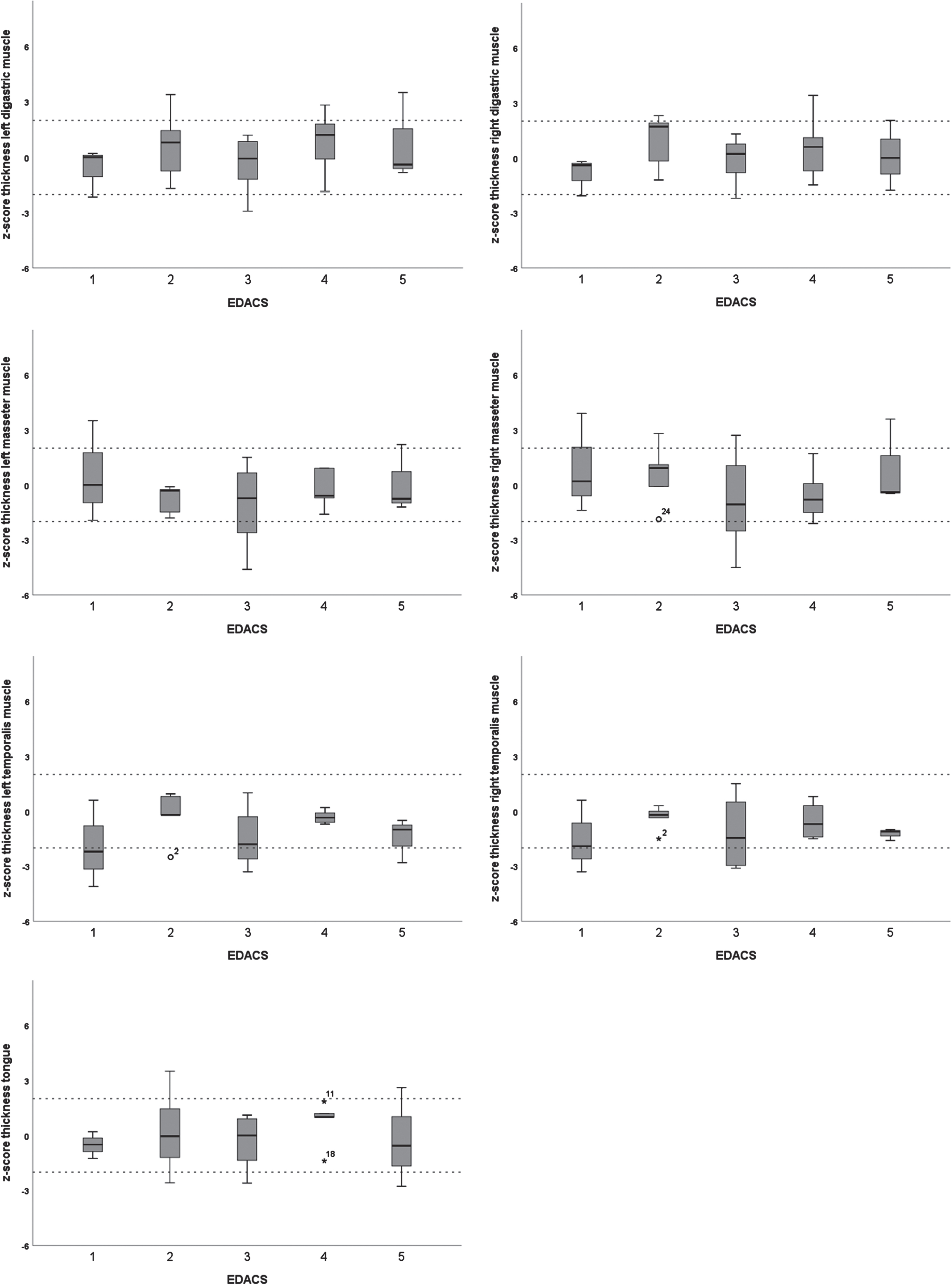
Fig.3
Boxplots of z-scores for echogenicity of submental and masticatory muscles per Eating and Drinking Ability Classification System (EDACS) level.
