Feasibility and Proof-of-Concept of Delivering an Autonomous Music-Based Digital Walking Intervention to Persons with Parkinson’s Disease in a Naturalistic Setting
Abstract
Background:
Reduced motor automaticity in Parkinson’s disease (PD) negatively impacts the quality, intensity, and amount of daily walking. Rhythmic auditory stimulation (RAS), a clinical intervention shown to improve walking outcomes, has been limited by barriers associated with the need for ongoing clinician input.
Objective:
To assess the feasibility, proof-of-concept, and preliminary clinical outcomes associated with delivering an autonomous music-based digital walking intervention based on RAS principles to persons with PD in a naturalistic setting.
Methods:
Twenty-three persons with PD used the digital intervention independently for four weeks to complete five weekly 30-minute sessions of unsupervised, overground walking with music-based cues. The intervention progressed autonomously according to real-time gait sensing. Feasibility of independent use was assessed by examining participant adherence, safety, and experience. Intervention proof-of-concept was assessed by examining spatiotemporal metrics of gait quality, daily minutes of moderate intensity walking, and daily steps. Preliminary clinical outcomes were assessed following intervention completion.
Results:
Participants completed 86.4% of sessions and 131.1% of the prescribed session duration. No adverse events were reported. Gait speed, stride length, and cadence increased within sessions, and gait variability decreased (p < 0.05). Compared to baseline, increased daily moderate intensity walking (mean Δ= +21.44 minutes) and steps (mean Δ= +3,484 steps) occurred on designated intervention days (p < 0.05). Quality of life, disease severity, walking endurance, and functional mobility were improved after four weeks (p < 0.05).
Conclusions:
Study findings supported the feasibility and potential clinical utility of delivering an autonomous digital walking intervention to persons with PD in a naturalistic setting.
INTRODUCTION
Parkinson’s disease (PD) gradually disables walking across multiple levels of human functioning. Dopamine loss in the basal ganglia disrupts motor automaticity, rhythmicity, and drive, [1, 2] subsequently manifesting as slower (bradykinetic) walking speed, reduced (hypokinetic) step length, and poorer walking economy [3–5]. Declines in daily minutes of moderate intensity walking and steps appear early in the disease process [6–9] and initiate a cycle of disability that can perpetuate a loss of independence with daily activities [10] and increased fall risk [11, 12]. Consequently, improving walking is a top priority for enhancing quality of life in persons with PD [13] and an important focus of contemporary research [14].
Rhythmic auditory stimulation (RAS) is a rehabilitation intervention that capitalizes on the human capacity to extract rhythm and synchronize movements to a beat (i.e., auditory motor entrainment) [15]. Entrainment generates an anticipatory time sequence that minimizes energy expenditure, assists in the normalization of gait patterns, and increases the capacity to maintain a steady and more stable walking rhythm [15–20]. Despite impaired motor automaticity, persons with PD maintain the ability to entrain walking cadence to rhythmic auditory cues by recruiting cerebello-thalamo-cortical motor pathways [21, 22], suggestive of an available bypass mechanism for improving walking ability. When used as a therapeutic intervention for persons with PD, RAS promotes increased stride length, improved muscle activation timing, and reduced step variability, resulting in improved gait rhythmicity and increased walking speeds [17, 18, 23–25]. These benefits may be advantageous for efforts to routinely walk at higher intensities, which may have disease modifying effects [26, 27], and thereby slow the progression of disability by preserving or improving walking capacity [6, 28–30].
While clinical studies support RAS-based intervention in PD [25, 31–34], its widespread deployment has been limited due to practical barriers associated with in-person clinical care (e.g., access to rehabilitation services, transportation, availability of clinician expertise, scheduling) [35, 36]. Perhaps more importantly, its primary dosing requirement (i.e., the frequent modulation of tempo based on user response) is difficult to meet when patients are seen intermittently in an outpatient setting. To address these limitations, an autonomous, music-based digital walking intervention based on RAS principles (MR-005, MedRhythms Inc., Portland, ME, USA) is being developed to be used independently in naturalistic settings by persons with PD. Unlike RAS protocols that require frequent clinician input to personalize and modulate stimulation tempo, the intervention utilizes a closed loop protocol that autonomously detects each user’s unique gait characteristics in real-time, adjusts auditory cues accordingly, and progresses to different phases of tempo without additional user or clinician input. With its portability and potentially widespread future availability, the intervention offers a promising, scalable approach for overcoming barriers associated with in-person care.
The aims of this exploratory study were 1) to investigate the feasibility of delivering the MR-005 walking intervention for four weeks in an unsupervised, naturalistic setting to persons with PD, and 2) to examine its proof-of-concept as an intervention for impacting gait quality (spatiotemporal characteristics), daily minutes of moderate intensity walking, and daily steps. In addition, we examined preliminary changes in disease severity, quality of life, and walking capacity after four-weeks of intervention use.
MATERIALS AND METHODS
Study design
The study was designed as a single-group pilot clinical trial involving participants recruited through clinical centers at Boston University (BU) and Johns Hopkins University (JHU). All study procedures were approved by BU and JHU Institutional Review Boards. Written informed consent, eligibility screening, baseline data collection, issuing of an activity monitor, and walking intervention training occurred during an initial in-person clinic visit with a member of the research team (Fig. 1A). Participants subsequently used the intervention independently for four weeks of unsupervised overground walking sessions conducted in a community environment of their choosing. Following intervention completion, participants returned for a final, in-person assessment of preliminary clinical outcomes. The study flow is depicted in Fig. 1B.
Fig. 1
A) The MR-005 system included a touchscreen device preloaded with music, bone conduction heaphones, and shoe-mounted movement sensors. The baseline visit included instruction on device operation. B) Study flow. C) Exemplar entrainment graph displaying a participant’s cadence relative to music tempo.
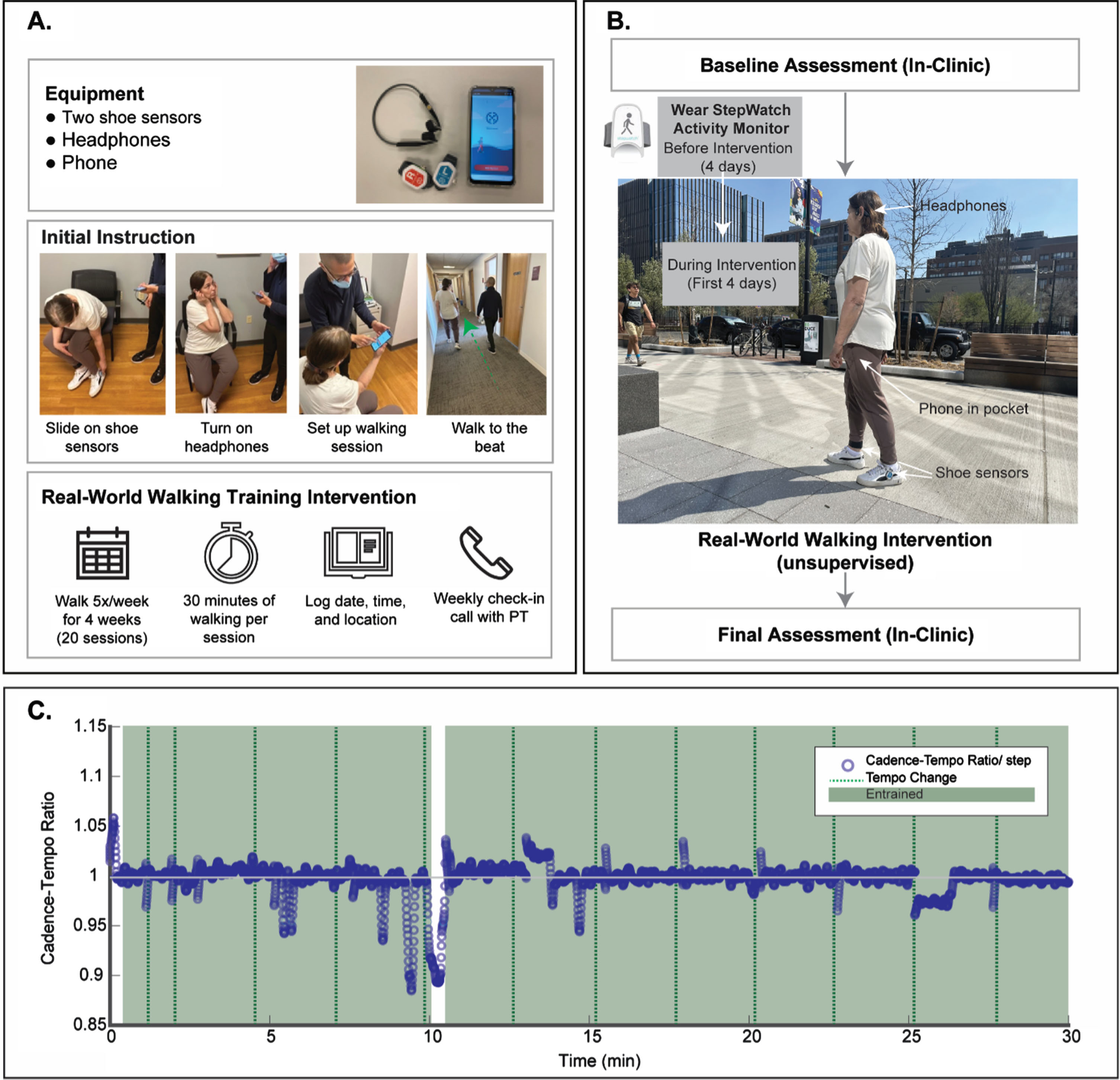
Participants
Study inclusion criteria included a diagnosis of idiopathic PD according to UK Brain Bank Criteria [37], mild to moderate PD severity (H&Y stages 1–3), and the ability to walk independently without physical assistance. Exclusion criteria included the presence of moderately or significantly disturbing freezing of gait episodes during daily walking, cognitive impairment (i.e., MoCA [Montreal Cognitive Assessment] score <24), the inability to walk at a comfortable speed ≥0.4 m/s, the inability to independently use the MR-005 walking intervention, self-reported significant hearing impairment, current participation in physical therapy, or the presence of cardiac or orthopedic conditions that limited the ability to safely walk in the community.
