Body-First Subtype of Parkinson’s Disease with Probable REM-Sleep Behavior Disorder Is Associated with Non-Motor Dominant Phenotype
Abstract
Background:
The hypothesis of body-first vs. brain-first subtype of PD has been proposed with REM-Sleep behavior disorder (RBD) defining the former. The body-first PD presumes an involvement of the brainstem in the pathogenic process with higher burden of autonomic dysfunction.
Objective:
To identify distinctive clinical subtypes of idiopathic Parkinson’s disease (iPD) in line with the formerly proposed concept of body-first vs. brain-first subtypes in PD, we analyzed the presence of probable RBD (pRBD), sex, and the APOE ɛ4 carrier status as potential sub-group stratifiers.
Methods:
A total of 400 iPD patients were included in the cross-sectional analysis from the baseline dataset with a completed RBD Screening Questionnaire (RBDSQ) for classifying as pRBD by using the cut-off RBDSQ≥6. Multiple regression models were applied to explore (i) the effect of pRBD on clinical outcomes adjusted for disease duration and age, (ii) the effect of sex on pRBD, and (iii) the association of APOE ɛ4 and pRBD.
Results:
iPD-pRBD was significantly associated with autonomic dysfunction (SCOPA-AUT), level of depressive symptoms (BDI-I), MDS-UPDRS I, hallucinations, and constipation, whereas significantly negatively associated with quality of life (PDQ-39) and sleep (PDSS). No significant association between sex and pRBD or APOE ɛ4 and pRBD in iPD was found nor did we determine a significant effect of APOE ɛ4 on the PD phenotype.
Conclusion:
We identified an RBD-specific PD endophenotype, characterized by predominant autonomic dysfunction, hallucinations, and depression, corroborating the concept of a distinctive body-first subtype of PD. We did not observe a significant association between APOE ɛ4 and pRBD suggesting both factors having an independent effect on cognitive decline in iPD.
INTRODUCTION
The phenotypic heterogeneity of Parkinson’s disease (PD) has been a challenge for both clinicians and researchers for decades. Several efforts were made to identify an underlying pattern explaining this heterogeneity by subtyping PD patients. They can be grouped into two distinct methods. The first approach uses a single clinical or genetic metric determining the clinical phenotype, such as age at onset, sex, motor phenotype, or being a carrier of the PD-causing rare genetic mutations. The second approach has been using hypothesis-free data-driven models identifying phenotypic clusters in PD based on clinical symptoms, but this approach failed reproducibility checks, possibly due to a limited methodological overlap between the studies and a wide variety of clinical metrics entering the models [1]. Interestingly, both approaches systematically reported REM-sleep behavior disorder (RBD) as a relevant clinical variable. Not only is RBD currently known as the most robust prodromal marker of future pheno-conversion to the alpha-synucleinopathies (i.e., PD, dementia with Lewy bodies or multiple system atrophy) [2], but it was suggested that RBD is associated with more rapid progression of motor symptoms, a higher burden of non-motor symptoms and lower quality of life [3–5].
RBD received increasing attention in the last years, with several cross-sectional and longitudinal studies investigating the association between RBD and the clinical phenotype of PD. On the one hand, we observe an overall consensus regarding a non-motor dominant profile of PD with higher autonomic dysfunction and more rapid cognitive decline. On the other hand, prior studies have reported contradictory findings on the effect of comorbid RBD on motor progression in PD [5–8]. Moreover, genetic risk factors and PD-causing rare mutations with a substantial effect on the clinical phenotype were rarely systematically addressed in the context of concomitant RBD and PD and their effect on the severity of the clinical phenotype. Recently, the APOE epsilon4 (APOE ɛ4) genotype has been linked to faster cognitive decline and motor progression in PD [9], although studies on the role of APOE ɛ4 and clinical progression of PD remain controversial [10, 11]. Whether an additive or multiplicative potentiation effect of RBD and APOE ɛ4 on cognitive decline in PD exists has not been adequately addressed so far. Currently, no association of the APOE ɛ4 carriers status with idiopathic RBD has been observed [12, 13], but a potential role of the APOE ɛ4 genotype as a modifier of the clinical phenotype of PD with RBD has not yet been explored.
RBD has been suggested to represent a key element in distinguishing body-first from brain-first subtype of PD, a concept recently proposed to explain the phenotypic differences and variability of dynamics in PD and supported by several clinical and imaging studies [14, 15]. It has been proposed that the body-first subtype of PD starts in the peripheral nervous system with spreading of neurodegeneration via brainstem thus associated with RBD, higher burden of autonomic dysfunction and higher rate of cognitive decline [16].
In order to test the hypothesis of body-first subtype of PD with comorbid pRBD, we used a large baseline visit dataset from the Luxembourg Parkinson’s Study, a monocentric longitudinal observational study with a previously described recruitment design [17]. In our study, we primarily aimed to determine the effect of pRBD on clinical outcomes in idiopathic PD (iPD) by excluding known PD-linked rare mutations or genetic risk variant carriers. Next, we investigated potential confounding effects of sex and the APOE ɛ4 carrier status as potential stratifiers of iPD.
MATERIALS AND METHODS
Study population
The data used in this study were acquired from participants recruited in the frame of the nationwide monocentric observational longitudinal Luxembourg Parkinson’s Study [17]. The diagnosis of PD relied on the UK Parkinson’s Disease Society Brain Bank (UKPDSBB) diagnostic criteria [18]. All participants were genotyped for disease-causing mutations and PD-associated risk variants using both NeuroChip® and PacBio sequencing. Available data on RBDSQ were analyzed after excluding six PD patients for 1st, 2nd, and 3rd degree relationships and after excluding 49 PD patients carrying PD-associated mutations. The overall study design, inclusion, and exclusion workflow are illustrated in Fig. 1. Though the diagnostic gold standard of RBD remains polysomnography (PSG) [19], the accessibility of the sleep laboratory and performing PSG on a large scale is problematic due to the sleep laboratory capacities and costs. We therefore applied a classification of probable RBD (pRBD) by REM-sleep behavior disorder screening questionnaire (RBDSQ) as used in several previous studies [20–24]. The group assignment of pRBD in iPD individuals uses the criterion RBDSQ≥6 to optimize the specificity and sensitivity for pRBD in line with the Oxford Discovery Study [24].
Fig. 1
Description of the study design and study dataset. PD, individuals with Parkinson’s disease; iPD, idiopathic Parkinson’s disease; pRBD, probable REM-sleep behavior disorder; RBDSQ, REM sleep behavior disorder screening questionnaire.
