Sex Differences in Brain Structure in de novo Parkinson’s Disease: A Cross-Sectional and Longitudinal Neuroimaging Study
Abstract
Background:
Parkinson’s disease (PD) varies in occurrence, presentation, and severity between males and females. However, the sex effects on the patterns of brain structure, cross-sectionally and longitudinally, are still unclear.
Objective:
We aimed to compare sex differences in brain features cross-sectionally and longitudinally using grey matter volume (GMV) and cortical thickness in a large sample of newly diagnosed drug-naive PD patients.
Methods:
Cognitive assessments and structural MR images of 262 PD patients (171 males) and 113 healthy controls (68 males) were selected from the Parkinson’s Progression Markers Initiative. Of these, 97 PD patients (66 males) completed 12- and 24-month follow-up examinations. After regressing out the expected effects of age and sex, brain maps of GMV and cortical thickness were compared using two-sample t tests cross-sectionally and were compared using repeated measurement analyses of variance longitudinally.
Results:
At baseline, male PD patients exhibited a greater extent of brain atrophy and cortical thickness reduction than females, which mainly occurred in the cerebellum, frontal lobe, parietal lobe, and temporal lobe. At follow-up, female and male PD patients showed similar dynamics of disease progression, as both groups declined over time while the females maintained the advantage. The cortical thickness of the right precentral gyrus at baseline was negatively associated with the longitudinal changes of motor function in male PD patients.
Conclusion:
The current findings might demonstrate sex effect in neuroanatomy during the course of PD, provide new insights into the neurodegenerative process, and facilitate the development of more effective sex-specific therapeutic strategies.
INTRODUCTION
Parkinson’s disease (PD) varies in occurrence, presentation, and severity between males and females [1]. Epidemiological studies have revealed a lower incidence and higher age at onset in females [2]. The clinical phenotype of PD in females is reported to be more benign, with tremor [2, 3] and anxiety disorder/depression [4] as the main clinical manifestations, while that in males is characterized by rigidity [2], daytime sleepiness [5], cognitive impairment [6], and rapid eye movement sleep behavioral disorder [7]. These sex-related differences suggest that PD may involve distinct pathogenetic mechanisms in women and men.
Previous studies have revealed that sex-related PD pathophysiology mainly included hormonal factors, genetic factors, neuroendocrine factors, biochemical and molecular factors, and clinical factors [8]. Estrogen plays a preponderant role with neuroprotective effects, anti-inflammatory effects, and effects on oxidative stress reduction in the female brain [9]. Sex differences in gene dysregulation associated with dopamine neurons were also reported in PD [10]. Males with PD show an overexpression of PD pathogenesis-related genes, such as alpha-synuclein and PINK1. In contrast, females with PD show an upregulation of genes related to signal transduction and neuronal maturation [11].
Several structural neuroimaging methods using magnetic resonance imaging (MRI) have been used to detect the neuroanatomical changes in PD [12]. For example, compared with heathy controls (HCs), grey matter volume (GMV) atrophy [13], cortical thinning [14], increased iron load [15], and reduced fractional anisotropy [16] in PD patients have been reported. Furthermore, few studies have explored the sex differences between women and men, revealing significantly smaller total cortical and subcortical GMV [17], reduced cortical thickness, lower connection strengths, and lower clustering coefficients [18] in males with PD.
However, corrections for brain volume, age or sex effects existing in HCs are rarely taken into consideration. In addition, the sex-related longitudinal changes in brain structure in PD remain largely unexplored. In the present study, the W-score approach proposed by La Joie et al. [19] was used to regress out the possible normal brain difference between sexes and the effect of age on brain structure. According to this method, Tremblay et al. [20] reported greater tissue loss and connectivity disruption in males with PD than in females. We aimed to compare sex differences in brain features cross-sectionally and longitudinally using GMV and cortical thickness in a large sample of newly diagnosed drug-naive PD patients.
METHODS
Participants
Data used in the present study were selected from the Parkinson’s Progression Markers Initiative database (http://www.ppmi-info.org/data), which is a large-scale, observational, international, multicenter study to identify PD progression biomarkers [21]. For each participating PPMI site, the study was approved by the ethical standards committee before study initiation. Written informed consent was obtained from all participants.
Inclusion criteria of PD patients were the following: 1) an asymmetric bradykinesia or asymmetric resting tremor or two symptoms, including resting tremor, bradykinesia, and rigidity, with diagnosis within 2 years; 2) Hoehn and Yahr stage 1 or 2 at baseline; 3) a dopamine transporter deficit confirmed by dopamine transporter imaging; 4) lack of treatment for PD within at least 6 months after baseline diagnosis; and 5) age of older than 30 years. PD patients who were taking PD-related medications or investigational drugs or devices within 60 days of baseline diagnosis were excluded from the study.
Inclusion criteria for HCs were as follows: 1) 30 years of age or older; 2) no significant neurologic dysfunction; 3) no first-degree family member with PD; 4) Montreal Cognitive Assessment (MoCA) score >26.
At baseline, 262 newly diagnosed, drug-naive nondemented PD patients (171 males, 91 females, defined as PD group 1) and 113 HCs (68 males, 45 females) were included. All the participants underwent T1-weighted MRI scans and clinical assessments.
At follow-up, among all 262 PD patients, 97 PD patients (66 males and 31 females, defined as PD group 2) completed the same MRI scans and clinical assessments at 12 months and 24 months, respectively. Therefore, 3 time points were included in PD group 2—baseline (T0), 12-month follow-up (T1), and 24-month follow-up (T2).
Clinical assessments
The Hoehn and Yahr stage score and the Movement Disorder Society–Unified Parkinson’s Disease Rating Scale III (MDS-UPDRS III) were used to define the disease stage and the disease severity in PD patients. Olfactory function was assessed by the University of Pennsylvania Smell Identification Test (UPSIT), and autonomic disorder was assessed by Scales for Outcomes in Parkinson’s Disease-Autonomic (SCOPA-AUT). All the participants underwent the neuropsychological tests, including the MoCA, the Geriatric Depression Scale (GDS), the Semantic Fluency, the Modified Schwab and England Activities of Daily Living (ADL), the Hopkins Verbal Learning Test (HVLT), and the Letter-Number Sequencing.
MRI data acquisition parameters
Philips Medical Systems, GE Medical Systems, and SIEMENS scanners were used for the MRI acquisition. T1 images were obtained from 78 subjects using GE Medical Systems with the following protocols: matrix size = 256×256, slice thickness = 1.2–1.4 mm, 152–248 slices, flip angle (FA): 8°–15°, echo time (TE) = 3.02–5.17 ms, and repetition time (TR) = 8.16–13 ms; imaging in 62 subjects was performed using Philips Medical Systems with the following protocols: matrix x = 240–268, y = 192–256, slice thickness = 1–1.2 mm, 136–170 slices, FA = 8°, TE = 3.16–4.01 ms, and TR = 6.83–8.51 ms; and imaging in 235 subjects was performed using SIEMENS with the following protocols: matrix x = 192–256, y = 192–256, slice thickness = 1 mm, 128–192 slices, FA = 8°–15°, TE = 2.27–3.65 ms, and TR = 1900–2400 ms.