Walking intervention
The MR-005 system included a proprietary software mobile application loaded onto a locked touchscreen device utilizing an Android operating system, two foot sensors that measure gait in three dimensions, a headset, and charging equipment (Fig. 1). The system was designed to be easy for participants to use without supervision and only required interaction with the touchscreen while walking to adjust the music volume, or to pause, resume, and end a session early. Touchscreen interaction was not required to set or modulate rhythmiccues.
The MR-005 system leveraged auditory motor entrainment to deliver an intervention based on the principles of RAS. In general, the software trained participants to produce a symmetrical gait pattern with minimal temporal variability. A typical walking session began with a brief period of system calibration, during which a participant was prompted by the system to walk at a comfortable pace without rhythmic cues while foot sensors collected gait data from approximately 20 strides. Once calibration was complete, music was initiated at a tempo corresponding to the participant’s natural cadence. Throughout the remainder of the session, the software algorithm continuously examined gait from sensor input and progressively adapted music tempo and rhythmic prominence features based on two components. The first component assessed participant auditory motor entrainment based on the alignment of walking cadence to music tempo. Although designed to promote entrainment, the algorithm also accommodated for periodic states outside of entrainment which may naturally occur (e.g., during tempo progressions). The second component monitored gait symmetry and step-to-step temporal variability to enhance safe walking characteristics. See Fig. 1C for a representative example of entrainment periods based on a participant’s cadence relative to the music tempo.
Participants were instructed to engage in five, 30-minute overground walking sessions per week for four weeks using the MR-005 system (i.e., 20 total intervention sessions). Instructions included recommendations for appropriate locations to walk without encountering substantial obstacles or safety hazards (e.g., in a walkable neighborhood; on an indoor or outdoor track). Participants were provided with an activity log to document the dates, times, and locations of the walking sessions and advised to take rest breaks as needed (Fig. 1). Participants received a weekly phone call from a member of the research team to ensure that the MR-005 system was functioning properly.
Measures
Feasibility
Participant adherence with the intervention protocol was examined by extracting session data from the MR-005 software application. Two adherence metrics were used: the mean number of walking sessions completed and the mean duration of walking sessions. Participant safety was examined using study diaries, in which participants were instructed to record the incidence of any adverse events (e.g., trips and falls) occurring during walking sessions. Participant experience using the intervention (e.g., ease of use; confidence in using) was examined with a 26-item self-report usability questionnaire [38] consisting of positively and negatively directed statements. Participants responded to each statement using a 3-item Likert scale (disagree, neutral, agree). Negatively directed questions were scored in the opposite direction, with “disagree” being the more optimal response. The frequency of responses in each Likert scale category (disagree, neutral, agree) was calculated for each question.
Proof-of-concept
Spatiotemporal metrics of gait quality were extracted from MR-005 foot sensor data collected during each walking session. The metrics included gait speed (m/s), stride length (m), cadence (steps/min), gait symmetry (interlimb swing time ratio), and gait variability (coefficient of variation (%) of stride-by-stride gait cycle duration). Daily minutes of moderate intensity walking and daily steps were captured using a StepWatch 4 Activity Monitor (SAM; Orthocare Innovations, Mountlake Terrace, WA), which participants attached above the lateral malleolus of their less impaired lower extremity using Velcro® straps. The leg of attachment for each participant was determined by the research team during the initial in-clinic visit using the Movement Disorders Society –Unified Parkinson’s Disease Rating Scale (MDS-UPDRS) Part III (motor) score [39]. Participants were instructed to wear the SAM for eight consecutive days during all waking hours (except when bathing or swimming). For the first four “baseline” days, participants were instructed to participate in their customary daily activities. For the next four days, participants were instructed to engage in their customary daily activities, and in addition, to begin the four-week walking intervention protocol. Beyond the 8th day, participants continued the intervention protocol without wearing the SAM. Rather than measuring true stride rate (i.e., cadence), the SAM counted the number of strides accumulated during each minute of recording, which may or may not have involved continuous walking or consistent cadence. A minute of moderate intensity walking was operationally defined according to an established threshold [40] as one containing at least 50 strides (i.e., 100 steps). The validity and reliability of the SAM for capturing stride counts have been demonstrated in persons with various neurologic disorders including PD [41–47].
Clinical outcomes
Preliminary clinical outcome measures were collected during the initial in-person clinic visit and following completion of the four-week walking intervention. The 39-item Parkinson’s Disease Questionnaire (PDQ-39) was used to assess health-related quality of life across 8 dimensions of functioning and well-being [48]. Both the PDQ-39 total score and PDQ-39 mobility dimension score were used in subsequent analysis. The MDS-UPDRS part III (motor) score was used as an indicator of disease severity [39]. The Six-Minute Walk Test (6MWT), which measured the maximum distance a person can walk in 6 minutes, was used as an indicator of walking endurance [49]. The Ten Meter Walk Test (10MWT) was used to measure comfortable-pace and fast-pace gait speed, [49] with the mean of two trials serving as variables of interest. The Five Times Sit to Stand Test (FTSTST), which measured the time taken to stand up and sit down five times as quickly as possible without arm support, was used to capture functional lower extremity strength and mobility during a transitional movement related to walking [50].
Statistical analysis
Study data were stored in a Research Electronic Data Capture (REDCap) database [51–53]. Statistical analyses were conducted using SPSS statistical software program version 26.0 (IBM Corp, Armonk, New York). Sample characteristics, feasibility metrics, and clinical outcomes were summarized descriptively. Some foot sensor, SAM, and clinical outcome data were missing (see Supplementary Material for details).
To examine the potential impact of the intervention on gait quality, we calculated mean values for each spatiotemporal parameter during four time intervals within each walking session: the initial, uncued MR-005 calibration period and early, middle, and late periods of cued walking created by partitioning sessions into thirds. We opted to examine time-normalized intervals (i.e., thirds) instead of absolute time intervals (e.g., every 5 minutes), because total walking duration varied across participants and sessions. Sample-wide mean values for each metric subsequently were calculated for each time period. Repeated measures ANOVA were used to compare gait quality during cued periods (i.e., early, middle, late) with gait quality during the uncued calibration period. For each metric, post-hoc multiple comparisons with Bonferonni corrections were used to examine pairwise differences between periods.