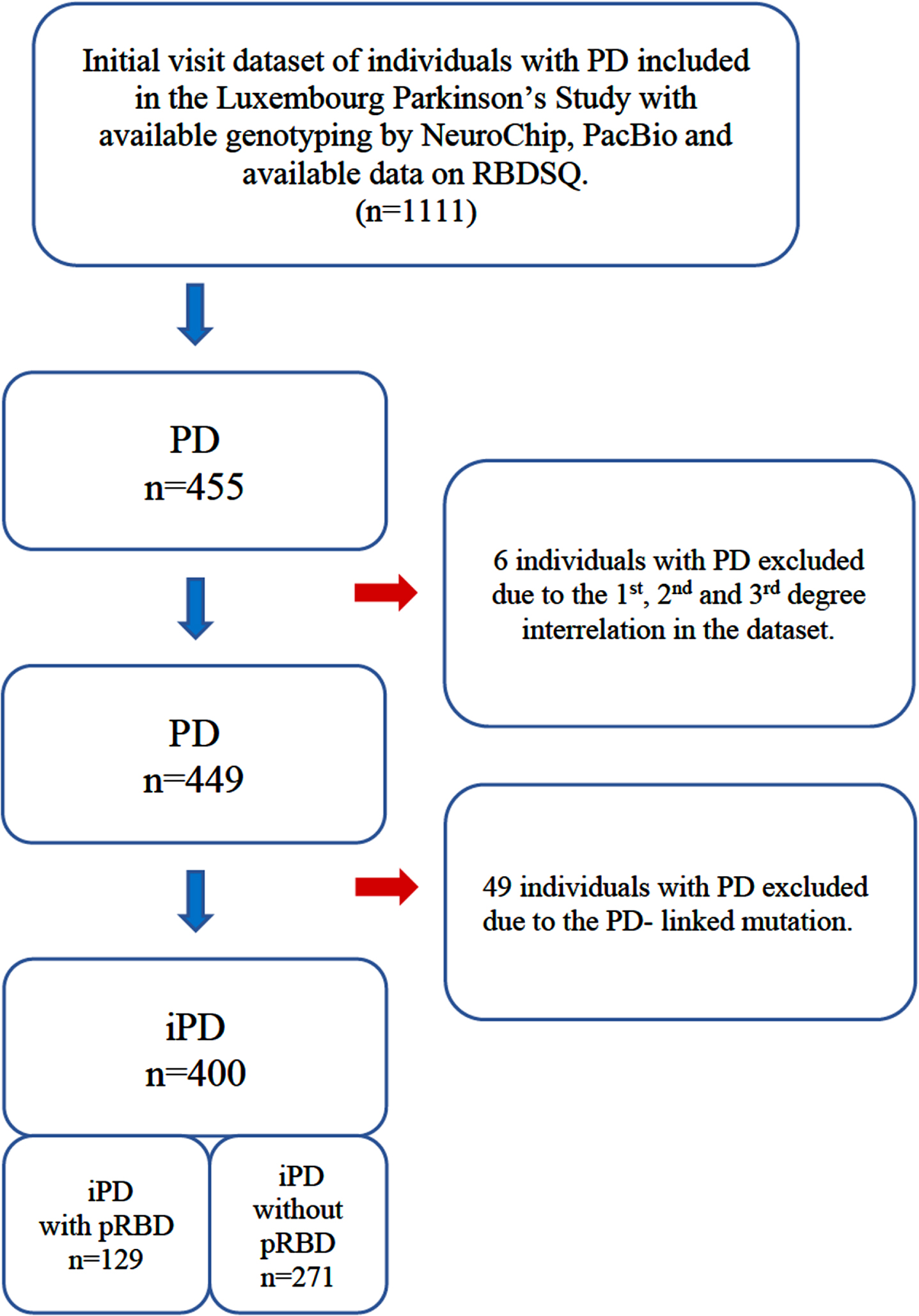
All participants taking part in the Luxembourg Parkinson’s Study agreed and signed a written informed consent. The study has been approved by the National Research Ethics Committee (CNER Ref: 201407/13).
Clinical assessment and data
The design and recruitment of the Luxembourg Parkinson’s Study were previously published in detail [17]. Sociodemographic characteristics and clinical outcomes validated for PD were chosen from the basic clinical assessment battery and are listed in Tables 1 and 2. All patients have been evaluated in medication ON state and, where applicable, in deep brain stimulation ON state. The clinical symptoms as scales are defined in detail in the Supplementary Material.
Table 1
Descriptive and comparative statistics of demographic data and frequency of APOE ɛ4 genotype in PD individuals with (right) and without (left) probable REM-sleep behavior disorder (pRBD). For intergroup comparisons, p-values are shown from Mann-Whitney U test for numerical variables and Fisher’s exact test for binary variables. Binary variables are annotated by asterisk. Results are shown as mean and standard deviation (SD) for numerical variables, number of zeros (’NO’) and ones (’YES’) for binary variables and percentage of YES, and number of missing values (NA). Single and double ticks indicate significance at the 5% level, and the Bonferroni-adjusted 5% level. Age at onset was calculated based on the year of the PD diagnosis. PD, Parkinson’s disease
PD non-pRBD (n = 271) | PD pRBD (n = 129) | ||||||
Mean or | SD or | NA | Mean or | SD or | NA | p | |
YES in % | NO/YES | YES in % | NO/YES | ||||
Disease duration since diagnosis (y) | 4.20 | 4.55 | 0 | 7.86 | 6.36 | 0 | 8.2e-11′′ |
Age at assessment (y) | 66.19 | 11.29 | 0 | 68.31 | 9.85 | 0 | 1.2e-01 |
Age at onset (y) | 62.01 | 11.64 | 0 | 60.48 | 11.98 | 0 | 2.5e-01 |
Sex (male)* | 65% | 96/175 | 0 | 74% | 34/95 | 0 | 8.6e-02 |
APOE (ɛ2/ ɛ4; ɛ3/ ɛ4;ɛ4/ɛ4)* | 21% | 213/58 | 0 | 26% | 95/34 | 0 | 3.1e-01 |
Years of education | 13.29 | 4.12 | 0 | 12.99 | 3.90 | 0 | 6.7e-01 |
Total languages spoken | 2.86 | 1.06 | 0 | 2.89 | 1.04 | 0 | 8.0e-01 |
Table 2
Descriptive and comparative statistics of clinical outcomes for iPD group with and without probable REM-sleep behavior disorder (pRBD). Results are shown as mean and standard deviation (SD) for numerical variables, number of zeros (’NO’) and ones (’YES’) for binary variables and percentage of YES, and number of missing values (NA). For intergroup comparisons, p-values are shown from Mann-Whitney U test for numerical variables and Fisher’s exact test for binary variables. Binary variables are annotated by asterisk. Single and double ticks indicate significance at the 5% level, and the Bonferroni-adjusted 5% level. All clinical outcomes are defined and described in the Supplementary Material
PD non-pRBD (n = 271) | PD pRBD (n = 129) | ||||||
Mean or | SD or | NA | Mean or | SD or | NA | p | |
YES in % | NO/YES | YES in % | NO/YES | ||||
H&Y | 2.12 | 0.78 | 2 | 2.37 | 0.75 | 0 | 1.2e-04′′ |
MDS-UPDRS III | 32.00 | 16.11 | 5 | 38.02 | 16.76 | 2 | 4.5e-04′′ |
MDS-UPDRS II | 9.79 | 7.45 | 3 | 14.50 | 8.64 | 3 | 1.0e-07′′ |
LEDD (g/day) | 0.45 | 0.38 | 0 | 0.68 | 0.41 | 0 | 2.8e-08′′ |
Gait disorder* | 48% | 141/130 | 0 | 71% | 37/92 | 0 | 1.0e-05′′ |
Repetitive falls* | 11% | 240/31 | 0 | 29% | 91/38 | 0 | 1.7e-05′′ |
MDS-UPDRS IV | 1.37 | 3.01 | 2 | 2.75 | 3.98 | 3 | 5.2e-05′′ |
Dyskinesia/day (hours) | 0.47 | 2.29 | 0 | 1.21 | 3.57 | 1 | 9.3e-05′′ |
OFF time/day (hours) | 0.40 | 1.41 | 0 | 0.72 | 1.38 | 2 | 3.2e-04′′ |
Dystonia/day (hours) | 0.027 | 0.15 | 1 | 0.088 | 0.31 | 1 | 7.3e-03′ |
Dyskinesia* | 9% | 246/25 | 0 | 20% | 103/26 | 0 | 3.5e-03′ |
Motor fluctuations* | 11% | 241/30 | 0 | 27% | 94/35 | 0 | 8.1e-05′′ |
Freezing of gait* | 16% | 227/44 | 0 | 34% | 85/44 | 0 | 9.4e-05′′ |
MoCA | 24.85 | 3.93 | 5 | 24.02 | 4.45 | 2 | 6.9e-02 |
Sniffin’ stick test | 8.52 | 3.34 | 7 | 7.50 | 3.27 | 3 | 1.0e-02′ |
PDQ-39 | 33.65 | 23.88 | 12 | 52.23 | 27.05 | 6 | 7.2e-11′′ |
SCOPA-AUT | 12.59 | 6.97 | 2 | 19.59 | 8.11 | 0 | 6.7e-15′′ |
MDS-UPDRS I | 8.54 | 5.78 | 6 | 13.62 | 7.36 | 4 | 5.1e-12′′ |
BDI-I | 8.79 | 6.65 | 7 | 12.62 | 7.33 | 3 | 6.2e-08′′ |
Starkstein Apathy Scale | 13.46 | 5.31 | 4 | 14.67 | 6.24 | 3 | 1.2e-01 |
PDSS | 111.40 | 21.55 | 4 | 92.64 | 23.05 | 3 | 2.3e-13′′ |
Probable RBD* | 0% | 271/0 | 0 | 100% | 0/129 | 0 | 1.4e-108′′ |
Excessive daily sleepiness* | 23% | 208/63 | 0 | 41% | 76/53 | 0 | 3.8e-04′′ |
Insomnia* | 24% | 205/66 | 0 | 21% | 102/27 | 0 | 5.3e-01 |
Hallucinations* | 9% | 247/24 | 0 | 29% | 91/38 | 0 | 4.8e-07′′ |
Impulse Control Disorder* | 6% | 255/16 | 0 | 16% | 108/21 | 0 | 1.4e-03′ |
Orthostatic hypotension* | 23% | 210/61 | 0 | 36% | 82/47 | 0 | 3.9e-03′ |
Dysphagia* | 20% | 218/53 | 0 | 33% | 87/42 | 0 | 5.6e-03′ |
Constipation* | 31% | 187/84 | 0 | 63% | 48/81 | 0 | 2.8e-09′′ |
Urinary Incontinence* | 27% | 197/74 | 0 | 39% | 79/50 | 0 | 2.8e-02′ |
Missing data statement
The absolute number of missing data per variable is described in Tables 1 and 2. Given the low proportions of missing values in the dataset, we used a pairwise deletion for all statistical models.
