Characterization of Novel Human β-glucocerebrosidase Antibodies for Parkinson’s Disease Research
Abstract
Background:
Mutations in GBA1, which encodes the lysosome enzyme β-glucocerebrosidase (also referred to as acid β-glucosidase or GCase), are the most common genetic risk factor for Parkinson’s disease (PD) and dementia with Lewy bodies (DLB). Evidence also suggests that loss of GCase activity is implicated in PD without GBA1 mutations. Consequently, therapies targeting GCase are actively being pursued as potential strategies to modify the progression of PD and related synucleinopathies. Despite this significant interest in GCase as a therapeutic target, the lack of well-characterized GCase antibodies continues to impede progress in the development of GCase-targeted therapies.
Objective:
This study aims to independently evaluate human GCase (hGCase) antibodies to provide recommendations for western blot, immunofluorescence, immunoprecipitation, and AlphaLISA (Amplified Luminescent Proximity Homogeneous Assay) assays.
Methods:
Two mouse monoclonal antibodies, hGCase-1/17 and hGCase-1/23, were raised against hGCase using imiglucerase, the recombinant enzyme developed to treat patients, as the antigen. These novel antibodies, alongside commonly used antibodies in the field, underwent evaluation in a variety of assays.
Results:
The characterization of hGCase-1/17 and hGCase-1/23 using genetic models including GBA1 loss-of-function human neuroglioma H4 line and neurons differentiated from human embryonic stem cells revealed their remarkable specificity and potency in immunofluorescence and immunoprecipitation assays. Furthermore, a hGCase AlphaLISA assay with excellent sensitivity, a broad dynamic range, and suitability for high throughput applications was developed using hGCase-1/17 and hGCase-1/23, which enabled a sandwich assay configuration.
Conclusions:
The hGCase immunofluorescence, immunoprecipitation, and AlphaLISA assays utilizing hGCase-1/17 and hGCase-1/23 will not only facilitate improved investigations of hGCase biology, but can also serve as tools to assess the distribution and effectiveness of GCase-targeted therapies for PD and related synucleinopathies.
INTRODUCTION
The lysosomal enzyme glucocerebrosidase (GCase) (EC 3.2.1.45), encoded by the gene GBA1, hydrolyzes its lipid substrate glucosylceramide (GlcCer) to ceramide and glucose. Gaucher disease (GD; OMIM #230800, 23090, and 23100) is an autosomal recessively inherited lysosomal storage disorder caused by biallelic pathologic variants in GBA1 that result in deficient GCase activity and lysosomal accumulation of lipid substrates, including GlcCer and glucosylsphingosine (GluSph) [1]. In 2009, a multicenter international collaborative study conclusively established the connection between GBA1 mutations and Parkinson’s disease (PD) highlighting GBA1 variants as common genetic risk factors for the development of PD [2]. GBA1 mutations also elevate the risk for dementia with Lewy bodies (DLB), providing further insight into the role of GCase in PD-related synucleinopathies [3]. This strong association spurred an upsurge in research on the cellular mechanism underlying the GBA1-PD link, as well as the potential of GCase as a therapeutic target for the synucleinopathies [4–6]. With the intensifying focus on GCase, the demand for reliable GCase antibodies for various applications has surged. In 2019, Qi et al. evaluated several existing GCase antibodies for western blotting, revealing that many had limited utility [7]. While both academic and commercial groups have pursued the development of new GCase antibodies, a comprehensive characterization of these newer GCase antibodies for the research community has not been available. The development of GCase antibodies recognizing external epitopes essential for immunostaining, immunoprecipitation, and enzyme-linked immunosorbent assays (ELISA) has proven challenging. Here, we report the development of two mouse monoclonal antibodies, hGCase-1/17 and hGCase-1/23, raised against human GCase (hGCase) using the recombinant enzyme imiglucerase as the antigen. Characterization of these antibodies with genetic models, including GBA1 loss-of-function human neuroglioma H4 line and human neurons differentiated from human embryonic stem cell (hESCs), demonstrated their superior specificity and potency in immunofluorescence, immunoprecipitation, and AlphaLISA (Amplified Luminescent Proximity Homogeneous Assay) assays. These well-characterized hGCase antibodies, soon available to the scientific community through the Michael J. Fox Foundation Research Tools Program, will prove invaluable for future translational research and biomarker development in the context of GD, PD, and related synucleinopathies.
MATERIALS AND METHODS
Cell culture
H4 cells were maintained in DMEM with GlutaMAX (Gibco, cat no: 10566016), supplemented with Sodium Pyruvate (Gibco, cat no: 11360070) and 10% Fetal Bovine Serum (R&D, cat no: S12450) in 6-well tissue culture plates. Human fibroblasts with the GBA1 genotypes wild-type/wild-type, N370S/N370S, and L444P/L444P were cultured in DMEM with GlutaMAX (Gibco, cat no: 10566016) with 15% Fetal Bovine Serum (R&D, cat no: S12450). Cells were kept in a 37°C incubator at 5% CO2. A full media change was performed every 2–3 days and cells were split by dissociation with TrypLE Express Enzyme (Gibco, cat no: 12605010).
H4 cell pellet preparation
Cells were washed in PBS, dissociated with TrypLE Express Enzyme, quenched with cell media, and spun down at 500×g for 5 min. Cells were resuspended in PBS, transferred to a microcentrifuge tube, re-pelleted at 500×g for 5 min, and PBS was discarded. Pellets were flash frozen in dry ice and stored at –80°C for subsequent experiments.
GBA1–/–human neurons
A GBA1–/–hESC line was previously generated and characterized [8]. Neural precursor cells (NPCs) were generated from the hESCs using a modified dual SMAD inhibition protocol [9], as previously described [10, 11]. For neuronal differentiation, NPC were dissociated with 0.05% trypsin-EDTA (ThermoFisher, Cat. 25300054) and plated on poly-L-ornithine/laminin (PLO)-coated dishes at a density of 10,000–15,000 cells/cm2 in N2B27 medium supplemented with 100 ng/ml FGF-8 (PeproTech, Cat.100-25), 200 ng/ml sonic hedgehog (PeproTech, Cat. 100-45), and 100μM ascorbic acid 2-phosphate (Sigma, Cat. A8960-5G) for seven days. Finally, the NPCs were plated on PLO-coated plates at a density of 50,000 cells/cm2 in BGAA medium, which was composed of N2B27 medium supplemented with 20 ng/ml BDNF (R&D System, Cat. 248-BDB-01M/CF), 10 ng/ml GDNF (PeproTech, Cat. 450-10), 500μM Dibutyryl-cAMP (STEMCELL Technologies, Cat. 100-0244), and 100μM ascorbic acid 2-phosphate (Sigma, Cat. A8960-5G). Neuronal cultures were maintained in BGAA medium for 6 weeks, and the cell culture medium was changed every 4 days.
Sanger sequencing
Genomic DNA was extracted from H4 cell pellets with Machery-Nagel genomic DNA extraction kit according to manufacturer instructions (cat no: 740952.250). Primers flanking exon 4 and exon 5 of GBA1 were ordered from IDT. Sequence for forward primer (P1) is aaggcaggtctcaaactcctcac (5’ to 3’) and for reverse primer (P2) is agaatgggcagagtgagattctgc (5’ to 3’). The PCR mix was prepared by combining Ampligold Taq Mastermix (cat no: 439888) without GC enhancer with 0.5 pM of each primer, 0.5μg of genomic DNA. PCR was programmed to the following conditions: 95°C 10 min for 1 cycle; 95°C 30 s, 57°C 1 min, 72°C 1 min for 40 cycles; 72°C 7 min for 1 cycle and held at 4°C. A single specific band corresponding to the 761 bp amplicon was observed following DNA electrophoresis. PCR purification was performed with the QIAquick PCR Purification Kit (Qiagen, cat no: 28106) according to provided protocol. Sanger sequencing was prepared according to Genewiz Sample Submission guidelines using primers P1 or P2 mixed with PCR purification product. Sequencing results were analyzed using SnapGene software.
Protein lysate preparation for western blot
150–200μL of 1% Triton-X Lysis buffer [1% Triton X-100, 10% glycerol, 150 mM NaCl, 25 mM HEPES pH 7.4, 1 mM EDTA, 1.5 mM MgCl2, supplemented with one EDTA-free protease inhibitor tablet per 10 mL of buffer (Pierce, cat no A32965)] was added to H4 pellets from three confluent wells of a 6-well plate. Pellets were pulse-sonicated at 420 Hz for 10 quick pulses and rested for 15–30 min on ice. Then, the cells were centrifuged for 15 min at 13000 xg at 4°C and the supernatant containing lysate was collected in a new tube. Protein concentration was determined by Pierce BCA Protein Assay kit (ThermoScientific, cat no: 23225) and lysates were stored in –80°C.
Resorufin β-D-glucopyranoside GCase assay
5×104 H4 cells were washed once with PBS and lysed in 30μl GCase lysis buffer (0.05 M citric acid, 0.05 M KH2PO4, 0.05 M K2HPO4, 0.11 M KCl, 0.01 M NaCl, 0.001 M MgCl2, pH 6.0 with 0.1% (v/v) TritonX-100, supplemented with freshly added protease inhibitor). 10μl of cell lysate were mixed with 10μl of 10 mM resorufin-β-D-glucopyranoside and baseline fluorescence was measured at t0 immediately. The build-up of fluorescent product (resorufin) was measured after incubation for 2 h at 37°C (λex = 535 nm and λem = 595 nm) indicating GCase activity. Data was normalized to total protein levels and depicted as a percentage of untreated GBA1 WT cells.