Data processing
The Computational Anatomy Toolbox 12 software (CAT12, http://www.neuro.unijena.de/cat) within SPM12 (https://www.fil.ion.ucl.ac.uk/spm) was used to conduct voxel-based morphometry (VBM) and surface-based morphometry (SBM) analysis to investigate structural abnormalities.
The VBM preprocessing included normalization into the standard Montreal Neurological Institute (MNI) space, segmentation, modulation, and smoothing with an 8-mm full-width at half-maximum (FWHM) Gaussian kernel. The total intracranial volume (TIV) represented the sum of the grey matter, white matter, and cerebrospinal fluid volumes.
Surface-based cortical thickness, the distance between the white and grey matter surfaces at each vertex, was computed using the segmented images. Then, the resulting images were topologically corrected, spherically mapped, spherically registered, normalized to standard MNI space, and smoothed by a 15 mm FWHM smoothing kernel.
W-score maps
Using the HCs group as a reference, W-score maps of GMV and cortical thickness were computed for each PD patient to regress out the expected effects of age and sex differences [20]. The W-score, similar to the Z-score, but accounting for covariates such as sex and age, represented the normal deviation of the neuroimaging measure in PD patient relative to the value expected in the control group for patient’s age and sex.
First, using data obtained from the HCs group, voxelwise linear regression (β1+β2×age+β3×sex+β4×TIV+ residuals) was performed to obtain β maps, containing intercept values (β1), age-related coefficients (β2), sex-related coefficients (β3), and TIV-related coefficients (β4) [22]. For cortical thickness, instead of TIV, a β4* of a proxy measure of brain volume (pBV = white matter + cerebrospinal fluid + subcortical grey matter) was included in the voxelwise linear regression (β1+β2×age+β3×sex+β4*×pBV+ residuals). Then, a predicted value was calculated [(β1+β2×patient’s age +β3×patient’s sex+β4×patient’s TIV) for GMV and (β1+β2×patient’s age +β3×patient’s sex+ β4*×patient’s pBV) for cortical thickness] using the β maps previously computed. Finally, the calculations of the W-score maps were as follows:
All the brain structural analyses were based on W-score maps. Negative W-score values indicated greater atrophy (reduced volume).
Statistical analysis
Demographic data and neuropsychological measures were analyzed by SPSS version 21 (IBM, Armonk, New York). In PD group 1, Student’s t tests were applied to compare continuous variables, including age, years of education, and neuropsychological scores, between females and males with PD. In PD group 2, repeated measurement analyses of variance were used to compare neuropsychological scores. Post hoc comparisons were further performed to determine differences between groups at each visit and longitudinal changes over time within groups.
In PD group 1, two-sample t tests were used to compare sex differences in GMV and cortical thickness. Then, the brain regions showing significant sex differences were defined as regions of interest (ROIs). In PD group 2, the study was organized into [2 (sex: female versus male PD patients)×3 (visit-time: T0 versus T1 versus T2)] full factorial designs. Repeated measurement analyses of variance were performed on SPM12 for whole-brain analysis and were also performed on SPSS for ROIs analysis to explore the sex differences in longitudinal changes between males and females. According to the purpose of the present study, we focused on the effect of time in males, effect of time in females, and interaction effect of “sex” by “time”.
The statistical significance thresholds for whole-brain analysis were set at p < 0.05 and were corrected for multiple comparisons by familywise error (FWE). The GMV results were reported in Anatomical Automatic Labelling templates and those of cortical thickness were reported in the Desikan-Kiliany Atlas.
Pearson’s correlations were performed to investigate a) in PD group 1, the relationships between clinical assessments and the W-scores of ROIs for males and females, separately; b) in PD group 2, the relationships between the annual changes of clinical assessments and the annual changes of GMV and cortical thickness (the W-scores) for males and females, separately; c) in PD group 2, the relationships between the annual changes of clinical assessments and the W-scores of ROIs at T0 for males and females, separately.
RESULTS
Clinical and demographical characteristics
There were no significant differences between PD group 1 and HCs in age (p = 0.11), education (p = 0.09) or sex (p = 0.35).
In PD group 1, no significant differences in age, education level, disease duration, disease stage, or disease severity were found between females and males. Olfactory dysfunction was significant in males. Regarding neuropsychological states, males showed significantly lower scores in semantic fluency, HVLT total recall and HVLT delayed recall (Table 1).
Table 1
Clinical results obtained from the 171 males and 91 females in PD group 1
Characteristics | Females | Males | p |
Age, y | 60.64/9.52 | 60.77/9.86 | 0.918 |
Education, y | 15.51/3.31 | 15.91/2.88 | 0.317 |
Disease duration, months | 7.95/8.29 | 6.16/6.02 | 0.071 |
Hoehn and Yahr stage | 1.51/0.50 | 1.55/0.51 | 0.553 |
MDS-UPDRS Part III | 18.52/8.35 | 19.72/8.35 | 0.270 |
UPSIT-score | 24.79/7.96 | 22.50/8.25 | 0.032 |
MoCA | 28.25/1.30 | 27.96/1.32 | 0.093 |
Semantic Fluency total | 53.34/10.32 | 48.13/10.77 | <0.001 |
HVLT-Total Recall | 26.81/4.23 | 24.50/4.62 | <0.001 |
HVLT-Delayed Recall | 9.31/2.00 | 8.54/2.24 | 0.005 |
LNS | 11.01/2.51 | 10.88/2.65 | 0.704 |
GDS | 1.40/1.20 | 1.60/1.27 | 0.229 |
ADL | 93.68/6.40 | 93.71/5.32 | 0.966 |
SCOPA-AUT | 8.98/5.85 | 8.46/5.21 | 0.456 |
MDS-UPDRS, Movement Disorder Society-Unified Parkinson’s Disease Rating Scale; UPSIT, University of Pennsylvania Smell Identification Test; MoCA, Montreal Cognitive Assessment; HVLT, Hopkins Verbal Learning Test; LNS, Letter-Number Sequencing; GDS, Geriatric Depression Scale; ADL, Modified Schwab and England Activities of Daily Living; SCOPA-AUT, Scales for Outcomes in Parkinson’s Disease–Autonomic. Values are expressed as the mean/SD. p values were derived from Student’s t tests comparing the two groups.
In PD group 2, the sex differences of clinical and demographical characteristics at baseline were consistent with those of PD group 1. At follow-up, significantly decreased living ability was found in both female and male PD patients. In addition, male PD patients showed significantly severe motor dysfunction, severe autonomic nervous system dysfunction, and decreased general cognition as the disease progressed (Table 2). However, no significant sex-related difference of longitudinal changes was found between male and female PD patients. Only the MDS-UPDRS III scores showed a trend of greater longitudinal decline in males than in females (p = 0.08).
