Motor function outcomes in children with open prenatal repair of Spina Bifida Aperta at 36-month follow-up: The Zurich cohort
Abstract
PURPOSE:
This study aimed to describe outcomes of motor function with a special focus on ambulation ability at 36 months among children with open prenatal repair of spina bifida aperta (SB).
METHODS:
A prospective cohort study was conducted including 87 patients with open prenatal repair of SB at the investigating center born between 2010 and 2018. Anatomic lesion level and motor function level in the neonatal period, as well as motor function level, ambulation status, and use of orthotics and assistive devices at 36 months were assessed.
RESULTS:
At 36 months, ambulation was assessed in 86 children; of those, 86% (n = 74) were ambulating. Independent of ambulation, orthotics were worn in 81.6% (71/87) and assistive devices in 47.1% (41/87). Children with a lower lumbar or sacral motor function level were the first to reach independent ambulation and were more likely to ambulate at 36 months than children with higher motor function levels (p = < .001). The anatomic lesion level determined on the neonatal MRI correlated with ambulation status at 36 months (p = < 0.001).
CONCLUSION:
At 36 months, most children with open prenatal repair for SB showed favourable ambulation status. However, most still used assistive devices or orthotics. Anatomic lesion level on neonatal MRI, motor function level during the neonatal period, and motor function level at 36 months were associated with ambulation status at 36 months.
What this paper adds
• Maintaining standardized, long-term multidisciplinary care and collecting and reporting outcome data for prenatal spina bifida repair can be achieved in a non-trial setting outside of the United States.
• Ambulation status at 36 months in a non-trial setting is comparable to the benchmark MOMS (Management of Myelomeningocele Study) and MOMS2 trials.
• Most children with prenatal spina bifida repair require assistive devices or orthotics for mobility.
• Anatomic lesion level in the neonatal MRI is associated with ambulation status at 36 months.
1Introduction
Spina bifida aperta (SB) is a severe congenital malformation characterized by incomplete closure of the neural tube and extrusion of the non-neurulated spinal cord through defects in the vertebral arches [1–3]. Depending on the level of the defect, SB leads to variable degrees of lifelong physical disabilities including motor and sensory deficits, which are usually below the level of lesion, causing limb weakness or paralysis, orthopaedic abnormalities, faecal and urinary incontinence and variable cognitive impairment [1, 4]. Initially, neurologic deficits and neurogenic problems associated with SB were generally thought to result solely from non-neurulation [5, 6]. However, with ongoing gestation, the neural tissue is increasingly exposed to trauma and neurotoxic elements of the amniotic fluids and is progressively damaged during pregnancy [6–8]. Furthermore, the neural tube defect allows leakage of cerebrospinal fluid (CSF), predisposing the fetus to the development of hindbrain herniation, one component of the Chiari II malformation that, in turn, may hinder CSF circulation and cause hydrocephalus [3, 9]. These findings support the two-hit pathogenesis of SB, postulating that besides the non-neurulation, i.e., the first malformative hit, there is a second, i.e., functional, hit consisting of progressive toxic and mechanical damage of the developing neurologic tissue in utero [7, 10].
The Management of Myelomeningocele Study (MOMS) showed significantly better results in the open prenatal versus postnatal repair group: lower shunt rate, improved neuromotor function, and an increased likelihood of independent ambulation at 30 months [4]. Despite maternal and fetal risks, this milestone trial established open prenatal repair as a viable option for a select group of patients with SB [4, 11, 12].
Even with increased knowledge on pathogenesis and improved treatment methods, SB continues to be a major cause of chronic disability, affecting 4.6/10,000 births in Europe [1, 13, 14]. Management is complex and requires multidisciplinary treatment [13].
The investigating center offers open prenatal repair of SB as a standard procedure and its cohort is amongst the largest worldwide. It offers comprehensive postnatal care from birth until transition to adult care. Previous outcome studies from this center have shown the comparability of its results with the MOMS trial [11, 15]. As of 30 June 2022, 188 patients had undergone prenatal SB repair in this center.
This current analysis focused on the motor function outcomes at 36 months of age following prenatal SB repair. Previous studies have shown that most children achieve ambulation, with a varying degree of orthotics use, but findings imply that some motor delay must be expected, even for children with low anatomic or motor function lesion levels [4, 16]. This study also aimed to identify predictors of ambulation status such as anatomic lesion levels and motor function level. Increased knowledge on this subject might help predict the motor function outcomes of patients, thereby improving treatment planning for each individual and possibly for expecting parents.
2Methods
2.1Population
All children with prenatal SB repair born from 2011 on are prospectively enrolled in a cohort study and data is prospectively entered in REDCap provided by the Clinical Trial Center of the University Hospital. The current analysis included children born between March 2011 and December 2018. Children who had been seen at the investigating center for their 36-month comprehensive follow-up visit by December 2021 were included. Data for the 36-month follow-up was extracted from medical reports from other centers for two patients.
2.2Outcome assessment
All patients underwent standard baseline neonatal diagnostic workup with extensive follow-up visits (3, 6, 12, 18, and 24 months corrected age, and 36 months of age) at the investigating center. Motor milestones were examined by a small team of experienced paediatric neurologists and rehabilitation specialists.