Liquid chromatography-mass spectrometry analysis of GluSph
The following analytes and internal standards were purchased from Avanti Polar Lipids:
D-glucosyl-β-1-1’-D-erythro-sphingosine (GluSph (d18 : 1); #860535) and D-glucosyl-β-1-1’-D-erythro-sphingosine-d5 as internal standard 1 (GluSph-d5 (d18 : 1); #860636); D-galactosyl-β-1-1’-D-erythro-sphingosine (GalSph (d18 : 1); #860537) and D-galactosyl-β-1-1’-D-erythro-sphingosine-d5 as internal standard 2 (GalSph-d5 (d18 : 1); #860637). For chromatography HPLC grade solvents as well as Millipore water was used. Acetonitrile (LiChrosolv #1.00030) and methanol (LiChrosolv #1.06007) were obtained from Supelco (Merck), ammonium acetate for mass spectrometry was purchased by Sigma-Aldrich (#73594).
Stock solutions for analytes and internal standards were prepared at 1 mM in DMSO and kept at –20°C. For further spiking solutions acetonitrile/water 9/1 (v/v) was used as solvent. Calibration solutions were prepared by serial dilution in acetonitrile/water 9/1 (v/v) containing 2% DMSO. The concentration ranged from C1 = 10μM to C9 = 0.0039μM.
Analysis was conducted on a LC-MS-MS system consisting of a Waters Xevo-TQ-S mass spectrometer connected to a complete Waters Acquity I-class UPLC system with a flow through needle sample manager using a mixture of acetonitrile/methanol/water 40/40/20 (v/v/v) as wash solvent. The auto-sampler temperature was set to 15 °C. The Xevo TQ-S instrument operated in positive ion electrospray mode with both quadrupoles tuned to unit mass resolution using nitrogen as nebulization- and desolvation gas. The nebulizer gas flow was set to 150 l/h and the desolvation gas flow to 800 l/h with a temperature of 500°C. Argon was used as collision gas at a flow rate of 0.15 ml/min. Analytes and internal standards were detected by multiple reaction monitoring mode (MRM) following the transitions m/z 462.3 to 282.3 and m/z 467.3 > 287.3 at a cone voltage of 30 V and a collision energy of 18 V.
Samples were analyzed on a BEH glycan amide column (100×2.1 mm, 1.7μm particle size, purchased from Waters, Switzerland) with a flow rate of 0.25 ml/min and an oven temperature of 30°C. Eluent A consisted of 100mM ammonium acetate and for eluent B acetonitrile was used. Glycospecific separation was achieved by isocratic elution with 90% B followed by a washing step with 10% B and column reconditioning. The overall analysis time was 12 min.
Sample preparation for in vitro GluSph measurements
50μl of distilled water were added to deep frozen cell pellets and samples were shaken for 30 min at 25°C until the cells thawed. Then, 100μl methanol containing 0.005μM internal standards was added. After shaking 5 min, the supernatant was transferred to a 96-well plate. Tubes were rinsed again with 100μl of methanol and supernatant was transferred to the same plate. The samples were evaporated to dryness and reconstituted in 100μl acetonitrile/water 90/10 (v/v) containing 1% DMSO and analyzed by LC-MS/MS. Lipids were quantified using the peak area ratio analyte/internal standard (= response).
Antibodies
Antibody | Source | Catalog # | Host | Clonality | Assays |
hGCase-1/17 | Roche &NHGRI | Available through MJFF soon | Mouse | Monoclonal | ICC: 10 –25μg mL |
hGCase-1/23 | Roche &NHGRI | Available through MJFF soon | Mouse | Monoclonal | ICC: 10 –25μg mL |
812201 | R&D systems | MAB7410 | Mouse | Monoclonal | Capillary WB 1 : 50 |
2E2 | Abnova | H00002629-M01 | Mouse | Monoclonal | Conventional WB: 1 : 1000 |
Capillary WB: 1 : 50 | |||||
EPR5143 | Abcam | ab128879 | Rabbit | Monoclonal | Conventional WB: 1 : 1000 |
Capillary WB: 1 : 50 | |||||
LAMP1 | Cell Signaling Tech | D2D11 | Rabbit | Monoclonal | ICC: 1 : 400 |
MAP2 | Neuromics | CH22103 | Chicken | Polyclonal | ICC: 1 : 400 |
LIMP2 | Abcam | ab176317 | Rabbit | Monoclonal | Conventional WB: 1 : 1000 |
Western blot: Conventional chemiluminescence platform
For each sample, 40μg of protein was loaded onto a 4–20% Mini-PROTEAN TGX PVDF gel (Bio-Rad Laboratories). After transfer with the Trans-Blot Turbo transfer system (Bio-Rad Laboratories), PVDF membranes (Bio-Rad Laboratories) were dried and reactivated with methanol. Methanol was rinsed off with water and TBST [1xTBS (from 10x TBS, 0.1% Tween] and the membrane was blocked for 1 h at RT with blocking solution [1×TBST, 5% (w/v) BSA (Sigma, cat no: A-7888)]. 5% (w/v) NFDM (Biorad, cat no: 1706404) blocking was also performed for each antibody; 5% BSA showed clearer bands and less background compared to 5% NFDM blocking for all antibodies. PVDF membranes were probed overnight at 4°C with primary antibodies in antibody diluent [2% (w/v) BSA, 1x TBST]. Primary antibodies were diluted so that each antibody had a final concentration of approximately 0.5μg/mL. PVDF membranes were washed 3×10 min at RT with TBST. This was followed by incubation with Goat HRP-coupled secondary anti-mouse IgG (Abcam, cat no: ab205719, 1 : 10K dilution) or anti-rabbit IgG (Abcam, cat no: ab 205718, 1 : 60K dilution) antibodies in antibody diluent. PVDF membranes were washed 3×10 min with TBST. The antigen–antibody complexes were detected with SuperSignal West Pico PLUS Chemiluminescent Substrate (ThermoScientific) using the ChemiDoc XRS + Imager System (Bio-Rad). Blots were exposed for 3 seconds.
Western blot: Automated capillary platform
Simple Western’s Fluorescence Separation Module kit and 12–230 kDa Fluorescence Separation Capillary Cartridges (cat no: SM-W004) were used to perform automated western blotting. Samples were prepared and loaded according to manufacturer instructions. Samples were prepared in 0.1x sample buffer (10x Sample Buffer diluted in water) and Fluorescence 5x Master Mix such that 5μg of protein was loaded per lane at a concentration of 1 mg/mL. Prepared samples in master mix as well as Biotinylated Ladder were boiled at 95°C for 10 min. All primary antibodies were diluted in the provided Antibody Diluent 2 at 1 : 50 dilution, except for R386 and GAPDH, which were diluted 1 : 1500 in Antibody Diluent 2. 10μL of diluted antibody was loaded in each lane. 10μL of HRP-conjugated mouse or rabbit secondary antibody was loaded to the corresponding lanes. Finally, 15μL peroxide-luminol mix was loaded into each lane, the cartridge was centrifuged for 5 min at 1000xg, and wash buffer was added according to the user manual. The cartridge and capillaries were loaded into the Jess instrument (ProteinSimple) and the loading times used were the default for HRP chemiluminescence detection. Results were visualized and analyzed with the Compass for Simple Western program.
Immunocytochemistry
Cells were seeded in 8-well imaging chambers at a density of 30K cells/chamber and grown for 48 h with daily feeding. After 48 h, cells were washed once in PBS and fixed in 4% paraformaldehyde (20 min, RT). Fixed cells were washed in PBS three times and incubated in primary antibody ICC antibody diluent [0.05% Saponin (filtered) and 1% BSA in PBS] overnight at 4°C. Wells were washed three times with PBS, followed by 3×5 min washes with PBS, then covered with aluminum foil and incubated with secondary antibody diluted in ICC antibody diluent in RT. Three PBS wash and 3 × 5 min washes in PBS were repeated, and cells were stored in PBS with NucBlue Fixed Cell ReadyProbes Reagent (DAPI) (ThermoFisher, cat no: R37606) in 4°C until imaging. Confocal images were acquired using a Zeiss LSM 880 confocal microscope using the 63x oil objective.
AlphaLISA
One million H4 cells were washed once with PBS and lysed in 80μl GCase lysis buffer (0.05 M citric acid, 0.05 M KH2PO4, 0.05 M K2HPO4, 0.11 M KCl, 0.01 M NaCl, 0.001 M MgCl2, pH 6.0 with 0.1% (v/v) TritonX-100, supplemented with freshly added protease inhibitor). Samples were diluted 1/5 or 1/10 in 1X Immunoassay buffer (Perkin Elmer, #AL000F). A 2-fold serial dilution of imiglucerase (Genzyme) ranging from 1250 pM –2.4 pM was generated as a standard curve. 10μl of samples or standards were incubated for 4 h at RT in the dark with 1 nM of biotinylated hGCase-1/23 and 20μg/ml of hGCase-1/17-conjugated Acceptor beads (beads:antibody 50 : 1). Subsequently, 40μg/ml of AlphaScreen Streptavidin Donor beads (Perkin Elmer, #6760002) were added and incubated 1 h at RT in the dark. Plate was read on a Tecan Spark plate reader with ex: 680 nm and em: 520–620 nm. GCase concentration was calculated based on a sigmoidal non-linear regression of the imiglucerase standard and then normalized to total protein concentration.
