Occupational Pesticide Exposure in Parkinson’s Disease Related to GBA and LRRK2 Variants
Abstract
Background:
The penetrance of common genetic risk variants for Parkinson’s disease (PD) is low. Pesticide exposure increases PD risk, but how exposure affects penetrance is not well understood.
Objective:
To determine the relationship between occupational pesticide exposure and PD in people with LRRK2 and GBA risk variants.
Methods:
Participants of the Parkinson’s Progression Markers Initiative (PPMI) with a LRRK2-G2019 S or GBA risk variant provided information about occupational pesticide exposure. We compared exposure in carriers with and without PD. Among carriers with PD, we used Cox proportional hazard models to compare time-to impairment in balance, cognition, and activities of daily living (ADLs) between participants with and without prior occupational pesticide exposure.
Results:
378 participants with a risk variant provided exposure information; 176 with LRRK2-G2019 S (54 with and 122 without PD) and 202 with GBA variants (47 with and 155 without PD). Twenty-six participants reported pesticide exposure. People with a GBA variant and occupational pesticide exposure had much higher odds of PD (aOR: 5.4, 95% CI 1.7–18.5, p < 0.01). People with a LRRK2 variant and a history of occupational pesticide exposure had non-significantly elevated odds of PD (aOR 1.3, 95% CI 0.4–4.6, p = 0.7). Among those with PD, pesticide exposure was associated with a higher risk of balance problems and cognitive impairment in LRRK2-PD and functional impairment in GBA-PD, although associations were not statistically significant.
Conclusions:
Occupational pesticide exposure may increase penetrance of GBA-PD and may be associated with faster symptom progression. Further studies in larger cohorts are necessary.
INTRODUCTION
Variants in LRRK2 and GBA represent the two most common genotypes that increase Parkinson’s disease (PD) risk, yet the penetrance of these variants is low and variable across populations [1, 2]. Factors that modify penetrance are not well understood. Pesticide exposure, a risk factor for PD development [3], increases oxidative stress and mitochondrial damage to the striatum [4], and may exacerbate pathogenic mechanisms of PD-related genetic risk variants [5]. However, how pesticide exposure relates to risk in people with PD and LRRK2 or GBA variants has not been well studied.
We therefore collected information on occupational pesticide exposure from people with either a pathogenic LRRK2 or GBA variant with or without PD (i.e., non-manifesting carriers [NMC]) in the Parkinson Progression Marker Initiative (PPMI) cohort. We further evaluated whether pesticide exposure was associated with progression of balance problems, cognitive impairment, or impairment in activities of daily living (ADLs) within these groups.
MATERIALS AND METHODS
Study populations
PPMI is an ongoing longitudinal, observational study that includes several cohorts who undergo systematic evaluation of motor and non-motor symptoms, neurologic exams, and biomarker collection occurring every 6 to 12 months [6]. In this study, we evaluated PPMI participants who had provided separate consent to be contacted by email, telephone, or postal mail, in parallel to the longitudinal in-clinic PPMI protocol. Participants who (i) had an email address and (ii) were English speaking were invited to complete the PD Risk Factor Questionnaire (PD-RFQ), which asks about lifetime exposure to several environmental and lifestyle factors related to PD, including occupational pesticide use (Appendix 1) [7]. Participants completed the questionnaire online and data were cleaned using email or telephone queries to participants.
Recruitment of participants occurred through participating PPMI sites and a centralized recruitment initiative, described in detail previously [8, 9]. People without a diagnosis of PD were eligible for genetic screening to determine whether they were non-manifesting carriers (NMC) if they were at least 45 years old and either (a) were of Ashkenazi Jewish ancestry and had a first degree relative with PD or (b) had a first degree relative with a known genetic risk variant. People with PD and a confirmed LRRK2 or GBA variant were enrolled if they were within 7 years of diagnosis. All PPMI participants subsequently had whole exome or whole genome sequencing, which was used to identify pathogenic variant carriers in the broader PPMI Cohort. For this study, we included PPMI participants with LRRK2 G2019S with PD (LRRK2-PD) or without PD (LRRK2-NMC) and people with a GBA variant considered pathogenic by the PPMI Genetics Core with PD (GBA-PD) or without PD (GBA-NMC), which included N370S, L444P, 84GG, IVS2 + 1 G>A, and R120W. We separately evaluated participants with both a GBA and a LRRK2 variant.
Determination of exposure
The PD-RFQ was used to determine history of occupational exposure, the duration of exposure, and the specific agent to which people were exposed, if known [7]. Study personnel contacted participants to resolve incomplete or invalid responses. A participant was considered exposed if the exposure occurred prior to diagnosis of PD or, for NMC, prior to enrollment in PPMI. We further stratified analyses according to duration of exposure among those exposed (≤5 years, > 5 years) and use of protective gloves. A lifelong history of tobacco use was also collected; regular smoking was defined as at least one cigarette daily for ≥6 months.
Determination of PD-related outcomes
Progression analyses focused on impairment in motor function, cognition, and ADLs. We defined impairment in motor function as new development of postural instability (i.e., progressing to Stage 3 or above on the Hoehn & Yahr Scale [H& Y] [10]) when evaluated in the medication OFF state. For cognitive progression, we determined a composite measure, defined as any one of three outcomes: 1) at least 1.5 standard deviations below normative scores on more than one neuropsychological test, 2) a Montreal Cognitive Assessment (MoCA) [11] score below 26, or 3) a determination of mild cognitive impairment or dementia by the site investigator. For impairment in ADLs, we defined progression as a response of 3 or greater on any one of the following items on the MDS-UPDRS Parts II and III: swallowing (item 2.3), eating (item 2.4), dressing (item 2.5), hygiene (item 2.6), or speech (item 3.1) in the ON or OFF medicationstate.