Muscle strength was graded according to the Medical Research Council (MRC) Scale for Muscle Strength [17] (used with the permission of the MRC) by manual testing on a 0 to 5 graded scale as follows: (M0) no spontaneous muscle contraction, (M1) trace of contraction, (M2) movement without gravity, (M3) active movement against gravity, (M4) active movement against resistance, and (M5) normal strength.
Motor function level was defined as the lowest myotome with full muscle strength (strength of M5) in the lower extremities. Any muscle function below that (M1-M4) was considered motor partial innervation below the motor function level according to the standard neurological examination of spinal cord injury [18]. In case of an asymmetrical level of paralysis or partial innervation, the poorer side was defining. In this study, the motor function level in the neonatal period and at 36 months were included. At 36 months, most of the children could actively participate in the strength assessment; therefore, the motor function level at 36 months was seen to be more accurate in comparison to earlier assessments. Anatomic SB lesion level was determined using spinal magnetic resonance imaging (MRI) during the neonatal period and was defined as the most cranial level of bone defect (first dysraphic vertebra). To clearly differentiate between anatomic and motor function level, different wording was chosen such as thoracic (Th), lumbar (L), or sacral (S) for motor function level and thoracic vertebral body (TVB), lumbar (LVB), or sacral (SVB) for anatomic lesion level.
For comparison purposes, patients were grouped into ambulators (those walking with or without orthotics or assistive devices) and non-ambulators. For more detail, ambulation status was further divided into three groups according to how the children would predominantly function in everyday life: walking without any aids, walking with orthotics and/or assistive devices, or not being able to walk.
2.3Statistical analysis
All statistical analyses were performed using standard statistical software (IBM SPSS v28.0, IBM Corp.). Univariate descriptive analysis was performed for demographic and clinical characteristics, and results were presented as median and interquartile range (IQR) or as numbers and percentages. Comparisons between groups for continuous variables were performed using Mann-Whitney U tests as variables were non-normally distributed. Comparisons of categorical variables were assessed using Fischer’s exact test. Correlation between the motor function level at birth and at 36 months was performed using Spearman correlation. Statistically significant differences were defined by p values < 0.05.
2.4Ethics and informed consent
The study was approved by the ethical committee of the Canton of Zurich (KEK-ZH No. 2021-01101). Written informed consent was obtained from all parents or legal guardians before participation in the study.
3Results
3.1Study population and baseline characteristics
From 20 December 2010 to 1 October 2018, 97 children underwent prenatal SB repair at this center. Of those 97 patients, three were excluded: one each due to refusal of consent, neonatal death, and prenatal surgery of a non-SB lesion. Of the remaining 94 patients, four were lost to follow-up and three had a delayed 36-month follow-up due to the SARS-CoV-2 pandemic. Thus, the study population consisted of 87 children (follow-up rate: 92.6%). The median age at follow-up was 2.9 years (IQR 0.1 years). Patient characteristics (n = 87) are presented in Table 1. In this cohort, 66.7% (58/87) were born prematurely (defined as a birth below a gestational age of 37 weeks).
Table 1
Overview of the characteristics of the cohort of 87 patients after open prenatal SB repair
Parameter | Value |
Country of Residence, no. (%) | Germany: 26 (29.9%) |
Switzerland: 23 (26.4%) | |
Austria: 13 (14.9%) | |
Russian Federation: 8 (9.2%) | |
France: 7 (8.0%) | |
Slovakia: 5 (5.7%) | |
Other: 5 (5.7%) | |
Clinical characteristics | |
Gestational age at prenatal surgery (weeks) | 25.0 (IQR 1.1) |
Gestational age at birth (weeks) | 36.1 (IQR 2.3) |
Birth weight (g) | 2630 (IQR 470) |
Female sex, no. (%) | 49 (56.3%) |
CSF diversion, no. (%) | 38 (43.7%) |
Age at CSF diversion placement (months) | 5 (IQR 7.75) |
- Type of CSF diversion | |
- ETV, no. (%) | 12 (31.6%) |
- VP shunt, no. (%) | 26 (68.4%) |
Values expressed as median and interquartile range (IQR) or as number (%). CSF = cerebrospinal fluid, ETV = endoscopic third ventriculostomy, g = grams, no.=number, VP shunt = ventriculoperitoneal shunt.
3.2Motor function level
At the age of 36 months, motor function level could not be assessed in four children (4.6%, 4/87): one each due to lower limb casting, compliance, increased lower limb muscle tone, and external reporting. Of those who could be assessed, 44.6% of the patients had a motor function level of L4 (37/83) while 12.0% (10/83) showed a higher level and 43.4% (36/83) a lower level. Partial innervation was present in 90.4% (75/83) of patients, thereof 92.0% (69/75) reaching level S1 or lower.
3.3Ambulation status
Ambulation status at 36 months was available for 86 patients and unclear for one patient due to a complicated orthopaedic course. Investigation showed that 86.0% (74/86) of all children were walking, 24.4% (21/86) were ambulating without any aids, 61.6% (53/86) were dependent on orthotics or assistive devices for ambulation, and 14.0% (12/86) were not able to walk.