Immunoprecipitation
For immunoprecipitation, the Dynabeads™ Protein A Immunoprecipitation Kit (Thermo Fisher, #10006D) was used. 10μg of hGCase-1/17 or hGCase-1/23 or mouse IgG (Merck Millipore, #NI03) were coupled to 50μl of Protein A magnetic Dynabeads in 200μl Ab Binding and Washing Buffer for 30 min at RT on a rotating wheel. Subsequently, supernatant was removed, and Ab-conjugated beads were mixed with 100μg of cell lysate and incubated for 1 h at RT on a rotating wheel (20μg of cell lysate were saved for analysis). After incubation, supernatant (= flowthrough, FT) was saved for analysis and beads were washed 3 times in 200μl Washing Buffer. For elution, beads were resuspended in 20μl of Elution Buffer mixed with 7μl of 4X LDS sample buffer (Thermo Fisher, # NP0007) and 3μl of 10X reducing agent (Thermo Fisher, # NP0004) and heated for 10 min to 70°C. Supernatant (= elution) was saved for analysis. Samples were analysed by SDS-PAGE loading 10μl of each fraction per lane (lysate and FT were mixed with appropriate volumes of 4X LDS sample buffer and 10X reducing agent prior to loading). Proteins were transferred to a nitrocellulose membrane using the iBlot 2 device and membranes were blocked for 1 h at RT in 5% (w/v) Blotting Grade Blocker/PBS (Biorad, #170-6404). GCase was detected using rb mAb to hGBA antibody (abcam, #128879, clone EPR5143(3)) at 1/1000 in blocking solution. After 3×5 min washes in PBS, membranes were incubated with goat anti-rabbit HRP-labelled antibody (Perkin Elmer, # NEF812001EA) at 1/10000 in 1 % (w/v) Blotting Grade Blocker/PBS for 1.5 h at RT. Proteins were detected using the SuperSignal West Dura Extended Duration Substrate Kit (Thermo Fisher, #34076).
RESULTS
Development of monoclonal antibodies against human β-glucocerebrosidase
Mice were immunized with imiglucerase (Cerezyme, Sanofi), the recombinant hGCase produced in Chinese hamster ovary cells for enzyme replacement therapy (ERT) in patients with GD [12]. Among the screened hybridoma clones, hGCase-1/17 and hGCase-1/23 exhibited selectivity for hGCase in initial screening assays including western blotting and immunofluorescence in HEK293-F cells overexpressing hGCase (Supplementary Figure 1). Notably, hGCase-1/23 demonstrated superior potency compared to hGCase-1/17 in both western blot and immunofluorescence assays. Neither of these antibodies cross-reacted with mouse β-glucocerebrosidase (mGCase) overexpressed in HEK293-F cells in western blotting or immunofluorescence, underscoring their specificity for hGCase over mGCase.
Generation of GCase loss-of-function human neuroglioma H4 cells
To support further hGCase-1/17 and hGCase-1/23 characterization, a GCase loss-of-function (GBA1–/–) human neuroglioma H4 line was generated. H4 (ATCC HTB-148) is a hypertriploid cell line with the modal chromosome number ranging from 63 to 78, with most cells having three copies of chromosome 1 and GBA1 alleles. To disrupt the multiple GBA1 alleles in the H4 cell genome, zinc-finger nuclease (ZFN)-mediated cleavage was combined with targeted integration of a gene disruption cassette into exon 4 of GBA1 alleles, following the workflow developed previously [8]. This targeting approach introduced a puromycin resistance cassette into one of the GBA1 alleles (Fig. 1A). H4 cells with the integrated resistance cassette were enriched using puromycin selection, followed by single cell cloning. Established single cell clones were screened for the resistance cassette by PCR and subsequently with an in vitro GCase activity assay using fluorogenic substrate resorufin-β-D-glucopyranoside (Res-Glc). The most promising clone underwent Sanger sequencing, confirming frameshift indels in the other two GBA1 alleles. A pair of PCR primers, P1 and P2, were designed to generate an amplicon of 761 bp, encompassing GBA1 exons 4 and 5 for Sanger sequencing. The GBA1 allele with the integrated puromycin resistance cassette could not produce PCR product due to the cassette’s size. The Sanger sequencing chromatogram of the chosen clone displayed double peaks near the Zinc-finger nuclease targeting site (Fig. 1B). Parsing the chromatogram with Poly Peak Parser (http://yost.genetics.utah.edu/software.ph) [13] indicated frameshift indels in the two GBA1 alleles that were targeted by ZFN, but without the resistance cassette integration (Supplementary Figure 2A). Given the disruption of all GBA1 alleles, this H4 clone is referred to as GBA1–/–, or the GCase knockout (KO) H4 line. The GBA1–/–H4 cells lacked GCase activity in the Res-Glc in vitro GCase activity assay (Fig. 1C). Corroborating the absence of GCase activity, the GBA1–/–H4 cells showed substantial GlcSph accumulation (Fig. 1D). Moreover, there were only minor changes in the GlcSph stereoisomer galactosylsphingosine (GalSph) in the GBA1–/–H4 cells, probably due to altered lysosomal homeostasis, indicating specific GBA1 targeting (Fig. 1E).
Fig. 1
Generation of a GCase loss-of-function (GBA1–/–) human neuroglioma H4 line. A) Disruption of GBA1 alleles by targeting exon 4 with Zinc-finger nuclease (ZFN) and inserting a puromycin resistance cassette. Puromycin selection assisted with the enrichment of edited cells, which was followed by single cell cloning and validation by Sanger sequencing. B) Sanger sequencing chromatogram showing double peaks in the region near the ZFN targeting site, demonstrating the presence of frameshift indel mutations in two GBA1 alleles targeted by ZFN but lacking resistance cassette integration. C) Lack of GCase activity in GBA1–/–H4 cells as determined with the Res-Glc in vitro GCase activity assay (n = 3 biological replicates). D, E) Glucosylsphingosine and galactosylsphingosine levels in the GBA1 + /+ and GBA1–/–H4 cells (n = 5 biological replicates).