In a separate analysis, we used multiple linear regression analysis to examine the movement strategies with which participants might alter their gait speed during sessions in response to MR-005 tempo progressions. Regression variables for change in stride length, cadence, and speed were created first by calculating the difference, for each participant, between 1) the first minute of cued walking when music tempo was close to baseline walking cadence, and 2) the middle and late third of the session when participants were more likely to have acclimated to tempo progressions. Mean change values were calculated across the sample. Stride length and cadence change variables were entered together into the regression analyses to obtain standardized beta coefficients pertaining to their relative contributions to gait speed modulation.
The number of strides recorded by the SAM during each minute was doubled to generate the corresponding number of steps. Total daily minutes of moderate intensity walking (i.e., the number of minutes containing at least 100 steps) and daily steps were calculated for each participant on each recording day (12:00AM–11:59PM). Paired t-tests (α= 0.05) were used to compare sample mean daily values at baseline (SAM wearing days 1–4) with mean daily values recorded after the intervention protocol had begun (SAM wearing days 5–8). Only days on which walking intervention sessions occurred were included in the analysis. Moderate intensity minutes and steps accumulated during walking sessions were identified by mapping SAM and MR-005 timestamps.
To assess clinical outcomes following completion of the four-week protocol, we used paired t-tests (α= 0.05) to compare baseline and post-intervention values for quality of life (PDQ-39 total score; PDQ-39 mobility dimension score), disease severity (UPDRS Part III score), walking endurance (6MWT distance), comfortable and fast gait speed (mean 10MWT values), and functional mobility (FTSTS time).
RESULTS
Twenty-three participants completed the study, 12 from BU and 11 from JHU. The full sample included older adults with mild to moderate PD (Table 1). Given the baseline values for mean daily step count, 6MWT distance, 10MWT gait speeds, and FTSTS time, the sample was considered “somewhat active” according to an established criterion [40] and relatively high functioning (Table 1). Although some between-site differences in sample characteristics and baseline measures were observed, none were statistically significant.
Table 1
Baseline Characteristics (n = 23)
Characteristics | Full Sample (n = 23) | BU (n = 12) | JHU (n = 11) | p |
Demographics | ||||
Age (y)* | 66.91 (8.78) | 66.58 (10.39) | 67.27 (7.13) | 0.856 |
Gender** | ||||
Male | 17 (73.9) | 7 (58.3) | 10 (90.9) | 0.082 |
Female | 6 (26.1) | 5 (41.7) | 1 (9.1) | |
Education** | 0.522 | |||
K-8 | 1 (4.3) | 1 (8.3) | 0 (0) | |
Some high school (9–12)/GED | 2 (8.7) | 2 (16.7) | 0 (0) | |
Some college or trade school | 2 (8.7) | 0 (0) | 2 (18.2) | |
College degree (Associates) | 18 (78.3) | 9 (75) | 9 (81.8) | |
Disease Severity | ||||
MoCA* | 26.52 (1.62) | 26.92 (1.51) | 26.09 (1.70) | 0.230 |
MDS-UPDRS III* | 34.62 (9.28) | 35.83 (9.41) | 33 (9.41) | 0.503 |
Modified Hoehn &Yahr** | ||||
2 | 20 (87) | 10 (83.3) | 10 (90.9) | 0.598 |
2.5 | 3 (13) | 2 (16.7) | 1 (91) | |
Clinical Outcome Measures | ||||
Walking Capacity (n = 21)* | ||||
10MWT Comfortable (m/s) | 1.25 (0.21) | 1.24 (0.20) | 1.25 (0.23) | 0.902 |
10MWT Fast (m/s) | 1.64 (0.23) | 1.58 (0.23) | 1.72 (0.21) | 0.169 |
6MWT (m) | 456.67 (96.71) | 462.06 (120.13) | 449.48 (58.66) | 0.776 |
FTSTS (s) (n = 20)* | 11.36 (3.07) | 10.67 (2.17) | 12.40 (4.02) | 0.226 |
PDQ39 (n = 21)* | ||||
Mobility Score | 15.5 (19.69) | 15.83 (18.5) | 15.06 (22.31) | 0.931 |
Total Score | 17.11 (16.23) | 17.46 (16.93) | 16.63 (16.24) | 0.910 |
Real-World Outcome Measures | ||||
Walking Activity (n = 16)* | ||||
Daily Steps | 8777 (4000) | 9662 (4505) | 7505 (3006) | 0.287 |
Moderate Intensity Minutes | 16.75 (20.01) | 17.55 (23.10) | 15.61 (16.28) | 0.851 |
*Mean (SD); **Frequency count (% of sample or subgroup); MoCA, Montreal Cognitive Assessment; MDS-UPDRS III, Modified Unified Parkinson’s Disease Rating Scale Part III; 10MWT, 10-meter Walk Test; 6MWT, 6-Minute Walk Test; FTSTS, Five Times Sit to Stand Test; PDQ39, Parkinson’s Disease Questionnaire-39.
Feasibility
Participants demonstrated adherence to the intervention protocol by completing a mean of 17.2 (86.4%) of 20 total sessions. Mean session length (39.0 minutes) was 131.1% of the expected 30-minute duration (Fig. 2). No adverse events (e.g., trips or falls) were reported. After four weeks, most participants reported positive experiences with the walking intervention (Fig. 3). For example, 20 out of 23 (87%) participants reported that the MR-005 system was easy to use, and all 23 participants (100%) reported being confident in their ability to use it.
Fig. 2
Participant adherence. Mean sessions completed appears along the left y-axis, with the target of 20 sessions indicated by the blue line. Mean session duration appears along the right y-axis, with the target of 30-minute sessions indicated by the orange line.
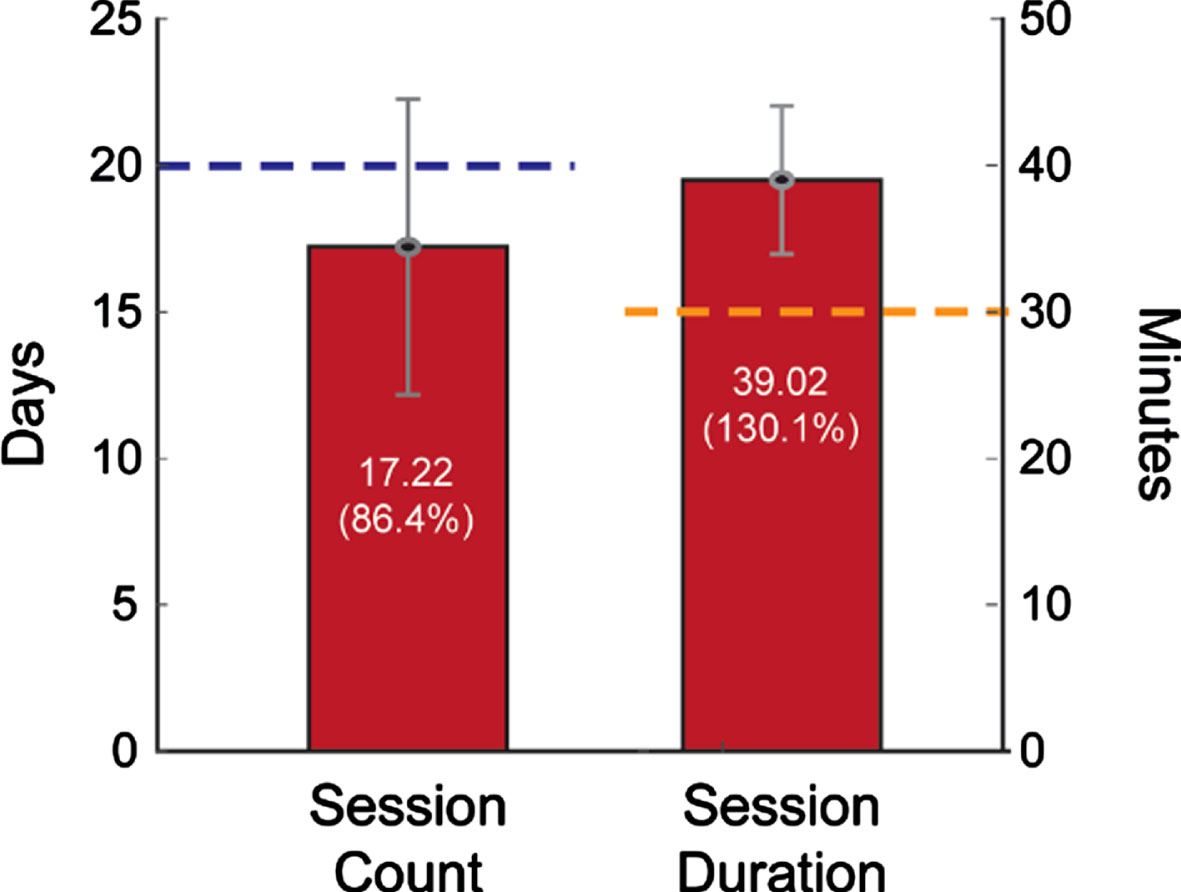
Fig. 3
Usability Scale Results (n = 23). Participants completed the questionnaire following completion of the four-week walking intervention.