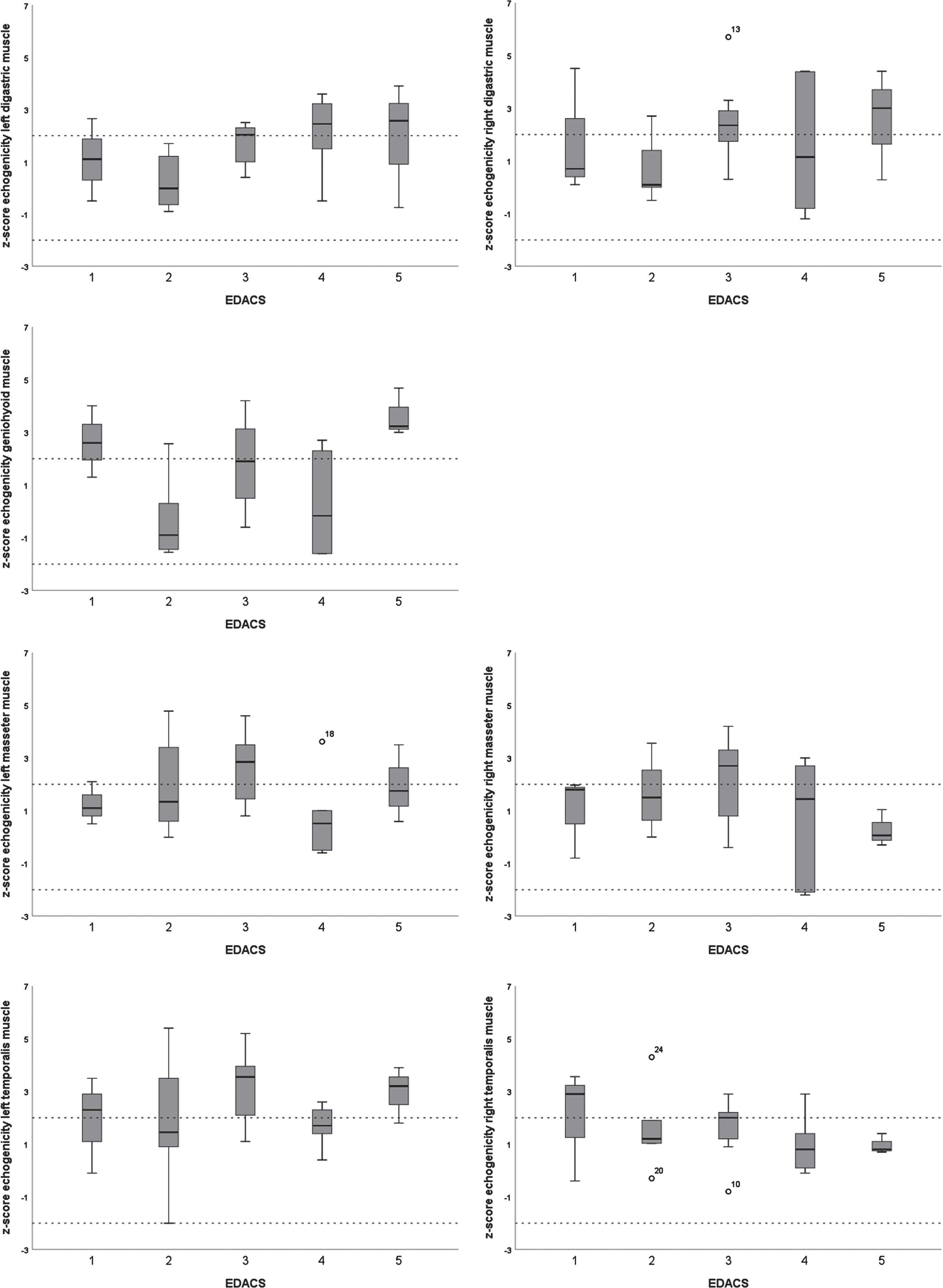
4Discussion
The results showed increased echogenicity of the submental and masticatory muscles in children with spastic CP, strengthening the idea that muscle ultrasound can measure structural abnormalities of muscles necessary for chewing and swallowing in these children. In contrast, muscle thickness as assessed with QMUS was less informative, as only the temporalis was significantly thinner in CP patients than in healthy subjects. The thickness of the masseter was not significantly different from the reference group. Previous research has shown that the muscle activity of the temporalis is more influenced by food texture than that of the masseter [24]. Since the participants in this study functioned to a large extent at EDACS levels where food is modified, the thinner temporalis in this CP group could be a consequence of disuse.