A lower motor function level was associated with a higher rate of ambulation at 36 months (Th12/L2 0%, L3 37.5%, L4 88.9%, L5 or S1 100%; p < .001). All 21 patients with a L5 or sacral level were ambulating (Fig. 1).
Fig. 1
Detailed classification of ambulation status according to motor function level at 36 months.
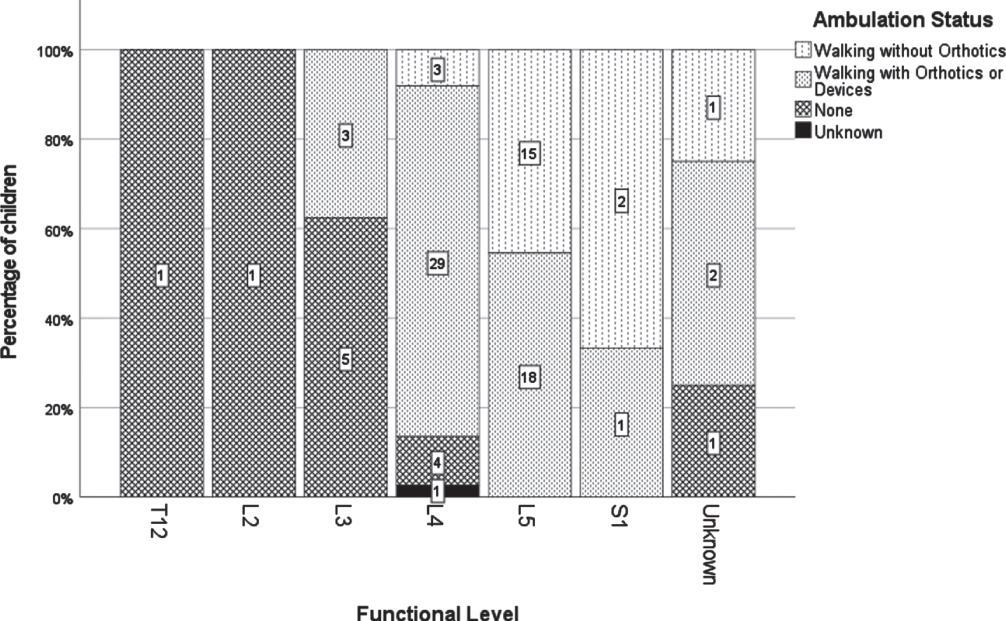
3.4Variables associated with ambulation at 36 months
Table 2 presents demographic and medical variables in relation to ambulation status at 36 months. CSF diversion was associated with poorer ambulation. Anatomic lesion level of neonatal MRI, motor function level during the neonatal period, and motor function level at 36 months were associated with ambulation at 36 months.
Table 2
Variables associated with ambulation at 36 months
Variable | Ambulators (n = 74) | Non-ambulators (n = 12) | p-value |
Gestational age at birth (weeks) | Median 36.1 | Median 36.2 | 0.288 |
(IQR 2.1) | (IQR 2.1) | ||
Birth weight (g) | Median 2670 | Median 2630 | 0.450 |
(IQR 488) | (IQR 515) | ||
Head circumference at birth (cm) | 34.1 (IQR 3) | 35 (IQR 6) | 0.303 |
Female sex, no. (%) | 40 (54.1%) | 8 (66.7%) | 0.632 |
CSF diversion, no. (%) | 27 (37%) | 10 (83.3%) | 0.002 |
Anatomic lesion level neonatal MRI (no.) | TVB10 : 0 | TVB10 : 2 | <0.001 |
TVB12 : 2 | TVB12 : 0 | ||
LVB1 : 0 | LVB1 : 2 | ||
LVB2 : 2 | LVB2 : 1 | ||
LVB3 : 9 | LVB3 : 2 | ||
LVB4 : 16 | LVB4 : 3 | ||
LVB5 : 29 | LVB5 : 1 | ||
SVB1 : 16 | SVB1 : 0 | ||
Unknown: 0 | Unknown: 1 | ||
Motor function level neonatal (no.) | L3 : 3 | L3 : 2 | 0.010 |
L4 : 24 | L4 : 5 | ||
L5 : 40 | L5 : 2 | ||
S1 : 4 | S1 : 1 | ||
Unknown: 3 | Unknown: 2 | ||
Motor function level 36 months (no.) | Th12 : 0 | Th12 : 1 | <0.001 |
L2 : 0 | L2 : 1 | ||
L3 : 3 | L3 : 5 | ||
L4 : 32 | L4 : 4 | ||
L5 : 33 | L5 : 0 | ||
S1 : 3 | S1 : 0 | ||
Unknown: 3 | Unknown: 1 |
Comparison of variables associated with ambulation at 36 months. Ambulation status missing n = 1. Cm = centimeters, CSF diversion = cerebrospinal fluid diversion, IQR = interquartile range, g = grams, no.=number, TVB = thoracic vertebral body, LVB = lumbar vertebral body, SVB = sacral vertebral body, Th = thoracic, L = lumbar, S = sacral.
3.5Age at onset of walking and motor function level
Age at onset of walking for those children who were ambulatory at 36 months ranged from 12 months (one child with motor function level L5) to 36 months (see Fig. 2). When stratified according to motor function level, 75.0% (27/36) with a level of L5–S1 were walking by 24 months and 100% by 36 months. In comparison, 34.8% (16/46) with a level of L2–L4 were walking by 24 months and 76.1% (35/46) by 36 months, respectively (p < .001).