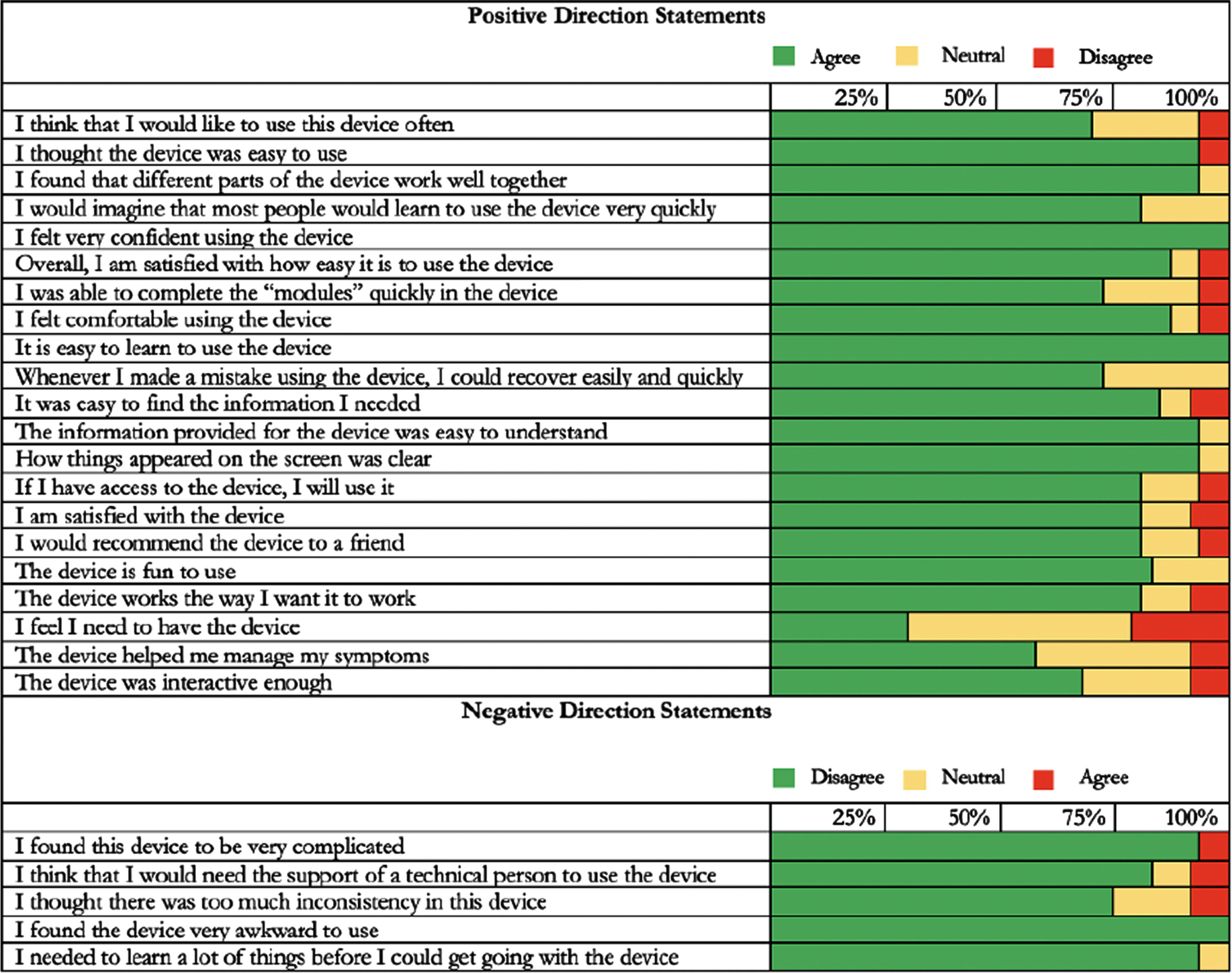
Proof-of-concept
Based on participant performance, MR-005 generally delivered tempos greater than 100 beats/min for most minutes of each session (mean(SD) = 35.26 (9.96) minutes). In turn, participants generated relatively high cadences during rhythmic cueing (mean(SD) = 114 (12) steps/min). Compared to uncued walking during the brief calibration period, participants displayed generally improved gait quality during early, middle, and late periods of cued walking (Fig. 4). Specifically, participants walked faster (Δ= Early: +0.13 (0.6) m/s; Middle: +0.12 (0.5) m/s, Late: +0.07 (0.07) m/s; p < 0.001), took longer strides (Δ= Early: +0.08 (0.04) m; Middle: +0.08 (0.04) m, Late: +0.04 (0.06) m/s; p < 0.001), walked at higher cadences (Δ= Early: +3.31 (2.39) steps/min; Middle: +2.43 (2.6) steps/min, Late: 1.33 (2.90) steps/min; p < 0.001), and had lower gait variability (Δ= Early: –5.58 (2.50)%; Middle: –6.11 (2.06)%, Late: –4.39 (2.49) %; p < 0.001). Only gait symmetry remained unchanged (p > 0.05) from its uncued value (interlimb swing time ratio = 1.02 (0.01)), which indicated minimal gait asymmetry across the sample.