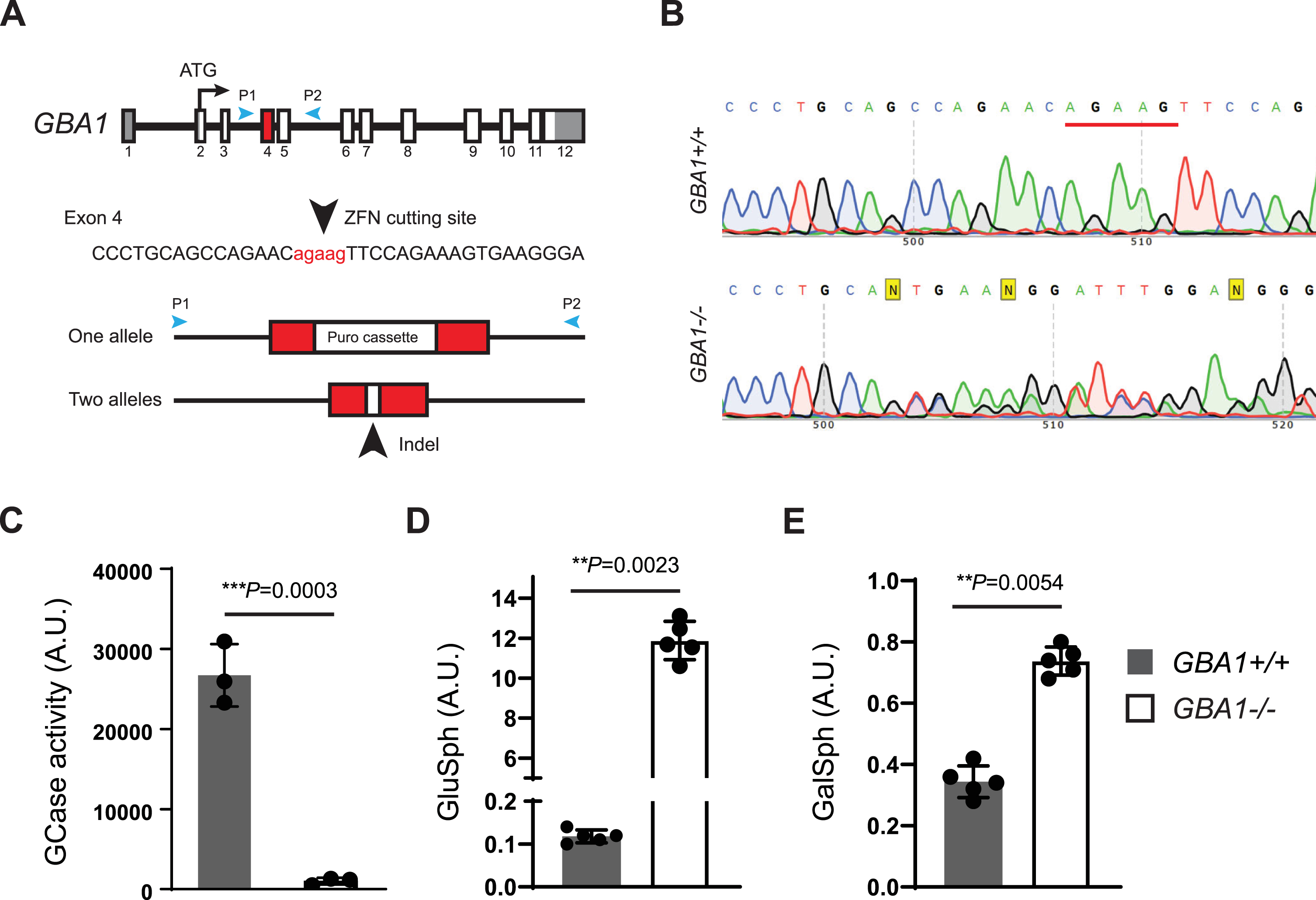
Benchmarking hGCase antibodies in traditional and automated capillary western blot
HGCase-1/17 and hGCase-1/23 were first evaluated by traditional western blotting using GBA1 + /+ and GBA1–/–H4 cell lysates. This assessment compared these new antibodies with three recent commercial hGCase antibodies not yet independently evaluated: mouse monoclonal IgG 812201 (R&D Systems, MAB7410), mouse monoclonal IgG 2E2 (Abcam, ab55080), rabbit monoclonal IgG EPR5143(3) (Abcam, ab128879), and the well-characterized in-house antibody rabbit polyclonal IgG R386 [14] (Table 1). While 2E2, EPR5143, and R386 detected specific hGCase bands in GBA1+ /+, but not GBA1–/–, cells in traditional western blots, 812201, hGCase-1/17, and hGCase-1/23 yielded unsatisfactory results despite extensive optimization (Fig. 2A and data not shown). Subsequently, hGCase antibodies were compared in an automated capillary western blotting system (Simple Western from ProteinSimple, Bio-Techne), which automates the protein separation and immunodetection steps in traditional western blot, thus minimizing error-prone steps. The three commercial antibodies and R386 successfully detected hGCase via automated capillary western blotting, indicating their compatibility with this approach (Fig. 2B). Notably, 812201 produced better results in capillary western blotting than in traditional westerns. However, hGCase-1/17 and hGCase-1/23 failed to detect endogenous hGCase in GBA1 + /+ cells using the capillary western blotting system. Hence, the three commercial hGCase antibodies were better suited for detecting endogenous hGCase in both traditional and capillary western blot. In contrast, hGCase-1/17 and hGCase-1/23 exhibited limited utility in western blot, detecting only overexpressed hGCase in HEK293-F cells (Supplementary Figure 1) but not at endogenous levels (Fig. 2).
Table 1
Antibodies used to detect hGCase by western blotting
hGCase Antibody | Source | Catalog # | Host | Clonality | Compatible Assays |
hGCase-1/17 | Roche &NHGRI | Available through MJFF soon | Mouse | Monoclonal | AlphaLISA, ICC, IP |
hGCase-1/23 | Roche &NHGRI | Available through MJFF soon | Mouse | Monoclonal | AlphaLISA, ICC, IP |
812201 | R&D systems | MAB7410 | Mouse | Monoclonal | Capillary WB |
2E2 | Abnova | H00002629-M01 | Mouse | Monoclonal | Conventional and Capillary WB |
EPR5143 | Abcam | ab128879 | Rabbit | Monoclonal | Conventional and Capillary WB |
R386 | In-house | N/A | Rabbit | Polyclonal | Conventional and Capillary WB |
Fig. 2
Performance of hGCase antibodies in detecting endogenous hGCase in traditional and automated capillary western blotting. A) Traditional western blotting analyzing GBA1 + /+ and GBA1–/–H4 cell lysates with hGCase antibodies 812201, 2E2, EPR5143, R386, hGCase-1/17 and hGCase-1/23. B) Automated capillary western blotting analyzing GBA1 + /+ and GBA1–/–H4 cell lysates with hGCase antibodies. Note that 812201 detected endogenous hGCase in GBA1 + /+ H4 lysate in automated capillary western blotting.
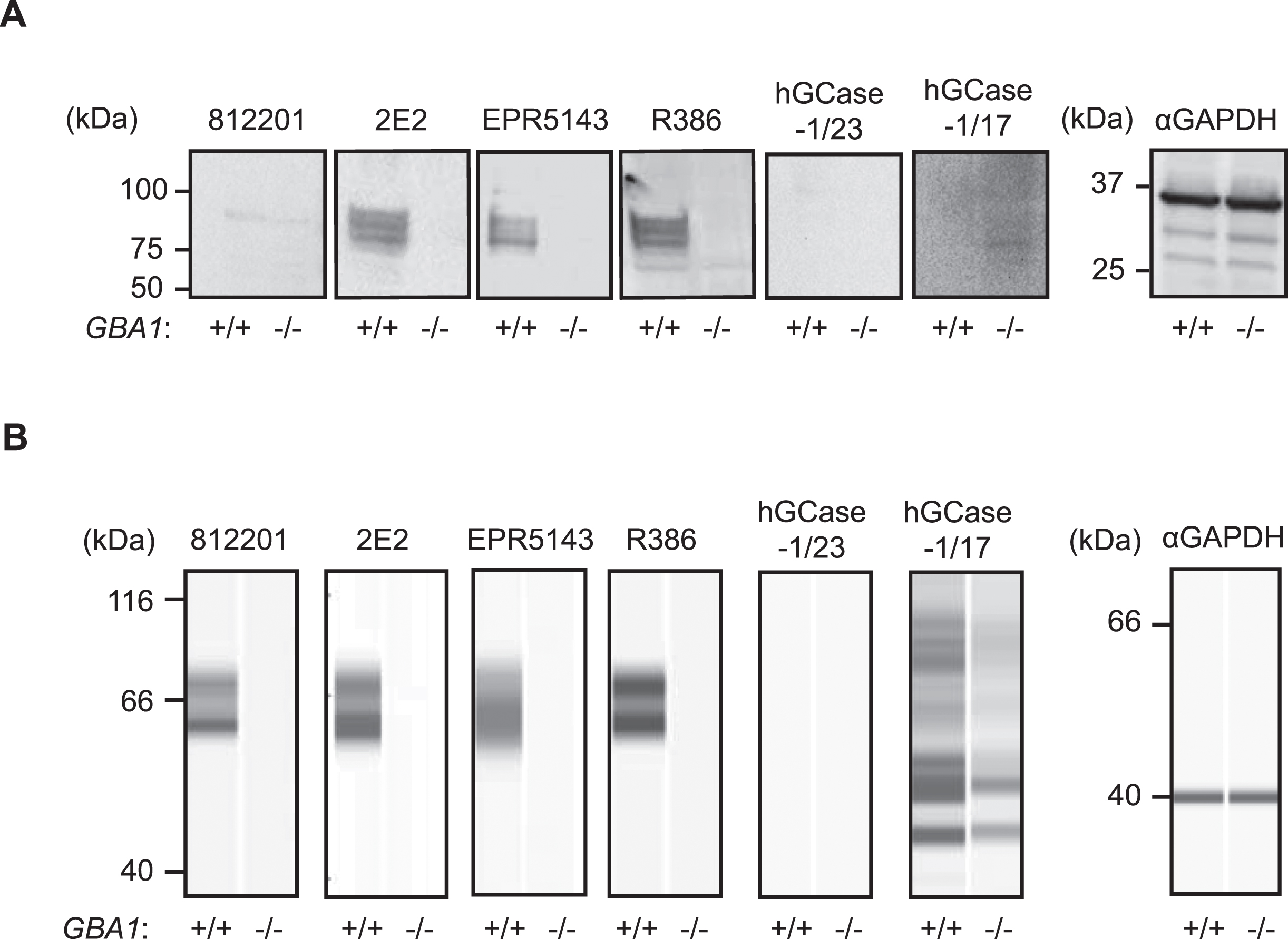
Characterization of hGCase-1/17 and hGCase-1/23 through immunostaining
Among the three commercial hGCase antibodies, 2E2 and 812201 were marketed as suitable for immunostaining assays. However, independent evaluation of 2E2 through immunostaining using GBA1 + /+ and GBA1–/–SH-SY5Y cells yielded unsatisfactory results [15]. Consequently, hGCase-1/17 and hGCase-1/23 were compared with 812201 in immunostaining with paraformaldehyde (PFA)-fixed GBA1 + /+ and GBA1–/–H4 cells. Both hGCase-1/17 and hGCase-1/23 displayed substantial selectivity towards endogenous hGCase, with staining confined to vesicular and tubular lysosomes that co-stained with the lysosomal membrane protein LAMP1, correlating with the expected lysosomal localization of hGCase (Fig. 3A). Impressively, GBA1–/–H4 cells exhibited virtually no background. Although both antibodies were selective towards hGCase, hGCase-1/17 exhibited weaker staining compared to hGCase-1/23, indicating a lower potency (Fig. 3A). Additional assessments of hGCase-1/23 in human neurons differentiated from GBA1 + /+ and GBA1–/–human embryonic stem cell (hESC) lines further affirmed its specificity and potency (Fig. 3B). In contrast, 812201 failed to show specific staining in GBA1 + /+ H4 cells (Supplementary Figure 3). Therefore, hGCase-1/23 emerged as the recommended choice for immunofluorescence assays.