Table 2
Clinical results obtained from the 66 males and 31 females in PD group 2
Characteristics | Baseline | 12-month follow-up | 24-month follow-up | p-Female | p-Male | ||||||
Female | Male | p | Female | Male | p | Female | Male | p | |||
Age, y | 59.21/8.58 | 61.96/8.84 | 0.153 | ||||||||
Education, y | 15.16/3.03 | 15.06/2.85 | 0.874 | ||||||||
Disease duration, months | 8.03/8.69 | 7.15/6.91 | 0.592 | ||||||||
UPSIT-score | 24.03/6.85 | 19.81/8.49 | 0.018 | – | – | – | – | – | – | – | – |
Hoehn and Yahr stage | 1.58/0.50 | 1.62/0.48 | 0.706 | – | – | – | – | – | – | – | – |
MDS-UPDRS Part III | 20.70/8.19 | 22.01/9.60 | 0.515 | 22.62/8.26 | 22.96/9.42 | 0.865 | 23.92/8.30 | 28.11/9.97 | 0.045 | 0.101 | <0.001 |
MoCA | 27.80/1.92 | 27.27/2.23 | 0.255 | 27.74/1.87 | 27.09/1.78 | 0.106 | 27.12/2.96 | 26.29/2.84 | 0.185 | 0.372 | 0.018 |
Semantic Fluency total | 51.67/10.56 | 46.98/9.50 | 0.040 | 53.35/10.82 | 47.87/10.80 | 0.028 | 52.98/10.52 | 47.89/10.02 | 0.033 | 0.568 | 0.657 |
HVLT-Total Recall | 26.58/5.16 | 23.19/5.24 | 0.004 | 25.69/4.38 | 22.98/6.12 | 0.031 | 25.78/5.90 | 23.12/5.47 | 0.032 | 0.500 | 0.946 |
HVLT-Delayed Recall | 9.03/2.13 | 7.56/2.86 | 0.014 | 8.92/1.99 | 7.77/2.83 | 0.045 | 8.90/2.93 | 7.61/3.17 | 0.060 | 0.977 | 0.793 |
LNS | 11.22/2.42 | 10.24/2.75 | 0.095 | 10.41/2.69 | 10.20/2.93 | 0.726 | 11.03/3.03 | 9.84/2.94 | 0.072 | 0.511 | 0.488 |
GDS | 2.22/2.76 | 2.48/2.67 | 0.661 | 2.10/2.50 | 2.50/2.70 | 0.497 | 2.03/2.35 | 2.85/2.48 | 0.125 | 0.883 | 0.312 |
ADL | 95.96/5.23 | 94.15/5.32 | 0.121 | 95.48/4.89 | 93.76/6.43 | 0.193 | 90.48/8.69 | 89.09/7.48 | 0.414 | <0.001 | <0.001 |
SCOPA-AUT | 8.83/5.92 | 9.56/6.71 | 0.610 | 10.39/6.27 | 11.00/5.97 | 0.644 | 9.64/5.12 | 11.19/4.87 | 0.153 | 0.238 | 0.041 |
MDS-UPDRS, Movement Disorder Society-Unified Parkinson’s Disease Rating Scale; UPSIT, University of Pennsylvania Smell Identification Test; MoCA, Montreal Cognitive Assessment; HVLT, Hopkins Verbal Learning Test; LNS, Letter-Number Sequencing; GDS, Geriatric Depression Scale; ADL, Modified Schwab and England Activities of Daily Living; SCOPA-AUT, Scales for Outcomes in Parkinson’s Disease–Autonomic. Values are expressed as the mean/SD. p values were derived from Student t tests between groups. p-Female values were derived from the effect of time for female PD patients in repeated measurement analysis of variance. p-Male values were derived from the effect of time for male PD patients in repeated measurement analysis of variance. - presents that scores of more than 30% of patients were absent.
Sex differences in brain volumes
In PD group 1, lower GMV in the bilateral cerebellum crus1/2, left precentral gyrus, right postcentral gyrus, left precuneus gyrus, and right superior frontal gyrus were detected in males than in females, while no significant increased GMV was observed (Table 3, Fig. 1a). Then, these 6 brain regions were defined as ROIs to detect sex differences in longitudinal changes in PD group 2. Significant sex differences in the bilateral cerebellum crus1/2 and left precentral gyrus were found at T0 (see Supplemental Table 2). However, the longitudinal change trends of the GMV in the 3 ROIs in males and in females were similar and the interaction between “sex” and “time” was not significant (Fig. 1b).
Table 3
Sex differences in brain structural characteristics in PD group 1
Brain Region | Cluster Size | MNI | t | ||
(voxels) | x | y | z | ||
GMV | |||||
Rt.Cerebellum_Crus1/2 | 1114 | 40 | –81 | –27 | 5.95 |
Lt.Cerebellum_Crus1/2 | 345 | –36 | –88 | –36 | 5.75 |
Lt.Precentral Gyrus (BA4) | 85 | –39 | –24 | 70 | 5.56 |
Rt.Postcentral Gyrus (BA1) | 55 | 57 | –16 | 31 | 5.28 |
Lt.Precuneus Gyrus (BA30) | 131 | –13 | –43 | 6 | 5.99 |
Rt.Superior Frontal Gyrus (BA9) | 56 | 4 | 70 | 18 | 5.45 |
Cortical thickness | |||||
Lt.Postcentral Gyrus | 185 | –45 | –11 | 51 | 5.04 |
Rt.Precentral Gyrus | 751 | 27 | 13 | 62 | 5.52 |
Rt.Postcentral Gyrus | 362 | 36 | 2 | 47 | 5.32 |
Lt.Posterior /Isthmus Cingulate | 864 | –7 | 29 | 45 | 6.98 |
Lt.Isthmus Cingulate | 243 | –3 | –3 | 28 | 6.37 |
Lt.Middle/Inferior Temporal Gyrus | 637 | –68 | –8 | 3 | 5.60 |
Lt.Superior Temporal Gyrus | 508 | –56 | 18 | 3 | 5.53 |
Lt.Inferior/Middle Temporal Gyrus | 108 | –49 | 43 | –27 | 4.77 |
Rt.Inferior Temporal Gyrus | 345 | 43 | 21 | –19 | 5.25 |
Rt.Superior Temporal Gyrus | 131 | 47 | 19 | 10 | 4.95 |
Rt.Temporal Pole/Entorhinal | 271 | 20 | 47 | –9 | 5.15 |
Rt.Lateral Orbital Frontal Gyrus | 267 | 0 | 74 | –3 | 6.70 |
Lt.Caudal Middle Frontal Gyrus | 286 | –49 | 43 | 46 | 4.97 |
Lt.Lateral Occipital Gyrus | 845 | –20 | –47 | –10 | 6.93 |
Rt.Lateral Occipital Gyrus | 1762 | 13 | –48 | –10 | 7.47 |
Rt.Inferior/Superior Parietal Gyrus | 297 | 27 | –10 | 62 | 4.77 |
Lt.Insula | 186 | –40 | 17 | 18 | 4.96 |
Rt.Insula | 108 | 25 | 47 | –2 | 4.85 |
GMV, grey matter volume; Bi., bilateral; Rt., right; Lt., left. BA, Brodmann Area.
