Cognitive Effects of Three β-Adrenoceptor Acting Drugs in Healthy Volunteers and Patients with Parkinson’s Disease
Abstract
Background:
Noradrenergic signaling declines in Parkinson’s disease (PD) following locus coeruleus neurodegeneration. Epidemiologic studies demonstrate that β-acting drugs slow PD progression.
Objective:
The primary objective was to compare the safety and effects of 3 β-adrenoceptor (β-AR) acting drugs on central nervous system (CNS) function after a single dose in healthy volunteers (HVs) and evaluate the effects of multiple doses of β-AR acting drugs in HVs and PD-patients.
Methods:
In Part A, HVs received single doses of 32 mg salbutamol, 160μg clenbuterol, 60 mg pindolol and placebo administered in a randomized, 4-way cross-over study. In Part B (randomized cross-over) and Part C (parallel, 2:1 randomized), placebo and/or clenbuterol (20μg on Day 1, 40μg on Day 2, 80μg on Days 3–7) were administered. CNS functions were assessed using the NeuroCart test battery, including pupillometry, adaptive tracking and recall tests.
Results:
Twenty-seven HVs and 12 PD-patients completed the study. Clenbuterol improved and pindolol reduced the adaptive tracking and immediate verbal recall performance. Clenbuterol and salbutamol increased and pindolol decreased pupil-to-iris ratios. Clenbuterol was selected for Parts B and C. In Part B, clenbuterol significantly increased performance in adaptive tracking with a tendency toward improved performance in immediate and delayed verbal recall. In Part C trends toward improved performance in immediate and delayed verbal recall were observed in PD-patients. Typical cardiovascular peripheral β 2-AR effects were observed with clenbuterol.
Conclusions:
This study demonstrates the pro-cognitive effects of clenbuterol in HVs with similar trends in PD-patients. The mechanism of action is likely activation of β2-ARs in the CNS.
Plain Language Summary
Aims and Purpose of the Research:
This research aimed to explore how three different drugs affect brain function. These drugs are salbutamol, clenbuterol, and pindolol and work in the brain by stimulating specific brain cells that can improve aspects like memory and coordination. The main question was to see how safe these drugs were and how they impact the brain function after one dose in healthy people, and after multiple doses in both healthy people and those with Parkinson’s disease.
Background of the Research:
Parkinson’s disease is a condition where brain cells start to die, which affects different areas of the brain, including movement function, as well as memory and attention. This research matters because finding drugs that affect the brain function could improve the lives of people with Parkinson’s disease.
Methods and Research Design:
The study was conducted in three parts. In the first part, healthy volunteers took one dose of each of the three drugs— salbutamol, clenbuterol, and pindolol— as well as a placebo (a harmless pill that has no effect). The researchers tested the participants’ brain functions using various tasks including memory tests and eye response measurements. In the second and third part, healthy people and people with Parkinson’s disease took the drug that performed best in healthy volunteers for seven days.
Results and Importance:
In the first part, a single dose of clenbuterol was safe and improved memory and attentions tasks in healthy people, and therefore was chosen for further testing in the second and third part. In these parts, multiple doses of clenbuterol were safe and helped improve memory and attention tasks in healthy people, with similar positive trends seen in people with Parkinson’s disease. The study suggests that clenbuterol might help improve brain function by activating specific receptors in the brain.
These results are important because they suggest that clenbuterol could be a potential treatment to help improve brain function in people with Parkinson’s disease. However, more research is needed to fully understand its effects and to confirm these findings.
INTRODUCTION
The adrenergic system plays a crucial role in regulating physiological processes such as heart rate, blood pressure, and metabolism via two transmitters, adrenaline (Adr) and noradrenaline (NA) that also play important stimulatory roles in central nervous system (CNS) function.1 Recent research has suggested that early dysfunction of the adrenergic system of the brain arising from the locus coeruleus (LC) may contribute to the development and progression of neurodegenerative disorders such as Parkinson’s disease (PD)2,3 and Alzheimer’sdisease (AD).4–7
PD is a common, progressive, and debilitating neurodegenerative disorder classically characterized by both motor and non-motor symptoms. Motor symptoms include tremor, rigidity, bradykinesia, and postural and balance disorders.8 There is a growing appreciation of the burden and poor medical management of non-motor symptoms,9–12 including cognitive dysfunction,13 neuropsychiatric symptoms,14 autonomic dysfunction,10,12 sleep disorders,15–18 and sensory dysfunction.19
Neuroanatomical staging studies show that neurodegeneration in the LC occurs very early in PD.20,21 Consistent with this, positron emission tomography (PET) studies with 11C-MeNER, a highly selective tracer for NA transporters, shows reduced binding, and by inference, reduced density of noradrenergic axon projections in the brain of PD-patients compared with age-matched healthy controls.22 Consequently, decline of this LC projection system may be causally associated with loss of cognition, mood, and brain health, and revitalizing the influence of adrenergic receptor stimulation represents a potentially important pharmacological approach for the treatment of PD symptoms and disease progression.
Several pharmacoepidemiologic studies suggest that chronic treatment with β-adrenoceptor (β-AR) acting drugs is associated with a significantly reduced incidence of PD.23–28 Additional data also suggest that the effect of β-AR acting drugs may generalize to other neurodegenerative disorders such as AD.29,30 The concept is further supported by evidence of pro-cognitive and/or disease modifying effects of β-AR acting drugs in rodent models of neurodegenerative diseases.31–33
This study investigated the effects of three β-AR acting drugs— salbutamol (also known as albuterol), pindolol, and clenbuterol— on CNS function in both healthy volunteers (HVs) and PD patients. Since it is not clear which β-AR subtype is likely to be the ideal one to target for the treatment of neurodegenerative disorders, this study investigated β-AR acting drugs that have a distinct pharmacology from one another and are able to cross the blood-brain barrier. Salbutamol is a potent β2-AR agonist with modest CNS absorption, normally used as short acting β-agonist to treat pulmonary disorders. Pindolol acts as a β2-AR antagonist and a β1-AR partial agonist with moderate to high CNS absorption. Clenbuterol is a selective β2-AR agonist with high CNS absorption, normally used as long acting β-agonist to treat pulmonary disorders (unpublished data).27,34–36 The study aimed to (a) characterize and compare the effects of these three agents with placebo on CNS function and safety parameters such as adverse events and effects on heart rate and blood pressure after a single oral dose; (b) assess the safety and CNS effects of multiple doses of one agonist selected from observations in the single-dose cohort in HVs; and (c) similarly evaluate the safety and CNS effects in a small cohort of PD patients.
MATERIALS AND METHODS
Participants
This 3-part, randomized, placebo-controlled study was conducted at a single site in the Netherlands in accordance with the International Conference for Harmonization (ICH) of Good Clinical Practice (GCP), the principles of the Declaration of Helsinki (1964) and ethical principles as referenced in EU Directive 2001/20/EC. The protocol and all study materials were approved by the Medical Research Ethics Committee of the BEBO foundation (Assen, the Netherlands) and prospectively registered in the Dutch Trial Register (number NL8002), and all volunteers provided their written informed consent before participation. The study was conducted between September 2019 and March 2020 at a single site in the Netherlands (Centre for Human Drug Research [CHDR], Leiden). The study was completed when the planned enrolment had been reached and all enrolled participants completed the scheduled study assessments.