Fig. 2
Age at onset of walking stratified by motor function level at 36 months. n = 82 due to four missing motor function levels at 36 months and one unknown age at onset of walking.
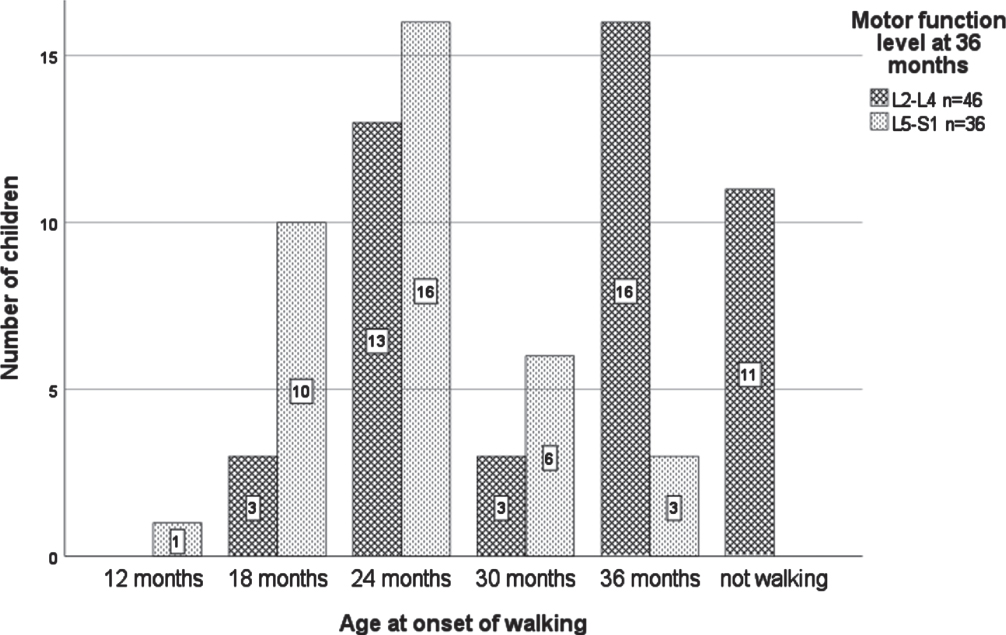
3.6Orthotics, assistive devices and physical therapy
At the age of 36 months, 81.6% (71/87) of all children wore orthotics for walking, standing, or the prevention of foot deformities. Thirty-six point eight percent (32/87) used ankle-foot orthoses, followed by supramalleolar orthoses in 14.9% (13/87), foot orthoses in 12.6% (11/87), and higher level of orthotic support including knee and hip orthoses in 4.6% (4/87). Additional upper body support (scoliosis brace) was needed for 2.3% (2/87), and 20.7% (18/87) required further types of orthotics for support (such as SWASH orthosis, orthopedic shoes, or stabilizing pressure input orthosis).
When stratified according to motor function level, orthotics were worn by 100% (1/1) of children with Th12 and L2, 75.0% (6/8) with L3, 94.6% (35/37) with L4, 72.7% (24/33) with L5, 33.3% (1/3) with S1, and 75.0% (3/4) with an unknown motor function level (p = 0.029). Orthotics were first documented at the corrected age of 12 months in 25.3% (22/87). With increasing age, more children used orthotics: 43.7% (38/87) at 18 months, 69% (60/87) at 24 months, and 81.6% (71/87) at 36 months.
Overall, assistive devices for mobility (wheelchair, walker, or stroller) were used by 47.1% (41/87). At 36 months, 14.9% (13/87) had been provided with a personalized manual wheelchair and 27.6% (24/87) with a walker; 46.2% (6/13) were solely dependent on their wheelchair for mobility; 53.8% (7/13) were wheelchair dependent for longer distances; and 10.6% (5/47) required a combination of wheelchair, walker, and/or crutches formobility.
At the adjusted age of three months, 82.8% had already started physical therapy with this percentage increasing to 90.8% (79/87) by 36 months.
3.7Comparison of anatomic lesion levels and motor function levels
Comparing the motor function level at 36 months with the anatomic lesion level determined in the neonatal MRI (Table 3), the motor function level was higher (i.e., worse = level loss) in 35.6% (31/87, range 1–2 levels) of cases, lower (i.e., better = level gain) in 24.1% (21/87, range 1–6 levels), and equal to the anatomic lesion level in 34.5% (30/87). As seen in Table 3, when grouped according to anatomic lesion level into high-level (TVB10-LVB3) and low-level (LVB4-SVB1) lesions, all children with high-level anatomic lesions (n = 20) demonstrated same or lower motor function levels (= level gain) at 36 months. However, those with low-level anatomic lesions (n = 66) often showed worse motor function levels (i.e., level loss). To detail this further, 20% (5/20) with anatomic lesion level LVB4, 40% (12/30) of LVB5, and 87.5% (14/16) of SVB1 showed level loss.
