Spinal Cord Stimulation for Gait Disorders in Parkinson’s Disease
Abstract
Background:
Spinal cord stimulation (SCS) is a therapeutic procedure widely used in the management of refractory chronic pain. Evidence from case reports and small descriptive studies has emerged suggesting a role for SCS in patients with gait dysfunction, such as freezing of gait (FoG) and postural imbalance. These are severely debilitating symptoms of advanced Parkinson’s disease (PD).
Objective:
To establish the current evidence base for the potential application of SCS on gait and balance dysfunction in PD patients.
Methods:
Three online databases were screened for relevant manuscripts. Two separate searches and four different search strategies were applied to yield relevant results. The main parameters of interest were postural and gait symptoms; secondary outcomes were Quality of Life (QoL) and adverse effects.
Results:
Nineteen studies fulfilled the inclusion criteria. Motor improvements using section III of the Unified Parkinson’s Disease Rating Score (UPDRS-III) were available in 13 studies. Measurements to assess FoG reported the following improvements: FoG questionnaires (in 1/19 studies); generalized freezing parameters (2); and walkway/wireless accelerometer measurements (2). Parameters of postural imbalance and falling improved as follows: BBS (1); posture sagittal vertical axis (1); and generalized data on postural instability (8). Two studies reported on adverse effects. QoL was shown to improve as follows: EQ-5D (2); ADL (1); SF-36 (1); BDI-II (1); PDQ-8 (1); HDRS (1); and VAS (5).
Conclusion:
SCS may have a therapeutic potential in advanced PD patients suffering from postural and gait-related symptoms. The existing evidence suggests that SCS positively affects patients’ QoL with an acceptable safety profile in this patient population.
INTRODUCTION
Parkinson’s disease (PD) is the second commonest neurodegenerative disorder following Alzheimer disease. It is a very heterogenous disorder regarding onset, disease duration, individual symptomatology, and disease progression. Moreover, it creates a burden on carers, healthcare systems, and society [1].
The first line of treatment generally involves dopamine replacement therapy. Most patients will respond well to medical therapy, but as the disease progresses, some patients will develop intolerable side effects to increasing medication doses or experience motor fluctuations due to a reduction of efficacy of dopamine replacement. Initially, most motor symptoms can be addressed with deep brain stimulation (DBS) (particularly in the subthalamic nucleus area) when there is still some dopamine sensitivity observed [2]. Having said that, not all patients are suitable for DBS due to cognitive impairments or brain atrophy; furthermore, axial motor symptoms tend to be refractory to DBS or even potentially worsened by it [3, 4].
Postural instability and gait disorder (PIGD) are two of such complications associated with advanced PD. PIGD poses a major burden on patients’ quality of life (QoL) and their psychological and emotional well-being [5]. In the earlier stages, it can be dopamine-sensitive with variable response to standard therapy. However, as the disease progresses, PIGD becomes refractory. Increased severity of instability, paired with frequent freezing episodes often lead to increased frequency of falls, this is associated with increased morbidity and mortality [4, 6]. Several promising treatment procedures have emerged with preliminary data showing some therapeutic potential (e.g., vagus nerve stimulation), but there is currently no clinically recognized and proven non-invasive procedure to safely address these axial symptoms once refractory to standard therapy [6–8]. Robust and controlled studies, which make use of objectively measurable, instrumental investigations, are urgently needed to establish a clinically proven link between such procedures and any potential therapeutic benefits observed in gait disturbance in PD patients. Wearable devices and the use of gait labs are two motion-tracking methods, which could be applied to augment gait analysis and provide a platform for collection of measurable, clinical evidence [9, 10].
Since its FDA approval for neuropathic pain, spinal cord stimulation (SCS) procedures have become widely used in clinical practice when other treatment options fail, accounting for 70% of the larger neuromodulation field [11, 12]. The potential value of SCS procedures in PD emerged due to serendipity after being used to treat pain in in other chronic pathological manifestations, such as leg ischemia, diabetic neuropathy, and refractory angina when some patients reported improvements in other Parkinsonian symptoms (e.g., gait disorders and postural imbalance) [13]. SCS has also been shown to induce therapeutic effects in other conditions affecting postural stability, such as primary orthostatic tremor (PTO). A proof-of-concept study in 16 subjects with PTO by Lamy et al. indicated that a single session of spinal DC stimulation significantly improved time in standing position in respect to sham both after 30 and 60 min [14].