Inclusion and exclusion criteria
In Part A and Part B, male or female HVs 35–60 years of age were eligible to participate if they weighed ≥50 kg, with BMI 18–35 kg/m2, and were free from clinically significant abnormalities based on medical history, physical examination, 12-lead electrocardiogram (ECG), and laboratory tests (serum chemistry, hematology, coagulation, urine drug screen, and urinalysis). Use of any prescription- or over-the-counter medication or herbal supplements were excluded, except paracetamol/acetaminophen up to 4 g/day, oral contraceptives, and hormone replacement therapy. In Part C, male or female patients with PD 40–75 years of age with a confirmed diagnosis of PD (defined as bradykinesia in addition to resting tremor, rigidity, or impairment of postural reflexes with no known or suspected cause37), were eligible if they were assessed to be at Hoehn & Yahr stage ≤3,38 had a Mini-Mental Status Examination score (MMSE) ≥2639 and no clinically significant abnormalities based on medical history, physical examination, ECG, and laboratory tests as deemed by the investigator. In these participants, use of dopamine agonists or catechol-O-methyltransferase inhibitors for treatment of PD was allowed if stable for ≥60 days and stable use (≥3 months) of medications for treatment of, e.g., hypertension, dyslipidemia blood pressure, and vitamin E (up to 400 IU daily), estrogens, aspirin (81–300 mg daily). Use of adrenergic agents was not permitted during the study except for β-AR blockers for treatment of tremors which were discontinued at least 48 h prior to NeuroCart testing. Pregnant or nursing women were excluded from participation in all parts of this study. Participants or their partners, unless confirmed sterile or postmenopausal, were required to use a condom or two effective birth control methods during penile-vaginal intercourse throughout the study.
Dose selection
In Part A, HVs were randomly assigned to receive single oral doses of salbutamol (32 mg), clenbuterol (160μg), pindolol (20 mg in the first five HVs and 60 mg in the subsequent HVs) and placebo in 1 of 4 treatment sequences (using a William’s square, 4 period crossover design) with a washout period of approximately 7 days between doses. The doses selected for the three active drugs conform with the upper ranges of daily allowed doses/exposures approved for use in humans for treatment of pulmonary or cardiovascular diseases.40–42 The decision to increase the dose of pindolol was based on emerging observations in a separate study, in which pindolol had no effect on cerebral blood flow in any of the brain regions of interest analyzed using Arterial Spin Labeling (unpublished data). Also, in this study, pindolol was tolerated well up to 20 mg (unpublished data). Commercially available oral tablets of salbutamol (4 mg tablets, GlaxoSmithKline), clenbuterol (Spiropent tablets, 20μg; Boehringer Ingelheim), and pindolol (10 mg tablets, Teva Canada) and placebo were administered.
After interim review of safety and CNS data from Part A, one of the three acting drugs, clenbuterol was selected to be administered in the subsequent parts of the study (Part B and Part C). Based on the long plasma half-life of clenbuterol, an oral dose of up to 80μg clenbuterol hydrochloride daily for 7 days was selected as this was anticipated to deliver a steady-state plasma concentration similar to the exposure achieved in Part A following a single dose of 160μg clenbuterol hydrochloride. In Part B, 16 HVs received 80μg clenbuterol and placebo in a double-blind, 2-period crossover design. HVs were randomized 1:1 to the treatment sequence (active-then-placebo, vs. placebo-then-active). Clenbuterol hydrochloride was up titrated over the dosing period as follows: 20μg on Day 1, 40μg on Day 2 and 80μg on Day 3 to Day 7. The two treatment periods were separated by a washout period of at least 14 days. Finally, in Part C of this study, 12 participants with PD were randomized 8:4 to receive 80μg clenbuterol or placebo, administered once daily for 7 days. Clenbuterol hydrochloride was up titrated over the dosing period as follows: 20μg on Day 1, 40μg on Day 2 and 80μg on Day 3 to Day 7. The final study visit was completed 7 (±2) days after the last study drug administration.
Randomization and blinding
For all parts of this study, randomizations codes were generated using SAS version 9.1.3 (SAS Institute Inc. Cary, NC, USA; 2004) by a statistician who was otherwise not involved in this study. Parts A and B were conducted in a double-blind fashion, while Part C of the study was single-blind (investigator blinded). In Part A, an unblinded physician administered the different tablets to the HVs who were blindfolded during drug administration to avoid recognition. Participants, study staff and investigators who evaluated safety and treatment effects were blind to treatment assignment; randomization codes were known only to the unblinded pharmacist who dispensed study medications and an unblinded physician who administered the doses.
Outcome measurements
In all parts of the study, safety and tolerability (including adverse events (AEs) vital signs, electrocardiograms, physical examinations, routine clinical chemistry, and hematology assessments), plasma drug concentrations and pharmacokinetics, and CNS effects were evaluated. CNS effects were measured using the NeuroCart test battery, a validated set of tests used to measure the CNS effects of drugs in a standardized manner.43 The tests included in the NeuroCart test battery and their related CNS domains are described in Table 1. The NeuroCart test battery included the following tests: saccadic eye movement, smooth pursuit, adaptive tracking, body sway, pupil size, visual verbal learning test, Stroop color-word interference task, visual analogue scale (VAS) Bond and Lader, VAS Bowdle, and pharmaco-electroencephalography.