Genotyping and quality-control analyses
The methods for genotyping in our dataset have been described previously [25]. PD causing rare variants were defined by the ClinVar classification as “pathogenic/likely pathogenic”. All PD-causing variants (listed in the Supplementary Material) identified by any method were Sanger validated, and all samples with a validated PD-causing variant were excluded from further analysis with a list of excluded variants described in the Supplementary Material.
APOE genotyping
APOE genotypes were called for all individuals from two SNPs investigated by NeuroChip array (rs429358, rs7412) that distinguish the ɛ2, ɛ3, and ɛ4 alleles classifying the respective APOE carriers. The NeuroChip provides high accuracy of 98.1% for genotyping of APOE ɛ4 [26], and the approach was aligned with other large studies [27].
Statistical analysis
Mann-Whitney’s U test was used for numerical variables and Fisher’s exact test for binary variables in intergroup comparison analyses (iPD pRBD vs. iPD non-pRBD; male sex iPD vs. female sex iPD). Multiple linear and logistic regression models were applied to investigate the effect of pRBD on clinical outcomes in iPD, adjusted for age at assessment (AAA) and disease duration. To investigate the potential effect of the APOE genotype on clinical outcomes, we pooled the heterozygotes (ɛ2/ɛ4; ɛ3/ɛ4) and homozygotes (ɛ4/ɛ4), allowing us to quantify a potential association between APOE ɛ4 genotype and pRBD in iPD. Furthermore, we applied regression of clinical symptoms in PD on APOE ɛ4, AAA and disease duration. For all analyses, we assessed significance at the 5% level and the Bonferroni-adjusted 5% level.
RESULTS
Frequency of pRBD and effect of pRBD on clinical outcomes in iPD
According to the RBDSQ classification of pRBD, we observed a relative pRBD frequency of 32.3% in the iPD group (129 iPD pRBD out of 400). The demographic characteristics of iPD pRBD (n = 129) and iPD non-pRBD patients (n = 271) are shown in Tables 1 and 2. We investigated the effect of pRBD on the clinical outcomes adjusted for AAA and disease duration.
As key results, we observed a significant positive association between iPD pRBD (as opposed to iPD non-pRBD) and burden of non-motor symptoms, i.e., autonomic dysfunction (SCOPA-AUT) and frequency of constipation; MDS-UPDRS I, burden of depression symptoms assessed by BDI-I, frequency of hallucinations and PDQ-39, showing lower quality of life in iPD pRBD, as demonstrated in Fig. 2. Furthermore, a significant negative association was determined between iPD pRBD and the Parkinson’s Disease Sleep Scale (PDSS), indicating lower quality of sleep in the group of iPD pRBD vs. iPD non-pRBD. Other considered clinical outcomes showed no significant associations after multiple testing correction.
Fig. 2
Multiple regression model for investigating effect of probable REM-Sleep behavior disorder on clinical outcomes in idiopathic Parkinson’s disease adjusted for age at assessment and disease duration. Forrest plot with estimated coefficients and corresponding confidence intervals (±1.96 x standard error) for pRBD, from linear/logistic regression of numerical/binary outcome on disease duration, age at assessment (AAA) and pRBD (binary outcomes are annotated by asterisk). The color blue indicates significant negative effects of pRBD on the clinical outcome, and the color red indicates significant positive effects at the Bonferroni-adjusted 5% level. Clinical symptoms and scales are described in the Supplementary Material.
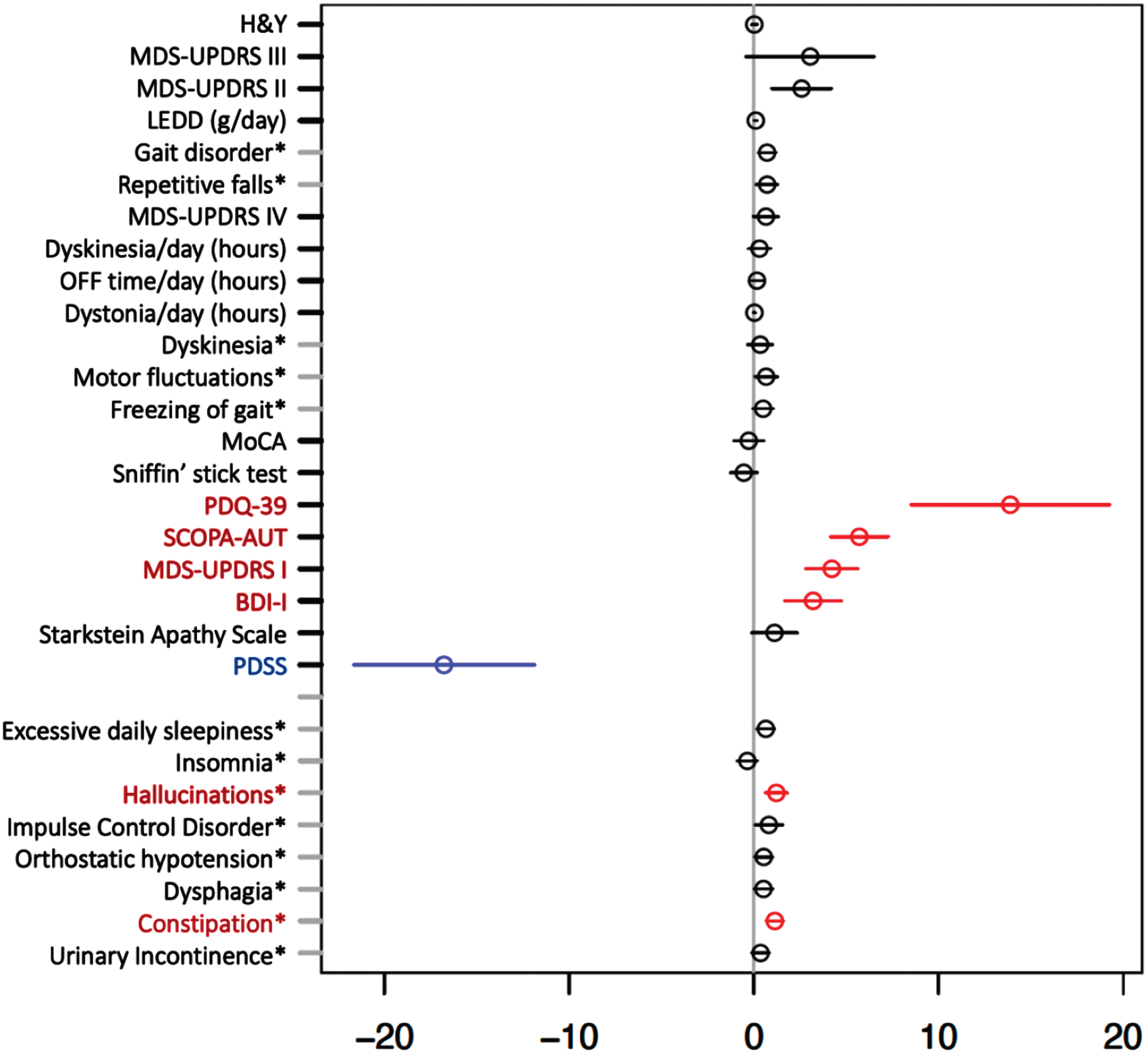
APOE genotype and iPD pRBD
We found no significant association between pooled heterozygote and homozygote APOE ɛ4 carriers and iPD with pRBD. Additionally, no significant association was observed between APOE ɛ4 and the clinical outcomes of iPD with pRBD vs. iPD non-pRBD adjusted for AAA and disease duration, as shown in Fig. 3.
Fig. 3
Multiple regression model investigating effect of APOE ɛ4 carrier status on clinical outcomes in idiopathic Parkinson’s disease adjusted for age at assessment and disease duration. Forrest plot with estimated coefficients and corresponding confidence intervals (±1.96 x standard error) for APOE ɛ4 genotype, from linear/logistic regression of numerical/binary outcome on disease duration, age at assessment (AAA), and APOE (binary outcomes are annotated by asterisk). The color blue indicates significant negative effects of APOE ɛ4 genotype on the clinical outcome, and the color red indicates significant positive effects at the Bonferroni-adjusted 5% level. Clinical symptoms and scales are described in the Supplementary Material.