Table 3
Comparison between motor function level and anatomic lesion level
Comparison of motor function level with anatomic lesion level, n = 86 | Anatomic lesion level | Anatomic lesion level |
TVB10-LVB3 | LVB4-SVB1 | |
Motor level 2 levels worse than anatomic level | 0 (0) | 6 (9.1) |
Motor level 1 level worse than anatomic level | 0 (0) | 25 (37.9) |
Motor level = anatomic level | 2 (10) | 28 (42.4) |
Motor level 1 level better than anatomic level | 6 (30) | 3 (4.5) |
Motor level≥2 levels better than anatomic level | 11 (55) | 1 (1.5) |
Unknown motor level | 1 (5) | 3 (4.5) |
Total | 20 (100) | 66 (100) |
Comparison between motor function level at 36 months and anatomic lesion level of neonatal MRI, values expressed as number (%). Percentages may not total 100 due to rounding. Data of neonatal MRI was missing for one child (n = 1). TVB = thoracic vertebral body, LVB = lumbar vertebral body, SVB = sacral vertebral body.
Motor function level during the neonatal period was associated with the motor function level at 36 months (p < 0.001), as well as ambulation status at 36 months (p = 0.01, Table 2).
4Discussion
Prenatal surgery has become the standard of care for the treatment of SB for qualifying patients [11, 15]. This cohort of children after prenatal SB repair is amongst the largest worldwide. In 2014, the “Fetal Myelomeningocele Maternal-Fetal Management Task Force” composed a position paper, outlining six key areas of importance for teams performing in utero prenatal SB repair [19]. These key areas include establishing criteria for fetal therapy centers, providing fetal myelomeningocele repair and perioperative management to secure optimal maternal and pediatric outcomes, ensuring long term care as well as regular counselling, commitment to outcome reporting and monitoring, as well as ensuring access to treatment [19].
This study reported and monitored outcomes from the 36-month follow-up. The results provide crucial information for counselling expecting parents, setting realistic outcome expectations, and improving the accuracy of treatment planning in the postnatal clinical setting.
Independence in mobility has been shown to be a key determinant for health-related quality of life in children with SB, promoting mobility and active participation in community activities [20]. Furthermore, negative effects of non-walking such as bone demineralization, decubital ulcers, and social isolation can be prevented [21]. Knowledge of the development of ambulation ability can help tailor treatment planning for rehabilitation specialists and physical therapists and will provide information for optimal parental counselling in the pre- and postnatal setting.
When assessing ambulation ability at the age of 36 months, the majority (86%) of children after prenatal repair were walking (61.6% with and 24.4% without orthotics or assistive devices). Comparably, in the benchmark MOMS trial, 71% of the children were walking (29% with and 42% without orthotics or assistive devices) at 30 months [4]. In a follow-up assessment of the MOMS trial (MOMS2) at a mean age of 7.5 years, Houtrow et al. [16] identified 90.8% as walkers in the prenatal repair group. In comparison, in the postnatal SB repair group, only 71.8% were walkers at the school-age follow-up. Their results demonstrated that ambulation development persists into school age, underlining the beneficial outcome of prenatal repair for spina bifida, and is a promising perspective for patients concerning ambulation. The investigating center is dedicated to following this cohort and their clinical course and to collecting and reporting data accordingly.
Motor function level and corresponding muscle strength are important factors for attaining and maintaining ambulation [20, 21]. The motor function level was defined as the lowest myotome with full muscle strength (i.e., strength of M5) and any muscle function below that (M1-M4) was considered partial innervation. This strict criteria of defining motor function level including information on partial innervation was chosen to allow for differentiation between the various levels of motor function. As no single classification system for defining motor function level has yet prevailed in the literature [16, 22, 23], there is a lack of uniform assessment for motor function in SB, which limits comparison of results between studies. This difficulty is undermined by the forthgoing efforts to find a common classification system, as reported by Dias et al. in 2021 [24]. In comparison to the Broughton classification applied by Houtrow et al. in the MOMS2 study [16], the classification system applied here was stricter. For example, the Broughton classification defines S2 level as 3–5/5 muscle strength in the plantar flexors [25]. The criteria applied in this study required 5/5 muscle grade of the plantar flexors for motor function S1 level. Partial strength of the plantar flexors would have resulted in a motor function level of L5 or higher.
Besides the association between the motor function level and ambulation status, the need for CSF diversion negatively correlated with ambulation status [26]. A possible explanation for this finding is that ventricular distention during brain development may injure or alter the corticospinal pathways with greater involvement of the lower extremities due to the longer course of the paracentral leg fibres [27].
In this study, the developmental aspect of mobility was also described. Children with a lower motor function level, and therefore better muscle function in the lower limbs, were ambulating earlier. Walking was first observed at the follow-up at the corrected age of 12 months. However, evaluation of walking ability showed a delay compared to typically developing children. In Switzerland, a longitudinal cohort study consisting of 220 normally developing children showed that 80% were already walking independently between 10.5 and 16 months of age compared to 85.1% of patients with prenatal SB repair walking at 36 months [28]. Clinical experience has shown that counselling parents regarding ambulation and its often delayed onset for their child must be thorough and timely. Therefore, it is important to present realistic level-dependent data that can be used as guidance for parental and caregiver counselling.