Table 1
Neurocart test battery, EEG and related CNS domains
NeuroCart test | Targeted function and Description of the test | Related CNS areas |
Saccadic eye movement | Alertness, vigilance.58,63 The measurement of saccadic eye movements, specifically saccadic peak velocity, is highly sensitive for assessing sedation. Saccadic eye movements are captured using a moving dot displayed on a computer screen. Parameters such as latency, saccadic peak velocity, and inaccuracy of saccades were measured and analyzed. | Superior colliculus, substantia nigra, amygdala |
Smooth pursuit | Visuomotor coordination.58,63 In the case of smooth pursuit eye movements, the target moved at frequencies ranging from 0.3 to 1.1 Hz, in increments of 0.1 Hz. The amplitude of target displacement corresponded to a rotation of 22.5 degrees for both sides of the eyeball. Four cycles were recorded for each stimulus frequency. The duration of time during which the eyes tracked the target in smooth pursuit was calculated for each frequency and expressed as a percentage of the stimulus duration. The average percentage of smooth pursuit across all stimulus frequencies was used as a parameter. | Midbrain |
Adaptive tracking | Attention, visuomotor coordination.64 The adaptive tracking test was conducted using custom equipment and software, which was developed by Hobbs and Strutt, according to specifications of Borland and Nicholson. Analysis involved the average performance and standard deviation of scores over a 3.5-minute period, excluding the initial 0.5-minute run-in time. The task required the subject to track a moving circle on the screen by controlling a joystick to keep a dot within the target circle. Performance was deemed to be successful if the participant tracked the target at high speeds, while failure occurred when the velocity for tracking the target was low. | Neocortex, basal nuclei, brain stem, cerebellum |
Body sway | Motor coordination, postural balance.65 Postural stability was assessed using a body sway meter, which measured body movements in a single plane to determine postural stability. The measurement of body sway was conducted using a pot string meter based on the Wright ataxiameter. A string was attached to the subject’s waist, capturing all body movements over a specific time period and quantifying them as millimeters of sway. The total measurement duration for body sway was two minutes. | Cerebellum, brain stem |
Pupil size | Brain stem function. A digital camera was used to measure the size of subject’s pupils at different time points. One picture was taken from both eyes simultaneously, and the ratio between the pupil- and iris diameter was measured. | Brain stem, medulla oblongata |
Visual verbal learning test (VVLT) | Episodic memory. Subjects that perform the VVLT were presented 30 words in three consecutive word trials, i.e. word learning test. Each trial ended with a free recall of the presented words (Immediate Recall) test to determine acquisition and consolidation of information). Approximately 2.5 h after start of the first trial, the subjects were asked to recall as many words as possible (Delayed Recall- this test measures active retrieval from long term memory). Immediately thereafter, the subjects underwent memory recognition test, which consisted of 15 presented words and 15 ‘distractors’ (Delayed Recognition- testing memory storage). A different word list was presented to each subject during the different occasions, in this way a subject was presented with a new word list each measurement. | Hippocampus |
Stroop color-word interference task | A two-trial version of the color-word Stroop task was presented to the test subjects. In the first trial, 6 colored items were presented at random. The subjects were asked to respond as fast and as accurately as possible by pressing the keys 1, 2 or 3 on the number pad with the index finger, middle finger and ring finger of the dominant hand, corresponding with the correct answer. In the second trial, which appeared directly after the first trial, 34 color and word pairs were presented randomly to the subject, forming either congruent or incongruent matches. The subjects were again asked to respond as fast as possible by pressing the keys 1, 2 or 3 on the numpad, corresponding with the correct answer. Three colors were shown, which are green, red and blue. The colored items were presented in a random order. | |
Visual Analogue Scale (VAS) Bond and Lader | Alertness, mood, calmness.66 Participants were asked to indicate with a mouse click on the computer screen how they felt on sixteen visual analogue scales from which the following 3 main factors were calculated as described by: alertness (from nine scores), contentedness (often called mood; from five scores), and calmness (from two scores). | Cortex, prefrontal cortex |
Visual Analogue Scale (VAS) Bowdle | Feeling high, internal and external perception.67–69 Potential subjective psychotomimetic (psychedelic) effects of antiglutamatergic agents can be evaluated using specific VAS. Bowdle Psychotomimetic Effects Scores consisted of thirteen 10 cm visual analogue lines ranging from 0 (‘not at all’) to 100 mm (‘extremely’), addressing various abnormal states of mind. The Bowdle VAS was administered electronically and took approximately 2 minutes to complete. | Cortex, prefrontal cortex, amygdala |
Pharmaco-electroencephalography (pEEG) | CNS actions of pharmacological substances.70 Resting-state pEEG recordings were conducted on subjects with open and closed eyes for 5 minutes each. Subjects were instructed to avoid staring, head and eye movements, and suppress eye blinks while facing a featureless wall. pEEG was recorded using a 40-channel system, with electrode placement following the international 10–20 system. Ocular artifacts were detected using vertical and horizontal pEEG recordings. The recorded signals were processed by applying filters, calculating power spectrum density, and analyzing specific electrode sites of interest. | All brain regions |
CNS, central nervous system; pEEG, pharmaco-electroencephalography; VAS, visual analogue scale; VVLT, Visual Verbal Learning Test.
In addition in Part C, subjects were also provided with a smartwatch (Withings, Withings Steel HR France) to measure heart rate during night and day, and polysomnography (PSG) (Trackit Mk3, Lifelines, USA) was used to measure the effect on sleep, including number of awakenings, R latency, sleep latency, time in bed, total sleep time, duration of N1, N2, N3 and R sleep, and sleep efficiency.
Safety was evaluated throughout the study by routine assessments of adverse events, blood levels of potassium and glucose, ECG (Marquette 2000 or 5500, GE Healthcare, USA), and vital signs including supine blood pressure and pulse rate, respiratory rate, and temperature. Plasma drug concentrations were measured using LC/MS-MS methods and drug pharmacokinetics were evaluated using standard non-compartmental analyses.
Statistical analysis
This was an exploratory study and therefore, we did not perform a formal sample size estimation. The sample size was based on practical considerations and is standard for this type of study.43 The study collected data on pharmacodynamic parameters for each subject, visit, and time point of treatment. Mixed model analysis of covariance (ANCOVA) and analysis of variance (ANOVA) were used to determine whether there were significant treatment effects on pharmacodynamic parameters, heart rate, and blood pressure. The models included factors such as treatment, time, and period, as well as random factors such as subject. Also, the Kenward-Roger approximation and the restricted maximum likelihood method was used to estimate model parameters and reported treatment effects with the estimated difference (ED) compared to placebo of the least squared means (LSM), 95% confidence intervals (95% CI), and p-values. P-values <0.05 were assessed as statistically significant. These ED, LSM and p-values will be reported in the results section. In Part A, specific contrasts were also calculated to compare the effects of specific treatments to a placebo. Parameters were initially analyzed without transformation, but if the data suggest otherwise, logtransformation was applied. Residual Q-Q plots were produced to check the assumption of normality of the error term in the mixed effects models. This was done by visual inspection and the Shapiro-Wilk test statistic.
Pharmacodynamic, pharmacokinetic and safety analyses were performed with SAS 9.4 for Windows (SAS Institute Inc. Cary, NC, USA, 2013). All available data were included in the analyses with no imputation of missing data and no correction for multiplicity. Data from Part A was evaluated prior to the initiation of the other parts of the study to inform which β-AR drug to take forward into Part B and Part C.
RESULTS
Disposition and demographics
A total of 79 HVs were screened for participation in the first 2 parts of this study, of whom 35 were enrolled. A total of 35 HVs received at least 1 dose of the study drug, and 27 completed the scheduled dosing and assessments. In Part C, 23 PD patients were screened for participation, of whom 13 were enrolled. All 12 PD patients were administered all doses during the study and completed the study. The numbers of participants who were screened, enrolled, and withdrawn/completed each of the 3 parts of the study is summarized in Fig. 1. The demographic characteristics of participants enrolled in this study are summarized in Table 2. No important differences were noted between treatment groups within each part, except for a difference in age groups as expected from the enrolment criteria between HVs in Parts A and B, and PD-patients in Part C.
Table 2
Demographic characteristics of all participants who received study drug
HVs | PD: multiple dose | ||||
Part A: | Part B: | Part C: | Part C: | Part C: | |
single dose | multiple dose | overall | Clenbuterol | Placebo | |
N | 19 | 16 | 12 | 8 | 4 |
Age (y) | 49.0 (4.7) | 51.6 (6.7) | 63.4 (6.6) | 64.1 (6.1) | 62 (8.3) |
Height (cm) | 178.47 (9.03) | 177.74 (9.98) | 177.93 (9.57) | 180.55 (5.59) | 172.68 (14.43) |
Weight (kg) | 81.52 (14.84) | 84.53 (10.97) | 77.68 (8.07) | 78.656 (6.753) | 75.73 (11.16) |
BMI (kg/m2) | 25.46 (3.35) | 26.84 (3.55) | 24.56 (1.98) | 24.16 (2.34) | 25.35 (0.58) |
Gender, male (percentage) | 68.4 | 56.3 | 83.3 | 100 | 50 |
MMSE | n.a. | n.a. | 29.3 (0.8) | 29.2 (0.9) | 29.3 (0.77) |
Hoehn and Yahr | n.a. | n.a. | 1.7 (0.9) | 1.8 (0.7) | 1.5 (1.3) |
BMI, body mass index; HVs, healthy volunteers; MMSE, Mini-Mental State Examination; n.a., not applicable; PD, Parkinson’s disease. Results are either presented as mean (standard deviation), or percentages.