Several preclinical studies investigated these beneficial effects using a wide variety of stimulation parameters in rat and primate PD models [15–19]. The promising results of these trials led to further investigations of SCS potential in a number of PD case series, case reports and a single systematic review released in the past decade [20–38]; however, the role of placebo vs. paresthesia-free stimulation is often neglected during the assessment of clinical efficacy of this procedure and needs to be carefully reported upon and evaluated during the design and discussion of any future research into this topic. Novel devices are able to provide stimulation parameters that do not result in paresthesia (such as high frequency stimulation) [39–42], but these have a drawback of high energy use requiring daily battery recharging. Another option is burst stimulation. These advances will undoubtedly allow for more blinded comparison of ON vs. OFF stimulation [43]. The objective of this study was to systematically review the existing literature that reports on the effects of SCS on gait (i.e., freezing of gait (FoG) and gait disorders) and postural imbalance (i.e., balance, falls) in PD patients. Other clinical parameters of interest were postoperative adverse effects and QoL measures.
METHODS
This systematic review was written in accordance with PRISMA guidelines for systematic reviews and meta-analyses. The PRISMA checklist is available as Supplementary Figure 1 [44].
Search keyterms
The following keyterms were used in the database search to identify a complete set of relevant literature: Parkinson disease (alt. Parkinson, Parkinsons or Parkinson’s), gait, proprioception, kinesthesis, postural balance (alt. kinesthes* or balanc* or postur*), spinal cord stimulation (alt. spine or spinal). In the case of secondary search, the following keyterms were replaced by the keyterm pain (alt. chronic pain): proprioception, kinesthesis, postural balance (alt. kinesthes* or balance* or postur*).
Search strategy
Three databases were searched: Medline (OvidSP version), Embase (OvidSP version), and Web of Sciences. Several different search strategies were applied. For specific search strategies used in individual databases, see Supplementary Figures 4 and 5.
Selection criteria
Subjects
The inclusion criteria were as follows: All studies reporting on changes in UPDRS-III, gait and/ or QoL measures following SCS in PD patients. Studies of SCS in PD patients that did not report on any of these measures (i.e., with a pure focus on pain) were excluded.
Study design
All studies were screened for eligibility. Once the pre-selected criteria described above were met, the full-text articles were retrieved and screened for relevant information to confirm the manuscript’s eligibility.
Language
Only studies published in the English language were included in this systematic review.
Outcome measures
The primary focus of this review was on the changes in gait-related pathology in PD and freezing of gait. We also assessed any changes in postural disbalances, namely balance and falls occurrence.
These postural instability and gait changes were assessed using any of the following measures/scales: freezing of gait (FoG) questionnaires, Movement Disorder Society-Sponsored Unified Parkinson’s Disease Rating Scale (MDS-UPDRS) score (primarily motor and ADL sub-scores), stand-walk-sit (SWS) test, Parkinson’s Disease Questionnaire –39 mobility items (PDQ-39), Timed Up and Go tests (TUG), Timed 20/10/7-Meter Walk test (T 10-M test) and gait analysis using GAITRite Electronic Walkway/Protokinetic Zeno Walkway/wireless accelerometer. Secondary outcomes were observed side effects and quality of life assessment using Activities of Daily Living score (ADL), EuroQoL- 5 Dimension (EQ-5D), 8-item Parkinson’s Disease questionnaire (PDQ-8), Short Form Questionnaire (SF-36), Beck Depression Inventory II (BDI- II), Hamilton Depression Rating Scale (HDRS), PDQ-39, General Functioning Questionnaire (GFQ-58) and Visual Analog Scale score (VAS), both pre and postoperatively.
RESULTS
Nineteen studies that fit the selection criteria were included in our systematic review after the eligibility screening. We identified that 8 of the included studies were case studies, and 11 were case series. No larger studies or controlled trials were identified.
As all studies in this systematic review are observational studies (i.e., case reports, case series) and patients are not allocated to study arms, a standard PICO framework was not applied [45].
The study selection stage is visualized using the PRISMA 2009 Flow Diagram (Fig. 1) [44]. Search periods and results yielded by individual databases can be found in Supplementary Figures 2 and 3. Study characteristics, stimulation parameters, and outcomes are detailed in the tables below (Tables 1, 2A, and 2B).