Fig. 1
(a) Brain regions showed significantly reduced GMV in males than in females in PD group 1. (b) In PD group 2, significantly reduced GMV in males than in females was observed in the bilateral cerebellum_crus 1/2 and left precentral gyrus, while no significant sex difference in longitudinal changes was observed. PDF, female PD patients; PDM, male PD patients; T0, baseline; T1, 12-month follow-up; T2, 24-month follow-up; Lt., left; Rt., right; GMV, grey matter volume. *p < 0.05.
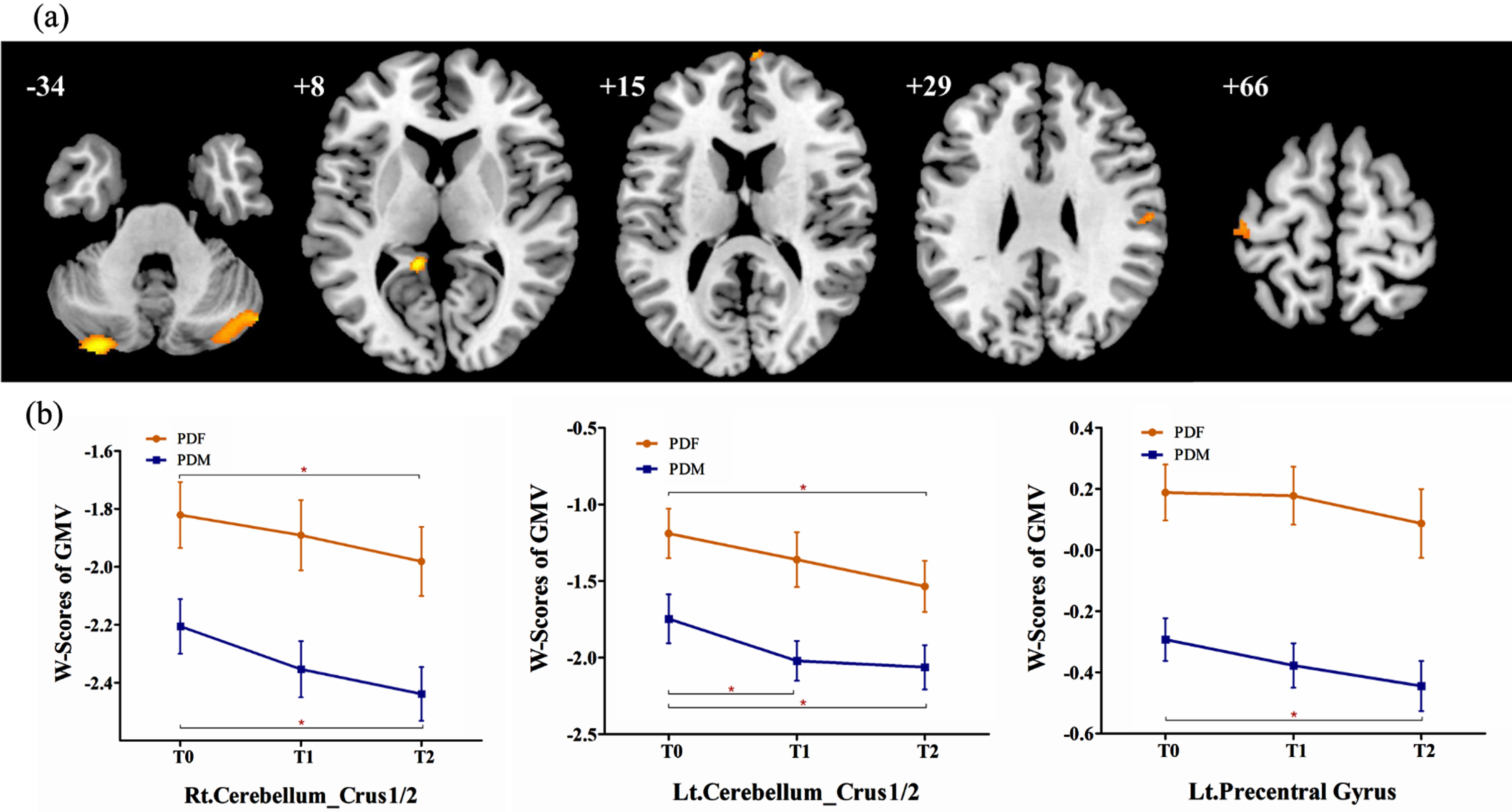
Furthermore, repeated measurement analysis of variance in the whole brain were applied in PD group 2 and revealed that: 1) at follow-up, male PD patients showed significant brain atrophy in the bilateral caudate, bilateral superior temporal gyrus, left inferior frontal orbital gyrus, right cerebellum_crus2, and left cerebellum_crus1; 2) at follow-up, female PD patients showed significant brain atrophy in the bilateral caudate; 3) however, no significant sex difference in longitudinal changes was found between males and females (Table 4).
Table 4
Longitudinal brain atrophy of males and females in PD group 2
Brain Region | Cluster Size | MNI | f | ||
(voxels) | x | y | z | ||
Male | |||||
Bi. Caudates | 756 | 7 | 4 | 19 | 44.24 |
Rt. Superior Temporal Gyrus (BA22) | 335 | 70 | –15 | 12 | 27.85 |
Lt. Superior Temporal Gyrus (BA22) | 110 | –66 | –22 | 13 | 21.71 |
Lt. Inferior Frontal Orbital Gyrus (BA11) | 158 | –48 | 15 | –7 | 20.55 |
Rt. Cerebellum_Crus2 | 112 | 3 | –90 | –34 | 18.46 |
Lt. Cerebellum_Crus1 | 137 | –34 | –87 | –27 | 16.57 |
Female | |||||
Rt. Caudate | 309 | 7 | 3 | 19 | 27.14 |
Lt. Caudate | 70 | –7 | 9 | 19 | 18.74 |
Rt., right; Lt., left; BA, Brodmann Area.
Sex differences in cortical thickness
In PD group 1, males showed extensively lower cortical thickness in the postcentral gyrus, precentral gyrus, cingulate, insula, occipital lobe, temporal lobe, frontal lobe, and parietal lobe than females, while no significant increase in cortical thickness was observed (Table 3, Fig. 2a). These brain regions were defined as ROIs to detect sex differences in longitudinal changes in PD group 2. Significant sex differences in the left cingulate, right temporal pole/entorhinal, and bilateral insula were observed at T0 (see Supplementary Table 2). However, the longitudinal change trends of the cortical thickness in the 4 ROIs in males and in females were similar and the interaction between “sex” and “time” was not significant (Fig. 2b).