Fig. 1
Flowchart showing disposition of all consenting participants.

Pharmacodynamic effects
Part A
In Part A, administration of a single dose of clenbuterol to HVs significantly increased the number of correctly recalled words during the first immediate recall trial test (ED of the LSM 1.30 [95% CI 0.10; 2.60], N = 15, p = 0.04, Fig. 2), increased the number of correctly recalled positive words during the delayed recall test (ED of the LSM 8.79 [95% CI 0.17; 17.41], p = 0.05) increased the performance in the adaptive tracking test during the first 4 h (ED of the LSM 2.23 [95% CI 0.51; 3.95], p = 0.01), increased the pupil/iris ratio in both the left eye (ED of the LSM 0.02 [95% CI 0.01; 0.04], p = 0.01) and the right eye (ED of the LSM 0.02 [95% CI 0.01; 0.04], p = 0.01), and decreased the smooth pursuit parameter of saccadic eye movements albeit non-significantly (ED of the LSM –0.97% [95% CI –3.10; 1.15]). In these participants, responses following administration of a single dose of salbutamol were qualitatively similar to clenbuterol, although a significant effect was observed only in the immediate word recall test (ED of the LSM 1.3 [95% CI 0.1; 2.6], p = 0.03, Fig. 2). Conversely, among the subset of participants (N = 10) who received 60 mg pindolol, there was a tendency towards worse performance in the cognitive tasks, while the pupil/iris ratio was significantly decreased in the left eye (ED of the LSM –0.03 [95% CI –0.05; – 0.02], p < 0.01), and the right eye (ED of the LSM – 0.03 [95% CI – 0.05; – 0.02], p < 0.01). Also, saccadic eye movement during smooth pursuit was significantly decreased (ED of the LSM –3.19 [95% CI – 5.68; – 0.71], p = 0.01). Data for the 5 participants who received 20 mg pindolol are not presented but generally consistent with the observations with 60 mg pindolol. In the presence of each of the three active drugs administered in Part A, there was a decrease in the number of correct responses in incongruent trials compared to placebo which was statistically significant with clenbuterol (ED of the LSM –0.2 [95% CI – 0.40; – 0.00], p = 0.04).
Fig. 2
Effects of single doses of clenbuterol, salbutamol and pindolol on immediate word recall, delayed word recall, adaptive tracking and pupil/iris ratio in part A. LS, Least Square; CI, Confidence Interval. Data for the cohort of healthy volunteers in Part A who received a single dose of placebo, clenbuterol (160μg, N = 16), salbutamol (32 mg, N = 17) and pindolol (60 mg, N = 10) in a randomized sequence separated by an approximately 7-day washout. LS mean (95% CI) differences between drug and placebo are plotted for repeat observations over the first 4 h after dosing on Day 1 for immediate word recall (trial 1, A), delayed word recall (B), adaptive tracking (C) and left pupil-to-iris ratio (D).
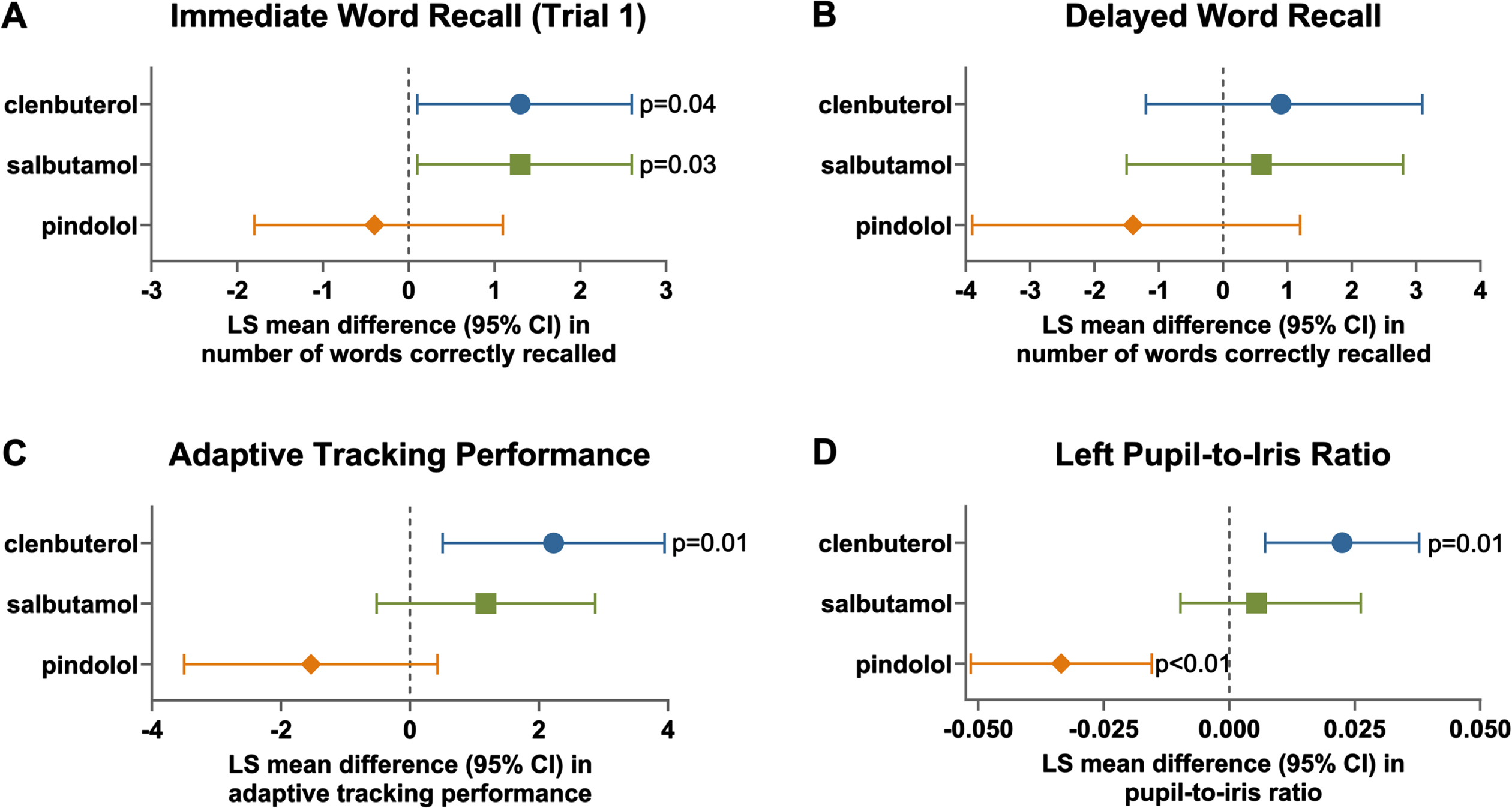
Part B
As with the single-dose assessments, a general, albeit non-significant, improvement in visual verbal learning tests was observed in HVs who received once-daily oral clenbuterol hydrochloride for 7 days in Part B (20μg on Day 1, 40μg on Day 2 and 80μg on Day 3 to Day 7, Fig. 3A). In addition, a significant improvement in performance was observed in the adaptive tracking test over the first 4 h after dosing on Day 7 (ED of the LSM 1.58% [95% CI 0.22; 2.94], p = 0.03, Fig. 3B), compared to placebo. Also, administration of clenbuterol significantly increased parieto-occipital delta-power with eyes open (ED of the LSM 11.10% uV2 [95% CI 5.20%; 17.30%], p = 0.01) and closed (ED of the LSM 9.50% uV2 [95% CI 3.20%; 16.10%], p = 0.01), compared to placebo.