Statistical analysis
We first summarized descriptive statistics and compared each of the PD and non-PD cohorts using Wilcoxon rank-sum non-parametric tests for continuous measures and chi-squared tests for categorical measures. To investigate the association between occupational pesticide exposure and PD, we calculated the odds ratio (OR) as an estimate of the risk of PD in exposed vs. unexposed participants. We used logistic regression to adjust for age at enrollment, sex, and cigarette smoking. For situations where the number of exposures were too few to calculate an OR, we used the Woolf correction method to calculate a risk estimate [12].
To determine the association between PD symptom progression and exposure, we performed a time-to-event analysis using our two motor outcomes, the cognitive composite outcome, and the composite ADL outcome. Time zero was defined as the time PD was diagnosed, and information was censored at the last available clinical observation and self-report (i.e., the time of data download on February 2, 2022). Cox proportional hazard models were then used to estimate hazard ratios (HR), adjusting for age at enrollment, duration of PD at baseline, and sex; years of education were also included for the cognitive outcome. Analyses were performed using R version 4.2.1 [13].
Standard protocol approvals, registrations, and patient consents
Approval for the PPMI study was obtained at each participating site. Written informed consent was obtained from all study participants upon enrollment in PPMI and then again prior to completion of the PD-RFQ. Approval for the implementation of questionnaires about environmental exposure was granted by the UCSF Committee on Human Research.
RESULTS
Study population
A total of 403 participants who consented to the PD-RFQ study and had LRRK2 or GBA variants were invited to fill out the PD-RFQ and 380 (94.3%) completed the questionnaire; two participants were excluded as age of first pesticide exposure was missing (Table 1). Among the GBA group, 193 participants had the N370S variant (41 with PD), 4 had the 84GG variant (3 with PD), 3 had the L444P variant (1 with PD), 1 individual with PD had the IVS2 + 1 G>A variant, and 1 individual with PD had the R120 W variant. Among the LRRK2 group, all 176 participants carried G2019 S (54 with PD). Compared to PPMI participants who did not complete or were not invited to complete the questionnaire, there was a higher proportion of males among LRRK2-PD respondents (61% vs. 42%; p = 0.02), but demographics were not otherwise significantly different. Among participants who completed the questionnaire, LRRK2-PD participants were slightly older than LRRK2-NMC, and both LRRK2-PD and GBA-PD groups had a higher proportion of males than their corresponding non-manifesting group (Table 1). Thirteen participants had both a LRRK2-G2019S and GBA-N370S variant, only one of whom had PD.
Table 1
Occupational pesticide questionnaire respondents by cohort
LRRK21 NMC | LRRK21 PD | p | GBA2 NMC | GBA PD3 | p | |
N = 122 | N = 54 | N = 155 | N = 47 | |||
Age at enrollment in PPMI, Mean (SD) | 62 (7) | 64 (7) | 0.019 | 62 (7) | 61 (10) | 0.7 |
Sex, n (%) | ||||||
Female | 73 (60) | 21 (39) | 0.016 | 88 (57) | 19 (40) | 0.072 |
Male | 49 (40) | 33 (61) | 67 (43) | 28 (60) | ||
Years of PD at enrollment in PPMI, Mean (SD) | – | 2.65 (2.08) | – | 2.80 (2.53) |
NMC, non-manifesting carriers (genetic variant present but have not been diagnosed with PD). 1LRRK2 variants include G2019S in both the NMC and PD group. 2GBA NMC variants include N370S, 84GG, and L444P. 3GBA PD variants include N370S, 84GG, L444P, R120W, and IVS2 + 1 G>A.
Table 2
Association of occupational pesticide use and genetic PD
LRRK2 NMC | LRRK2 PD | aOR | 95% CI | p | |
N = 122 | N = 54 | ||||
Any pesticide, n (%) | 7 (5.7) | 5 (9.3) | 1.32 | 0.36, 4.56 | 0.7 |
Herbicides, n (%) | 7 (5.7) | 2 (3.9) | 0.39 | 0.05, 1.83 | 0.3 |
Insecticides, n (%) | 3 (2.5) | 3 (5.8) | 2.34 | 0.40, 13.5 | 0.3 |
Fungicides, n (%) | 2 (1.7) | 3 (5.8) | 3.03 | 0.45, 24.9 | 0.3 |
GBA NMC | GBA PD | aOR | 95% CI | p | |
N = 155 | N = 47 | ||||
Any pesticide, n (%) | 6 (3.9) | 8 (17) | 5.35 | 1.65, 18.5 | 0.006 |
Herbicides, n (%) | 4 (2.6) | 4 (9.3) | 4.14 | 0.87, 19.8 | 0.066 |
Insecticides, n (%) | 4 (2.6) | 7 (15) | 8.08 | 2.14, 34.9 | 0.003 |
Fungicides, n (%) | 1 (0.7) | 3 (7.1) | 14.6 | 1.59, 321 | 0.030 |
Pesticide use defined as occupational exposure prior to index date, i.e., diagnosis in people with PD and study enrollment in NMC. Odds ratio adjusted for age, sex, and regular tobacco use. NMC, Non-manifesting carrier.