There was no relation found between muscle thickness or echogenicity and EDACS levels, which does not corroborate the initial expectation that more severely affected children would show more severe abnormalities in their muscle architecture. The most likely explanation for this unexpected result is that QMUS measures specific aspects of isolated muscles and the EDACS provides an overall functional score for the daily safety and efficiency of eating and drinking. The observed lack of relationship may imply that QMUS adds distinct information that complements such an overall assessment, which is underscored by the finding that muscles can show increased echogenicity in patients with EDACS level 1. This notion corresponds to clinical findings from an earlier study in children with CP, showing that those with EDACS level 1 had a significantly lower dysphagia limit than their peers and, thus, did not have the same capacities related to eating and drinking as healthy children [25]. Combining these findings suggests that QMUS could be useful to assess even children with CP at EDACS level 1, who might need closer monitoring when their muscles show increased echogenicity.
Although the primary lesion causing the movement disorder in children with CP is located in the central nervous system, previous research has shown that skeletal muscles in people with central nervous system disorders may show abnormal echogenicity [13, 26]. As in previous studies, the increased muscle echogenicity found in the current study most likely can be explained by secondary motor neuron denervation due to pyramidal tract damage, fitting the notion of transsynaptic degeneration in central nervous system disorders [27, 28].
This study showed that there is potentially a wide variation in echogenicity of submental and masticatory muscles amongst individuals with CP. This raises the question whether training (for example, to improve masticatory performance) would be beneficial, particularly in those children with relatively preserved muscle architecture. In the future, insight about the structure of submental and masticatory muscles of children with CP may provide direction for whether therapy should consist of performance training or focus on compensatory strategies.
A limitation of this study is that a relatively small group of patients was included, so the results should be interpreted with caution. Future studies should focus on bilateral ultrasound measurements of submental and masticatory muscles in a larger group of participants to get a better sense of the relationship between oral muscle function and muscle architecture. For instance, it might be interesting to investigate the correlation between the structure of masticatory muscles and chewing performance tests or between submental muscles and swallowing capacity tests, such as the test of mastication and swallowing of solids or the dysphagia limit [29, 30]. In addition, it would be of interest to study the differences between the paretic and non-paretic side in patients with unilateral CP to better understand underlying (neural and non-neural) mechanisms of altered muscle architecture. Future research should also collect longitudinal ultrasound muscle data in children with CP to gain insight into adaptation processes of the muscles affected by spastic CP.
So far, muscle ultrasound is not yet widely used as part of the clinical assessment of speech-language therapists. With this study, it was shown that QMUS is suitable to measure the (affected) structure of submental and masticatory muscles in children with CP. Orofacial muscles in children with CP are affected, but the quality is independent of EDACS level.
Acknowledgments
The authors have no acknowledgements.
Conflict of interest
Nens van Alfen worked as an ultrasound instructor for SonoSkills and performs editorial services for Wiley Publishing; all payments go to their employer. The other authors have no conflict of interest to report.
Funding
This research was financially supported by the “Phelps Stichting voor spastici” (2018006).