Fig. 3
Effects of repeat, once-daily oral doses of clenbuterol (80μg) in healthy subjects in immediate word recall (A) and adaptive tracking (B) in part B. LS, Least Square; CI, Confidence Interval. Data for the cohort of 14-15 healthy volunteers in Part B who received placebo and clenbuterol (up-titrated over the dosing period from 20μg on Day 1, 40μg on Day 2 and 80μg on Days 3–7) in a 7-day crossover are presented. LS mean (95% CI) differences between clenbuterol and placebo are plotted for repeat observations at 1, 2, and 8 h after dosing on Days 1, 4, and 7 for the number of correct responses for the immediate word recall (trial 1), delayed word recall and delayed word recognition (B), and repeat observations over the first 4 h after dosing on Days 1, 4, and 7 for adaptive tracking (B).
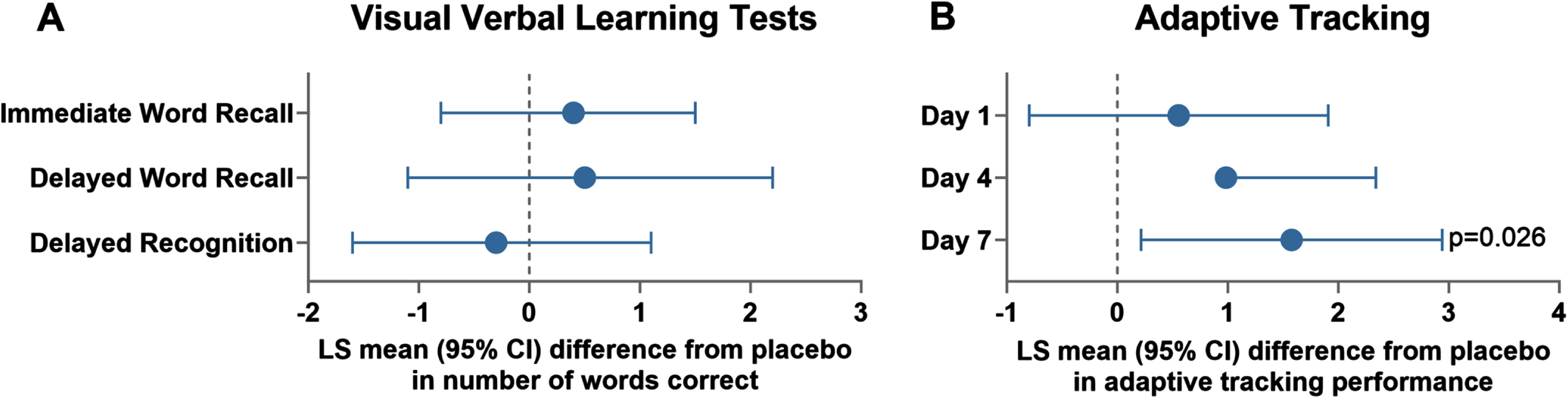
Part C
Unlike in the larger, crossover cohorts in Parts A and B of this study, evaluation of the effects of treatment in participants with PD enrolled in Part C of the study was significantly confounded by the small sample size, especially in the placebo group (N = 4) of this parallel-group assessment. Nonetheless, a tendency toward increased recall and recognition of words was observed (ED of the LSM for number of words correct over all test days in immediate word recall [trial 1] 1.10 [95% CI – 2.80; 5.10], and delayed word recall 0.90 [95% CI – 3.30; 5.10]) albeit without attaining statistical significance. Administration of clenbuterol did not have significant effects on EEG frequency bands. Polysomnography evaluations in Part C showed that administration of clenbuterol in participants with PD significantly decreased the time before waking up after sleep onset compared to placebo (ED of the LSM – 68.59 min [95% CI – 135.55; –1.635], p = 0.05).
Safety and pharmacokinetics
Clenbuterol, salbutamol and pindolol have been approved for use in humans for several decades, and the safety profile observed for these drugs was similar to previously reported experience. The treatment emergent AEs that were reported most frequently while receiving the β 2-AR acting drugs, salbutamol or clenbuterol, were typical for the drug class: headache, tremor, palpitations, tachycardia, fatigue, nausea, and dizziness.44 The most commonly reported adverse events with the β 2-AR antagonist/β 1-AR partial agonist, pindolol, were headache, dizziness and nausea.
Of the 43 HVs or participants with PD who were enrolled and received active drug, 5 HVs discontinued due to AEs, most frequently dizziness, nausea, vomiting, cold sweat, headache, paraesthesia, muscular weakness, palpitations, somnolence, tremor, and/or fatigue while on salbutamol or clenbuterol. One HV withdrew from study due to adverse events of syncope, somnolence, nausea, cold sweat while receiving pindolol. All withdrawn HVs recovered from the adverse events within seven days without intervention. No deaths or serious AEs were observed. Paracetamol was occasionally used as concomitant medication for headache or malaise.
Also, in keeping with the β 2-AR agonist mechanism of action, increases in supine heart rate were observed following repeat administration of 80μg clenbuterol to HVs over multiple measurements during Days 4 through 7 compared to placebo (ED of the LSM 11.50 bpm [95% CI 8.00; 14.90], p = 0.01), with a modest increase in systolic blood pressure compared to placebo (ED of the LSM 1.80 mmHg [95% CI 0.40; 3.20], p = 0.02) and no effect on diastolic blood pressure (ED of the LSM –0.2 mmHg [95% CI – 2.7; 2.2], p = 0.83). Similarly, significant increases in supine heart rate were observed with clenbuterol compared with placebo in participants with PD during waking hours (ED of the LSM 13.30 bpm [95% CI 8.20; 18.40], p = 0.01 on Day 7) as well as during sleep (ED of the LSM in the median percentile of 11.10 bpm [95% CI 7.05; 15.15], p = 0.01, across all dosing days), without significant changes in supine systolic (ED of the LSM –4.4 mmHg [95% CI – 16.9; 8.1], p = 0.45) or diastolic blood pressure (ED of the LSM –3.7 mmHg [95% CI – 10.7; 3.3], p = 0.27).