Fig. 4
Within-session effects of MR-005 on gait quality
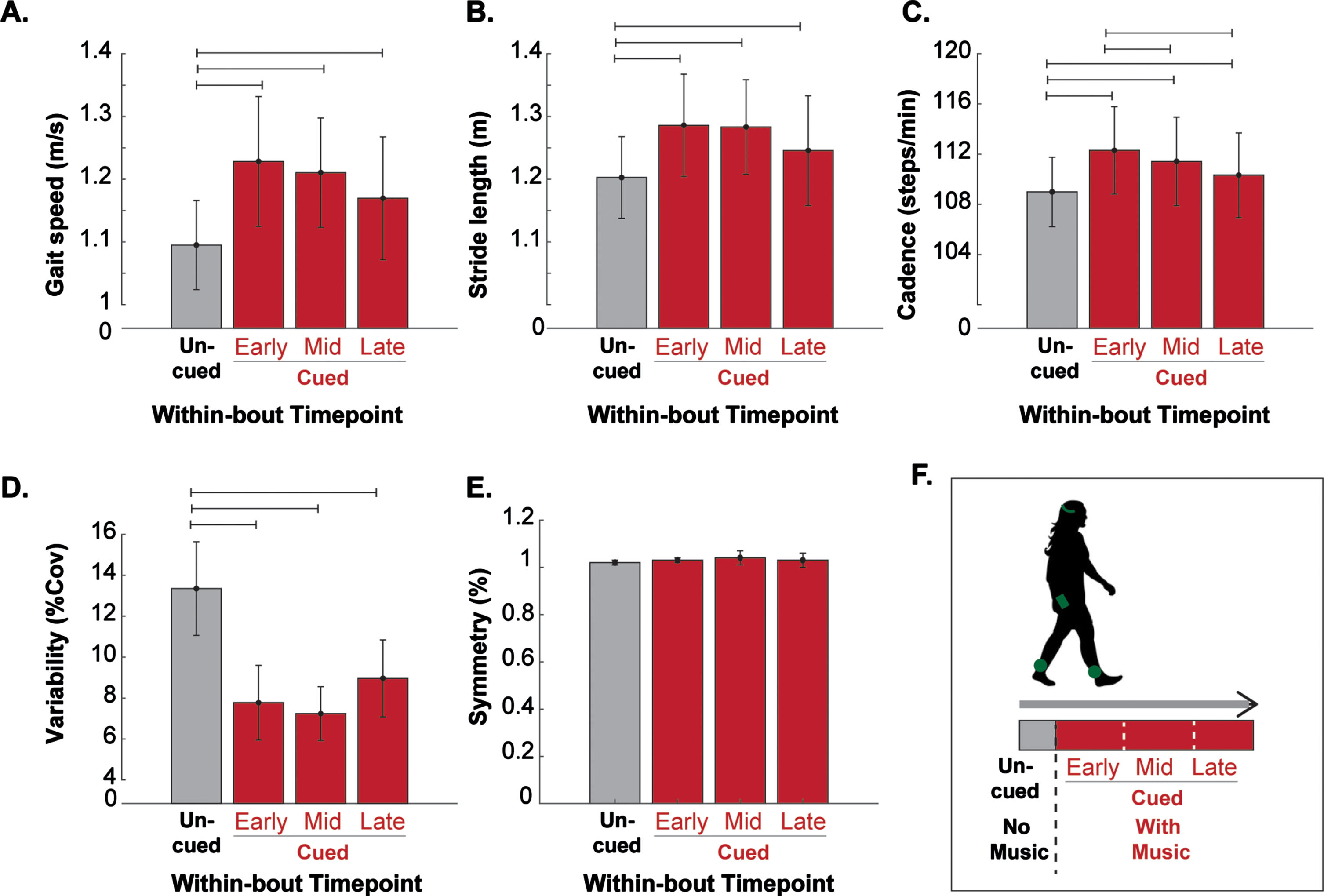
Changes in gait speed were observed during tempo progressions within sessions. Compared to the first minute of cued walking during the early period, participants increased their gait speed by 0.07 (0.04) m/s during the middle period and by 0.03 (0.05) m/s during the late period. Standardized beta values generated from multiple regression analyses revealed that both increases in gait speed were more strongly related to changes in stride length than cadence (Table 2).
Table 2
Speed modulation while walking with MR-005 at Mid and Late bout
Model Outcome, | R-Square | F-Value | Overall | Standardized | Coefficient |
predictors | model | coefficients | |||
P-value | P-Value | ||||
Mid Bout | |||||
Speed | 0.94 | 130.93 | < 0.001 | ||
Stride length | 0.69 | < 0.001 | |||
Cadence | 0.46 | < 0.001 | |||
Late Bout | |||||
Speed | 0.90 | 79.03 | < 0.001 | ||
Stride length | 0.75 | < 0.001 | |||
Cadence | 0.32 | 0.002 |
Participants engaged on average in 3.06 (1.18) walking sessions during SAM recording days 5–8. On those days, participants accumulated significantly more minutes of moderate intensity walking (mean increase = 21.44 (56.14%) and took significantly more steps (mean increase = 3,484 (28.42%) than at baseline (p < 0.05). Of the 38.19 mean daily moderate intensity minutes and 12,261 mean daily steps accumulated on intervention days, 29.62 moderate intensity minutes and 4,307 steps were directly attributed to walking interventionsessions.
Clinical outcomes
Following completion of the four-week intervention protocol, participants reported significantly better overall health-related quality of life (PDQ-39 total score; p = 0.027) and had lower disease severity (UPDRS-3 score; p = 0.010), improved walking endurance (6MWT distance; p = 0.044), and improved functional mobility (FTSTST time; p = 0.001) compared to baseline (Table 3). No significant changes were observed in self-selected or fast gait speed (10MWT) or in mobility-related quality of life (PDQ39 mobility dimension score).
Table 3
Clinical outcomes
Baseline (SD) | Final (SD) | Mean Difference (SD) | 95% CI | p | |
PDQ39 Mob | 15.50 (19.69) | 13.69 (17.40) | –1.81 (7.61) | (–5.27, 1.65) | 0.289 |
PDQ39 Total | 17.10 (16.23) | 14.43 (15.39) | –2.68 (5.14) | (–5.02, –0.34) | 0.027 |
MDS-UPDRS III | 34.62 (9.28) | 30.95 (10.00) | –3.67 (5.89) | (–6.35, –0.99) | 0.010 |
6MWT (m) | 456.67 (96.71) | 468.12 (103.46) | 13.83 (29.43) | (.44, 27.22) | 0.044 |
10MWT Comfortable (m/s) | 1.25 (.21) | 1.28 (0.21) | 0.03 (0.14) | (–0.02, 0.10) | 0.279 |
10MWT Fast (m/s) | 1.64 (.23) | 1.64 (0.25) | 0.001 (0.17) | (–0.08, 0.08) | 0.979 |
FTSTS | 11.36 (3.07) | 9.90 (2.42) | –1.46 (1.73) | (–2.27, –0.64) | 0.001 |
Mean difference for clinical outcomes is represented as difference between baseline and final assessments. PDQ39, Parkinson’s Disease Questionnaire-39 [0–100; lower score indicates higher reported quality of life]; MDS-UPDRS III, Modified Unified Parkinson’s Disease Rating Scale Part III [0–132; lower score indicates lower disease severity]; 6MWT, 6-Minute Walk Test [meters; greater distance indicates higher walking endurance]; 10MWT, 10-meter Walk Test [m/s; greater speed indicates higher walking capacity]; FTSTS, Five Times Sit to Stand Test [seconds; lower time indicates higher functional mobility].
DISCUSSION
Study findings supported the feasibility and proof-of-concept of delivering an autonomous music-based digital walking intervention to persons with mild to moderate PD in a naturalistic setting. The study expanded on other real-world closed-loop RAS work [54, 55] by including more than one recruitment site and a wider array of outcome measures, and analyses. Given its design features (i.e., provision of personalized, gait-specific rhythmic auditory cues; frequent modulation of dosing parameters; simple operation intended for independent, real-world use) and our preliminary outcomes, the MR-005 system shows promise for overcoming practical barriers associated with in-person clinical care and improving quality, intensity and amount of walking in PD.
Feasibility
Despite the lack of clinician supervision, study participants completed a large percentage of the recommended number of walking sessions and exceeded recommendations for session duration (Fig. 2). The high adherence rate was especially encouraging, given that disease-specific motor (e.g., bradykinesia, reduced movement output, gait variability) and non-motor (e.g., depression; apathy) symptoms are known contributors to physical inactivity in persons with PD [56]. Several factors may have contributed to participant adherence. First, during the initial, in-person session, participants received brief training on how to use the MR-005 system and demonstrated their ability to independently use it prior to departing. Second, the musical basis for the intervention, which was designed to enhance activation of the motor system and neural networks involved in walking [57], may have positively reinforced adherence by contributing to improvements in walking quality. Third, the MR-005 system ran autonomously, requiring minimal participant input once sessions began. These elements collectively may have contributed to high numbers of participants reporting the system was easy to use and confidence in their ability to operate it successfully (Fig. 3). It also is worth considering that high adherence rates could have been influenced by a Hawthorne effect associated with weekly check-in calls and the keeping of a study diary.
No safety issues were reported during the study. The absence of adverse events (e.g., falls) provided support for the idea that the autonomous digital intervention could be used reliably and safely to modulate the gait characteristics of persons with mild to moderate PD in a progressively challenging manner. Participant safety was likely a result of the “closed-loop” nature of the music delivery, which modulated rhythm stimulation according to user walking cadence while simultaneously evaluating gait quality metrics (e.g., temporal symmetry).
Proof-of-concept
RAS principles incorporated into the digital intervention were intended specifically to leverage neural mechanisms underlying auditory motor entrainment [21, 22]. The goal was to help persons with PD maintain a more efficient and stable walking rhythm despite potentially impaired internal rhythm generation and motor drive mechanisms [1, 2]. Although the study was not designed to determine the specific contribution of RAS over other factors (e.g., the musical context for cues), several findings were suggestive of its influence.