Pesticides and PD risk
Out of 378 participants who completed the questionnaire, 26 (6.8%) reported exposure to occupational pesticides, 12/176 (6.8%) with a LRRK2 variant and 14/202 (6.9%) participants with a GBA variant (Table 3). The proportions within each analytic group and adjusted ORs are shown in Table 2. Among people with a GBA variant, pesticide exposure was associated with a 5.4 (95% CI 1.7– 18.5, p < 0.01) times higher odds of PD, with a particularly high odds associated with insecticide and fungicide exposure. Among people with a LRRK2 variant, pesticide exposure was associated with a 1.3 (95% CI 0.4– 4.6, p = 0.7) times higher odds of PD. Among the 8 GBA-PD exposed to pesticides, 7 had the N370S variant and 1 had the 84GG variant; all 6 GBA-NMC participants who were exposed to pesticides had the N370 S variant. Most participants who reported pesticide exposure did not name the specific agents to which they were exposed. Three GBA-PD participants did report exposure to specific agents, with exposure to paraquat, permethrin, and parathion being common among all three. Two LRRK2-PD participants reported exposure to specific agents, which included copper fungicides (n = 1) and aldrin/dieldrin products and DDT (n = 1). Farming and landscaping were the most common occupations reported among participants who were exposed (Supplementary Table 2). Among the 13 participants with both a LRRK2 and GBA variant, none reported pesticide exposure.
Table 3
Characteristics of participants with and without pesticide exposure
LRRK2 NMC | LRRK2 PD | |||||
No occupational pesticide exposure | Occupational pesticide exposure | p | No occupational pesticide exposure | Occupational pesticide exposure | p | |
N = 115 | N = 7 | N = 49 | N = 5 | |||
Age at baseline, Mean (SD) | 62 (7) | 66 (8) | 0.2 | 65 (7) | 61 (8) | 0.4 |
Sex, n (%) | 0.017 | >0.9 | ||||
Female | 72 (63) | 1 (14) | 19 (39) | 2 (40) | ||
Male | 43 (37) | 6 (86) | 30 (61) | 3 (60) | ||
Regular tobacco smoking, n (%) | 42 (37) | 5 (71) | 0.11 | 19 (39) | 2 (40) | >0.9 |
Years of PD at baseline | 1.92 (0.83, 3.17) | 4.67 (2.25, 6.51) | 0.056 | |||
GBA NMC | GBA PD | |||||
No occupational pesticide exposure | Occupational pesticide exposure | p | No occupational pesticide exposure | Occupational pesticide exposure | p | |
N = 149 | N = 6 | N = 39 | N = 8 | |||
Age at baseline, Mean (SD) | 62 (7) | 62 (6) | >0.9 | 61 (10) | 64 (7) | 0.4 |
Sex, n (%) | 0.006 | 0.4 | ||||
Female | 88 (59) | 0 (0) | 17 (44) | 2 (25) | ||
Male | 61 (41) | 6 (100) | 22 (56) | 6 (75) | ||
Regular tobacco smoking, n (%) | 42 (29) | 1 (17) | 0.7 | 18 (50) | 2 (25) | 0.3 |
Years of PD at baseline | 1.92 (0.58, 4.13) | 3.42 (0.88, 5.54) | 0.3 |
NMC, non-manifesting carriers (genetic variant present but have not been diagnosed with PD.).
We then stratified analyses based on duration of pesticide exposure. Among carriers of a LRRK2 variant, longer pesticide exposure was non-significantly associated with a higher risk of PD (Table 4; p-trend = 0.08). Among carriers of a GBA variant, longer duration of pesticide exposure was associated with a substantially higher PD risk than shorter duration, although confidence limits were broad (Table 4; p-trend<0.01). No relationships were found in models stratified by use of protective gloves (data notshown).
Table 4
Association of duration of occupational pesticide exposure and genetic PD
LRRK2 NMC | LRRK2 PD | p∧ | OR | 95% CI | p | |
N = 122 | N = 54 | |||||
No pesticide exposure, n (%) | 115 (96) | 49 (92) | 0.08 | Reference | ||
Pesticide exposure< =5 y, n (%) | 5 (4.2) | 1 (1.9) | 0.47 | 0.02, 3.01 | 0.50 | |
Pesticide exposure > 5 y, n (%) | 0 (0) | 3 (5.7) | 16.3 | 0.83, 322.1 | –* | |
Not reported | 2 | 1 | ||||
GBA NMC | GBA PD | p∧ | OR | 95% CI3 | p | |
N = 155 | N = 47 | |||||
No pesticide exposure, n (%) | 149 (97) | 39 (83) | <0.001 | Reference | ||
Pesticide exposure< =5 y, n (%) | 4 (2.6) | 4 (8.5) | 3.82 | 0.87, 16.8 | 0.066 | |
Pesticide exposure > 5 y, n (%) | 0 (0) | 4 (8.5) | 34.1* | 1.80, 646 | – | |
Not reported | 2 | 0 |
∧p-value indicates chi-squared for trend in proportions. *Woolf correction method used to calculate unadjusted OR and confidence interval.