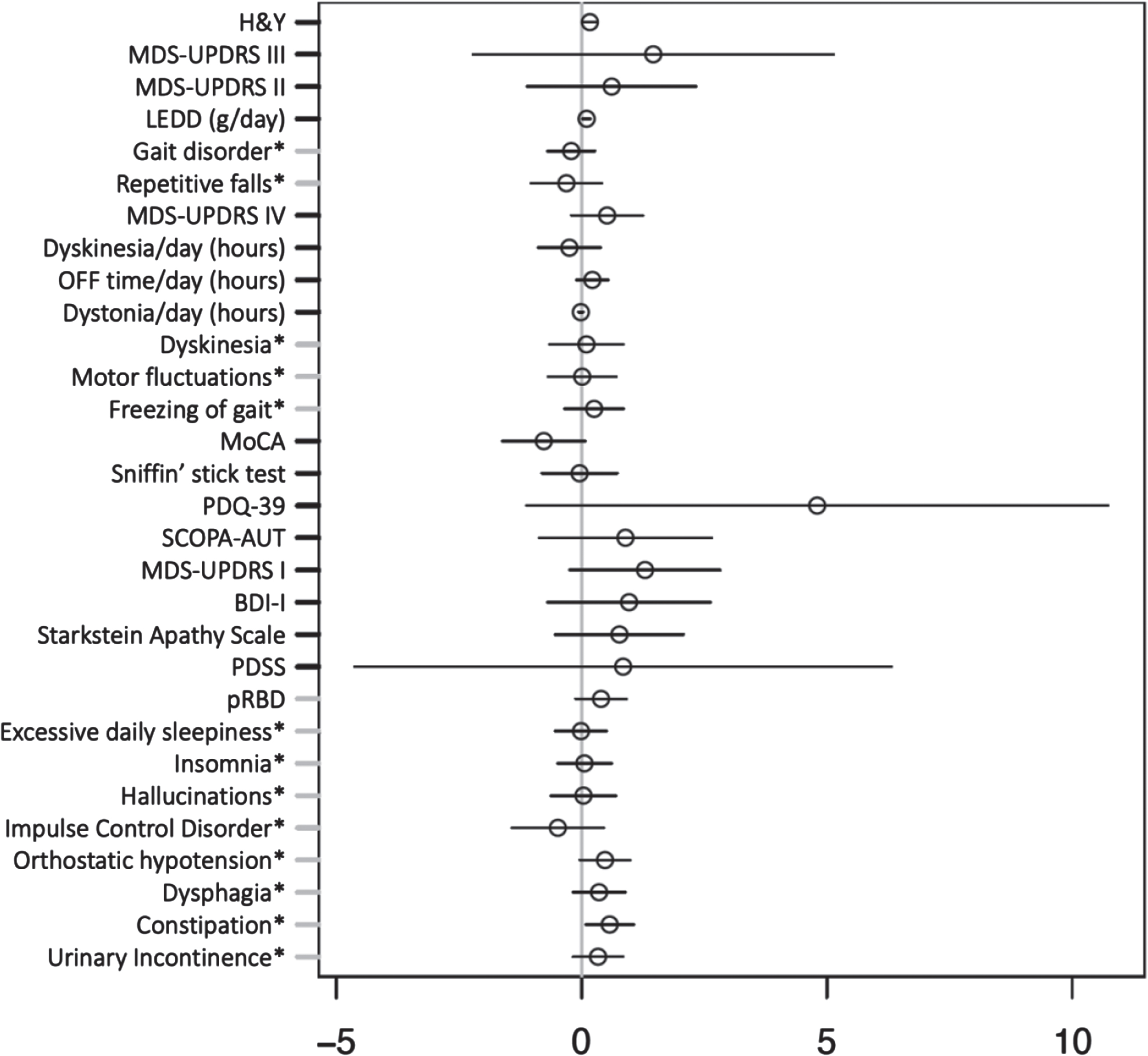
Effect of sex on frequency of pRBD and other clinical outcomes in iPD
Clinical and demographic characteristics and outcomes of sex-stratified iPD are shown in Table 3. We did not observe a significant effect of male sex on the frequency of pRBD in iPD. Interestingly, from all the putative variables, only olfactory performance (measured by Sniffin’ Stick test) was significantly negatively, and FOG significantly positively associated with male sex in PD after adjustment for AAA and disease duration (see Fig. 4).
Table 3
Descriptive statistics for sex stratified iPD. Results are shown as mean and standard deviation (SD) for numerical variables, number of zeros (’NO’) and ones (’YES’) for binary variables and percentage of YES, and number of missing values (NA). The last column shows p-values from Mann-Whitney U test for numerical variables and Fisher’s exact test for binary variables. Binary variables are annotated by asterisk. Single and double ticks indicate significance at the 5% level and the Bonferroni-adjusted 5% level. Age at onset was calculated based on the year of the PD diagnosis
PD female (n = 130) | PD male (n = 270) | ||||||
Mean or | SD or | NA | Mean or | SD or | NA | p | |
YES in % | NO/YES | YES in % | NO/YES | ||||
Disease duration since diagnosis (y) | 5.44 | 5.53 | 0 | 5.35 | 5.46 | 0 | 8.1e-01 |
Age at assessment (y) | 66.71 | 10.74 | 0 | 66.95 | 10.97 | 0 | 9.1e-01 |
Age at onset (y) | 61.30 | 11.03 | 0 | 61.62 | 12.11 | 0 | 8.6e-01 |
H&Y | 2.21 | 0.84 | 1 | 2.20 | 0.75 | 1 | 9.3e-01 |
MDS-UPDRS III | 33.49 | 18.03 | 2 | 34.17 | 15.81 | 5 | 4.3e-01 |
MDS-UPDRS II | 11.09 | 8.38 | 2 | 11.40 | 8.04 | 4 | 4.7e-01 |
LEDD (g/day) | 0.47 | 0.36 | 0 | 0.55 | 0.42 | 0 | 1.2e-01 |
Gait disorder* | 50% | 65/65 | 0 | 58% | 113/157 | 0 | 1.3e-01 |
Repetitive falls* | 20% | 104/26 | 0 | 16% | 227/43 | 0 | 3.2e-01 |
MDS-UPDRS IV | 1.90 | 3.61 | 4 | 1.77 | 3.30 | 1 | 9.3e-01 |
Dyskinesia/day (h) | 0.87 | 3.22 | 1 | 0.63 | 2.55 | 0 | 8.6e-01 |
OFF time/day (h) | 0.55 | 1.91 | 2 | 0.48 | 1.10 | 0 | 7.3e-01 |
Dystonia/day (h) | 0.035 | 0.17 | 2 | 0.052 | 0.24 | 0 | 1.0e-01 |
Dyskinesia* | 12% | 115/15 | 0 | 13% | 234/36 | 0 | 7.5e-01 |
Motor fluctuations* | 11% | 116/14 | 0 | 19% | 219/51 | 0 | 4.3e-02′ |
Freezing of gait* | 13% | 113/17 | 0 | 26% | 199/71 | 0 | 2.9e-03′ |
MoCA | 24.92 | 3.84 | 3 | 24.41 | 4.24 | 4 | 3.4e-01 |
Sniffin’ stick test | 9.10 | 3.26 | 4 | 7.76 | 3.30 | 6 | 2.2e-04′′ |
PDQ-39 | 43.28 | 26.38 | 8 | 37.92 | 26.26 | 10 | 4.0e-02′ |
SCOPA-AUT | 14.92 | 8.01 | 2 | 14.83 | 8.08 | 0 | 1.0e+00 |
MDS-UPDRS I | 10.22 | 6.32 | 3 | 10.14 | 6.96 | 7 | 5.5e-01 |
BDI-I | 11.20 | 7.75 | 4 | 9.47 | 6.71 | 6 | 2.9e-02′ |
Starkstein Apathy Scale | 13.84 | 5.77 | 6 | 13.86 | 5.60 | 1 | 9.7e-01 |
PDSS | 102.64 | 25.08 | 4 | 106.68 | 22.94 | 3 | 1.3e-01 |
Probable RBD* | 26% | 96/34 | 0 | 35% | 175/95 | 0 | 8.6e-02 |
Excessive daily sleepiness* | 20% | 104/26 | 0 | 33% | 180/90 | 0 | 6.7e-03′ |
Insomnia* | 27% | 95/35 | 0 | 21% | 212/58 | 0 | 2.6e-01 |
Hallucinations* | 16% | 109/21 | 0 | 15% | 229/41 | 0 | 8.8e-01 |
Impulse Control Disorder* | 7% | 121/9 | 0 | 10% | 242/28 | 0 | 3.6e-01 |
Orthostatic hypotension* | 27% | 95/35 | 0 | 27% | 197/73 | 0 | 1.0e+00 |
Dysphagia* | 26% | 96/34 | 0 | 23% | 209/61 | 0 | 4.5e-01 |
Constipation* | 40% | 78/52 | 0 | 42% | 157/113 | 0 | 7.5e-01 |
Urinary Incontinence* | 30% | 91/39 | 0 | 31% | 185/85 | 0 | 8.2e-01 |
Fig. 4
Multiple regression model investigating effect of sex on clinical outcomes in idiopathic Parkinson’s disease adjusted for age at assessment and disease duration. Forrest plot with estimated coefficients and corresponding confidence intervals (±1.96 x Standard error) for sex, from linear/logistic regression of numerical/binary outcome on disease duration, AAA, and sex (binary outcomes are annotated by asterisk). The color blue indicates significant negative effects of male vs. female sex on the clinical outcome, and the color red indicates significant positive effects at the Bonferroni-adjusted 5% level. Clinical symptoms and scales are described in the Supplementary Material.