Fig. 2
(a) Brain regions showed lower cortical thickness in males than in females in PD group 1; (b) in PD group 2, significantly lower cortical thickness in males than in females was observed in the left cingulate, the right temporal pole/entorhinal, and the bilateral insula, while no significant sex difference in longitudinal changes was observed. PDF, female PD patients; PDM, male PD patients; T0, baseline; T1, 12-month follow-up; T2, 24-month follow-up; Lt., left; Rt., right. *p < 0.05. **p < 0.001.
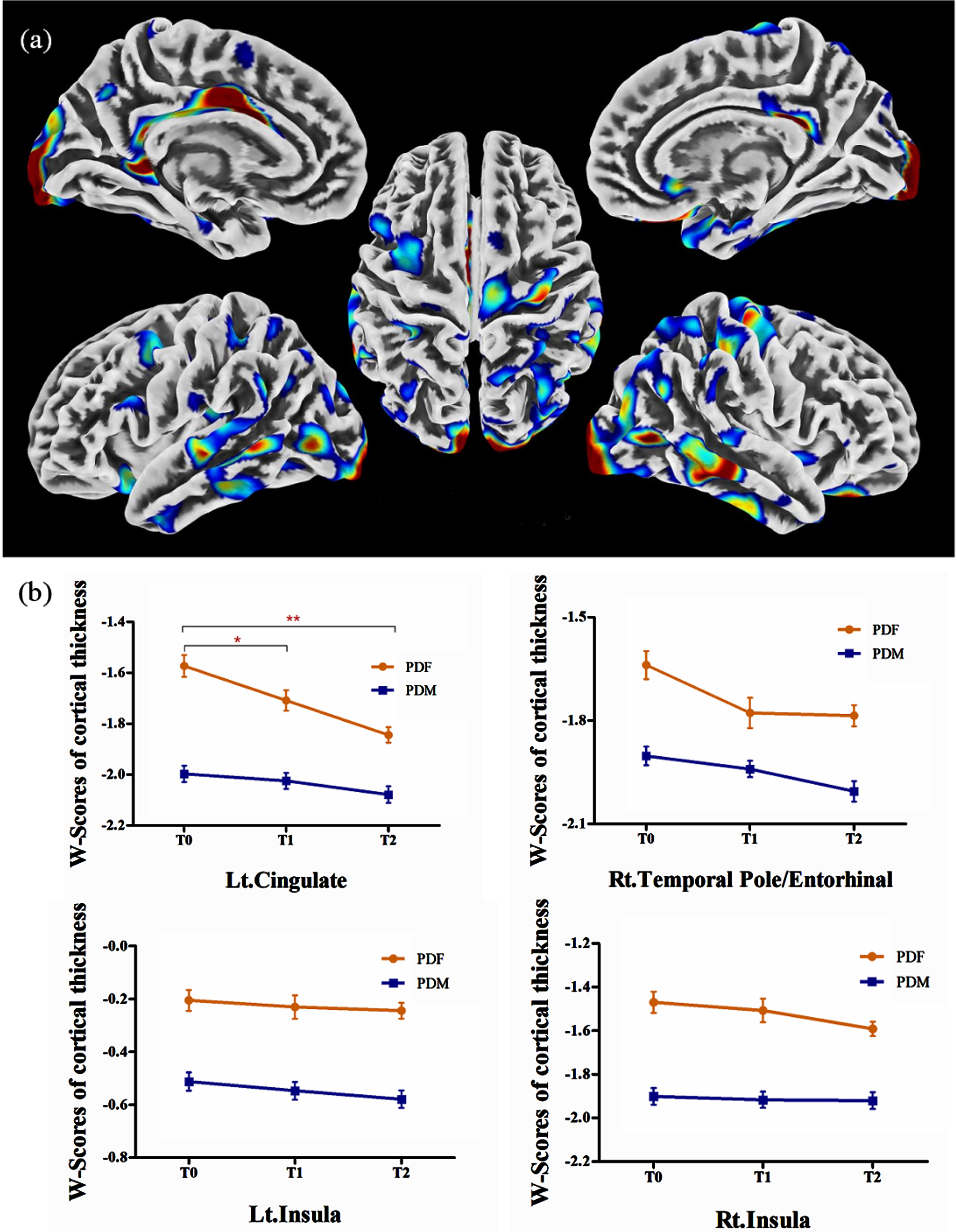
Furthermore, repeated measurement analysis of variance in the whole brain was applied in PD group 2 and no significant results were observed in the effect of time in males, the effect of time in females, and the interaction effect of “sex” by “time”.
Correlation analysis
The cortical thickness of the right precentral gyrus at baseline were negatively associated with the annual changes in MDS-UPDRS Part III scores in male PD patients (p = 0.005, r = –3.23) (Fig. 3), while no significant correlation was observed in female PD patients.
Fig. 3
The cortical thickness of the right precentral gyrus at baseline was negatively associated with the annual changes in MDS-UPDRS Part III scores over time in male PD patients (p = 0.005, r = –3.23). MDS-UPDRS, Movement Disorder Society-Unified Parkinson’s Disease Rating Scale; PDM, male PD patients.