Fig. 3
Specific immunostaining of hGCase in H4 cells and human neurons with hGCase-1/23 and hGCase-1/17. A) GBA1 + /+ and GBA1–/–H4 cells were fixed with 4% PFA, permeabilized with 0.05% Saponin, and stained with hGCase-1/23 and hGCase-1/17, together with a LAMP1 antibody. Scale bars: 10μm, and 1.5μm in inserts. (B) GBA1 + /+ and GBA1–/–human neurons were stained with hGCase-1/23, together with LAMP1 and MAP2 antibodies. Scale bars: 100μm, and 25μm in inserts.
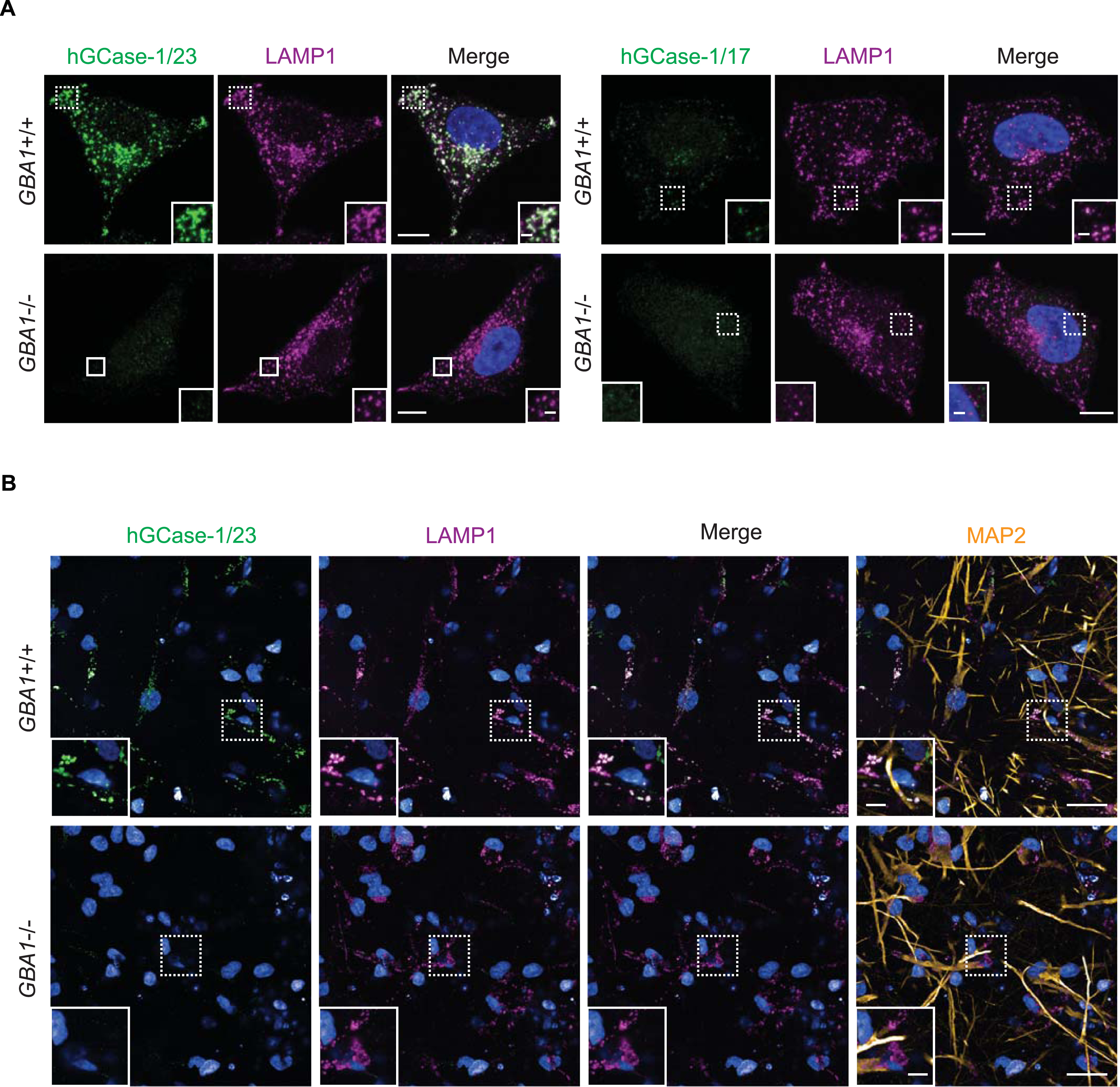
Evaluation of hGCase-1/23 with hGCase mutants through immunostaining
The effectiveness of hGCase-1/23 in immunostaining hGCase wild-type (WT) prompted further evaluation with hGCase mutants N370S and L444P that are common in patients with PD. Human fibroblasts expressing hGCase WT, N370S, and L444P were stained with hGCase-1/23. The antibody successfully detected both hGCase WT and N370S, revealing their presence within lysosomes marked by LAMP1 (Fig. 4, WT/WT and N370S/N370S). While the staining intensity notably decreased in L444P/L444P fibroblasts (Fig. 4, L444P/L444P), there was still a minimal presence of hGCase L444P/L444P detected in lysosomes (Fig. 4, L444P/L444P contrast adjusted). These findings collectively indicate that the epitope recognized by hGCase-1/23 remains largely unaffected by the amino acid changes in hGCase N370S and L444P mutations.
Fig. 4
Immunostaining of hGCase WT, N370S, and L444P with hGCase-1/23. Fibroblasts from a healthy individual (WT/WT) and patients with GD (GBA1: N370S/N370S and L444P/L444P) were fixed with 4% PFA, permeabilized with 0.05% Saponin, and stained with hGCase-1/23 and a LAMP1 antibody. Scale bars: 15μm, and 5μm in inserts.
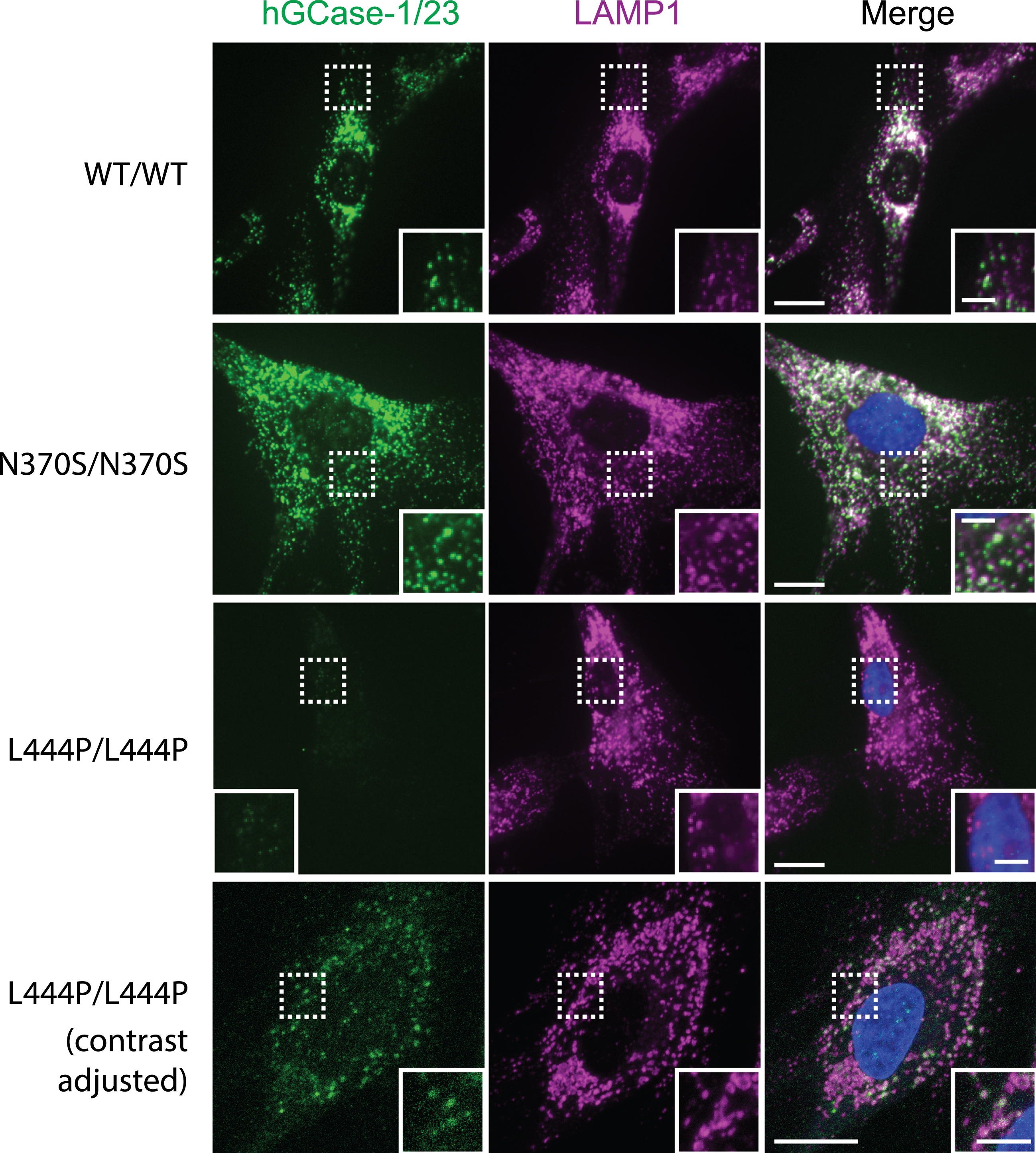
Immunoprecipitation of hGCase using hGCase-1/17 and hGCase-1/23
Capitalizing on their success in recognizing external epitopes of hGCase in immunostaining, hGCase-1/17 and hGCase-1/23 were tested in immunoprecipitation assays for their ability to capture hGCase in cell lysates. Both antibodies effectively pulled down hGCase in GBA1 + /+ H4 cell lysates (Fig. 5A). Notably, hGCase-1/23 demonstrated superior capture efficiency than hGCase-1/17, in line with its superiority in the immunostaining assay (Fig. 5A). To further demonstrate their value in studying proteins interacting with hGCase, hGCase in human neuron lysates was captured with hGCase-1/23. Then, co-immunoprecipitated proteins were examined for the presence of lysosomal integral membrane protein-2 (LIMP-2/SCARB2), the trafficking receptor for hGCase [16–18]. LIMP-2 was co-immunoprecipitated with hGCase by hGCase-1/23 in GBA1 + /+, but not GBA1–/–, neuron lysates, indicating LIMP-2 co-immunoprecipitation relied on its interaction with endogenous hGCase (Fig. 5B).