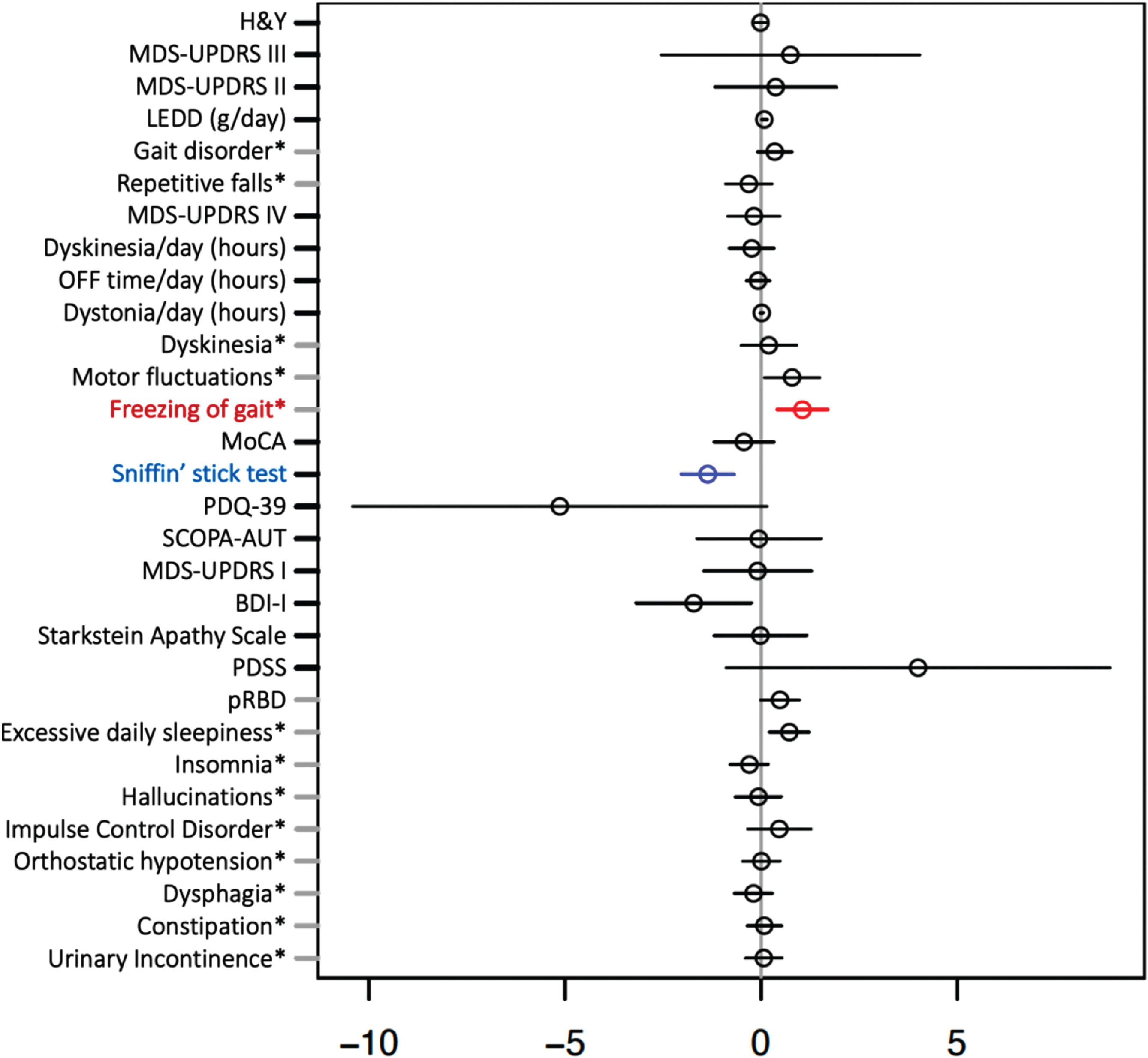
Effect of education and number of spoken languages on cognitive performance
We analyzed a potential confounding effect of the years of education (YoE) and the total languages spoken (TLS) on cognitive performance in our dataset. As shown in the Supplementary Table 1, only YoE (not TLS) had a significant positive effect on Montreal Cognitive Assessment (MoCA) in a multiple regression model adjusted for AAA and disease duration.
DISCUSSION
The results of our study support the classification of RBD as a distinctive characteristic of the body-first subtype by identifying a significant association of iPD pRBD with the non-motor dominant disease profile, a result that matched remarkably well with the majority of previous studies [4–8]. It favors the concept of pathological process beginning in the peripheral nervous system with further centripetal spreading of alpha-synuclein in a subgroup of PD patients and hence the associated neurodegeneration causing a significantly higher autonomic dysfunction, higher depression burden as well as hallucinations through dysregulation of dopaminergic and noradrenergic system in the brainstem. Although we assessed RBD via a screening questionnaire, our results were consistent with a prior study using PSG-proven RBD, which indicated an association of a non-motor dominant phenotype in PD with PSG-proven RBD [4]. However, we observed only a trend in the negative effect of pRBD on global cognitive performance in PD, which did not correspond to several cross-sectional and longitudinal studies [8]. To assess a potential independent variable influencing cognitive performance, we identified a protective effect of YoE on cognitive decline in the overall PD group, but we did not identify a significant difference in pRBD PD vs. non-pRBD PD in terms of YoE or TLS. Therefore, we did not consider these two factors (YoE and TLS) as confounding factors for the effect of pRBD on cognitive performance assessed by MoCA in our dataset. Moreover, the APOE ɛ4 genotype, known to exacerbate beta amyloid pathology in Alzheimer’s disease, has been suggested to play a role in accelerated cognitive decline in PD [27, 28]. As RBD was associated with a higher rate of cognitive decline and dementia in previous studies, we explored a potential association between pRBD and APOE ɛ4 carrier status. However, no significant association between the two was observed in our study. This would argue for an independent effect of pRBD and APOE ɛ4 status without a synergistic effect on cognitive decline in iPD. Therefore, we conclude that APOE ɛ4 genotype might not play a role as a stratifier in body-first vs. brain-first concept. It is important to stress that we excluded a potential effect of PD-linked genetic mutations and genetic risk factors for PD, which may have contributed to confounding effects on clinical phenotype in other studies, as in the case of highly prevalent mutations in the GBA gene [29].
Our investigation of potential sex-related differences in iPD phenotype did not reveal a significant association between pRBD and male sex, as suggested by several prior studies using either a similar screening questionnaire approach or PSG [30–32]. This adds to the open debate about whether there are significant differences in the prevalence of RBD in males vs. females. We would like to point out that the higher frequency of RBD in males was observed in studies using the dataset of individuals referred primarily to sleep laboratories which may cause a referral bias, given the fact that males are reported to have more violent RBD symptoms and are therefore more likely to be referred for PSG [33–36].