Pesticides and early PD Progression
To understand how prior occupational pesticide exposure affected PD progression, we evaluated the progression of motor, cognitive, and functional outcomes and their relationship to exposure in people with PD and a LRRK2 or GBA variant. Among LRRK2-PD, pesticide exposure was associated with an increased risk of faster development of motor and cognitive problems, though neither was statistically significant (aHR for motor: 3.7, 95% CI 0.3–41.7, p = 0.3; aHR for MCI: 4.07, 95% CI 0.80–20.6, p = 0.090; Table 5 and Supplementary Table 3). Among GBA-PD, pesticide exposure was associated with a faster rate of developing problems with ADLs, though again this difference was not statistically significant (aHR 4.4, 95% CI 0.7–29.0, p = 0.1; Fig. 1, Table 5 and Supplementary Table 4). These associations were even stronger for participants who reported greater than 5 years of pesticide exposure compared to those without any pesticide exposure (p-trend 0.03; Supplementary Tables 5 and 6).
Table 5
Development of motor and cognitive outcomes based on pesticide exposure prior to index date
LRRK2-PD | n (%) with outcome | aHR | 95% CI | p |
H &Y 3 | ||||
No pesticide exposure, n = 45 | 11 (24) | – | – | – |
Pesticide exposure, n = 4 | 1 (25) | 3.67 | 0.32, 41.7 | 0.3 |
MCI | ||||
No pesticide exposure, n = 47 | 17 (36) | – | – | – |
Pesticide exposure, n = 5 | 3 (60) | 4.07 | 0.80, 20.6 | 0.090 |
ADL Impairment | ||||
No pesticide exposure, n = 49 | 6 (12) | – | – | – |
Pesticide exposure, n = 5 | 0 (0) | N/A | ||
GBA-PD | n (%) with outcome | aHR | 95% CI | p |
H &Y 3 | ||||
No pesticide exposure, n = 38 | 10 (26) | – | – | – |
Pesticide exposure, n = 8 | 2 (25) | 0.25 | 0.04, 1.55 | 0.14 |
MCI | ||||
No pesticide exposure, n = 37 | 19 (51) | – | – | – |
Pesticide exposure, n = 8 | 4 (50) | 0.70 | 0.22, 2.19 | 0.5 |
ADL Impairment | ||||
No pesticide exposure, n = 39 | 4 (10) | – | – | – |
Pesticide exposure, n = 8 | 3 (38) | 4.38 | 0.66, 29.0 | 0.13 |
aHR indicates Cox proportional hazard adjusted for age of PD diagnosis, years of PD at enrollment in PPMI, sex, and, in the case of MCI, education. ADLs, Activities of daily living. MCI defined by composite outcome (see methods).
Fig. 1
Kaplan-Meier curve showing progression of impairment in activities of daily living among people with PD and a GBA variant with and without a history of pesticide exposure.
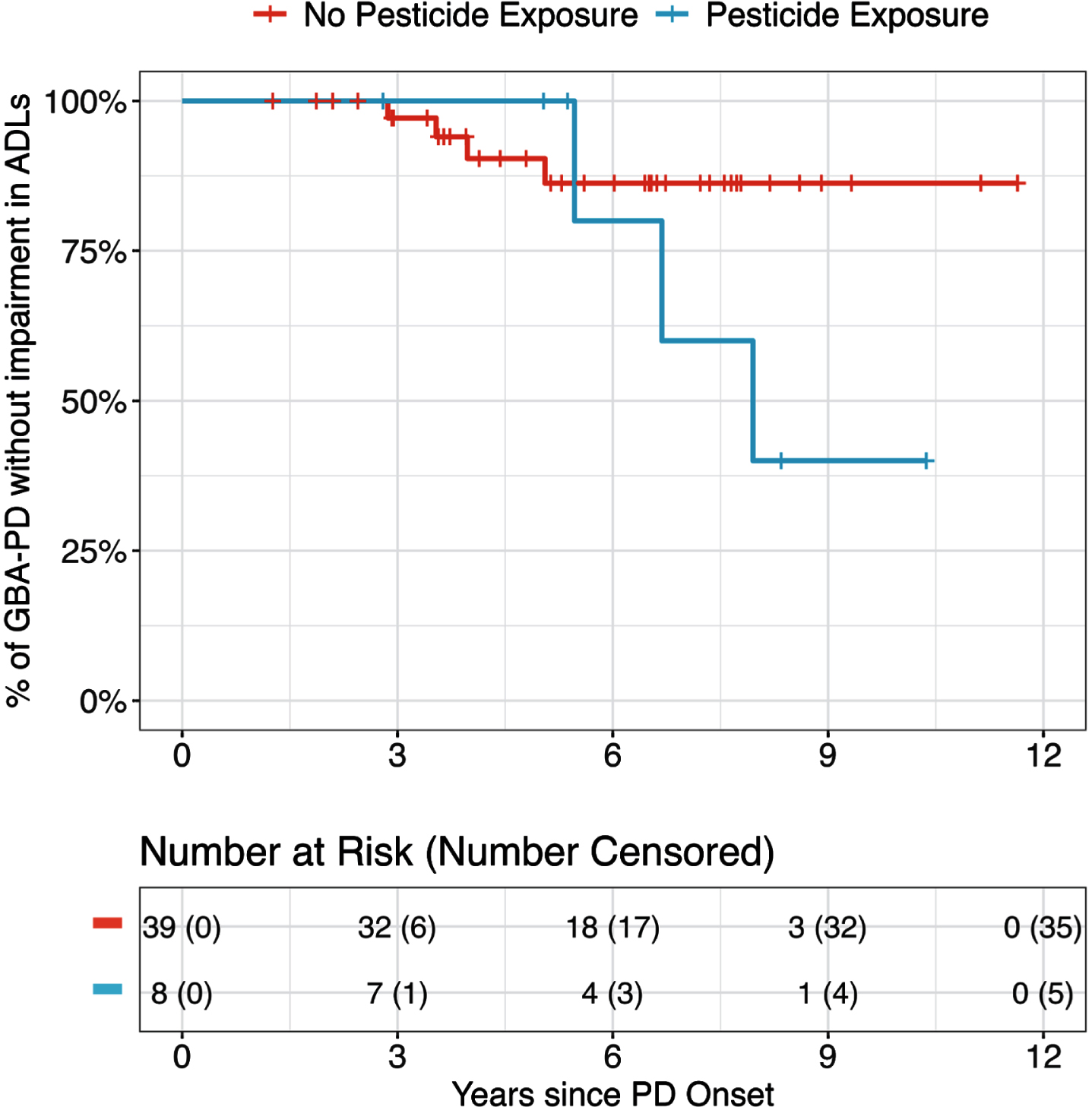
DISCUSSION
Our study found that among people with a GBA variant, occupational pesticide exposure was associated with more than five times the odds of having PD, suggesting that pesticide exposure may play an important role in GBA penetrance. A longer duration of pesticide exposure was associated with even higher odds of having PD, supporting the biological plausibility of this finding. To our knowledge, this association has not been previously reported (for a summary of prior relevant studies see Supplementary Table 7). One study found that a higher proportion of people with GBA-PD reported pesticide exposure compared to people with PD without any known genetic variant (n = 35 in each group; 29% vs. 6.1%) [14]. While supportive of our findings, this study did not include carriers of GBA variants without PD and could not comment on the potential effect of pesticides on GBA penetrance. A recent study found no interaction between GBA carrier status and use of pesticides around the home or garden in a typical month [15]. However, the high prevalence of reported pesticide use in this study (42% in people with PD and 38% in controls) may indicate a large variability of exposure levels and duration which were not explored. Furthermore, pesticide use was not limited to pre-diagnostic exposure, and residential use likely involves different types of agents and levels of exposure compared to occupational use.
Reduction in GCase activity due to GBA variants, including N370 S, impairs autophagy-lysosomal function [16], which may lead to decreased pesticide clearance and render people with these variants particularly susceptible to pesticide toxicity [17, 18]. Impaired autophagy may also lead to mitochondrial dysfunction [19], which may exacerbate mitochondrial injury occurring from pesticide exposure [4]. Interestingly, all GBA-PD participants that listed specific agents reported exposure to multiple pesticides, including paraquat and permethrin, both of which have been associated with PD in prior studies [20]. Given the low penetrance of GBA risk variants— estimated at 10–20% depending on age [1]— identifying environmental factors that affect penetrance could be important for understanding GBA pathophysiology and targeted prevention efforts.