Fig. 5
Application of hGCase-1/17 and hGCase-1/23 in immunoprecipitation. A) HGCase in H4 cell lysates was immunocaptured with hGCase-1/17 or hGCase-1/23 and immunoblotted with hGCase antibody EPR5143 after elution. B) Co-immunoprecipitation of LIMP2 with hGCase by hGCase-1/23. hGCase in neuron lysates was immunocaptured with hGCase-1/23, and the presence of LIMP2 in GCase co-precipitates was detected with a LIMP2 antibody.
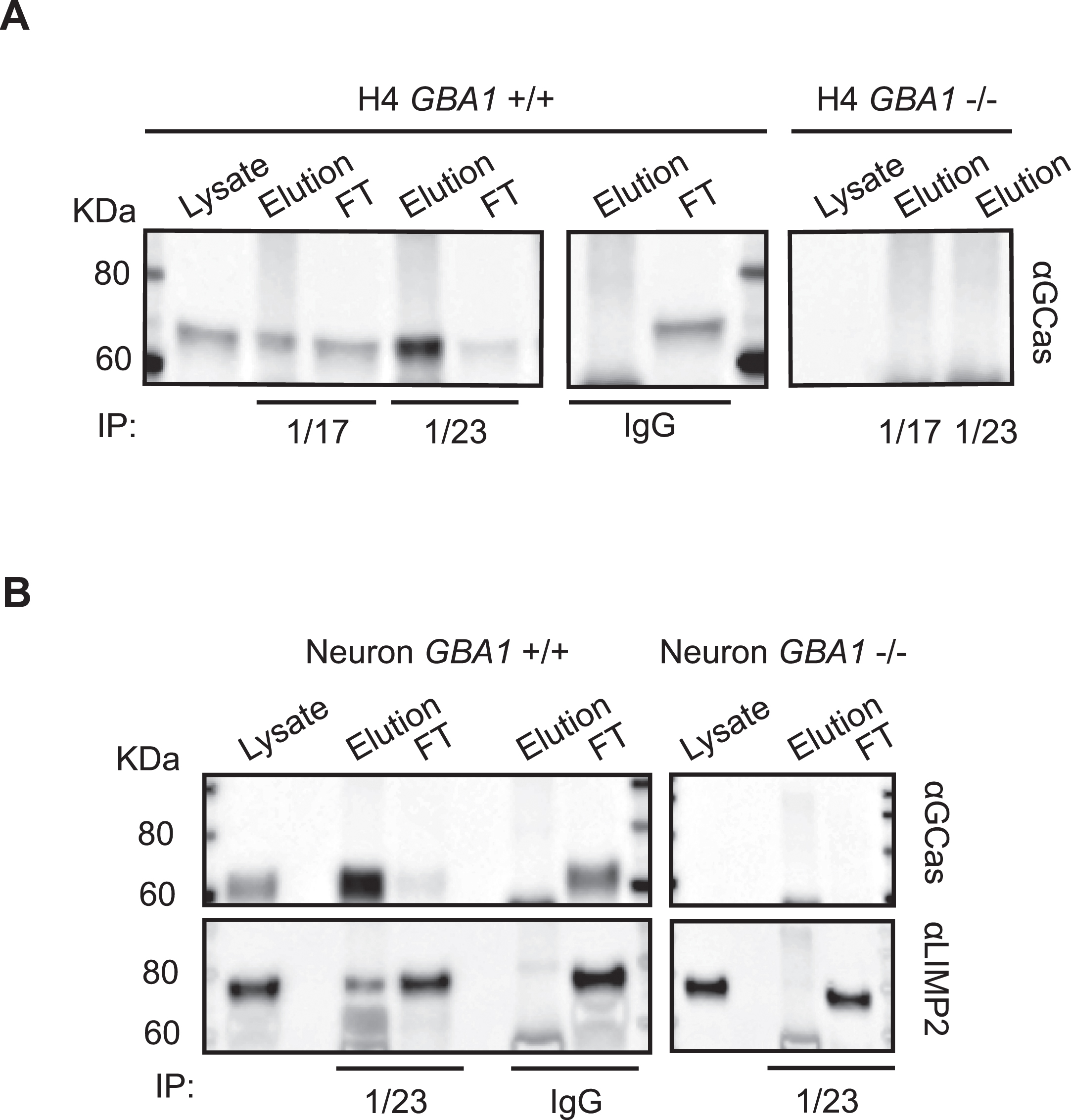
hGCase AlphaLISA assay with hGCase-1/17 and hGCase-1/23
The AlphaLISA (Amplified Luminescent Proximity Homogeneous Assay) is a bead-based luminescent amplification assay developed by PerkinElmer to detect and quantify biomolecules in diverse sample types (Fig. 6A). It offers a streamlined workflow, enhanced sensitivity, expansive dynamic range, and adaptability for high-throughput applications. In the AlphaLISA assay, a standard sandwich configuration employs two distinct antibodies recognizing non-overlapping epitopes on the target molecule. One biotinylated antibody is coupled with an Alpha streptavidin-coated Donor bead, while the other antibody is directly conjugated to the AlphaLISA Acceptor bead. When the analyte is present, the Donor and Acceptor beads come together. Upon excitation with a 680 nm laser, a photosensitizer inside the Donor bead converts ambient oxygen to an excited singlet state. Singlet oxygen diffuses up to 200 nm to produce a chemiluminescent reaction in the Acceptor bead, emitting light at 615 nm proportional to the analyte amount.
Fig. 6
HGCase AlphaLISA assay. A) In the sandwich AlphaLISA assay, hGCase-1/23 was biotinylated and associated with the Alpha Donor Bead, and hGCase-1/17 was directly conjugated to the Alpha Acceptor Bead. B) Sensitivity and dynamic range of hGCase AlphaLISA assay (n = 3 technical replicates). C, D) Determination of hGCase levels in GBA1 + /+ and GBA1–/–H4 cells with hGCase AlphaLISA assay. A 2 h treatment with imiglucerase led to a dose-dependent increase in hGCase levels (C, n = 3 biological replicates; D, n = 4 to 12 technical replicates). E, F) Determination of hGCase levels in GBA1 + /+ and GBA1–/–human neurons with hGCase AlphaLISA assay. A 2 h treatment with imiglucerase led to a dose-dependent increase in hGCase levels (E, n = 3 biological replicates; F, n = 4 to 12 technical replicates).