Next, we studied the potential confounding effects of sex on other motor and non-motor symptoms. We observed a higher frequency of males vs. females in the overall PD group (67.5% vs. 32.5%), in line with the results from recently published large cohort studies [37–39]. Interestingly, we found only olfactory dysfunction and FOG to be positively associated with males, while other putative motor and non-motor outcomes showed no significant associations with sex after multiple testing correction. These findings might indicate that sex does not play a substantial role in defining the phenotype of iPD and thus do not account for the phenotypic differences associated with pRBD.
Our study displays several specific strengths: (i) a large dataset was analyzed relative to previous studies; (ii) PD cases were genetically stratified by NeuroChip and targeted sequencing of GBA, avoiding a potential confounding by PD-causing mutations that are known to significantly influence the clinical phenotype; (iii) the study design included all disease stages of PD regardless of the cognitive status, and (iv) a monocentric data collection assured the consistency of the dataset.
Conversely, some limitations of our study should also be noted: We investigated the research questions using a cross-sectional analysis, and further studies on longitudinal data are still warranted. Additionally, RBD was not assessed by gold standard PSG but by a more accessible method using a screening questionnaire, potentially including in part false positive patients for RBD with another sleep pathology. Furthermore, the presence of hallucinations might be wrongly considered by the patients to classify as RBD symptoms. Nevertheless, the association of RBD in PD with hallucinations has been widely reported in the literature [40–42], thus we do not consider the significant positive association of pRBD with hallucinations in our dataset as a potential mis-classifier of pRBD vs. non-pRBD. Finally, we did not have complementary data on the time relation between pRBD and PD, i.e., describing whether pRBD preceded PD or evolved during the clinical phase of PD.
However, the overall concordance of the results on the association of pRBD in PD with a non-motor dominant phenotype indicates that applying RBDSQ may provide a useful tool for patient stratification in future studies and clinical trials. It might prove to be a clinically relevant mean to screen for pRBD during the regular follow-up of PD patients in order to personalize and adapt the therapy and its potential secondary effects by the treating physicians. Finally, this study adds to the prior body of evidence that PD subtyping, in general, may serve the patient by providing treatment-relevant phenotype-genotype stratifications as a tool for future clinical trials.
ACKNOWLEDGMENTS
We would like to give special thanks to all participants in the Luxembourg Parkinson’s Study. Furthermore, we acknowledge the joint effort of the NCER-PD consortium members generally contributing to the Luxembourg Parkinson’s Study as listed below:
Geeta ACHARYA2, Gloria AGUAYO2, Myriam ALEXANDRE2, Muhammad ALI1, Wim AMMERLANN2, Rudi BALLING1, Michele BASSIS1, Katy BEAUMONT2, Regina BECKER1, Camille BELLORA2, Guy BERCHEM3, Daniela BERG11, Alexandre BISDORFF5, Kathrin BROCKMANN11, Jessica CALMES2, Lorieza CASTILLO2, Gessica CONTESOTTO2, Giuseppe ARENA1, Nico DIEDERICH3, Rene DONDELINGER5, Daniela ESTEVES2, Guy FAGHERAZZI2, Jean-Yves FERRAND2, Manon GANTENBEIN2, Thomas GASSER11, Piotr GAWRON1, Soumyabrata GHOSH1, Marijus GIRAITIS2,3, Enrico GLAAB1, Clarissa GOMES1, Elisa GÓMEZ DE LOPE1, Jérôme GRAAS2, Mariella GRAZIANO17, Valentin GROUES1, Anne GRÜNEWALD1, Wei GU1, Gaël HAMMOT2, Anne-Marie HANFF2, Linda HANSEN1,3, Maxime HANSEN1,3, Michael HENEKA1, Estelle HENRY2, Sylvia HERBRINK6, Sascha HERZINGER1, Michael HEYMANN2, Michele HU8, Alexander HUNDT2, Ivana PACCOUD2, Nadine JACOBY18, Jacek JAROSLAW LEBIODA1, Yohan JAROZ1, Quentin KLOPFENSTEIN1, Jochen KLUCKEN1,2,3, Rejko KRÜGER1,2,3, Pauline LAMBERT2, Zied LANDOULSI1, Roseline LENTZ7, Inga LIEPELT11, Robert LISZKA14, Laura LONGHINO3, Victoria LORENTZ2, Paula Cristina LUPU2, Clare MACKAY10, Walter MAETZLER15, Katrin MARCUS13, Guilherme MARQUES2, Tainá MARQUES1, Patricia MARTINS CONDE1, Patrick MAY1, Deborah MCINTYRE2, Chouaib MEDIOUNI2, Francoise MEISCH1, Myriam MENSTER2, Maura MINELLI2, Michel MITTELBRONN1,4, Brit MOLLENHAUER12, Carlos MORENO1, Friedrich MÜHLSCHLEGEL4, Romain NATI3, Ulf NEHRBASS2, Sarah NICKELS1, Beatrice NICOLAI3, Jean-Paul NICOLAY19, Fozia NOOR2, Marek OSTASZEWSKI1, Sinthuja PACHCHEK1, Claire PAULY1,3, Laure PAULY1, Lukas PAVELKA1,2,3, Magali PERQUIN2, Rosalina RAMOS LIMA2, Armin RAUSCHENBERGER1, Rajesh RAWAL1, Dheeraj REDDY BOBBILI1, Eduardo ROSALES2, Isabel ROSETY1, Kirsten RUMP1, Estelle SANDT2, Stefano SAPIENZA1, Venkata SATAGOPAM1, Margaux SCHMITT2, Sabine SCHMITZ1, Reinhard SCHNEIDER1, Jens SCHWAMBORN1, Jean-Edouard SCHWEITZER1, Amir SHARIFY2, Ekaterina SOBOLEVA1, Kate SOKOLOWSKA2, Olivier TERWINDT1,3, Hermann THIEN2, Elodie THIRY3, Rebecca TING JIIN LOO1, Christophe TREFOIS1, Johanna TROUET2, Olena TSURKALENKO2, Michel VAILLANT2, Mesele VALENTI2, Sijmen VAN SCHAGEN1, Liliana VILAS BOAS3, Maharshi VYAS1, Richard WADE-MARTINS9, Paul WILMES1, Evi WOLLSCHEID-LENGELING1, Gelani ZELIMKHANOV3
1Luxembourg Centre for Systems Biomedicine, University of Luxembourg, Esch-sur-Alzette, Luxembourg
2Luxembourg Institute of Health, Strassen, Luxembourg
3Centre Hospitalier de Luxembourg, Strassen, Luxembourg
4Laboratoire National de Santé, Dudelange, Luxembourg
5Centre Hospitalier Emile Mayrisch, Esch-sur-Alzette, Luxembourg
6Centre Hospitalier du Nord, Ettelbrück, Luxembourg
7Parkinson Luxembourg Association, Leudelange, Luxembourg
8Oxford Parkinson’s Disease Centre, Nuffield Department of Clinical Neurosciences, University of Oxford, Oxford, UK
9Oxford Parkinson’s Disease Centre, Department of Physiology, Anatomy and Genetics, University of Oxford, Oxford, UK
10Oxford Centre for Human Brain Activity, Wellcome Centre for Integrative Neuroimaging, Department of Psychiatry, University of Oxford, Oxford, UK
11Center of Neurology and Hertie Institute for Clinical Brain Research, Department of Neurodegenerative Diseases, University Hospital Tübingen, Tübingen, Germany
12Paracelsus-Elena-Klinik, Kassel, Germany
13Ruhr-University of Bochum, Bochum, Germany
14Westpfalz-Klinikum GmbH, Kaiserslautern, Germany
15Department of Neurology, University Medical Center Schleswig-Holstein, Kiel, Germany
16Department of Neurology Philipps, University Marburg, Marburg, Germany
17Association of Physiotherapists in Parkinson’s Disease Europe, Esch-sur-Alzette, Luxembourg
18Private practice, Ettelbruck, Luxembourg
19Private practice, Luxembourg, Luxembourg
Funding sources
The National Centre of Excellence in Research on Parkinson’s Disease (NCER-PD) is funded by the Luxembourg National Research Fund (FNR/NCER13/BM/11264123), the PEARL program (FNR/P13/6682797 to RK), FNR grant projects PD-Strat (INTER/11651464 to EG), DIGIPD (ERAPERMED 2020-314 to EG); MotaSYN (12719684 to RK), MAMaSyn (to RK), MiRisk-PD (C17/BM/11676395 to RK, EG, PM), the FNR/DFG Core INTER (ProtectMove, FNR11250962 to PM), and the PARK-QC DTU (PRIDE17/12244779/PARK-QC to RK, SP).