We also evaluated occupational pesticide exposure and PD risk in LRRK2 variant carriers. We found a non-significantly elevated odds of PD, an association that to our knowledge has not been previously identified though has been suggested through several laboratory-based studies. In dopaminergic neuron-like cells, rotenone, a mitochondrial Complex I inhibiting pesticide associated with PD [21], impaired clearance of alpha-synuclein fibrils through upregulation of LRRK2 phosphorylation, a consequence mitigated by a LRRK2 inhibitor [22]. In mice, combined exposure to the fungicide maneb and herbicide paraquat, associated with increased PD risk in humans [21, 23], reduced expression of genes related to cellular differentiation, an effect magnified among mice with a LRRK2-G2019S variant [24]. LRRK2 knockout mice were protected from the mortality, weight loss, and behavioral changes induced by paraquat, while mice with LRRK2-G2019S showed increases in stress-related markers after paraquat exposure [25]. Similar studies in humans are more limited: two studies found no significant interaction between common LRRK2 risk variants and pesticide exposure in terms of increased PD risk [26, 27], but neither study included people with LRRK2-G2019 S variants. Whether similar mechanisms of interaction are present in humans needs to be furtherelucidated.
We further evaluated how pesticide exposure relates to symptom progression in these groups, as some studies have suggested an association between pesticide exposure and symptom progression in PD [28, 29]. While the small number of exposed people with PD made our estimates imprecise, our results suggest that pesticide exposure may hasten cognitive problems in people with LRRK2-PD and functional impairment in people with GBA-PD. Among LRRK2-PD, few participants developed balance problems or problems with ADL’s, which may reflect the slower motor progression thought to be associated with LRRK2-PD [30]. Similarly, a high proportion of people with GBA-PD with and without pesticide exposure developed cognitive impairment, consistent with the known relationship between GBA and cognitive problems [31]. Pesticide exposure may therefore be an important risk factor for progression to involve domains in addition to those classically associated with a specific genotype.
These findings should be interpreted in the context of several limitations. Our overall sample size was small with a low number of participants exposed. This limited some of our analyses— for instance, the stronger association we found between longer duration of pesticide exposure and PD may have been confounded by sex, as men were more likely to report exposure to occupational pesticides. Our sample size may have been too small to detect a moderate relationship between LRRK2-G2019S, pesticide exposure, and PD and could explain the non-significant association we found. Despite this limitation, the association that we found among carriers of the GBA variant was large. The exposure assessment was more complete than the simple categorical associations reported in many studies, allowing assessment for the effects of duration of exposure and, in some, type of pesticide. These results are indirectly supported by laboratory studies. Exposure data was measured through self-report and recall bias may have led to an over-estimation of the risk of PD after pesticide exposure. Recall bias may have also contributed to our progression analysis, though this effect is less likely: the diagnosis and age of PD were determined through interviews by site personnel that occurred separately from exposure ascertainment, and the outcomes consisted of clinically determined measures by neurologists unaware of exposure status. We were not able to evaluate formally the relationship between progression and specific types of pesticides, due to the small number of participants endorsing exposure to specific agents.