Plasma drug concentrations were measured by LC/MS-MS and submitted to non-compartmental analyses for determination of pharmacokinetic parameters, including the observed time to maximal plasma concentration (Tmax) and half-file. The observations generally conformed with published values. After administration of 160μg clenbuterol hydrochloride, the Tmax was 3 h and longer, and half-life longer than could be estimated based on the amount of samples in the study (prior studies report >30 h half-lives for clenbuterol).45 After administration of 32 mg salbutamol, Tmax was 6-7 h and half-life was 1-2 h. After administration of 60 mg pindolol, Tmax was 1 h, and half-life was 4 h.
DISCUSSION
Degeneration of the LC neurons likely contributes to the loss of cognitive function in diseases such as PD46 and AD.47 Although a role of the LC in attention, learning and memory has been known for over 50 years, the specific adrenergic receptor(s) that produce these effects are not well-established.48 This study sought to evaluate the effects of two potent β 2-AR acting drugs (clenbuterol and salbutamol) and a non-selective β 1-AR agonist/β 2-AR antagonist (pindolol) on CNS measures with the aim to select a drug for future trials investigating the potential to reduce cognitive dysfunction in PD. In this study, we observed enhancements in working memory, attention, visuomotor coordination and pupil/iris ratios among HVs with the CNS penetrating β 2-AR acting drug, clenbuterol. Responses to salbutamol were similar to clenbuterol, but weaker in magnitude, presumably due to its lower CNS absorption, whole the effects were not observed for the β1-AR agonist/β2-AR antagonist, pindolol. These findings suggest that centrally acting β2-AR agonists may improve cognitive function in individuals affected by neurodegenerative diseases like PD. In addition, we demonstrated the safety of and single and repeat doses of clenbuterol in HVs and PD patients.
The observations in the present study are in line with a prior study in approximately 40 HVs, in which salbutamol produced pro-cognitive effects on emotional memory and attention (unpublished data, NCT01957293).23 The observations are additionally consistent with the pro-cognitive effects of direct stimulation of β-Ars using clenbuterol and indirect stimulation using the norepinephrine reuptake inhibitor, atomoxetine, in a rat model of visuospatial attention.49 However, this is the first study in humans to compare different β-AR properties, including β 1- vs. β 2 selectivity, and CNS absorption to enable identification of the key properties for a potential future drug for treating cognitive decline. While significant improvements in immediate word recall were observed with both clenbuterol and salbutamol, the improvements in other cognitive tasks that were observed with clenbuterol but not salbutamol suggest that penetration into the CNS may contribute to the broader range of observed effects. The contrasting tendency of pindolol to worsen performance in word recall and adaptive tracking lend further support to the hypothesized pro-cognitive role of β 2-AR activation, as pindolol is an antagonist at this receptor and may be blocking endogenous stimulation.50 However, whether the latter effect may also be the result of partial agonism of β 1-AR, or some other nonselective that cannot be concluded definitively from the present study.
The data from Part A suggest that CNS-penetrating β 2-AR acting drugs may mediate the effects of LC innervation on memory and alertness. This informed the decision to evaluate clenbuterol in Part B and Part C of this study. The observed effects of a single dose of clenbuterol in Part A were corroborated following once-daily administration of clenbuterol to HVs for 1 week in Part B. Specifically, there was a trend towards improved performance in the immediate and delayed word recall tests, and statistically significant improvement in performance in the adaptive tracking task. Similar evaluation of the effects of clenbuterol in patients with PD in Part C was confounded by sample size, especially in the placebo comparator arm (N = 4). In addition, motor deficits in the PD population may impact measurement of response, especially in tasks with greater motor involvement such as the adaptive tracking task. Since cognitive impairment varies not only with disease severity but also between different neurodegenerative diseases,51 presumably reflecting the different brain regions, pathways and cell types involved, we cannot expect a single drug target to rescue all cognitive domains. In the case of clenbuterol, some of the improvements in performance of cognitive tasks may reflect effects on attention and alertness, while non-neuronal changes in metabolism, inflammation and perfusion mediated by β2-adrenergic receptors expressed on astrocytes and microglial may provide more sustained benefit.52
In Part A of this study, the two β 2-AR agonists, clenbuterol and salbutamol, resulted in qualitatively similar effects on cognition in HVs, including significant improvements in the immediate verbal recall test, and a tendency toward improved performance in delayed verbal recall. Performance in visual verbal tests has been correlated with short-term memory and is known to be improved after administration of caffeine.53–55 In addition, clenbuterol significantly improved performance in the adaptive tracking task. The adaptive tracking test is a marker of drug effects on coordination and vigilance.56,57 The performance during the adaptive tracking test is worsened by sedatives such as diazepam and temazepam,58,59 and increased by psychoactive drugs such as meta-chlorophenyl piperazine.60 In keeping with its lower absorption into the CNS, the observed effects of salbutamol in this study were qualitatively similar but typically more modest compared with those of clenbuterol.36 In contrast, the effects of pindolol were directionally different from clenbuterol and salbutamol, including worsening of performance in the immediate and delayed verbal recall tests as well as in adaptive tracking.
When administered in single or repeat doses, the peripheral effects observed for clenbuterol were similar to those often reported for the β 2-AR agonist drug class.61,62 The most frequently reported AEs by HVs were palpitations and tremor with increases in mean supine heart rate of more than 10 bpm that led to 5 withdrawals and that likely impacted the evaluations of cognition undertaken in this study. There were no withdrawals of PD-patients. Chronic dosing with clenbuterol, as would be anticipated if used for treatment of cognitive decline due to PD or AD, will necessitate better control of these effects in the periphery, e.g., by using strategic co-administration of a selective β 2-AR antagonist that achieves minimal penetration into the CNS. However, this needs to be confirmed in future trials.
This study had some limitations. Firstly, this study involved a small number of PD-patients, which may not fully represent the diverse patient populations that will ultimately use the drug. Consequently, the findings may not accurately predict how clenbuterol will affect patients with various medical conditions or demographics. Secondly, this study assessed the short-term effects of the treatment. Similar effects on the long term will need to be corroborated in a follow-up study. Additionally, the controlled environment of this study may not fully reflect real-world conditions, which is inherent to an early phase clinical study. Factors such as patient compliance, concomitant medications, and environmental influences can affect drug outcomesdifferently.
Taken together, the observations from this study identify the β 2-AR as a candidate receptor that may result in pro-cognitive effects. Moreover, the superior effects of the CNS-penetrating drug, clenbuterol, compared with salbutamol reveal the potential utility of selective, CNS-penetrant β 2-AR agonism, especially if the agonistic effect can be limited to the brain. These data support further investigation of the effects of clenbuterol on measures of cognition and alertness in patients with a neurodegenerative disease such as PD, especially under conditions where the peripheral effects of β 2-AR agonism are controlled.
ACKNOWLEDGMENTS
The authors would like to thank the study participants.
FUNDING
The study was funded by CuraSen Therapeutics, San Carlos, CA, USA (2019 to 2020).
CONFLICT OF INTEREST
This study was funded by CuraSen Therapeutics, Inc. PE, LB, SP, LM, BvK, EvB, EK, PK, GJ are investigators, statisticians and site personnel at the clinical investigative site for this study; EB is a paid consultant to CuraSen; RM, AF and GV are employees of CuraSen Therapeutics, Inc.