First, participants walked faster, took longer strides, had a higher cadence and had lower stride to stride variability when exposed to rhythmic cues. The delivery of each progressive change in tempo was contingent on participants’ ability to entrain to auditory cues while producing satisfactory gait quality, as assessed in real-time using the closed-loop design of the MR-005 system. Importantly, as participants increased their gait speed in response to music tempo modulation, they generally did so by increasing step length more than cadence. This finding had important implications, given that interventions which increase cadence without a concurrent increase in stride length may decrease stability when walking [31, 32, 58].
Second, the digital intervention appeared to elicit a substantial increase in moderate intensity walking during sessions (Fig. 5B, C). Considering that persons with PD tend to naturally accumulate far fewer than 100 steps in a given minute [8], it seems reasonable to infer that the delivery of rhythmic cues above 100 beats per minute using a closed loop protocol may have helped drive increases in minutes of modeate intensity walking, especially given that participants had not received instructions to walk at a specific pace. It also seems reasonable to infer that improved efficiency and stability of the gait rhythm, as demonstrated by reduced gait variability, may have contributed to participants’ ability to sustain moderate intensity walking throughout sessions. Considering that persons with PD tend to walk naturally in relatively short bouts [8], and that a one-year, naturalistic study of persons with PD reported 40% decline in daily minutes of moderate intensity walking [7], extended periods of walking at moderate intensity may convey important health benefits [26, 27]. Indeed, the sample at baseline accumulated on average 16 minutes of moderate-intensity walking per day. During the first few days of intervention, however, participants averaged 38 minutes of daily moderate intensity walking (Fig. 4), which if sustained, would easily exceed recommended weekly physical activity targets [59, 60].
Fig. 5
Mean daily steps (A) and moderate intensity minutes (B) at baseline and during intervention. Example single-day SAM data (C) displays steps recorded each minute, including moderate intensity minutes containing > /= 100 steps. Walking during intervention appears in red.
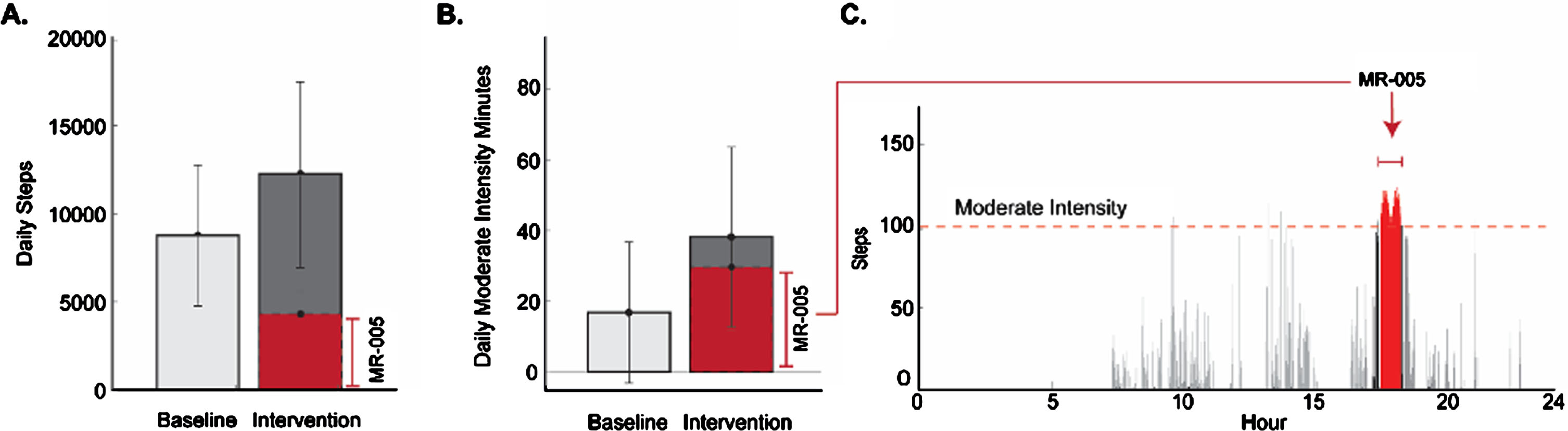
Step counts on intervention days may have increased for a variety of reasons unrelated to the RAS-component of the intervention (e.g., researcher instructions to engage in five, 30-minute sessions per week; external factors beyond the study). The finding, however, was not necessarily expected. Based on the results of previous PD research [27], we had wondered if participants in our study might limit their walking activity during hours before and/or after using MR-005, possibly resulting in reduced (or no meaningful net change in) daily step count. Clearly this did not occur (Fig. 5A): the increase in daily minutes of moderate intensity walking did not come at the cost of reduced overall walking activity. Taken together, these findings suggested that the intervention may help persons with PD to access a latent capacity to increase both the amount and intensity of walking activity.
Clinical outcomes
Reducing disability and enhancing quality of life are major focuses of interventions for persons with PD. Our results revealed significant improvements in quality of life, disease severity, walking endurance, and functional mobility among participants following completion of the four-week walking intervention. Importantly, the improvements in most clinical outcomes were modest, perhaps due to the short-term nature of the intervention (4 weeks) or to measurement ceiling effects associated with the relatively high functioning sample at baseline. Although significant and clinically meaningful improvements were observed in the MDS-UPDRS score, uncontrolled factors (e.g., medication timing, interrater variability between sites) may have influenced these results. Nonetheless, the promising clinical outcomes warrant further consideration of the idea that routine use of the intervention might contribute to long-term health outcomes and potential slowing of disability progression.
Study limitations
The small sample, which consisted primarily of highly educated, persons with mild to moderate disease severity who were relatively high functioning at baseline, limited the generalizability of findings to a broader population of persons with PD with greater disease severity. The baseline value of 8,777 mean daily steps was considerably higher than daily step counts reported in previous studies of persons with PD (∼5,400 steps/day) [6, 61] and was suggestive of individuals who were higher functioning and more motivated to be physically active. Given the previously untested safety of MR-005 among persons with PD, and because participants would be unsupervised during walking sessions, we intentionally excluded persons with greater disease severity, more disabling gait patterns (e.g., freezing of gait), and cognitive impairment.
The lack of a non-RAS control group limited our assessment of intervention impact above and beyond a music-only walking intervention or a structured walking program without a digital component. To minimize participant burden, SAM data were recorded for only a few days early in the intervention and were not recollected after four weeks. The study duration was relatively short, and no retention analysis was conducted. The unsupervised, real-world nature of the intervention likely contributed to the prevalence of missing data and uncontrolled sources of variability in gait performance (e.g., stopping at intersections, unanticipated environmental distractions, and other episodic events).
Future directions
Future studies involving MR-005 are warranted. Special consideration should be given to varying responses to auditory cues and rhythmic abilities among persons with PD [46–48]. The utility of MR-005 for either gait training purposes or as a long-term aid to assist daily walking has yet to be determined. Larger randomized controlled trials with more diverse samples and longer follow-up periods are needed to more fully assess its effectiveness, especially in more impaired individuals who may benefit most from RAS-based interventions.
ACKNOWLEDGMENTS
The digital intervention described in this manuscript (MR-005, MedRhythms, Inc. Portland, ME) is investigational and currently in development. Any findings expressed are not indicative of claims around efficacy. At the time of publication, MR-005 had not been approved or cleared by the U.S. Food & Drug Administration for any indication.
FUNDING
This study was funded by MedRhythms Inc., 1F31D110123-01 (J.A.Z.), and supported by the Foundation for Physical Therapy Research PODS II Scholarship (J.A.Z.). Clinical trials registry #NCT04891107.
CONFLICT OF INTEREST
The authors declare the following potential conflicts of interest with respect to the research, authorship, and/or publication of this article: L.N.A. is a paid advisor to MedRhythms Inc. B.A.H. is the cofounder and CEO of MedRhythms, Inc. K.E.S. is a Senior Applied Researcher at MedRhythms Inc.
DATA AVAILABILITY
The data supporting the findings of this study are available within the article and/or its supplementary material.
SUPPLEMENTARY MATERIAL
[1] The supplementary material is available in the electronic version of this article: https://dx.doi.org/10.3233/JPD-230169.