Our findings are hypothesis generating, given the small sample size, and require replication. Evaluating this relationship through other means of ascertaining pesticide exposure, such as geographic information systems (GIS) [32] may also identify specific pesticides or classes with strong association. Broadening to other types of exposure beyond occupational, such as through residential use or proximity to agricultural areas, could increase the number of participants exposed. Several avenues of further exploration are also suggested. Studies assessing the effects of pesticide exposure on PD risk within groups with different GBA risk variants as well as the relationship between pesticide exposure and glucocerebrosidase activity may clarify the mechanism underlying this association. Among non-manifesting carriers of genetic PD risk variants, or other populations at risk of PD, evaluating the temporal relationship between pesticide exposure and PD-related symptom progression would improve our understanding of how pesticides contribute to disease development. The relationship between pesticides and PD may be further clarified through studies of potential pathways of involvement, such as metabolomic studies investigating mitochondrial function, inflammatory changes, or oxidative stress [33]. Such studies may identify biomarkers or even provide an objective means of measuring the biologic effects ofexposure.
In conclusion, we found that pesticides substantially increase the risk of PD in people with a GBA risk variant and may contribute to symptom progression in people with PD and risk variants in GBA or LRRK2. Pesticide exposure may therefore play an important role in genetic penetrance in PD as well as in progression after PD develops. Targeted prevention efforts may be needed in these geneticsubgroups.
ACKNOWLEDGMENTS
This study was funded by the Michael J. Fox Foundation for Parkinson’s research. Data used in the preparation of this article were obtained on February 2, 2022, from the Parkinson’s Progression Markers Initiative (PPMI) database (http://www.ppmi-info.org/access-data-specimens/download-data), RRID:SCR 006431. For up-to-date information on the study, visit http://www.ppmi-info.org. PPMI – a public-private partnership – is funded by the Michael J. Fox Foundation for Parkinson’s Research and funding partners, including 4D Pharma, Abbvie, AcureX, Allergan, Amathus Therapeutics, Aligning Science Across Parkinson’s, AskBio, Avid Radiopharmaceuticals, BIAL, BioArctic, Biogen, Biohaven, BioLegend, BlueRock Therapeutics, Bristol-Myers Squibb, Calico Labs, Capsida Biotherapeutics, Celgene, Cerevel Therapeutics, Coave Therapeutics, DaCapo Brainscience, Denali, Edmond J. Safra Foundation, Eli Lilly, Gain Therapeutics, GE HealthCare, Genentech, GSK, Golub Capital, Handl Therapeutics, Insitro, Janssen Neuroscience, Jazz Pharmaceuticals, Lundbeck, Merck, Meso Scale Discovery, Mission Therapeutics, Neurocrine Biosciences, Neuropore, Pfizer, Piramal, Prevail Therapeutics, Roche, Sanofi, Servier, Sun Pharma Advanced Research Company, Takeda, Teva, UCB, Vanqua Bio, Verily, Voyager Therapeutics, the Weston Family Foundation and Yumanity Therapeutics.
FUNDING
This study is funded by the Michael J. Fox Foundation for Parkinson’s Disease Research.
CONFLICT OF INTEREST
EB receives grant support from the Michael J. Fox Foundation (MJFF), the UCSF EaRTH Center, the National Institutes of Health (NIH), and Gateway LLC. He has received consulting fees from Guidepoint and Rune Labs.
SG receives grant support from the NIH, Department of Defense (DoD), MJFF, and the Health Resources and Services Administration (HRSA). He has received consulting fees from MJFF.
CC receives grant support from the NIH/National Institute of Neurologic Disorders and Stroke (NINDS) and MJFF.
AS receives grant support from MJFF and the NIH. He has received consulting fees from SPARC Therapeutics, Capsida Therapeutics, and the Parkinson Study Group. He has received honoraria from Bial. He has participated on a Data Safety Monitoring Board or Advisory Board for Wave Life Sciences, Inhibikase, Prevail, the Huntington Study Group, and Massachusetts General Hospital.
TS receives grant support from MJFF, the Parkinson’s Foundation, as an investigator for NINDS, Amneal, Biogen, Roche, Neurodergm, Sanofi, Prevail, and UCB. She has received consulting fees from AcureX, Adamas, AskBio, Amneal, Blue Rock Therapeutics, Critical Path for Parkinson’s Consortium (CPP), Denali, MJFF, Neuroderm, Sanofi, Sinopia, Roche, Takeda, and Vanqua Bio. She has participated on an Advisory Board for AcureX, Adamas, AskBio, Biohaven, Denali, GAIN, Neuron23, Roche, Koneksa, Neuroderm, Sanofi, and UCB.
MB receives research support from MJFF.
KM has received consulting fees from MJFF, Roche, UCB, Denali, Biohaven, Neuron23, Aprinoia, Prothena, Calico, Inhibikase, Invicro, Koneksa, Lilly, Amyl, and IRlab.
CMT receives grant support from MJFF, NIH, Gateway LLC, DoD, Roche, Biogen, Parkinson’s Foundation, and the Marcus Program in Precision medicine. She has received consulting fees from CNS Ratings, Australian Parkinson’s Mission, Biogen, Evidera. She has participated on a Data Safety Monitoring Board or Advisory Board for Cadent, Adamas, Biogen, Kyowa Kirin, Lundbeck, Jazz/Cavion, Acorda, Bia, and Genentech.