References
[1] | Rosenbaum P , Paneth N , Leviton A , et al. A report: the definition and classification of cerebral palsy April 2006. Dev Med Child Neurol Suppl. (2007) ;109: (suppl 109):8–14. |
[2] | Reid SM , Carlin JB , Reddihough DS . Distribution of motor types in cerebral palsy: how do registry data compare? Dev Med Child Neurol. (2011) ;53: (3):233–8. |
[3] | Oskoui M , Coutinho F , Dykeman J , Jetté N , Pringsheim T . An update on the prevalence of cerebral palsy: a systematic review and meta-analysis. Dev Med Child Neurol. (2013) ;55: (6):509–19. |
[4] | Calis EA , Veugelers R , Sheppard JJ , Tibboel D , Evenhuis HM , Penning C . Dysphagia in children with severe generalized cerebral palsy and intellectual disability. Dev Med Child Neurol. (2008) ;50: (8):625–30. |
[5] | Parkes J , Hill N , Platt MJ , Donnelly C . Oromotor dysfunction and communication impairments in children with cerebral palsy: a register study. Dev Med Child Neurol. (2010) ;52: (12):1113–9. |
[6] | Erasmus CE , van Hulst K , Rotteveel JJ , Willemsen MA , Jongerius PH . Clinical practice: swallowing problems in cerebral palsy. Eur J Pediatr. (2012) ;171: (3):409–14. 10.1007/s00431-011-1570-y |
[7] | Weir KA , McMahon S , Taylor S , Chang AB . Oropharyngeal aspiration and silent aspiration in children. Chest. (2011) ;140: (3):589–97. |
[8] | Van Hulst K , Snik DA , Jongerius PH , Sellers D , Erasmus CE , Geurts AC . Reliability, construct validity and usability of the Eating and Drinking Ability Classification System (EDACS) among Dutch children with Cerebral Palsy. J Pediatr Rehabil Med. (2018) ;11: (2):115-–24. 10.3233-PRM-170515 |
[9] | Kim J-S , Han Z-A , Song DH , Oh H-M , Chung ME . Characteristics of dysphagia in children with cerebral palsy, related to gross motor function. Am J Phys Med Rehabil. (2013) ;92: (10):912–9. 10.1097/PHM.0b013e318296dd99 |
[10] | Pillen S , Tak RO , Zwarts MJ , et al. Skeletal Muscle Ultrasound: Correlation Between Fibrous Tissue and Echo Intensity. Ultrasound Med Biol. (2009) ;35: (3):443–6. 10.1016/j.ultrasmedbio.2008.09.016 |
[11] | Van den Engel-Hoek L , Lagarde M , Van Alfen N . Ultrasound of oral and masticatory muscles: Why every neuromuscular swallow team should have an ultrasound machine. Clin Anat. (2017) ;30: (2):183–93. 10.1002/ca.22818 |
[12] | Boon AJ , Wijntjes J , O’Brien TG , Sorenson EJ , Cazares Gonzalez ML , van Alfen N . Diagnostic accuracy of gray scale muscle ultrasound screening for pediatric neuromuscular disease. Muscle Nerve. (2021) ;64: (1):50–58. 10.1002/mus.27211 |
[13] | Berenpas F , Martens A-M , Weerdesteyn V , Geurts AC , van Alfen N . Bilateral changes in muscle architecture of physically active people with chronic stroke: a quantitative muscle ultrasound study. Clin Neurophysiol. (2017) ;128: (1):115–22. 10.1016/j.clinph.2016.10.096 |
[14] | Ohata K , Tsuboyama T , Ichihashi N , Minami S . Measurement of muscle thickness as quantitative muscle evaluation for adults with severe cerebral palsy. Phys Ther. (2006) ;86: (9):1231–9. 10.2522/ptj.20050189 |
[15] | Williams SA , Stott NS , Valentine J , Elliott C , Reid SL . Measuring skeletal muscle morphology and architecture with imaging modalities in children with cerebral palsy: a scoping review. Dev Med Child Neurol. (2021) ;63: (3):263–73. 10.1111/dmcn.14714 |