CONFLICT OF INTEREST
All authors have no conflict of interest to report.
DATA AND CODE AVAILABILITY STATEMENT
The dataset for this manuscript is not publicly available as it is linked to the Luxembourg Parkinson’s Study and its internal regulations. Any requests for accessing the dataset can be directed to [email protected]. The code for the statistical models is available at: https://doi.org/10.17881/sw04-1w80.
SUPPLEMENTARY MATERIAL
[1] The supplementary material is available in the electronic version of this article: https://dx.doi.org/10.3233/JPD-223511.
REFERENCES
[1] | Berg D , Borghammer P , Fereshtehnejad SM , Heinzel S , Horsager J , Schaeffer E , Postuma RB ((2021) ) Prodromal Parkinson disease subtypes –key to understanding heterogeneity. Nat Rev Neurol 17: , 349–361. |
[2] | Postuma RB , Iranzo A , Hu M , Högl B , Boeve BF , Manni R , Oertel WH , Arnulf I , Ferini-Strambi L , Puligheddu M , Antelmi E , Cochen De Cock V , Arnaldi D , Mollenhauer B , Videnovic A , Sonka K , Jung KY , Kunz D , Dauvilliers Y , Provini F , Lewis SJ , Buskova J , Pavlova M , Heidbreder A , Montplaisir JY , Santamaria J , Barber TR , Stefani A , St Louis EK , Terzaghi M , Janzen A , Leu-Semenescu S , Plazzi G , Nobili F , Sixel-Doering F , Dusek P , Bes F , Cortelli P , Ehgoetz Martens K , Gagnon JF , Gaig C , Zucconi M , Trenkwalder C , Gan-Or Z , Lo C , Rolinski M , Mahlknecht P , Holzknecht E , Boeve AR , Teigen LN , Toscano G , Mayer G , Morbelli S , Dawson B , Pelletier A ((2019) ) Risk and predictors of dementia and parkinsonism in idiopathic REM sleep behaviour disorder: a multicentre study. Brain 142: , 744–759. |
[3] | Roguski A , Rayment D , Whone AL , Jones MW , Rolinski M ((2020) ) A neurologist’s guide to REM sleep behavior disorder. Front Neurol 11: , 610. |
[4] | Neikrug AB , Avanzino JA , Liu L , Maglione JE , Natarajan L , Corey-Bloom J , Palmer BW , Loredo JS , Ancoli-Israel S ((2014) ) Parkinson’s disease and REM sleep behavior disorder result in increased non-motor symptoms. Sleep Med 15: , 959–966. |
[5] | Postuma RB , Bertrand JA , Montplaisir J , Desjardins C , Vendette M , Rios Romenets S , Panisset M , Gagnon JF ((2012) ) Rapid eye movementsleep behavior disorder and risk of dementia in Parkinson’s disease:a prospective study. Mov Disord 27: , 720–726. |
[6] | Barber TR , Muhammed K , Drew D , Lawton M , Crabbe M , Rolinski M , Quinnell T , Zaiwalla Z , Ben-Shlomo Y , Husain M , Hu MTM ((2018) ) Apathy in rapid eye movement sleep behaviour disorder is common and under-recognized. Eur J Neurol 25: , 469–e32. |
[7] | Assogna F , Liguori C , Cravello L , Macchiusi L , Belli C , Placidi F , Pierantozzi M , Stefani A , Mercuri B , Izzi F , Caltagirone C , Mercuri NB , Pontieri FE , Spalletta G , Pellicano C ((2021) ) Cognitive and neuropsychiatric profiles in idiopathic rapid eye movement sleep behavior disorder and Parkinson’s disease. J Pers Med 11: , 51. |
[8] | Mao J , Huang X , Yu J , Chen L , Huang Y , Tang B , Guo J ((2020) ) Association between REM sleep behavior disorder and cognitive dysfunctions in Parkinson’s disease: a systematic review and meta-analysis of observational studies. Front Neurol 11: , 577874. |
[9] | Pu JL , Jin CY , Wang ZX , Fang Y , Li YL , Xue NJ , Zheng R , Lin ZH , Yan YQ , Si XL , Chen Y , Liu Y , Song Z , Yan YP , Tian J , Yin XZ , Zhang BR ((2022) ) Apolipoprotein E genotype contributes to motor progression in Parkinson’s disease. Mov Disord 37: , 196–200. |
[10] | Mengel D , Dams J , Ziemek J , Becker J , Balzer-Geldsetzer M , Hilker R , Baudrexel S , Kalbe E , Schmidt N , Witt K , Liepelt-Scarfone I , Gräber S , Petrelli A , Neuser P , Schulte C , Linse K , Storch A , Wittchen HU , Riedel O , Mollenhauer B , Ebentheuer J , Trenkwalder C , Klockgether T , Spottke A , Wüllner U , Schulz JB , Reetz K , Heber IA , Ramirez A , Dodel R ((2016) ) Apolipoprotein E ɛ4 does not affect cognitive performance in patients with Parkinson’s disease. Parkinsonism Relat Disord 29: , 112–116. |
[11] | Federoff M , Jimenez-Rolando B , Nalls MA , Singleton AB ((2012) ) A large study reveals no association between APOE and Parkinson’s disease. Neurobiol Dis 46: , 389–392. |
[12] | Sunwoo JS , Byun JI , Jun JS , Kim TJ , Shin JW , Kim HJ , Jung KY ((2021) ) Apolipoprotein E ɛ4 is not associated with cognitive impairment in patients with idiopathic REM sleep behavior disorder. Parkinsonism Relat Disord 92: , 13–14. |
[13] | Gan-Or Z , Montplaisir JY , Ross JP , Poirier J , Warby SC , Arnulf I , Strong S , Dauvilliers Y , Leblond CS , Hu MTM , Högl B , Stefani A , Monaca CC , De Cock VC , Boivin M , Ferini-Strambi L , Plazzi G , Antelmi E , Young P , Heidbreder A , Barber TR , Evetts SG , Rolinski M , Dion PA , Desautels A , Gagnon JF , Dupré N , Postuma RB , Rouleau GA ((2017) ) The dementia-associated APOE ɛ4 allele is not associated with rapid eye movement sleep behavior disorder. Neurobiol Aging 49: , 218.e13–218.e15. |
[14] | Borghammer P , Van Den Berge N ((2019) ) Brain-first versus gut-first Parkinson’s disease: a hypothesis. J Parkinsons Dis 9: , S281–S295. |
[15] | Horsager J , Andersen KB , Knudsen K , Skjærbæk C , Fedorova TD , Okkels N , Schaeffer E , Bonkat SK , Geday J , Otto M , Sommerauer M , Danielsen EH , Bech E , Kraft J , Munk OL , Hansen SD , Pavese N , Göder R , Brooks DJ , Berg D , Borghammer P ((2020) ) Brain-first versus body-first Parkinson’s disease: a multimodal imaging case-control study. Brain 143: , 3077–3088. |
[16] | Horsager J , Knudsen K , Sommerauer M. Clinical and imaging evidence of brain-first and body-first Parkinson’s disease ((2022) ) Clinical and imaging evidence of brain-first and body-first Parkinson’s disease. Neurobiol Dis 164: , 105626. |
[17] | Hipp G , Vaillant M , Diederich NJ , Roomp K , Satagopam VP , Banda P , Sandt E , Mommaerts K , Schmitz SK , Longhino L , Schweicher A , Hanff AM , Nicolai B , Kolber P , Reiter D , Pavelka L , Binck S , Pauly C , Geffers L , Betsou F , Gantenbein M , Klucken J , Gasser T , Hu MT , Balling R , Krüger R ((2018) ) The Luxembourg Parkinson’s Study: a comprehensive approach for stratification and early diagnosis. Front Aging Neurosci 10: , 326. |
[18] | Litvan I , Bhatia KP , Burn DJ , Goetz CG , Lang AE , McKeith I , Quinn N , Sethi KD , Shults C , Wenning GK , Movement Disorders Society Scientific Issues Committee ((2003) ) Movement Disorders Society Scientific Issues Committee report: SIC Task Force appraisal of clinical diagnostic criteria for Parkinsonian disorders. Mov Disord 18: , 467–486. |
[19] | Antelmi E , Lippolis M , Biscarini F , Tinazzi M , Plazzi G ((2021) ) REM sleep behavior disorder: Mimics and variants. Sleep Med Rev 60: , 101515. |