DATA AVAILABILITY
The data supporting the findings of this study are available on request from the corresponding author.
REFERENCES
1. | Routledge C and Marsden CA . Adrenaline in the CNS: in vivo evidence for a functional pathway innervating the hypothalamus. Neuropharmacology (1987) ; 26: : 823–830. |
2. | Cash R , Ruberg M , Raisman R , et al. Adrenergic receptors in Parkinson’s disease. Brain Res (1984) ; 322: : 269–275. |
3. | Beitz JM . Parkinson’s disease: a review. Front Biosci (Schol Ed) (2014) ; 6: : 65–74. |
4. | Bekdash RA . The cholinergic system, the adrenergic system and the neuropathology of Alzheimer’s disease. Int J Mol Sci (2021) ; 22: : 1273. |
5. | Russo-Neustadt A and Cotman CW . Adrenergic receptors in Alzheimer’s disease brain: selective increases in the cerebella of aggressive patients. J Neurosci (1997) ; 17: : 5573–5580. |
6. | Marien MR , Colpaert FC and Rosenquist AC . Noradrenergic mechanisms in neurodegenerative diseases: a theory. Brain Res Brain Res Rev (2004) ; 45: : 38–78. |
7. | Lu’o’ng KV and Nguyen LT . The role of beta-adrenergic receptor blockers in Alzheimer’s disease: potential genetic and cellular signaling mechanisms. Am J Alzheimers Dis Other Demen (2013) ; 28: : 427–439. |
8. | Kalia LV and Lang AE . Parkinson’s disease. Lancet (2015) ; 386: : 896–912. |
9. | Khan NL , Graham E , Critchley P , et al. Parkin disease: a phenotypic study of a large case series. Brain (2003) ; 126: : 1279–1292. |
10. | Truong DD , Bhidayasiri R and Wolters E . Management of non-motor symptoms in advanced Parkinson disease. J Neurol Sci (2008) ; 266: : 216–228. |
11. | Sauerbier A , Jenner P , Todorova A , et al. Non motor subtypes and Parkinson’s disease. Parkinsonism Relat Disord (2016) ; 22: (Suppl 1), S41–S46. |
12. | Zhang T-M , Yu S-Y , Guo P , et al. Nonmotor symptoms in patients with Parkinson disease: A cross-sectional observational study. Medicine (2016) ; 95: : e5400. |
13. | Aarsland D , Londos E and Ballard C . Parkinson’s disease dementia and dementia with Lewy bodies: different aspects of one entity. Int Psychogeriatr (2009) ; 21: : 216–219. |
14. | Kehagia AA , Barker RA and Robbins TW . Neuropsychological and clinical heterogeneity of cognitive impairment and dementia in patients with Parkinson’s disease. Lancet Neurol (2010) ; 9: : 1200–1213. |
15. | Chaudhuri KR . Nocturnal symptom complex in PD and its management. Neurology (2003) ; 61: : S17–S23. |
16. | Havlikova E , Rosenberger J , Nagyova I , et al. Impact of fatigue on quality of life in patients with Parkinson’s disease. Eur J Neurol (2008) ; 15: : 475–480. |
17. | Lou J-S . Physical and mental fatigue in Parkinson’s disease: epidemiology, pathophysiology and treatment. Drugs Aging (2009) ; 26: : 195–208. |
18. | Abbott RD , Ross GW , White LR , et al. Excessive daytime sleepiness and subsequent development of Parkinson disease. Neurology (2005) ; 65: : 1442–1446. |
19. | Ponsen MM , Stoffers D , Booij J , et al. Idiopathic hyposmia as a preclinical sign of Parkinson’s disease. Ann Neurol (2004) ; 56: : 173–181. |
20. | Vermeiren Y and De Deyn PP . Targeting the norepinephrinergic system in Parkinson’s disease and related disorders: The locus coeruleus story. Neurochem Int (2017) ; 102: : 22–32. |
21. | Braak H and Del Tredici K . Neuropathological staging of brain pathology in sporadic Parkinson’s disease: separating the wheat from the chaff. J Parkinsons Dis (2017) ; 7: : S71–S85. |
22. | Nahimi A , Sommerauer M , Kinnerup MB , et al. Noradrenergic deficits in Parkinson disease imaged with (11)C-MeNER. J Nucl Med (2018) ; 59: : 659–664. |
23. | Hopfner F , Höglinger GU , Kuhlenbäumer G , et al. β-adrenoreceptors and the risk of Parkinson’s disease. Lancet Neurol (2020) ; 19: : 247–254. |
24. | Aaseth J , Dusek P and Roos PM . Prevention of progression in Parkinson’s disease. Biometals (2018) ; 31: : 737–747. |
25. | Gronich N , Abernethy DR , Auriel E , et al. β2-adrenoceptor agonists and antagonists and risk of Parkinson’s disease. Mov Disord (2018) ; 33: : 1465–1471. |
26. | Magistrelli L and Comi C . Beta2-adrenoceptor agonists in Parkinson’s disease and other synucleinopathies. J Neuroimmune Pharmacol (2019) ; 15: : 74–81. |
27. | Mittal S , Bjørnevik K , Im DS , et al. β2-Adrenoreceptor is a regulator of the α-synuclein gene driving risk of Parkinson’s disease. Science (2017) ; 357: : 891–898. |
28. | Searles Nielsen S , Gross A , Camacho-Soto A , et al. β2-adrenoreceptor medications and risk of Parkinson disease. Ann Neurol (2018) ; 84: : 683–693. |
29. | Chalermpalanupap T , Kinkead B , Hu WT , et al. Targeting norepinephrine in mild cognitive impairment and Alzheimer’s disease. Alzheimers Res Ther (2013) ; 5: : 21. |
30. | Coutellier L , Ardestani PM and Shamloo M . β1-adrenergic receptor activation enhances memory in Alzheimer’s disease model. Ann Clin Transl Neurol (2014) ; 1: : 348–360. |
31. | Colangelo AM , Johnson PF and Mocchetti I . β-Adrenergic receptor-induced activation of nerve growth factor gene transcription in rat cerebral cortex involves CCAAT/enhancer-binding protein δ. Proc Natl Acad Sci U S A (1998) ; 95: : 10920–10925. |
32. | Chai G-S , Wang Y-Y , Zhu D , et al. Activation of β(2)-adrenergic receptor promotes dendrite ramification and spine generation in APP/PS1 mice. Neurosci Lett (2017) ; 636: : 158–164. |
33. | Chai G-S , Wang Y-Y , Yasheng A , et al. Beta 2-adrenergic receptor activation enhances neurogenesis in Alzheimer’s disease mice. Neural Regen Res (2016) ; 11: : 1617–1624. |
34. | McAinsh J and Cruickshank JM . Beta-blockers and central nervous system side effects. Pharmacol Ther (1990) ; 46: : 163–197. |
35. | Joseph SS , Lynham JA , Molenaar P , et al. Intrinsic sympathomimetic activity of (-)-pindolol mediated through a (-)-propranolol-resistant site of the beta1-adrenoceptor in human atrium and recombinant receptors. Naunyn Schmiedebergs Arch Pharmacol (2003) ; 368: : 496–503. |
36. | Caccia S and Fong MH . Kinetics and distribution of the beta-adrenergic agonist salbutamol in rat brain. J Pharm Pharmacol (1984) ; 36: : 200–202. |