This study also highlighted the importance of orthotic management. Early orthotic management and physical therapy are important to support the development of physical functioning and motor potential and should have a positive effect on achieving and maintaining later mobility, as well as preventing secondary structural deformities during growth [29]. Children with SB benefit from early orthotic provisions to compensate for weakness and instability and promote ambulation. Despite the improved motor function after prenatal SB repair, most of the ambulating children in this study still required assistive devices or orthotics for mobility. Approximately 24% ambulated without the need for bracing or devices. Over half of the children ambulated at the age of 36 months with free use of their hands, because they walked either completely independently or with orthotics but did not require a walker or wheelchair for mobility. The free use of hands allows greater freedom and independence in performing everyday tasks and encourages social interaction with other children. Physical therapy was usually initiated, often at an early age. At 36 months, only three children had never had physical therapy in the outpatient setting. The type, for example Bobath or Vojta, and frequency of physical therapy administered varied greatly since children in this cohort came from Russia and various countries across Europe.
The first motor function level after birth is determined during the neonatal period and is expected to be a predictor of motor function outcome. The motor function level after birth was associated with ambulation status at the age of 36 months. However, it must be noted that it is challenging to determine a reliable motor function level in newborns, as differentiation between voluntary motor function and reflexive movements is demanding. In this study, the anatomic lesion level determined in the neonatal MRI was also associated with ambulation outcome. Importantly, the results of the prenatal MRI were not included. A previous study from this center showed that prenatal imaging using ultrasound or MRI for determining anatomic lesion level predicted neonatal anatomical level within one level margin of error, more likely predicting a higher lesion level [30]. Therefore, caution must be taken when predicting outcomes of motor function based on anatomic lesion levels in the prenatal MRI. In this study, the anatomic lesion level was defined using the neonatal MRI. On the other hand, both the MOMS and MOMS2 trials determined the anatomic lesion level using x-ray of the spine at the age of 12 months [4, 16]. The aim was to define possible prognostic factors for outcome on motor function at 36 months and beyond; therefore, the focus was placed on precise imaging using the neonatal MRI.
Still, caution must be taken in predicting outcomes on motor function based solely on anatomical lesion levels. In looking closer at the results of the neonatal MRI, children with a high-level anatomic lesion more frequently had motor function levels that were better than their anatomic lesion level (i.e., level gain), whereas those with low-level anatomic lesions were less likely to have motor function levels better than their anatomic lesion level (i.e., level loss). In this study population, the majority of children with a lesion level of SVB1 (87.5%, 14/16) alarmingly showed worse than predicted motor function levels (level loss). The reason is not entirely clear. On one hand, a motor function level of S1 was only assigned when the plantar flexors and hip extensors showed full muscle strength (M5). This constellation of clinical findings is rare due to the nature of the neural defect. Other factors need to be evaluated, such as the effect of size and type of lesion (myelomeningocele versus myeloschisis) on motor function outcome. This finding should be taken into account during prenatal counselling. Further investigation of the causes of level loss is necessary.
Over the past years, continual progress has been made in the treatment of patients undergoing prenatal surgery for SB. Nevertheless, SB continues to be a major cause of chronic disability requiring multidisciplinary treatment and there is still only limited information about the long-term ambulation prognosis. This study provided an overview of current level-dependent outcomes of motor function of children at the age of 36 months after prenatal SB repair in a non-trial setting outside of the United States. These results can be used as guidance for caregivers for realistic outcomes of motor function, to help tailor rehabilitative efforts, as well as to convey expectations for parents and caregivers. The onset of ambulation usually started by the end of the second year of life and continued until most children were ambulatory at the age of 36 months. Despite the improved motor function after prenatal SB repair, most ambulating children still required assistive devices or orthotics for mobility. These findings accentuate the uncertainty and difficulty in precise prediction of motor function outcome along with the challenges in comparison of findings between centers. They also affirm the importance of long-term care by a stable, experienced clinical team. Further investigations should continue to evaluate predictors and whether these findings are stable during adolescence, early adulthood, and beyond.
5Limitations & strengths
There were several limitations of this study. A postnatal comparison group would have been helpful. However, due to the small number of patients undergoing postnatal repair for SB at this center (n = 19 since 2011) with divergent inclusion and comparison criteria (such as syndromic disease or maternal factors), a real control group could not be established. Importantly, based on the favourable outcomes of prenatal repair for SB reported in the MOMS and MOMS2 benchmark trials, a randomized controlled trial is no longer justifiable [4, 16].
Furthermore, due to differences in functional level assessment throughout the literature, outcome comparisons were limited. Efforts are ongoing to reach a consensus. The center’s extensive databank allows for further analysis and contribution to these efforts.
Patients’ origins were limited to Europe and Russia. Results may differ in other ethnic groups, especially as larger studies in the US have shown that Hispanics have a higher SB prevalence compared with non-Hispanic whites [31].
Due to the young age at follow-up, further long-term re-evaluations will be necessary for more detailed conclusions regarding the developmental trajectories of ambulation status during growth. Correct assessment of the motor function level can be challenging and therefore imprecise in young children and is prone to subjectivity. Due to inhomogeneous distribution of ambulation status at 36 months resulting in small groups, statistical testing could not be performed to determine possible predictors of ambulation outcome at 36 months.