DATA AVAILABILITY
The de-identified data used in this manuscript is available to researchers through ppmi-info.org.
SUPPLEMENTARY MATERIAL
[1] The supplementary material and Appendix is available in the electronic version of this article: https://dx.doi.org/10.3233/JPD-240015.
REFERENCES
[1] | Balestrino R , Tunesi S , Tesei S , Lopiano L , Zecchinelli AL , Goldwurm S ((2020) ) Penetrance of glucocerebrosidase (GBA) mutations in Parkinson’s disease: A kin cohort study. Mov Disord 35: , 2111–2114. |
[2] | Lee AJ , Wang Y , Alcalay RN , Mejia-Santana H , Saunders-Pullman R , Bressman S , Corvol JC , Brice A , Lesage S , Mangone G , Tolosa E , Pont-Sunyer C , Vilas D , Schüle B , Kausar F , Foroud T , Berg D , Brockmann K , Goldwurm S , Siri C , Asselta R , Ruiz-Martinez J , Mondragón E , Marras C , Ghate T , Giladi N , Mirelman A , Marder K ((2017) ) Penetrance estimate of LRRK2 p.G2019S mutation in individualsof non-Ashkenazi Jewish ancestry. Mov Disord 32: , 1432–1438. |
[3] | Ascherio A , Schwarzschild MA ((2016) ) The epidemiology of Parkinson’s disease: Risk factors and prevention. Lancet Neurology 15: , 1257–1272. |
[4] | Goldman SM ((2014) ) Environmental toxins and Parkinson’s disease. Annu Rev Pharmacol Toxicol 54: , 141–164. |
[5] | Kline EM , Houser MC , Herrick MK , Seibler P , Klein C , West A , Tansey MG ((2021) ) Genetic and environmental factors in Parkinson’s disease converge on immune function and inflammation. Mov Disord 36: , 25–36. |
[6] | Parkinson’s Progression Marker Initiative. https://www.ppmi-info.org/, Accessed July 28, 2023. |
[7] | NINDS Common Data Elements: Risk Factor Questionnaire (RFQ-U). https://www.commondataelements.ninds.nih.gov/report-viewer/23723/Risk%20Factor%20Questionnaire%20(RFQ-U)%20-%20Pesticides%20(Work)#:∼:text=The%20Risk%20Factor%20Questionnaire%20was,epidemiologic%20studies%20of%20Parkinson’s%20disease,Accessed April 21, 2023. |
[8] | Foroud T , Smith D , Jackson J , Verbrugge J , Halter C , Wetherill L , Sims K , Xin W , Arnedo V , Lasch S , Marek K ((2015) ) Novel recruitment strategy to enrich for LRRK2 mutation carriers. Mol Genet Genomic Med 3: , 404–412. |
[9] | Marek K , Chowdhury S , Siderowf A , Lasch S , Coffey CS , Caspell-Garcia C , Simuni T , Jennings D , Tanner CM , Trojanowski JQ , Shaw LM , Seibyl J , Schuff N , Singleton A , Kieburtz K , Toga AW , Mollenhauer B , Galasko D , Chahine LM , Weintraub D , Foroud T , Tosun-Turgut D , Poston K , Arnedo V , Frasier M , Sherer T ((2018) ) The Parkinson’s progression markers initiative (PPMI) - establishing a PD biomarker cohort. Ann Clin Transl Neurol 5: , 1460–1477. |
[10] | Hoehn MM , Yahr MD ((1967) ) Parkinsonism. Neurology 17: , 427. |
[11] | Nasreddine ZS , Phillips NA , Bédirian V , Charbonneau S , Whitehead V , Collin I , Cummings JL , Chertkow H ((2005) ) The Montreal Cognitive Assessment, MoCA: A brief screening tool for mild cognitive impairment. J Am Geriatr Soc 53: , 695–699. |
[12] | Lawson R ((2004) ) Small sample confidence intervals for the odds ratio. Commun Stat Simul Comput 33: , 1095–1113. |
[13] | R Core Team ((2022) ) R: A language and environment for statistical computing. R Foundation for Statistical Computing, Vienna, Austria. |
[14] | Simitsi A , Koros C , Moraitou M , Papagiannakis N , Antonellou R , Bozi M , Angelopoulou E , Stamelou M , Michelakakis H , Stefanis L ((2018) ) Phenotypic characteristics in GBA-associated Parkinson’s disease: A study in a Greek population. J Parkinsons Dis 8: , 101–105. |
[15] | Reynoso A , Torricelli R , Jacobs BM , Shi J , Aslibekyan S , Norcliffe-Kaufmann L , Noyce AJ , Heilbron K ((2024) ) Gene-environment interactions for Parkinson’s disease. Ann Neurol 95: , 677–687. |
[16] | Smith L , Schapira AHV ((2022) ) GBA variants and Parkinson disease: mechanisms and treatments. Cells 11: , 1261. |
[17] | Velayati A , Yu WH , Sidransky E ((2010) ) The role of glucocerebrosidase mutations in Parkinson disease and Lewy body disorders. Curr Neurol Neurosci Rep 10: , 190–198. |
[18] | He B , Wang X , Yang C , Zhu J , Jin Y , Fu Z ((2020) ) The regulation of autophagy in the pesticide-induced toxicity: Angel or demon? Chemosphere 242: , 125138. |