[16] | Barrett RS , Lichtwark GA . Gross muscle morphology and structure in spastic cerebral palsy: a systematic review. Dev Med Child Neurol. (2010) ;52: (9):794–804. 10.1111/j.1469-8749.2010.03686.x |
[17] | Von Walden F , Jalaleddini K , Evertsson B , Friberg J , Valero-Cuevas FJ , Pontén E . Forearm flexor muscles in children with cerebral palsy are weak, thin and stiff. Front Comput Neurosci. (2017) ;11: :30. 10.3389/fncom.2017.00030 |
[18] | Noble JJ , Charles-Edwards GD , Keevil SF , Lewis AP , Gough M , Shortland AP . Intramuscular fat in ambulant young adults with bilateral spastic cerebral palsy. BMC Musculoskelet Disord. (2014) ;15: (1):1–8. 10.1186/1471-2474-15-236 |
[19] | Lagarde M , van den Engel-Hoek L . Quantitative Ultrasound of Orofacial Muscles in Infants from 6 Months to 5 Years: Collecting Normal Values. Curr Med Im Rev. (2017) ;13: (3):332–8. |
[20] | Van den Engel-Hoek L , van Alfen N , de Swart BJ , de Groot IJ , Pillen S . Quantitative ultrasound of the tongue and submental muscles in children and young adults. Muscle Nerve. (2012) ;46: (1):31–7. |
[21] | Sellers D , Mandy A , Pennington L , Hankins M , Morris C . Development and reliability of a system to classify the eating and drinking ability of people with cerebral palsy. Dev Med Child Neurol. (2014) ;56: (3):245–51. |
[22] | Pillen S , van Keimpema M , Nievelstein RA , Verrips A , van Kruijsbergen-Raijmann W , Zwarts MJ . Skeletal muscle ultrasonography: visual versus quantitative evaluation. Ultrasound Med Biol. (2006) ;32: (9):1315–21. 10.1016/j.ultrasmedbio.2006.05.028 |
[23] | Van den Engel-Hoek L , de Groot IJ , Sie LT , et al. Dystrophic changes in masticatory muscles related chewing problems and malocclusions in Duchenne muscular dystrophy. Neuromuscul Disord. (2016) ;26: (6):354–60. |
[24] | Mioche L , Bourdiol P , Martin J-F , Noël Y . Variations in human masseter and temporalis muscle activity related to food texture during free and side-imposed mastication. Arch Oral Biol. (1999) ;44: (12):1005–12. 10.1016/s0003-9969(99)00103-x |
[25] | Schepers FV , van Hulst K , Spek B , Erasmus CE , van den Engel-Hoek L . Dysphagia limit in children with cerebral palsy aged 4 to 12 years. Dev Med Child Neurol. (2022) ;64: (2):253–258. 10.111/dmcn.15031 |
[26] | Lieber RL , Steinman S , Barash IA , Chambers H . Structural and functional changes in spastic skeletal muscle. Muscle Nerve. (2004) ;29: (5):615–27. |
[27] | Lukács M . Electrophysiological signs of changes in motor units after ischaemic stroke. Clin Neurophysiol. (2005) ;116: (7):1566–70. 10.1016/j.clinph.2005.04.005 |
[28] | Gunreben G , Bogdahn U . Real-time sonography of acute and chronic muscle denervation. Muscle Nerve. (1991) ;14: (7):654–64. |
[29] | Ertekin C , Aydoğdu I , Yüceyar N . Piecemeal deglutition and dysphagia limit in normal subjects and in patients with swallowing disorders. J Neurol Neurosurg Psychiatry. (1996) ;61: (5):491–6. |
[30] | Frank U , van den Engel-Hoek L , Nogueira D , Schindler A , Adams S , Curry M , Huckabee M-L . International standardisation of the test of masticating and swallowing solids in children. J Oral Rehabil. (2019) ;46: (2):161–169. 10.1111/joor.12728 |