All of the children in this study underwent standard baseline diagnostic workup with extensive follow-up appointments and data was collected on a regular basis. Motor function levels and the developmental progress of each individual were closely monitored over time, enabling a precise description of this patient cohort. Using pre- and neonatal MRI allowed for accurate determination of anatomical lesion level compared to the x-ray of the spine used in the MOMS trial [4]. This commitment to collecting extensive data paves the way for substantial contributions to future research on prenatal SB repair and long-term outcomes, especially to define prenatal prognostic factors for favourable outcomes.
6Conclusion
At 36 months, most children with open prenatal repair for SB showed favourable ambulation status. However, most still required assistive devices or orthotics. Anatomic lesion level of neonatal MRI, motor function level during the neonatal period, and motor function level at 36 months were associated with ambulation status at 36 months. Reliable predictors of long-term motor function outcome are still being determined.
Acknowledgments
The authors want to first thank all families who participated in this research. In addition, we thank our contributing study group without whom this research would not be possible. From the University Children’s Hospital this includes Barbara Casanova, Thomas Dreher, Ruth Etter, Patrice Grehten, Domenic Grisch, Annette Hackenberg, Cornelia Hagmann, Raimund Kottke, Niklaus Krayenbuehl, Claudia M. Kuzan-Fischer, Maya Horst Luethy, Markus A. Landolt, Luca Mazzone, Andreas Meyer-Heim, Theres Moehrlen, Svea Muehlberg, Silke Quanz, Brigitte Seliner, Mithula Shellvarajah, Sandra P. Toelle, Julia Velz, Alexandra Wattinger and Noemi Zweifel. From the University Hospital Zurich, our study group consists of Dirk Bassler, Lukas Kandler, Salome Meyer, Nicole Ochsenbein, Christian Schaer, Nele Struebing and Ladina Vonzun. We thank the Clinical Trial Center, University Hospital of Zurich, for hosting the REDCap® Database.
Conflict of interest
The authors have no conflicts of interest or funding to report.
References
[1] | Copp AJ , Adzick NS , Chitty LS , Fletcher JM , Holmbeck GN , Shaw GM . Spina bifida. Nat Rev Dis Primers. (2015) ;1: :15007. doi: 10.1038/nrd2015.7 |
[2] | Farmer DL , Thom EA , Brock JW , et al. The Management of Myelomeningocele Study: full cohort 30-month pediatric outcomes. Am J Obstet Gynecol. (2018) ;218: (2):256.e1–256.e13. doi: 10.1016/j.ajog.2017.12.001 |
[3] | Peranteau WH , Adzick NS . Prenatal surgery for myelomeningocele. Curr Opin Obstet Gynecol. (2016) ;28: (2):111–8. doi: 10.1097/GCO.0000000000000253 |
[4] | Adzick NS , Thom EA , Spong CY , et al. A randomized trial of prenatal versus postnatal repair of myelomeningocele. N Engl J Med. (2011) ;364: (11):993-–1004. doi: 10.1056/NEJMoa1014379 |
[5] | Meuli M , Moehrlen U . Fetal surgery for myelomeningocele is effective: a critical look at the whys. Pediatr Surg Int. (2014) ;30: (7):689–97. doi: 10.1007/s00383-014-3524-8 |
[6] | Stiefel D , Copp AJ , Meuli M . Fetal spina bifida in a mouse model: loss of neural function in utero. J Neurosurg. (2007) ;106: (3 Suppl):213–21. doi: 10.3171/ped.2007.106.3.213 |
[7] | Meuli M , Meuli-Simmen C , Hutchins GM , et al. In utero surgery rescues neurological function at birth in sheep with spina bifida. Nat Med. (1995) ;1: (4):342–7. doi: 10.1038/nm0495-342 |
[8] | Adzick NS , Sutton LN , Crombleholme TM , Flake AW . Successful fetal surgery for spina bifida. Lancet. (1998) ;352: (9141):1675–6. doi: 10.1016/S0140-6736(98)00070-1 |
[9] | Bouchard S , Davey MG , Rintoul NE , Walsh DS , Rorke LB , Adzick NS . Correction of hindbrain herniation and anatomy of the vermis after in utero repair of myelomeningocele in sheep. J Pediatr Surg. (2003) ;38: (3):451–8; discussion 451-8. doi: 10.1053/jpsu.2003.50078 |
[10] | Meuli M , Meuli-Simmen C , Yingling CD , et al. Creation of myelomeningocele in utero: a model of functional damage from spinal cord exposure in fetal sheep. J Pediatr Surg. (1995) ;30: (7)::1028–32; discussion 1032-3. doi:10.1016/0022-3468(95)90335-6 |
[11] | Möhrlen U , Ochsenbein-Kölble N , Mazzone L , et al. Benchmarking against the MOMS Trial: Zurich Results of Open Fetal Surgery for Spina Bifida. Fetal Diagn Ther. (2020) ;47: (2):91–7. doi: 10.1159/000500049 |