REFERENCES
[1] | Wu T , Liu J , Zhang H , Hallett M , Zheng Z , Chan P ((2015) ) Attention to automatic movements in Parkinson’s disease: Modified automatic mode in the striatum. Cereb Cortex 25: , 3330–3342. |
[2] | Gilat M , Bell PT , Ehgoetz Martens KA , Georgiades MJ , Hall JM , Walton CC , Lewis SJG , Shine JM ((2017) ) Dopamine depletion impairs gait automaticity by altering cortico-striatal and cerebellar processing in Parkinson’s disease. Neuroimage 152: , 207–220. |
[3] | Morris ME , Huxham F , McGinley J , Dodd K , Iansek R ((2001) ) The biomechanics and motor control of gait in Parkinson disease. Clin Biomech 12: , 459–470. |
[4] | Padmanabhan P , Sreekanth Rao K , Gonzalez AJ , Pantelyat AY , Chib VS , Roemmich RT ((2021) ) The cost of gait slowness: Can persons with Parkinson’s disease save energy by walking faster? J Parkinsons Dis 11: , 2073–2084. |
[5] | Gallo PM , McIsaac TL , Garber CE ((2014) ) Walking economy during cued versus non-cued self-selected treadmill walking in persons with Parkinson’s disease. J Parkinsons Dis 4: , 705–716. |
[6] | Lord S , Godfrey A , Galna B , Mhiripiri D , Burn D , Rochester L ((2013) ) Ambulatory activity in incident Parkinson’s: More than meets the eye? J Neurol 260: , 2964–2972. |
[7] | Cavanaugh JT , Ellis TD , Earhart GM , Ford MP , Foreman KB , Dibble LE ((2012) ) Capturing ambulatory activity decline in Parkinson’s disease. J Neurol Phys Ther 36: , 51–57. |
[8] | Girnis J , Cavanaugh JT , Baker T , Duncan RP , Fulford D , LaValley MP , Lawrence M , Nordahl T , Porciuncula F , Rawson KS , Saint-Hilaire M , Thomas C , Zajac J , Earhart GM , Ellis TD ((2023) ) Natural walking intensity in persons with Parkinson disease. J Neurol Phys Ther 47: , 146–154. |
[9] | Mirelman A , Bonato P , Camicioli R , Ellis TD , Giladi N , Hamilton JL , Hass CJ , Hausdorff JM , Pelosin E , Almeida QJ ((2019) ) Gait impairments in Parkinson’s disease. Lancet Neurol 18: , 697–708. |
[10] | Hariz GM , Forsgren L ((2011) ) Activities of daily living and quality of life in persons with newly diagnosed Parkinson’s disease according to subtype of disease, and in comparison to healthy controls. Acta Neurol Scand 123: , 20–27. |
[11] | Schaafsma JD , Giladi N , Balash Y , Bartels AL , Gurevich T , Hausdorff JM ((2003) ) Gait dynamics in Parkinson’s disease: Relationship to Parkinsonian features, falls and response to levodopa. J Neurol Sci 212: , 47–53. |
[12] | Lord S , Galna B , Yarnall AJ , Coleman S , Burn D , Rochester L ((2016) ) Predicting first fall in newly diagnosed Parkinson’s disease: Insights from a fall-naïve cohort. Mov Disord 31: , 1829–1836. |
[13] | Port RJ , Rumsby M , Brown G , Harrison IF , Amjad A , Bale CJ ((2021) ) People with Parkinson’s disease: What symptoms do they most want to improve and how does this change with disease duration? J Parkinsons Dis 11: , 715–724. |
[14] | Bohnen NI , Costa RM , Dauer WT , Factor SA , Giladi N , Hallett M , Lewis SJG , Nieuwboer A , Nutt JG , Takakusaki K , Kang UJ , Przedborski S , Papa SM , Committee TMSI ((2022) ) Discussion of research priorities for gait disorders in Parkinson’s disease. Mov Disord 37: , 253–263. |
[15] | Thaut MH , McIntosh GC , Hoemberg V ((2015) ) Neurobiological foundations of neurologic music therapy: Rhythmic entrainment and the motor system. Front Psychol 5: , 1185. |
[16] | Wu Z , Kong L , Zhang Q ((2022) ) Research progress of music therapy on gait intervention in patients with Parkinson’s disease. Int J Environ Res Public Health 19: , 9568. |
[17] | Hausdorff JM , Lowenthal J , Herman T , Gruendlinger L , Peretz C , Giladi N ((2007) ) Rhythmic auditory stimulation modulates gait variability in Parkinson’s disease. Eur J Neurosci 26: , 2369–2375. |
[18] | Thaut MH , McIntosh GC , Rice RR , Miller RA , Rathbun J , Brault JM ((1996) ) Rhythmic auditory stimulation in gait training for Parkinson’s disease patients. Mov Disord 11: , 193–200. |
[19] | Thaut MH , Kenyon GP , Schauer ML , McIntosh GC ((1999) ) The connection between rhythmicity and brain function. IEEE Eng Med Biol Mag 18: , 101–108. |
[20] | Molinari M , Leggio MG , Martin MD , Cerasa A , Thaut M ((2003) ) Neurobiology of rhythmic motor entrainment. Ann N Y Acad Sci 999: , 313–321. |
[21] | Sen S , Kawaguchi A , Truong Y , Lewis MM , Huang X ((2010) ) Dynamic changes in cerebello-thalamo-cortical motor circuitry during progression of Parkinson’s disease. Neuroscience 166: , 712–719. |
[22] | Ashoori A , Eagleman DM , Jankovic J ((2015) ) Effects of auditory rhythm and music on gait disturbances in Parkinson’s disease. Front Neurol 6: , 234. |
[23] | Park KS , Hass CJ , Janelle CM ((2021) ) Familiarity with music influences stride amplitude and variability during rhythmically-cued walking in individuals with Parkinson’s disease. Gait Posture 87: , 101–109. |
[24] | Frenkel-Toledo S , Giladi N , Peretz C , Herman T , Gruendlinger L , Hausdorff JM ((2005) ) Effect of gait speed on gait rhythmicity in Parkinson’s disease: Variability of stride time and swing time respond differently. J Neuroeng Rehabil 2: , 23. |
[25] | Ginis P , Heremans E , Ferrari A , Dockx K , Canning CG , Nieuwboer A ((2017) ) Prolonged walking with a wearable sensor system providing intelligent auditory input in people with Parkinson’s disease. Front Neurol 8: , 128. |
[26] | Mak MKY , Wong-Yu ISK ((2021) ) Six-month community-based brisk walking and balance exercise alleviates motor symptoms and promotes functions in people with Parkinson’s disease: A randomized controlled trial. J Parkinsons Dis 11: , 1431–1441. |
[27] | Schenkman M , Moore CG , Kohrt WM , Hall DA , Delitto A , Comella CL , Josbeno DA , Christiansen CL , Berman BD , Kluger BM , Melanson EL , Jain S , Robichaud JA , Poon C , Corcos DM ((2018) ) Effect of high-intensity treadmill exercise on motor symptoms in patients with de novo Parkinson disease: A phase 2 randomized clinical trial. JAMA Neurol 75: , 219–226. |
[28] | Rawson KS , Cavanaugh JT , Colon-Semenza C , DeAngelis T , Duncan RP , Fulford D , LaValley MP , Mazzoni P , Nordahl T , Quintiliani LM , Saint-Hilaire M , Thomas CA , Earhart GM , Ellis TD ((2020) ) Design of the WHIP-PD study: A phase II, twelve-month, dual-site, randomized controlled trial evaluating the effects of a cognitive-behavioral approach for promoting enhanced walking activity using mobile health technology in people with Parkinson-disease. BMC Neurol 20: , 146. |
[29] | Ellis TD , Cavanaugh JT , Earhart GM , Ford MP , Foreman KB , Thackeray A , Thiese MS , Dibble LE ((2016) ) Identifying clinical measures that most accurately reflect the progression of disability in Parkinson disease. Parkinsonism Relat Disord 25: , 65–71. |
[30] | Shulman LM ((2010) ) Understanding disability in Parkinson’s disease. Mov Disord 25: , S131–S135. |