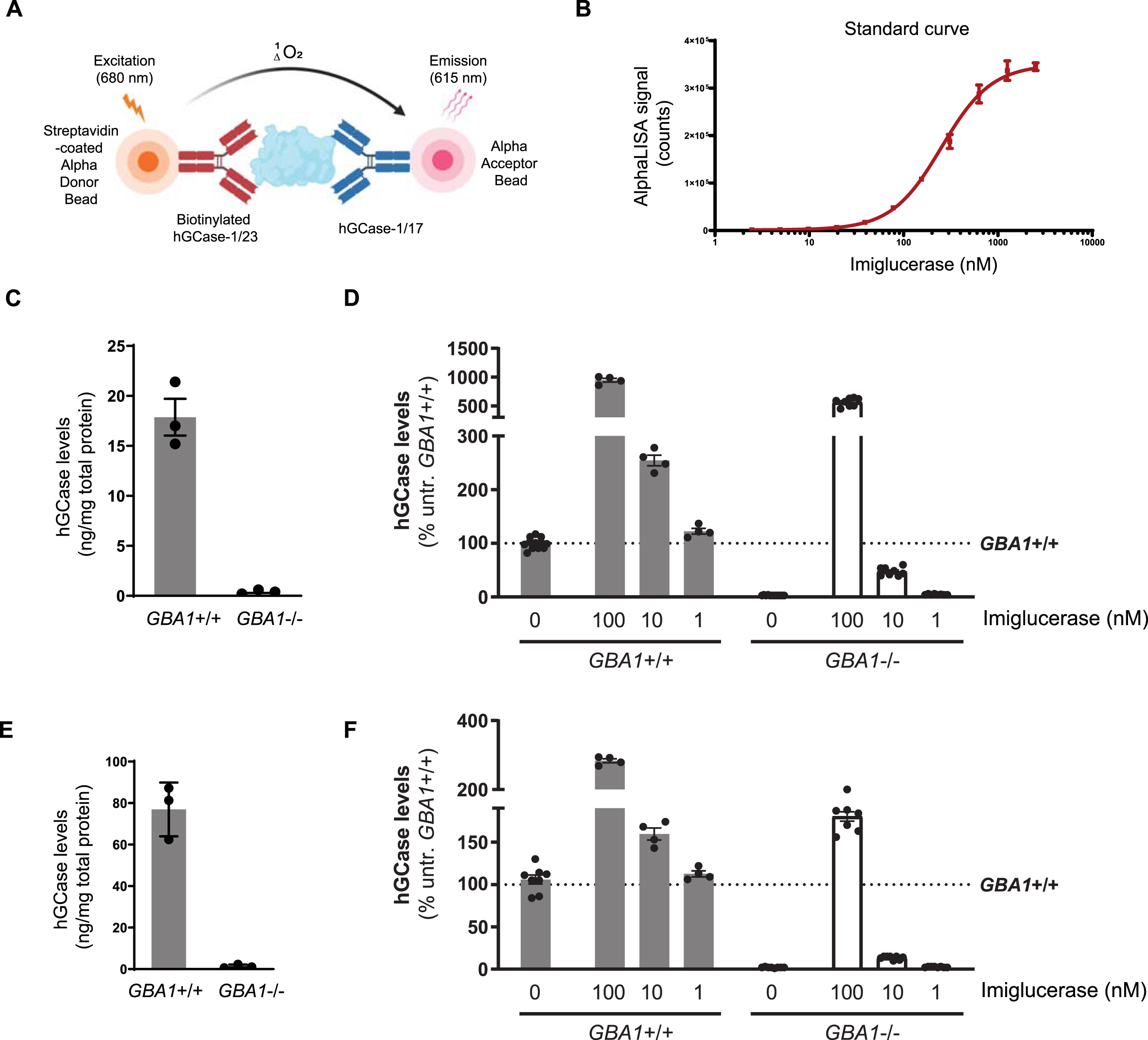
The potential of hGCase-1/17 and hGCase-1/23 for the AlphaLISA assay was explored by generating a dose-response curve with imiglucerase. The AlphaLISA assay utilized a sandwich configuration where hGCase-1/23 was biotinylated and associated with the Alpha Donor Bead, and hGCase-1/17 was directly conjugated to the Alpha Acceptor Bead. This assay effectively quantified imiglucerase levels, exhibiting exceptional sensitivity, broad dynamic range, and substantial signal-to-background ratio (Fig. 6B). With this assay, hGCase protein per milligram of cell lysate was estimated at approximately 20 ng in GBA1 + /+ H4 cells and around 80 ng in GBA1 + /+ neurons, while GBA1–/–H4 or human neuron cell lysates showed no hGCase protein, underscoring the assay’s specificity towards hGCase (Fig. 6C, E). Imiglucerase treatment increased hGCase levels in H4 cells and human neurons, regardless of GBA1 genotype (Fig. 6D, F). This quantitative immuno-based assay has been previously employed to determine hGCase levels in PD and DLB postmortem brains [19].
DISCUSSION
This study unveils the development and characterization of hGCase-1/17 and hGCase-1/23, two mouse monoclonal antibodies with great utility for hGCase immunofluorescence, immunoprecipitation, and AlphaLISA assays. This achievement was made possible by immunizing with imiglucerase (recombinant full length hGCase) instead of peptide fragments, and by screening for selectivity at each stage of hybridoma clone selection. The resulting antibodies exhibited remarkable selectivity for hGCase and potent performance in immunofluorescence, immunoprecipitation, and AlphaLISA assays.
In immunofluorescence assays, hGCase-1/17 and hGCase-1/23 readily detected endogenous hGCase in GBA1 + /+ H4 cells and human neurons, while exhibiting minimal background staining in GBA1–/–cells (Figs. 3 and 4). In immunoprecipitation assays, these antibodies successfully captured endogenous hGCase in both H4 cells and neurons. The co-immunoprecipitation of LIMP2 with hGCase demonstrated the antibodies’ value for investigating potential hGCase interactors (Fig. 5). Importantly, hGCase-1/17 and hGCase-1/23 bind to distinct epitopes on hGCase surface, enabling the development of the hGCase AlphaLISA assay with a sandwich configuration. This assay displayed high sensitivity and a wide dynamic range (Fig. 6). It holds potential for measuring hGCase as a translational biomarker and has already been used to determine GCase protein levels in PD and DLB postmortem brains [19].
Numerous pathological variants in GBA1 compromise the trafficking of hGCase from the ER to lysosomes [20–22], and decreased levels of lysosomal GCase has been proposed to contribute to PD risk [23, 24]. Consequently, hGCase is the therapeutic target in various disease-modifying therapies currently under development for PD. Strategies to restore lysosomal GCase activity include gene therapy to deliver exogenous hGCase [25] and small molecule chaperones to facilitate proper folding and trafficking of hGCase mutants [5, 26–29]. The development of hGCase-1/17 and hGCase-1/23 now provides essential assays for evaluating the effectiveness of these therapeutic approaches in delivering hGCase to lysosomes in future clinical trials.
ACKNOWLEDGMENTS
We would like to thank Jean-Philippe Carralot and Nicole Soder for support with antibody generation, Judith Rothe and Karlheinz Baumann for support with the generation of GBA1–/–cell models, and Iris Ruf for support with lipid measurements.
FUNDING
The work of E.S., Y.C. and T.J. was funded by the Intramural Research Programs of the National Human Genome Research Institute and the National Institutes of Health. Additionally, this research was supported in part by Aligning Science Across Parkinson’s [ASAP-000458] and the Michael J. Fox Foundation for Parkinson’s Research (MJFF-022588). For the purpose of open access, the author has applied a CC-BY public copyright license to all Author Accepted Manuscripts arising from this submission.
CONFLICT OF INTEREST
The authors have no conflict of interest to report.
DATA AVAILABILITY
The data supporting the findings of this study are available within the article and/or its supplementary material.
SUPPLEMENTARY MATERIAL
[1] Supplementary material can be found here: https://dx.doi.org/10.3233/JPD-230295.