[20] | Ashraf-Ganjouei A , Moradi K , Aarabi M , Abdolalizadeh A , Kazemi SZ , Kasaeian A , Vahabi Z ((2021) ) The association between REM sleep behavior disorder and autonomic dysfunction in Parkinson’s disease. J Parkinsons Dis 11: , 747–755. |
[21] | Barasa A , Wang J , Dewey RB Jr ((2021) ) Probable REM sleep behavior disorder is a risk factor for symptom progression in Parkinson disease. Front Neurol 12: , 651157. |
[22] | Long K , Wan C , Xiang Y , Liu J , Xu Q , Sun Q , Wang Z , Tian Y , Fang L , Yang Y , Yan X , Tang B , Guo J ((2020) ) Study on the clinical features of Parkinson’s disease with probable rapid eye movement sleep behavior disorder. Front Neurol 11: , 979. |
[23] | Liu Y , Zhu XY , Zhang XJ , Kuo SH , Ondo WG , Wu YC ((2017) ) Clinical features of Parkinson’s disease with and without rapid eye movement sleep behavior disorder. Trans Neurodegener 6: , 35. |
[24] | Liu Y , Lawton MA , Lo C , Bowring F , Klein JC , Querejeta-Coma A , Scotton S , Welch J , Razzaque J , Barber T , Ben-Shlomo Y , Hu MT ((2021) ) Longitudinal changes in Parkinson’s disease symptoms with and without rapid eye movement sleep behavior disorder: The Oxford Discovery Cohort Study. Mov Disord 36: , 2821–2832. |
[25] | Pavelka L , Rauschenberger A , Landoulsi Z , Pachchek S , May P , Glaab E , Krüger R ; NCER-PD Consortium ((2022) ) Age at onset as stratifier in idiopathic Parkinson’s disease –effect of ageing and polygenic risk score on clinical phenotypes. NPJ Parkinsons Dis 8: , 102. |
[26] | Blauwendraat C , Faghri F , Pihlstrom L , Geiger JT , Elbaz A , Lesage S , Corvol JC , May P , Nicolas A , Abramzon Y , Murphy NA , Gibbs JR , Ryten M , Ferrari R , Bras J , Guerreiro R , Williams J , Sims R , Lubbe S , Hernandez DG , Mok KY , Robak L , Campbell RH , Rogaeva E , Traynor BJ , Chia R , Chung SJ , ; International Parkinson’s Disease Genomics Consortium (IPDGC), COURAGE-PD Consortium, Hardy JA , Brice A , Wood NW , Houlden H , Shulman JM , Morris HR , Gasser T , Krüger R , Heutink P , Sharma M , Simón-Sánchez J , Nalls MA , Singleton AB , Scholz SW ((2017) ) NeuroChip, an updated version of the NeuroX genotyping platform to rapidly screen for variants associated with neurological diseases. Neurobiol Aging 57: , 247.e9–247.e13. |
[27] | Tunold JA , Geut H , Rozemuller JMA , Henriksen SP , Toft M , van de Berg WDJ , Pihlstrøm L ((2021) ) APOE and MAPT are associated with dementia in neuropathologically confirmed Parkinson’s disease. Front Neurol 12: , 631145. |
[28] | Davis AA , Inman CE , Wargel ZM , Dube U , Freeberg BM , Galluppi A , Haines JN , Dhavale DD , Miller R , Choudhury FA , Sullivan PM , Cruchaga C , Perlmutter JS , Ulrich JD , Benitez BA , Kotzbauer PT , Holtzman DM ((2020) ) APOE genotype regulates pathology and disease progression in synucleinopathy. Sci Trans Med 12: , eaay3069. |
[29] | Gan-Or Z , Liong C , Alcalay RN ((2018) ) GBA-associated Parkinson’s disease and other synucleinopathies. Curr Neurol Neurosci Rep 18: , 44. |
[30] | Sixel-Döring F , Trautmann E , Mollenhauer B , Trenkwalder C ((2011) ) Associated factors for REM sleep behavior disorder in Parkinson disease. Neurology 77: , 1048–1054. |
[31] | Bjørnarå KA , Dietrichs E , Toft M ((2013) ) REM sleep behaviordisorder in Parkinson’s disease–is there a gender difference? Parkinsonism Relat Dis 19: , 120–122. |
[32] | Baumann-Vogel H , Hor H , Poryazova R , Valko P , Werth E , Baumann CR ((2020) ) REM sleep behavior in Parkinson disease: Frequent, particularly with higher age. PloS One 15: , e0243454. |
[33] | Wong JC , Li J , Pavlova M , Chen S , Wu A , Wu S , Gao X ((2016) ) Risk factors for probable REM sleep behavior disorder: A community-based study. Neurology 86: , 1306–1312. |
[34] | Haba-Rubio J , Frauscher B , Marques-Vidal P , Toriel J , Tobback N , Andries D , Preisig M , Vollenweider P , Postuma R , Heinzer R ((2018) ) Prevalence and determinants of rapid eye movement sleep behavior disorder in the general population. Sleep 41: , zsx197. |
[35] | Kang SH , Yoon IY , Lee SD , Han JW , Kim TH , Kim KW ((2013) ) REM sleep behavior disorder in the Korean elderly population: prevalence and clinical characteristics. Sleep 36: , 1147–1152. |
[36] | Postuma RB , Montplaisir JY , Pelletier A , Dauvilliers Y , Oertel W , Iranzo A , Ferini-Strambi L , Arnulf I , Hogl B , Manni R , Miyamoto T , Mayer G , Stiasny-Kolster K , Puligheddu M , Ju Y , Jennum P , Sonka K , Santamaria J , Fantini ML , Zucconi M , Leu-Semenescu S , Frauscher B , Terzaghi M , Miyamoto M , Unger MM , Cochen De Cock V , Wolfson C ((2012) ) Environmental risk factors for REM sleep behavior disorder: a multicenter case-control study. Neurology 79: , 428–434. |
[37] | Gan-Or Z , Rao T , Leveille E , Degroot C , Chouinard S , Cicchetti F , Dagher A , Das S , Desautels A , Drouin-Ouellet J , Durcan T , Gagnon JF , Genge A , Karamchandani J , Lafontaine AL , Sun SLW , Langlois M , Levesque M , Melmed C , Panisset M , Parent M , Poline JB , Postuma RB , Pourcher E , Rouleau GA , Sharp M , Monchi O , Dupré N , Fon EA ((2020) ) The Quebec Parkinson Network: a researcher-patient matching platform and multimodal biorepository. J Parkinsons Dis 10: , 301–313. |
[38] | Marek K , Chowdhury S , Siderowf A , Lasch S , Coffey CS , Caspell-Garcia C , Simuni T , Jennings D , Tanner CM , Trojanowski JQ , Shaw LM , Seibyl J , Schuff N , Singleton A , Kieburtz K , Toga AW , Mollenhauer B , Galasko D , Chahine LM , Weintraub D , Foroud T , Tosun-Turgut D , Poston K , Arnedo V , Frasier M , Sherer T Parkinson’s Progression Markers Initiative ((2018) ) The Parkinson’s progression markers initiative (PPMI) –establishing a PD biomarker cohort. Ann Clin Transl Neurol 5: , 1460–1477. |
[39] | Mollenhauer B , Trautmann E , Sixel-Döring F , Wicke T , Ebentheuer J , Schaumburg M , Lang E , Focke NK , Kumar KR , Lohmann K , Klein C , Schlossmacher MG , Kohnen R , Friede T , Trenkwalder C ; DeNoPa Study Group ((2013) ) Nonmotor and diagnostic findings in subjects with de novo Parkinson disease of the DeNoPa cohort. Neurology 81: , 1226–1234. |
[40] | Onofrj M , Thomas A , D’Andreamatteo G , Iacono D , Luciano AL , Di Rollo A , Di Mascio R , Ballone E , Di Iorio A ((2002) ) Incidence of RBD and hallucination in patients affected by Parkinson’s disease: 8-year follow-up. Neurol Sci 23: , S91–S94. |
[41] | Gjerstad MD , Boeve B , Wentzel-Larsen T , Aarsland D , Larsen JP ((2008) ) Occurrence and clinical correlates of REM sleep behaviour disorder in patients with Parkinson’s disease over time. J Neurol Neurosurg Psychiatry 79: , 387–391. |
[42] | Lenka A , Hegde S , Jhunjhunwala KR , Pal PK ((2016) ) Interactions of visual hallucinations, rapid eye movement sleep behavior disorder and cognitive impairment in Parkinson’s disease: A review. Parkinsonism Relat Disord 22: , 1–8. |