[31] | Forte R , Tocci N , De Vito G ((2021) ) The impact of exercise intervention with rhythmic auditory stimulation to improve gait and mobility in Parkinson disease: An umbrella review. Brain Sci 11: , 685. |
[32] | Ye X , Li L , He R , Jia Y , Poon W ((2022) ) Rhythmic auditory stimulation promotes gait recovery in Parkinson’s patients: A systematic review and meta-analysis. Front Neurol 13: , e11060685. |
[33] | Lim I , van Wegen E , de Goede C , Deutekom M , Nieuwboer A , Willems A , Jones D , Rochester L , Kwakkel G ((2005) ) Effects of external rhythmical cueing on gait in patients with Parkinson’s disease: A systematic review. Clin Rehabil 19: , 695–713. |
[34] | Osborne JA , Botkin R , Colon-Semenza C , DeAngelis TR , Gallardo OG , Kosakowski H , Martello J , Pradhan S , Rafferty M , Readinger JL , Whitt AL , Ellis TD ((2022) ) Physical therapist management of Parkinson disease: A clinical practice guideline from the American Physical Therapy Association. Phys Ther 102: , e302. |
[35] | Ellis TD , Earhart GM ((2021) ) Digital therapeutics in Parkinson’s disease: Practical applications and future potential. J Parkinsons Dis 11: , S95–S101. |
[36] | Lang S , McLelland C , MacDonald D , Hamilton DF ((2022) ) Do digital interventions increase adherence to home exercise rehabilitation? A systematic review of randomised controlled trials. Arch Physiother 12: , 24. |
[37] | Hughes AJ , Daniel SE , Kilford L , Lees AJ ((1992) ) Accuracy of clinical diagnosis of idiopathic Parkinson’s disease: A clinico-pathological study of 100 cases. J Neurol Neurosurg Psychiatry 55: , 181–184. |
[38] | Ben-Zeev D , Brenner CJ , Begale M , Duffecy J , Mohr DC , Mueser KT ((2014) ) Feasibility, acceptability, and preliminary efficacy of a smartphone intervention for schizophrenia. Schizophr Bull 40: , 1244–1253. |
[39] | Goetz CG , Tilley BC , Shaftman SR , et al. ((2008) ) Movement Disorder Society-sponsored revision of the Unified Parkinson’s Disease Rating Scale (MDS-UPDRS): Scale presentation and clinimetric testing results. Mov Disord 23: , 2129–2170. |
[40] | Tudor-Locke C , Hatano Y , Pangrazi RP , Kang M ((2008) ) . Revisiting “how many steps are enough?”. Med Sci Sports Exer 40: , S537–S543. |
[41] | Shepherd EF , Toloza E , McClung CD , Schmalzried TP ((1999) ) Step activity monitor: Increased accuracy in quantifying ambulatory activity. J Orthop Res 17: , 703–708. |
[42] | Coleman KL , Smith DG , Boone DA , Joseph AW , del Aguila MA ((1999) ) Step activity monitor: Long-term, continuous recording of ambulatory function. J Rehabil Res Dev 36: , 8–18. |
[43] | Macko RF , Haeuber E , Shaughnessy M , Coleman KL , Boone DA , Smith GV , Silver KH ((2002) ) Microprocessor-based ambulatory activity monitoring in stroke patients. Med Sci Sports Exer 34: , 394–399. |
[44] | Salarian A , Russmann H , Vingerhoets FJ , Dehollain C , Blanc Y , Burkhard PR , Aminian K ((2004) ) Gait assessment in Parkinson’s disease: Toward an ambulatory system for long-term monitoring. IEEE Trans Biomed Eng 51: , 1434–1443. |
[45] | Gaines J , Coleman KL , Beck J , Shao YL ((2005) ) The effectiveness of the stepwatch activity monitor. Gerontologist 45: , 524. |
[46] | Manns PJ , Baldwin E ((2009) ) Ambulatory activity of stroke survivors: Measurement options for dose, intensity, and variability of activity. Stroke 40: , 864–867. |
[47] | Speelman AD , van Nimwegen M , Borm GF , Bloem BR , Munneke M ((2011) ) Monitoring of walking in Parkinson’s disease: Validation of an ambulatory activity monitor. Parkinsonism Relat Disord 17: , 402–404. |
[48] | Martínez-Martin P , Carod-Artal FJ , da Silveira Ribeiro L , Ziomkowski S , Vargas AP , Kummer W , Mesquita HM ((2008) ) Longitudinal psychometric attributes, responsiveness, and importance of change: An approach using the SCOPA-Psychosocial questionnaire. Mov Disord 23: , 1516–1523. |
[49] | Steffen T , Seney M ((2008) ) Test-retest reliability and minimaldetectable change on balance and ambulation tests, the 36-itemshort-form health survey, and the unified Parkinson disease ratingscale in people with parkinsonism. Phys Ther 88: , 733–746. |
[50] | Duncan RP , Leddy AL , Earhart GM ((2011) ) Five times sit-to-stand test performance in Parkinson’s disease. Arch Phys Med Rehabil 92: , 1431–1436. |
[51] | Harris PA , Taylor R , Thielke R , Payne J , Gonzalez N , Conde JG ((2009) ) Research electronic data capture (REDCap)–a metadata-driven methodology and workflow process for providing translational research informatics support. J Biomed Inform 42: , 377–381. |
[52] | Wright A ((2016) ) REDCap: A tool for the electronic capture of research data. J Electron Resour Med Libraries 13: , 197–201. |
[53] | Harris PA , Taylor R , Minor BL , Elliott V , Fernandez M , O’Neal L , McLeod L , Delacqua G , Delacqua F , Kirby J , Duda SN REDCap Consortium ((2019) ) The REDCap consortium: Building an international community of software platform partners. J Biomed Inform 95: , e103208. |
[54] | Cochen De Cock V , Dotov DG , Ihalainen P , Bégel V , Galtier F , Lebrun C , Picot MC , Driss V , Landragin N , Geny C , Bardy B , DallaBella S ((2018) ) Rhythmic abilities and musical training inParkinson’s disease: Do they help? NPJ Parkinsons Dis 4: , 8. |
[55] | Cochen De Cock V , Dotov D , Damm L , Lacombe S , Ihalainen P , Picot MC , Galtier F , Lebrun C , Giordano A , Driss V , Geny C , Garzo A , Hernandez E , Van Dyck E , Leman M , Villing R , Bardy BG , Dalla Bella S ((2021) ) BeatWalk: Personalized music-based gait rehabilitation in Parkinson’s disease. Front Psychol 12: , e655121. |
[56] | van Nimwegen M , Speelman AD , Hofman-van Rossum EJ , Overeem S , Deeg DJ , Borm GF , van der Horst MH , Bloem BR , Munneke M ((2011) ) Physical inactivity in Parkinson’s disease. J Neurol 258: , 2214–2221. |
[57] | Koshimori Y , Strafella AP , Valli M , Sharma V , Cho S , Houle S , Thaut MH ((2019) ) Motor synchronization to rhythmic auditory stimulation (RAS) attenuates dopaminergic responses in ventral striatum in young healthy adults: [11C]-(+)-PHNO PET study. Front Neurosci 13: , 106. |
[58] | Burrai F , Apuzzo L , Zanotti R (2021) Effectiveness of rhythmic auditory stimulation on gait in Parkinson disease: A systematic review and meta-analysis. Holistic Nursing Practice, doi: 10.1097/HNP.0000000000000462. |
[59] | Liguori G (2021) ACSM’s guidelines for exercise testing and prescription. Vol 11. Wolters Kluwer, Philadelphia. |
[60] | World Health Organization (2020) WHO guidelines on physical activity and sedentary behaviour. https://www.who.int/publications/i/item/9789240015128.Last updated November 20, 2020. Accessed April 18, 2023. |
[61] | Christiansen C , Moore C , Schenkman M , Kluger B , Kohrt W , Delitto A , Berman B , Hall D , Josbeno D , Poon C , Robichaud J , Wellington T , Jain S , Comella C , Corcos D , Melanson E ((2017) ) Factors associated with ambulatory activity in de novo Parkinson disease. J Neurol Phys Ther 41: , 93–100. |