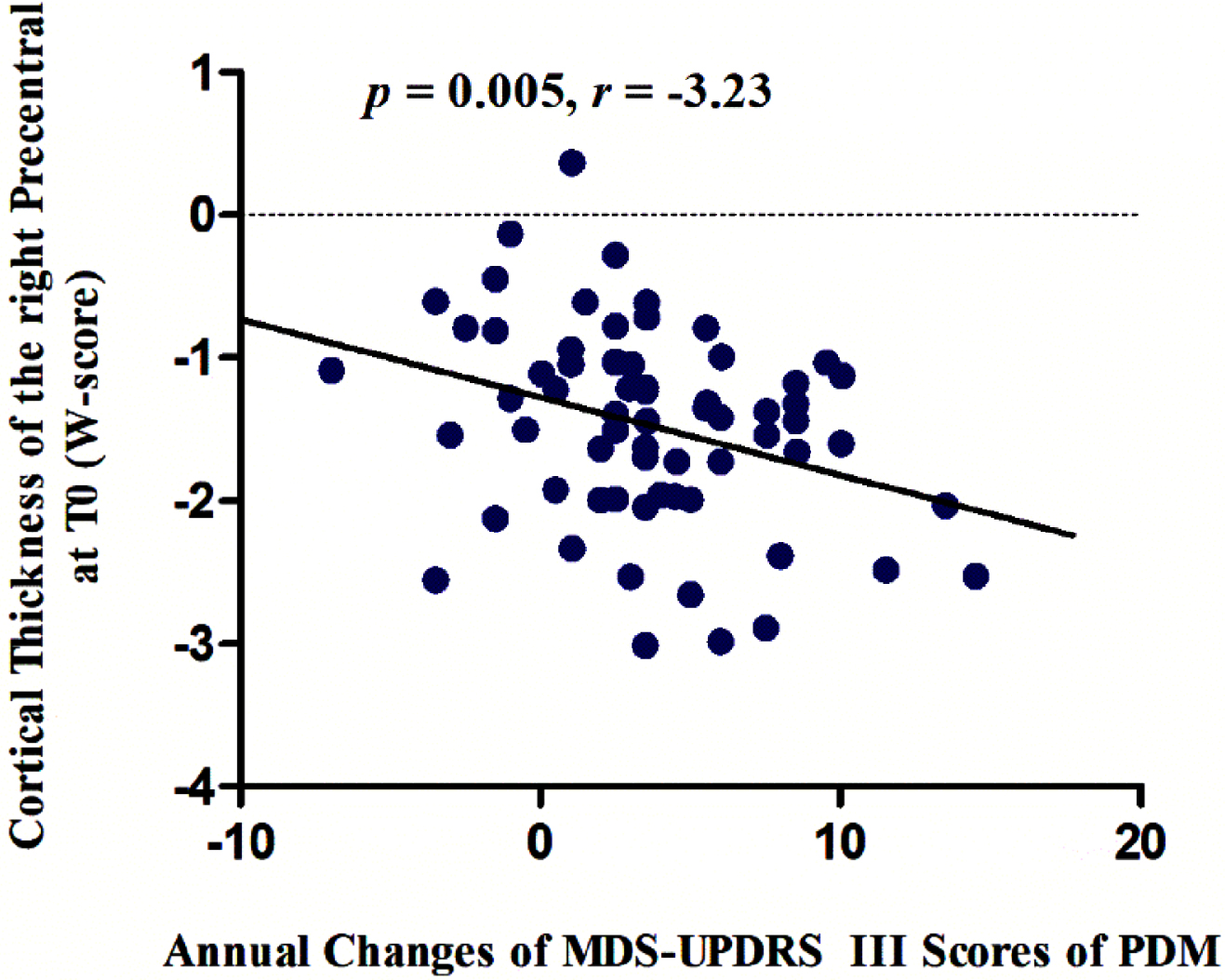
DISCUSSION
The aim of the present study was to investigate sex differences in brain structure at baseline and to investigate sex differences in longitudinal changes at follow-up between female and male PD patients after regressing out the expected effects of age and sex. At baseline, male PD patients exhibited significantly lower brain volume and decreased cortical thickness than age-matched female PD patients. At follow-up, these sex differences remained stable and analogous progressive brain atrophy with no sex interaction was found in male and female PD patients, suggesting similar dynamics of disease progression between sex over time.
Sex-related brain structural differences at baseline
Few studies had been performed with de novo PD samples to explored the sex-related brain structural differences. Tremblay et al. reported greater brain atrophy and disrupted connectivity in male PD patients [20]. Oltra et al. reported cortical thinning in postcentral and precentral regions, as well as greater global cortical and subcortical atrophy in males [17]. Our results showed greater brain abnormalities in males at baseline in extensive brain regions, including cerebellum, temporal lobe, frontal lobe, occipital brain lobe, insula, and cingulate. These results consistently suggested more marked neurodegenerations in males or neuroprotective effect in females, which might be related with the dysregulated gene expression, sex hormones, vulnerability in the dopaminergic system, neuroinflammatory cells, and oxidative stress [8, 9, 20].
PD is thought to be a movement disorder mainly related to dysfunction of the motor circuit [23]. Cerebellum and precentral gyrus are important components of the motor circuit [24, 25]. In the present study, although male PD patients showed lower brain volume and cortical thickness in motor circuit related brain regions, no significant sex difference was observed in the motor symptoms at baseline. Previous studies revealed that compared with individuals with low motor reserve, those with high motor reserve might manifest similar parkinsonian symptoms at more serious levels of PD pathology [26, 27]. In particular, some premorbid experiences (i.e., physical activity) could modulate an individual’s motor reserve [28]. Although whether sex could affect motor reserve in PD remained controversial, the current study might potentially imply sex difference in motor reserve at the beginning of the disease, but this remained to be further investigated.
In addition to motor-related brain regions, the brain regions that showed significant sex differences were primarily involved in critical functions, including cognitive, language, and olfactory functions. Atrophy in temporal and parietal regions had been repeatedly reported in previous studies [14, 29] and was associated with cognitive impairment in PD. Semantic fluency was related to thickness reductions in temporal-parietal regions and the precuneus [29]. Cortical thinning in the entorhinal cortex played an important role in olfactory dysfunction [30] and cognitive impairment [31, 32]. Consistent with previous studies, significant sex differences in the cingulate, insula, entorhinal cortex, and some temporal and parietal brain regions were observed in the present study, that maybe associated with symptom discrepancies in cognition, language, and olfactory functions between male and female PD patients.
Sex role in longitudinal evaluation in PD
Previous longitudinal studies revealed that quantitative structural MRI characteristics derived from T1-weighted, diffusion-weighted, iron-sensitive, and neuromelanin-sensitive images had the potential to track disease progression in PD. Significant atrophy at follow-up was reported in total GMV and in the volume of the caudate, precuneus, occipital fusiform gyrus, insula, and hippocampus [33–36]. In the present study, both male and female PD patients showed significant GMV reduction in bilateral caudates. These results were consistent with our previous finding [36] and further advanced them to reveal no significant sex interaction as the disease progressed.
Picillo et al. explored sex-related longitudinal changes of motor features, non-motor symptoms, cerebrospinal fluid markers and DaTscanTM binding in a five-year longitudinal study using the data selected from PPMI. Consistent with the results of the present study, no significant sex interaction for longitudinal changes was reported in all the above-mentioned clinical features and biological biomarkers [37]. In addition, sex was not associated with the time to dementia onset and did not affect the relationship between the APOE4 allele and dementia onset in PD patients [38]. Estrogen-related studies revealed that the beneficial effect of Estrogen use was observed in young females [39] but not in old females [40]. These results suggested that the longitudinal changes in some neuroanatomical and biological characteristics were not significantly different between sexes in PD.
This study also had some limitations. First, there were more males than females in all three groups: PD group 1, PD group 2, and the HCs group. These proportions were consistent with the prevalence of PD in the PPMI database and the population. Second, the data used in the present study were acquired from multiple centers and scanners around the world that might introduce some heterogeneity. However, PPMI has strict protocols and guidelines for data acquisition to ensure standardization. Third, in the longitudinal analysis, the sample size was relatively small, and no HCs group was included. Studies with larger sample size and HCs group at follow-up are needed to validate the results in the future.
In summary, by calculating the W-score maps of GMV and cortical thickness to regress out the expected effects of age and sex, the present study revealed extensive PD-related morphological brain differences between sexes at baseline and similar progressive brain atrophy with no sex interaction at follow-up, suggesting similar dynamics of disease progression in male and female PD patients. The current findings might provide new insights into sex role in neuroanatomy during the course of PD and facilitate the development of more effective sex-specific therapeutic strategies. The present findings also supported the importance of stratification of data by sex and analysis of sex interactions in PD studies.