[19] | Li H , Ham A , Ma TC , Kuo SH , Kanter E , Kim D , Ko HS , Quan Y , Sardi SP , Li A , Arancio O , Kang UJ , Sulzer D , Tang G ((2019) ) Mitochondrial dysfunction and mitophagy defect triggered by heterozygous GBA mutations. Autophagy 15: , 113–130. |
[20] | Tanner CM , Ross GW , Jewell SA , Hauser RA , Jankovic J , Factor SA , Bressman S , Deligtisch A , Marras C , Lyons KE , Bhudhikanok GS , Roucoux DF , Meng C , Abbott RD , Langston JW ((2009) ) Occupation and risk of parkinsonism: A multicenter case-control study. Arch Neurol 66: , 1106–1113. |
[21] | Tanner CM , Kamel F , Ross GW , Hoppin JA , Goldman SM , Korell M , Marras C , Bhudhikanok GS , Kasten M , Chade AR , Comyns K , Richards MB , Meng C , Priestley B , Fernandez HH , Cambi F , Umbach DM , Blair A , Sandler DP , Langston JW ((2011) ) Rotenone, paraquat, and Parkinson’s disease. Environ Health Perspect 119: , 866–872. |
[22] | Ho DH , Nam D , Seo MK , Park SW , Seol W , Son I ((2021) ) LRRK2 kinase inhibitor rejuvenates oxidative stress-induced cellular senescence in neuronal cells. Oxid Med Cell Longev 2021: , 9969842. |
[23] | Costello S , Cockburn M , Bronstein J , Zhang X , Ritz B ((2009) ) Parkinson’s disease and residential exposure to maneb and paraquat from agricultural applications in the central valley of California. Am J Epidemiol 169: , 919–926. |
[24] | Desplats P , Patel P , Kosberg K , Mante M , Patrick C , Rockenstein E , Fujita M , Hashimoto M , Masliah E ((2012) ) Combined exposure to Maneb and Paraquat alters transcriptional regulation of neurogenesis-related genes in mice models of Parkinson’s disease. Mol Neurodegener 7: , 49. |
[25] | Rudyk C , Dwyer Z , Hayley S ((2019) ) Leucine-rich repeat kinase-2 (LRRK2) modulates paraquat-induced inflammatory sickness and stress phenotype. J Neuroinflammation 16: , 120. |
[26] | Lin C-H , Wu R-M , Tai C-H , Chen M-L , Hu F-C ((2011) ) Lrrk2 S1647T and BDNF V66M interact with environmental factors to increase risk of Parkinson’s disease. Parkinsonism Relat Disord 17: , 84–88. |
[27] | Chung SJ , Armasu SM , Anderson KJ , Biernacka JM , Lesnick TG , Rider DN , Cunningham JM , Ahlskog JE , Frigerio R , Maraganore DM ((2013) ) Genetic susceptibility loci, environmental exposures, and Parkinson’s disease: a case-control study of gene-environment interactions. Parkinsonism Relat Disord 19: , 595–599. |
[28] | Schneider Medeiros M , S PR , M PS , Schumacher-Schuh AF , Mello Rieder CR ((2020) ) Occupational pesticide exposure and the risk of death in patients with Parkinson’s disease: an observational study in southern Brazil. Environ Health 19: , 68. |
[29] | Li S , Ritz B , Gong Y , Cockburn M , Folle AD , Del Rosario I , Yu Y , Zhang K , Castro E , Keener AM , Bronstein J , Paul KC ((2023) ) Proximity to residential and workplace pesticides application and the risk of progression of Parkinson’s diseases in Central California. Sci Total Environ 864: , 160851. |
[30] | Saunders-Pullman R , Mirelman A , Alcalay RN , Wang C , Ortega RA , Raymond D , Mejia-Santana H , Orbe-Reilly M , Johannes BA , Thaler A , Ozelius L , Orr-Urtreger A , Marder KS , Giladi N , Bressman SB ((2018) ) Progression in the LRRK2-asssociated Parkinson disease population. JAMA Neurol 75: , 312–319. |
[31] | Davis MY , Johnson CO , Leverenz JB , Weintraub D , Trojanowski JQ , Chen-Plotkin A , Van Deerlin VM , Quinn JF , Chung KA , Peterson-Hiller AL , Rosenthal LS , Dawson TM , Albert MS , Goldman JG , Stebbins GT , Bernard B , Wszolek ZK , Ross OA , Dickson DW , Eidelberg D , Mattis PJ , Niethammer M , Yearout D , Hu S-C , Cholerton BA , Smith M , Mata IF , Montine TJ , Edwards KL , Zabetian CP ((2016) ) Association of GBA mutations and the E326K polymorphism with motor and cognitive progression in Parkinson disease. JAMA Neurol 73: , 1217–1224. |
[32] | Paul KC , Sinsheimer JS , Rhodes SL , Cockburn M , Bronstein J , Ritz B ((2016) ) Organophosphate pesticide exposures, nitric oxide synthase gene variants, and gene-pesticide interactions in a case-control study of Parkinson’s Disease, California (USA). Environ Health Perspect 124: , 570–577. |
[33] | Yan Q , Paul KC , Walker DI , Furlong MA , Del Rosario I , Yu Y , Zhang K , Cockburn MG , Jones DP , Ritz BR ((2021) ) High-resolution metabolomic assessment of pesticide exposure in Central Valley, California. Chem Res Toxicol 34: , 1337–1347. |