REFERENCES
[1] | Rosenbloom BE , Weinreb NJ ((2013) ) Gaucher disease: A comprehensive review. Adv Gauch Dis Basic Clin Perspect 18: , 27–49. |
[2] | Sidransky E , Nalls MA , Aasly JO , Aharon-Peretz J , Annesi G , Barbosa ER , Bar-Shira A , Berg D , Bras J , Brice A , Chen C-M , Clark LN , Condroyer C , De Marco EV , Dürr A , Eblan MJ , Fahn S , Farrer MJ , Fung H-C , Gan-Or Z , Gasser T , Gershoni-Baruch R , Giladi N , Griffith A , Gurevich T , Januario C , Kropp P , Lang AE , Lee-Chen G-J , Lesage S , Marder K , Mata IF , Mirelman A , Mitsui J , Mizuta I , Nicoletti G , Oliveira C , Ottman R , Orr-Urtreger A , Pereira LV , Quattrone A , Rogaeva E , Rolfs A , Rosenbaum H , Rozenberg R , Samii A , Samaddar T , Schulte C , Sharma M , Singleton A , Spitz M , Tan E-K , Tayebi N , Toda T , Troiano AR , Tsuji S , Wittstock M , Wolfsberg TG , Wu Y-R , Zabetian CP , Zhao Y , Ziegler SG ((2009) ) Multicenter analysis of glucocerebrosidase mutations in Parkinson’s disease. N Engl J Med 361: , 1651–1661. |
[3] | Nalls MA , Duran R , Lopez G , Kurzawa-Akanbi M , McKeith IG , Chinnery PF , Morris CM , Theuns J , Crosiers D , Cras P , Engelborghs S , De Deyn PP , Van Broeckhoven C , Mann DMA , Snowden J , Pickering-Brown S , Halliwell N , Davidson Y , Gibbons L , Harris J , Sheerin UM , Bras J , Hardy J , Clark L , Marder K , Honig LS , Berg D , Maetzler W , Brockmann K , Gasser T , Novellino F , Quattrone A , Annesi G , De Marco EV , Rogaeva E , Masellis M , Black SE , Bilbao JM , Foroud T , Ghetti B , Nichols WC , Pankratz N , Halliday G , Lesage S , Klebe S , Durr A , Duyckaerts C , Brice A , Giasson BI , Trojanowski JQ , Hurtig HI , Tayebi N , Landazabal C , Knight MA , Keller M , Singleton AB , Wolfsberg TG , Sidransky E ((2013) ) A multicenter study of glucocerebrosidase mutations in dementia with Lewy bodies. JAMA Neurol 70: , 727–735. |
[4] | Chatterjee D , Krainc D ((2023) ) Mechanisms of glucocerebrosidase dysfunction in Parkinson’s disease: Mechanisms of GBA1-PD. J Mol Biol 435: , 168023. |
[5] | Menozzi E , Schapira AHV ((2020) ) Enhancing the activity of glucocerebrosidase as a treatment for Parkinson disease. CNS Drugs 34: , 915–923. |
[6] | Pitcairn C , Wani WY , Mazzulli JR ((2019) ) Dysregulation of the autophagic-lysosomal pathway in Gaucher and Parkinson’s disease. Neurobiol Dis 122: , 72–82. |
[7] | Qi W , Davidson BA , Nguyen M , Lindstrom T , Grey RJ , Burnett R , Aflaki E , Sidransky E , Westbroek W ((2019) ) Validation of anti-glucocerebrosidase antibodies for western blot analysis on protein lysates of murine and human cells. Biochem J 476: , 261–274. |
[8] | Gündner AL , Meyer CA , Aigner S , Christensen K , Patsch C , Jagasia R , Baumann K , Burcin M ((2017) ) Generation of a homozygous GBA deletion human embryonic stem cell line. Stem Cell Res 23: , 122–126. |
[9] | Chambers SM , Fasano CA , Papapetrou EP , Tomishima M , Sadelain M , Studer L ((2009) ) Highly efficient neural conversion of human ES and iPS cells by dual inhibition of SMAD signaling. Nat Biotechnol 27: , 275–280. |
[10] | Costa V , Aigner S , Vukcevic M , Sauter E , Behr K , Ebeling M , Dunkley T , Friedlein A , Zoffmann S , Meyer CA , Knoflach F , Lugert S , Patsch C , Fjeldskaar F , Chicha-Gaudimier L , Kiialainen A , Piraino P , Bedoucha M , Graf M , Jessberger S , Ghosh A , Bischofberger J , Jagasia R ((2016) ) MTORC1 inhibition corrects neurodevelopmental and synaptic alterations in a human stem cell model of tuberous sclerosis. Cell Rep 15: , 86–95. |
[11] | Pandya NJ , Wang C , Costa V , Lopatta P , Meier S , Zampeta FI , Punt AM , Mientjes E , Grossen P , Distler T , Tzouros M , Martí Y , Banfai B , Patsch C , Rasmussen S , Hoener M , Berrera M , Kremer T , Dunkley T , Ebeling M , Distel B , Elgersma Y , Jagasia R ((2021) ) Secreted retrovirus-like GAG-domain-containing protein PEG10 is regulated by UBE3A and is involved in Angelman syndrome pathophysiology. Cell Rep Med 2: , 100360. |
[12] | Grabowski GA , Barton NW , Pastores G , Dambrosia JM , Banerjee TK , McKee MA , Parker C , Schiffmann R , Hill SC , Brady RO ((1995) ) Enzyme therapy in type 1 Gaucher disease: Comparative efficacy of mannose- terminated glucocerebrosidase from natural and recombinant sources. Ann Intern Med 122: , 33–39. |
[13] | Hill JT , Demarest BL , Bisgrove BW , Su Y , Smith M , Yost HJ ((2014) ) Poly Peak Parser: Method and software for identification of unknown indels using Sanger sequencing of polymerase chain reaction products. Dev Dyn 243: , 1632–1636. |
[14] | Ginns EI , Brady RO , Pirruccello S , Moore C , Sorrell S , Furbish FS , Murray GJ , Tager J , Barranger JA ((1982) ) Mutations of glucocerebrosidase: Discrimination of neurologic and non-neurologic phenotypes of Gaucher disease. Proc Natl Acad Sci U S A 79: , 5607–5610. |
[15] | Kuo SH , Tasset I , Cheng MM , Diaz A , Pan MK , Lieberman OJ , Hutten SJ , Alcalay RN , Kim S , Ximénez-Embún P , Fan L , Kim D , Ko HS , Yacoubian T , Kanter E , Liu L , Tang G , Muñoz J , Sardi SP , Li A , Gan L , Cuervo AM , Sulzer D ((2022) ) Mutant glucocerebrosidase impairsα-synuclein degradation by blockade of chaperone-mediatedautophagy. , eabm. Sci Adv 8: , 6393. |
[16] | Hughes H , Blanz J , Jin X , Reczek D , Schwake M , Schro J , Brondyk W , Patten Van S , Edmunds T , Saftig P ((2007) ) LIMP-2 is a receptor for lysosomal targeting of b-glucocerebrosidase. Cell 2: , 770–783. |
[17] | Zunke F , Andresen L , Wesseler S , Groth J , Arnold P , Rothaug M , Mazzulli JR , Krainc D , Blanz J , Saftig P , Schwake M ((2016) ) Characterization of the complex formed by β-glucocerebrosidase and the lysosomal integral membrane protein type-2. Proc Natl Acad Sci U S A 113: , 3791–3796. |
[18] | Zhao Y , Ren J , Padilla-Parra S , Fry EE , Stuart DI ((2014) ) Lysosome sorting of β-glucocerebrosidase by LIMP-2 is targeted by the mannose 6-phosphate receptor. Nat Commun 5: , 4321. |
[19] | Gündner AL , Duran-Pacheco G , Zimmermann S , Ruf I , Moors T , Baumann K , Jagasia R , van de Berg WDJ , Kremer T ((2019) ) Path mediation analysis reveals GBA impacts Lewy body disease status by increasing α-synuclein levels. Neurobiol Dis 121: , 205–213. |
[20] | Ron I , Horowitz M ((2005) ) ER retention and degradation as the molecular basis underlying Gaucher disease heterogeneity. Hum Mol Genet 14: , 2387–2398. |
[21] | Yang C , Wang H , Zhu D , Hong CS , Dmitriev P , Zhang C , Li Y , Ikejiri B , Brady RO , Zhuang Z ((2015) ) Mutant glucocerebrosidase in Gaucher disease recruits Hsp27 to the Hsp90 chaperone complex for proteasomal degradation. Proc Natl Acad Sci U S A 112: , 1137–1142. |
[22] | Lu J , Chiang J , Iyer RR , Thompson E , Kaneski CR , Xu DS , Yang C , Chen M , Hodes RJ , Lonser RR , Brady RO , Zhuang Z ((2010) ) Decreased glucocerebrosidase activity in Gaucher disease parallels quantitative enzyme loss due to abnormal interaction with TCP1 and c-Cbl. Proc Natl Acad Sci U S A 107: , 21665–21670. |
[23] | Wallings RL , Humble SW , Ward ME , Wade-Martins R ((2019) ) Lysosomal dysfunction at the centre of Parkinson’s disease and frontotemporal dementia/amyotrophic lateral sclerosis. Trends Neurosci 42: , 899–912. |
[24] | Gegg ME , Menozzi E , Schapira AHV ((2022) ) Glucocerebrosidase-associated Parkinson disease: Pathogenic mechanisms and potential drug treatments. Neurobiol Dis 166: , 105663. |
[25] | Abeliovich A , Hefti F , Sevigny J ((2021) ) Gene therapy for Parkinson’s disease associated with GBA1 mutations. J Parkinsons Dis 11: , S183–S188. |
[26] | Yang SY , Taanman JW , Gegg M , Schapira AHV ((2022) ) Ambroxol reverses tau and α-synuclein accumulation in a cholinergic N370S GBA1 mutation model. Hum Mol Genet 31: , 2396–2405. |
[27] | Mazzulli JR , Zunke F , Tsunemi T , Toker NJ , Jeon S , Burbulla LF , Patnaik S , Sidransky E , Marugan JJ , Sue CM , Krainc D ((2016) ) Activation of β-glucocerebrosidase reduces pathological α-synuclein and restores lysosomal function in Parkinson’s patient midbrain neurons. J Neurosci 36: , 7693–7706. |
[28] | Aflaki E , Borger DK , Moaven N , Stubblefield BK , Rogers SA , Patnaik S , Schoenen FJ , Westbroek W , Zheng W , Sullivan P , Fujiwara H , Sidhu R , Khaliq ZM , Lopez GJ , Goldstein DS , Ory DS , Marugan J , Sidransky E ((2016) ) A new glucocerebrosidase chaperone reduces α-synuclein and glycolipid levels in iPSC-derived dopaminergic neurons from patients with Gaucher disease and Parkinsonism. J Neurosci 36: , 7441–7452. |
[29] | Goldin E , Zheng W , Motabar O , Southall N , Choi JH , Marugan J , Austin CP , Sidransky E ((2012) ) High throughput screening for small molecule therapy for Gaucher disease using patient tissue as the source of mutant glucocerebrosidase. PLoS One 7: , 29861. |