ACKNOWLEDGMENTS
This work was supported by grants from the National Natural Science Foundation of China (62076169), R&D Program of Beijing Municipal Education Commission (KM202310025013), Multidisciplinary Clinical Research Innovation Team Project (CYDXK202201, CYDXK202207), Beijing Hospitals Authority’s Ascent Plan (DFL20220303), and Beijing Key Specialists in Major Epidemic Prevention and Control.
CONFLICT OF INTEREST
The authors have no conflict of interest to report.
DATA AVAILABILITY
Data used in this article were obtained from the PPMI database (www.ppmi-info.org/data). For up-to-date information on the study, visit:www.ppmi-info.org .
SUPPLEMENTARY MATERIAL
[1] The supplementary material is available in the electronic version of this article: https://dx.doi.org/10.3233/JPD-225125
REFERENCES
[1] | Iwaki H , Blauwendraat C , Leonard HL , Makarious MB , Kim JJ , Liu G , Maple Grødem J , Corvol JC , Pihlstrøm L , Nimwegen M , Smolensky L , Amondikar N , Hutten SJ , Frasier M , Nguyen KDH , Rick J , Eberly S , Faghri F , Auinger P , Scott KM , Wijeyekoon R , Van Deerlin VM , Hernandez DG , Gibbs RJ , Day Williams AG , Brice A , Alves G , Noyce AJ , Tysnes OB , Evans JR , Breen DP , Estrada K , Wegel CE , Danjou F , Simon DK , Andreassen OA , Ravina B , Toft M , Heutink P , Bloem BR , Weintraub D , Barker RA , Williams Gray CH , Warrenburg BP , Van Hilten JJ , Scherzer CR , Singleton AB , Nalls MA ((2021) ) Differences in the presentation and progression of Parkinson’s disease by sex. Mov Disord 36: , 106–117. |
[2] | Haaxma CA , Bloem BR , Borm GF , Oyen WJG , Leenders KL , Eshuis S , Booij J , Dluzen DE , Horstink MWIM ((2007) ) Gender differences in Parkinson’s disease. J Neurol Neurosurg Psychiatry 78: , 819–824. |
[3] | Baba Y , Putzke JD , Whaley NR , Wszolek ZK , Uitti RJ ((2005) ) Gender and the Parkinson’s disease phenotype. J Neurol 252: , 1201–1205. |
[4] | Riedel O , Klotsche J , Spottke A , Deuschl G , Förstl H , Henn F , Heuser I , Oertel W , Reichmann H , Riederer P , Trenkwalder C , Dodel R , Wittchen HU ((2008) ) Cognitive impairment in 873 patients with idiopathic Parkinson’s disease. Results from the German Study on Epidemiology of Parkinson’s Disease with Dementia (GEPAD). J Neurol 255: , 255–264. |
[5] | Martinez-Martin P , Falup PC , Odin P , van Hilten JJ , Antonini A , Rojo-Abuin JM , Borges V , Trenkwalder C , Aarsland D , Brooks DJ , Ray CK ((2012) ) Gender-related differences in the burden of non-motor symptoms in Parkinson’s disease. J Neurol 259: , 1639–1647. |
[6] | Anang JB , Gagnon JF , Bertrand JA , Romenets SR , Latreille V , Panisset M , Montplaisir J , Postuma RB ((2014) ) Predictors of dementia in Parkinson disease: A prospective cohort study. Neurology 83: , 1253–1260. |
[7] | Yoritaka A , Ohizumi H , Tanaka S , Hattori N ((2009) ) Parkinson’s disease with and without REM sleep behaviour disorder: Are there any clinical differences? Eur Neurol 61: , 164–170. |
[8] | Vaidya B , Dhamija K , Guru P , Sharma SS ((2021) ) Parkinson’s disease in women: Mechanisms underlying sex differences. Eur J Pharmacol 895: , 173862. |
[9] | Cerri S , Mus L , Blandini F ((2019) ) Parkinson’s disease in women and men: What’s the difference? J Parkinsons Dis 9: , 501–515. |
[10] | Simunovic F , Yi M , Wang Y , Stephens R , Sonntag KC ((2010) ) Evidence for gender-specific transcriptional profiles of nigral dopamine neurons in Parkinson disease. PLoS One 5: , e8856. |
[11] | Cantuti-Castelvetri I , Keller-Mcgandy C , Bouzou B , Asteris G , Clark TW , Frosch MP , Standaert DG ((2007) ) Effects of gender on nigral gene expression and Parkinson’s disease. Neurobiol Dis 26: , 606–614. |
[12] | Pyatigorskaya N , Gallea C , Garcia-Lorenzo D , Vidailhet M , Lehericy S ((2014) ) A review of the use of magnetic resonance imaging in Parkinson’s disease. Ther Adv Neurol Disord 7: , 206–220. |
[13] | Banwinkler M , Dzialas V , Hoenig MC , van Eimeren T ((2022) ) Gray matter volume loss in proposed brain-first and body-first Parkinson’s disease subtypes, Mov Disord 37: ), 2066–2074. |
[14] | Uribe C , Segura B , Baggio HC , Abos A , Garcia-Diaz AI , Campabadal A , Marti MJ , Valldeoriola F , Compta Y , Tolosa E , Junque C ((2018) ) Cortical atrophy patterns in early Parkinson’s disease patients using hierarchical cluster analysis. Parkinsonism Relat Disord 50: , 3–9. |
[15] | Depierreux F , Parmentier E , Mackels L , Baquero K , Degueldre C , Balteau E , Salmon E , Phillips C , Bahri MA , Maquet P , Garraux G ((2021) ) Parkinson’s disease multimodal imaging: F-DOPA PET, neuromelanin-sensitive and quantitative iron-sensitive MRI. NPJ Parkinsons Dis 7: , 57. |
[16] | Atkinson-Clement C , Pinto S , Eusebio A , Coulon O ((2017) ) Diffusion tensor imaging in Parkinson’s disease: Review and meta-analysis. Neuroimage Clin 16: , 98–110. |
[17] | Oltra J , Uribe C , Campabadal A , Inguanzo A , Monté-Rubio GC , Martí MJ , Compta Y , Valldeoriola F , Junque C , Segura B ((2022) ) Sex differences in brain and cognition in de novo Parkinson’s disease. Front Aging Neurosci 13: , 791532. |
[18] | Yadav SK , Kathiresan N , Mohan S , Vasileiou G , Singh A , Kaura D , Melhem ER , Gupta RK , Wang E , Marincola FM , Borthakur A , Haris M ((2016) ) Gender-based analysis of cortical thickness and structural connectivity in Parkinson’s disease. J Neurol 263: , 2308–2318. |
[19] | La Joie R , Perrotin A , Barre L , Hommet C , Mezenge F , Ibazizene M , Camus V , Abbas A , Landeau B , Guilloteau D , de La Sayette V , Eustache F , Desgranges B , Chetelat G ((2012) ) Region-specific hierarchy between atrophy, hypometabolism, and β-amyloid (Aβ) load in Alzheimer’s disease dementia. J Neurosci 32: , 16265–16273. |