37. | Postuma RB , Berg D , Stern M , et al. MDS clinical diagnostic criteria for Parkinson’s disease. Mov Disord (2015) ; 30: : 1591–1601. |
38. | Hoehn MM and Yahr MD . Parkinsonism: onset, progression and mortality. Neurology (1967) ; 17: : 427–442. |
39. | Folstein MF , Folstein SE and McHugh PR . ‘Mini-mental state’. A practical method for grading the cognitive state of patients for the clinician. J Psychiatr Res (1975) ; 12: : 189–198. |
40. | Food and Drug Administration. VENTOLIN HFA (albuterol sulfate) Inhalation Aerosol, www.fda.gov/medwatch. (2014). |
41. | Collins IS . Visken. Med J Aust (1972) ; 1: : 498. |
42. | Bloch R and Denis JM . Spiropenten. Angew Chem (1980) ; 92: : 969–970. |
43. | Groeneveld GJ , Hay JL , Van Gerven JM . Measuring blood– brain barrier penetration using the NeuroCart, a CNS test battery. Drug Discov Today Technol (2016) ; 20: : 27–34. |
44. | Highlights of Prescribing Information - Ventolin HFA, dailymed https://dailymed.nlm.nih.gov/dailymed/fda/fdaDrugXsl.cfm?setid=938ab43d-bf4c-477f-b918-aabe738d562b&type=display (2021). |
45. | Yamamoto I , Iwata K and Nakashima M . Pharmacokinetics of plasma and urine clenbuterol in man, rat, and rabbit. J Pharmacobiodyn (1985) ; 8: : 385–391. |
46. | Zhou C , Guo T , Bai X , et al. Locus coeruleus degeneration is associated with disorganized functional topology in Parkinson’s disease. Neuroimage Clin (2021) ; 32: : 102873. |
47. | Chen Y , Chen T and Hou R . Locus coeruleus in the pathogenesis of Alzheimer’s disease: A systematic review. Alzheimers Dement (N Y) (2022) ; 8: : e12257. |
48. | Anlezark GM , Crow TJ and Greenway AP . Impaired learning and decreased cortical norepinephrine after bilateral locus coeruleus lesions. Science (1973) ; 181: : 682–684. |
49. | Pattij T , Schetters D , Schoffelmeer A , et al. On the improvement of inhibitory response control and visuospatial attention by indirect and direct adrenoceptor agonists. Psychopharmacology (Berl) (2011) ; 219: : 327–340. |
50. | Simon P , Lecrubier Y , Jouvent R , et al. Experimental and clinical evidence of the antidepressant effect of a beta-adrenergic stimulant. Psychol Med (1978) ; 8: : 335–338. |
51. | Millan MJ , Agid Y , Brüne M , et al. Cognitive dysfunction in psychiatric disorders: characteristics, causes and the quest for improved therapy. Nat Rev Drug Discov (2012) ; 11: : 141–168. |
52. | Matt RA , Martin RS , Evans AK , et al. Locus coeruleus and noradrenergic pharmacology in neurodegenerative disease. In Handbook of Experimental Pharmacology. Berlin, Heidelberg: Springer. https://doi.org/10.1007/164_2023_677. |
53. | Goulart AA , Lucatelli A , Silveira PSP , et al. Comparison of digital games as a cognitive function assessment tool for current standardized neuropsychological tests. Braz J Anesthesiol (2022) ; 72: : 13–20. |
54. | Chen X , De Haas S , De Kam M , et al. An overview of the CNS-pharmacodynamic profiles of nonselective and selective GABA agonists. Adv Pharmacol Sci. 2012. |
55. | Schmitt JAJ . Serotonin, caffeine and cognition: psychopharmacological studies in human cognitive functioning. PhD Thesis, Maastricht University, 2001. |
56. | Cohen AF , Ashby L , Crowley D , et al. Lamotrigine (BW430C), a potential anticonvulsant. Effects on the central nervous system in comparison with phenytoin and diazepam. Br J Clin Pharmacol (1985) ; 20: : 619–629. |
57. | Nicholson AN . Performance studies with diazepam and its hydroxylated metabolites. Br J Clin Pharmacol (1979) ; 8: : 39S–42S. |
58. | van Steveninck AL , Schoemaker HC , Pieters MS , et al. A comparison of the sensitivities of adaptive tracking, eye movement analysis and visual analog lines to the effects of incremental doses of temazepam in healthy volunteers. Clin Pharmacol Ther (1991) ; 50: : 172–180. |
59. | van Steveninck AL . Methods of assessment of central nervous system effects of drugs in man. PhD Thesis, State University Leiden 1993–. |
60. | Gijsman HJ , Van Gerven JM , Tieleman MC , et al. Pharmacokinetic and pharmacodynamic profile of oral and intravenous meta-chlorophenylpiperazine in healthy volunteers. J Clin Psychopharmacol (1998) ; 18: : 289–295. |
61. | Löfdahl CG , Sigvaldasson A , Skoogh BE , et al. Broxaterol, a new beta 2-adrenoceptor agonist compared to salbutamol in asthmatics, oral and inhalation treatment. Respiration (1989) ; 55: (Suppl 2), 15–19. |
62. | Lötvall J and Ankerst J . Long duration of airway but not systemic effects of inhaled formoterol in asthmatic patients. Respir Med (2008) ; 102: : 449–456. |
63. | Baloh RW , Sills AW , Kumley WE , et al. Quantitative measurement of saccade amplitude, duration, and velocity. Neurology (1975) ; 25: : 1065–1070. |
64. | Borland RG and Nicholson AN . Visual motor co-ordination and dynamic visual acuity. Br J Clin Pharmacol (1984) ; 18: (Suppl 1): 69S–72S. |
65. | Wright BM . A simple mechanical ataxia-meter. J Physiol (1971) ; 218: (Suppl): 27p–28p. |
66. | Bond A and Lader M . The use of analogue scales in rating subjective feelings. Br J Med Psychol (1974) ; 47: : 211–218. |
67. | Harborne GC , Watson FL , Healy DT , et al. The effects of sub-anaesthetic doses of ketamine on memory, cognitive performance and subjective experience in healthy volunteers. J Psychopharmacol (1996) ; 10: : 134–140. |
68. | Hartvig P , Valtysson J , Lindner KJ , et al. Central nervous system effects of subdissociative doses of (S)-ketamine are related to plasma and brain concentrations measured with positron emission tomography in healthy volunteers. Clin Pharmacol Ther (1995) ; 58: : 165–173. |
69. | van Steveninck AL , Gieschke R , Schoemaker HC , et al. Pharmacodynamic interactions of diazepam and intravenous alcohol at pseudo steady state. Psychopharmacology (Berl) (1993) ; 110: : 471–478. |
70. | Jobert M , Wilson FJ , Ruigt GSF , et al. Guidelines for the recording and evaluation of pharmaco-EEG data in man: the International Pharmaco-EEG Society (IPEG). Neuropsychobiology (2012) ; 66: : 201–220. |