[12] | Moldenhauer JS , Soni S , Rintoul NE , et al. Fetal myelomeningocele repair: the post-MOMS experience at the Children’s Hospital of Philadelphia. Fetal Diagn Ther. (2015) ;37: (3):235–40. doi: 10.1159/000365353 |
[13] | Protzenko T , Bellas A , Pousa MS , et al. Reviewing the prognostic factors in myelomeningocele. Neurosurg Focus. (2019) ;47: (4):E2. doi: 10.3171/2019.7.FOCUS19462 |
[14] | Khoshnood B , Loane M , de Walle H , et al. Long term trends in prevalence of neural tube defects in Europe: population based study. BMJ. (2015) ;351: :h5949. doi: 10.1136/bmj.h5949 |
[15] | Moehrlen U , Ochsenbein N , Vonzun L , et al. Fetal surgery for spina bifida in Zurich: results from 150 cases. Pediatr Surg Int. (2021) ;37: (3):311–6. doi: 10.1007/s00383-020-04824-8 |
[16] | Houtrow AJ , MacPherson C , Jackson-Coty J , et al. Prenatal Repair and Physical Functioning Among Children With Myelomeningocele: A Secondary Analysis of a Randomized Clinical Trial. JAMA Pediatr. (2021) ;175: (4):e205674. doi: 10.1001/jamapediatrics.2020.5674 |
[17] | Medical Research Council. Aids to examination of the peripheral nervous system. Memorandum no. 45. London (GB): Her Majesty’s Stationary Office; 1976. |
[18] | Roberts TT , Leonard GR , Cepela DJ . Classifications In Brief: American Spinal Injury Association (ASIA) Impairment Scale. Clin Orthop Relat Res. (2017) ;475: (5):1499–504. doi: 10.1007/s11999-016-5133-4 |
[19] | Cohen AR , Couto J , Cummings JJ , et al. Position statement on fetal myelomeningocele repair. Am J Obstet Gynecol. (2014) ;210: (2):107–11. doi: 10.1016/j.ajog.2013.09.016 |
[20] | Schoenmakers MAGC , Uiterwaal CSPM , Gulmans VAM , Gooskens RHJM , Helders PJM . Determinants of functional independence and quality of life in children with spina bifida. Clin Rehabil. (2005) ;19: (6):677–85. doi: 10.1191/0269215505cr865oa |
[21] | Pauly M , Cremer R . Levels of mobility in children and adolescents with spina bifida-clinical parameters predicting mobility and maintenance of these skills. Eur J Pediatr Surg. (2013) ;23: (2):110–4. doi: 10.1055/s-0032-1324689 |
[22] | Danzer E , Gerdes M , Bebbington MW , et al. Lower extremity neuromotor function and short-term ambulatory potential following in utero myelomeningocele surgery. Fetal Diagn Ther. (2009) ;25: (1):47–53. doi: 10.1159/000197359 |
[23] | Tita AC , Frampton JR , Roehmer C , Izzo SE , Houtrow AJ , Dicianno BE . Correlation Between Neurologic Impairment Grade and Ambulation Status in the Adult Spina Bifida Population. Am J Phys Med Rehabil. (2019) ;98: (12):1045–1050. doi: 10.1097/PHM.0000000000001188 |
[24] | Dias LS , Swaroop VT , de Angeli LRA , Larson JE , Rojas AM , Karakostas T . Myelomeningocele: a new functional classification. J Child Orthop. (2021) ;15: (1):1–5. doi: 10.1302/1863-2548.15.200248 |
[25] | Broughton NS , Menelaus MB , Cole WG , Shurtleff DB . The natural history of hip deformity in myelomeningocele. J Bone Joint Surg Br. (1993) ;75: (5):760–763. doi: 10.1302/0301-620X.75B5.8376434 |
[26] | Dicianno BE , Karmarkar A , Houtrow A , et al. Factors Associated with Mobility Outcomes in a National Spina Bifida Patient Registry. Am J Phys Med Rehabil. (2015) ;94: (12):1015–25. doi: 10.1097/PHM.0000000000000404 |
[27] | Palm WM , Saczynski JS , van der Grond J , et al. Ventricular dilation: association with gait and cognition. Ann Neurol. (2009) ;66: (4):485–93. doi: 10.1002/ana.21739 |
[28] | Jenni OG , Chaouch A , Caflisch J , Rousson V . Infant motor milestones: poor predictive value for outcome of healthy children. Acta Paediatr. (2013) ;102: (4):e181–4. doi: 10.1111/apa.12129 |
[29] | Schmid T , Strehl E , Trollmann R , Forst R , Fujak A . Orthotic Care Based on the Ferrari Concept for Children and Adults with Meningomyelocele. [Orthotic Care Based on the Ferrari Concept for Children and Adults with Meningomyelocele]. Z Orthop Unfall. (2019) ;157: (6):695–705. doi: 10.1055/a-0853-8009 |
[30] | Vonzun L , Kahr MK , Wille D , et al. Determination of Anatomical Levels in Spina Bifida Fetuses with Ultrasound and MRI. Ultraschall Med. (2022) ;43: (2):181–185. doi: 10.1055/a-1264-7785 |
[31] | Canfield MA , Ramadhani TA , Shaw GM , et al. Anencephaly and spina bifida among Hispanics: maternal, sociodemographic, and acculturation factors in the National Birth Defects Prevention Study. Birth Defects Res A Clin Mol Teratol. (2009) ;85: (7):637–46. doi: 10.1002/bdra.20582 |