[20] | Tremblay C , Abbasi N , Zeighami Y , Yau Y , Dadar M , Rahayel S , Dagher A ((2020) ) Sex effects on brain structure in de novo Parkinson’s disease: A multimodal neuroimaging study. Brain 143: , 3052–3066. |
[21] | Marek K , Jennings D , Lasch S , Siderowf A , Tanner C , Simuni T , Coffey C , Kieburtz K , Flagg E , Chowdhury S , Poewe W , Mollenhauer B , Klinik P , Sherer T , Frasier M , Meunier C , Rudolph A , Casaceli C , Seibyl J , Mendick S , Schuff N , Zhang Y , Toga A , Crawford K , Ansbach A , De Blasio P , Piovella M , Trojanowski J , Shaw L , Singleton A , Hawkins K , Eberling J , Brooks D , Russell D , Leary L , Factor S , Sommerfeld B , Hogarth P , Pighetti E , Williams K , Standaert D , Guthrie S , Hauser R , Delgado H , Jankovic J , Hunter C , Stern M , Tran B , Leverenz J , Baca M , Frank S , Thomas C , Richard I , Deeley C , Rees L , Sprenger F , Lang E , Shill H , Obradov S , Fernandez H , Winters A , Berg D , Gauss K , Galasko D , Fontaine D , Mari Z , Gerstenhaber M , Brooks D , Malloy S , Barone P , Longo K , Comery T , Ravina B , Grachev I , Gallagher K , Collins M , Widnell KL , Ostrowizki S , Fontoura P , Ho T , Luthman J , Brug MVD , Reith AD , Taylor P ((2011) ) The Parkinson Progression Marker Initiative (PPMI). Prog Neurobiol 95: , 629–635. |
[22] | Jia X , Ling C , Li Y , Zhang J , Li Z , Jia X , Wang DJJ , Zhang Z , Yuan Y , Yang Q ((2023) ) Sex differences in frontotemporal atrophy in CADASIL revealed by 7-Tesla MRI. Neuroimage Clin 37: , 103298. |
[23] | Rodriguez-Oroz MCM , Jahanshahi MM , Krack PM , Litvan IM , Macias RM , Bezard EP , Obeso JAM ((2009) ) Initial clinical manifestations of Parkinson’s disease: Features and pathophysiological mechanisms. Lancet Neurol 8: , 1128–1139. |
[24] | Stoodley CJ , Schmahmann JD ((2010) ) Evidence for topographic organization in the cerebellum of motor control versus cognitive and affective processing. Cortex 46: , 831–844. |
[25] | Blaszczyk JW ((1998) ) Motor deficiency in Parkinson’s disease. Acta Neurobiol Exp (Wars) 58: , 79–93. |
[26] | Chung SJ , Lee JJ , Lee PH , Sohn YH ((2020) ) Emerging concepts of motor reserve in Parkinson’s disease. J Mov Disord 13: , 171–184. |
[27] | Bastos P , Barbosa R ((2022) ) Motor reserve: How to build neuronal resilience against ageing and neurodegeneration? Rev Neurol (Paris) 178: , 845–854. |
[28] | Olsson TT , Svensson M , Hållmarker U , James S , Deierborg T ((2021) ) Delayed clinical manifestation of Parkinson’s disease among physically active: Do participants in a long-distance ski race have a motor reserve? J Parkinsons Dis 11: , 373. |
[29] | Segura B , Baggio HC , Marti MJ , Valldeoriola F , Compta Y , Garcia Diaz AI , Vendrell P , Bargallo N , Tolosa E , Junque C ((2014) ) Cortical thinning associated with mild cognitive impairment in Parkinson’s disease. Mov Disord 29: , 1495–1503. |
[30] | Georgiopoulos C , Warntjes M , Dizdar N , Zachrisson H , Engström M , Haller S , Larsson E ((2017) ) Olfactory impairment in Parkinson’s disease studied with diffusion tensor and magnetization transfer imaging. J Parkinsons Dis 7: , 301–311. |
[31] | Jia X , Wang Z , Yang T , Li Y , Gao S , Wu G , Jiang T , Liang P ((2019) ) Entorhinal cortex atrophy in early, drug-naive Parkinson’s disease with mild cognitive impairment. Aging Dis 10: , 1221. |
[32] | Goldman JG , Stebbins GT , Bernard B , Stoub TR , Goetz CG , Detoledo-Morrell L ((2012) ) Entorhinal cortex atrophy differentiates Parkinson’s disease patients with and without dementia. Mov Disord 27: , 727–734. |
[33] | Gee M , Dukart J , Draganski B , Wayne MW , Emery D , Camicioli R ((2017) ) Regional volumetric change in Parkinson’s disease with cognitive decline. J Neurol Sci 373: , 88–94. |
[34] | Diez-Cirarda M , Ojeda N , Pena J , Cabrera-Zubizarreta A , Lucas-Jimenez O , Gomez-Esteban JC , Gomez-Beldarrain MA , Ibarretxe-Bilbao N ((2018) ) Long-term effects of cognitive rehabilitation on brain, functional outcome and cognition in Parkinson’s disease. Eur J Neurol 25: , 5–12. |
[35] | Mollenhauer B , Zimmermann J , Sixel-Doring F , Focke NK , Wicke T , Ebentheuer J , Schaumburg M , Lang E , Trautmann E , Zetterberg H , Taylor P , Friede T , Trenkwalder C ((2016) ) Monitoring of 30 marker candidates in early Parkinson disease as progression markers. Neurology 87: , 168–177. |
[36] | Jia X , Liang P , Li Y , Shi L , Wang D , Li K ((2015) ) Longitudinal study of gray matter changes in Parkinson disease. AJNR Am J Neuroradiol 36: , 2219–2226. |
[37] | Picillo M , Lafontant DE , Bressman S , Caspell-Garcia C , Coffey C , Cho HR , Burghardt EL , Dahodwala N , Saunders-Pullman R , Tanner CM , Amara AW ((2022) ) Sex-related longitudinal change of motor, non-motor, and biological features in early Parkinson’s disease. J Parkinsons Dis 12: , 421–436. |
[38] | Umeh CC , Mahajan A , Mihailovic A , Pontone GM ((2022) ) APOE4 allele, sex, and dementia risk in Parkinson’s disease: Lessons from a longitudinal cohort. J Geriatr Psychiatry Neurol 35: , 810–815. |
[39] | Saunders-Pullman R , Gordon-Elliott J , Parides M , Fahn S , Saunders HR , Bressman S ((1999) ) The effect of estrogen replacement on early Parkinson’s disease. Neurology 52: , 1417–1421. |
[40] | Strijks E , Kremer JA , Horstink MW ((1999) ) Effects of female sex steroids on Parkinson’s disease in postmenopausal women. Clin Neuropharmacol 22: , 93–97. |