Table 1
Table showing demographic and clinical patient information in individual studies
Series | Study ID, Year | Number of Patients (N) | Sex (F/M) | Received DBS Prior To SCS (Yes/No) | Mean Age (y) | Mean Disease Duration (y) | Follow-up | Indication for SCS |
1 | Mazzone et al., 2019 | 18 | 2/16 | NR | 68.35 | 14.1 | 1 year | 1st group: PAIN 2nd group: PIGD |
2 | Hubsch et al., 2019 | 5 | 0/5 | Yes: 1 patient; No: 4 patients | 68.8 | 14.8 | 2 months | PIGD |
3 | Samotus et al., 2018 | 5 | 0/5 | NR | 71 | 14 | 6 months | PIGD |
4 | De Lima Pardini et al., 2018/De Souza et al., 2017 | 4 | 1/3 | Yes | 64.25 | 21.25 | 1 month, 3 months, 6 months | PIGD |
5 | Rohani et al., 2017 | 2 | 1/1 | NR | 67.5 | NR | 5 months; 24 months | PIGD |
6 | Cécile et al., 2017 | 5 | NR | NR | NR | NR | 3 days, 15 days, 60 days | PIGD |
7 | Thevathasan et al., 2010 | 2 | NR | NR | 76 | NR | 10 days | PIGD |
8 | Lai et al., 2020 | 1 | 0/1 | Yes | 73 | 13 | 4 days, 2 months | 1st: PAIN + PIGD 2nd: Electrode dislocation |
9 | Kobayashi et al., 2018 | 1 | 0/1 | NR | 74 | 3 | 2 weeks | PAIN + PIGD |
10 | Ichikawa et al., 2013 | 6 | NR | NR | NR | 12.2 | 3 to 5 weeks after SCS | PAIN |
11 | Akiyama et al., 2017 | 1 | 1/0 | Yes (5 years prior) | 65 | 12 | 11 days. 29 days | PAIN |
12 | Soltani and Lalkhen, 2013 | 1 | 1/0 | NR | 68 | NR | NR | PAIN |
13 | Weise et al., 2010 | 1 | 1/0 | Yes | 72 | 17 | NR | PAIN |
14 | Mitsuyama et al., 2013 | 2 | NR | NR | NR | 8.5 | NR | PAIN |
15 | Nishioka and Nakajima, 2015 | 3 | 1/2 | NR | 74.3 | 9.3 | 2 weeks, 3 months, 1 year | PAIN |
16 | Agari and Date, 2012 | 15 | 10/5 | Yes: 7 patients; No: 8 patients | 71.1 | 17.2 | 3 months; 12 months | PAIN |
17 | Hassan et al., 2013 | 1 | 1/0 | NR | 43 | 8 | Every 3 months for 2 years | PAIN |
18 | Landi et al., 2013 | 1 | 1/0 | Yes | 65 | NR | 16 months | PAIN |
19 | Fénelon et al., 2011 | 1 | 0/1 | NR | 74 | 5 | Four sessions 2– 5 weeks apart | PAIN |
Top table section, white = Indication for SCS is PIGD; Bottom section, grey = indication for SCS is PAIN; Series 1,8 and 9 both indications. N, number; NR, not reported; F, female; M, male; DBS, deep brain stimulation; SCS, spinal cord stimulation; PIGD, postural instability and gait disturbance. Studies with follow-up <3 months or NR are highlighted.
Table 2A
Table listing the stimulation parameters and outcome measures in individual studies (+ indicates outcome improvement following SCS, – indicates outcome worsening following SCS, 0 indicates no effect on outcome following SCS)
Series | Study ID, Year | Type of Electrode | SCS Spinal Level | Amplitude Range (V/mA) | Pulse Width (μs) | Frequency (Hz/bps) | MDS-UPDRS Outcome | Other Gait and Postural Measures | QoL Measures |
1 | Mazzone et al., 2019 | Quadripolar/Octopolar | C1-C3 | 3 V (G1);0.5 mA (G2) | 93.3 (G1); 1000 (G2) | 150 Hz (G1); 479.2 Hz (G2) | + burst high cervical SCS better than tonic HCSCS | + gait parameters | + VAS score, SS behavioral task |
2 | Hubsch et al., 2019 | Octopolar | T10-T11 | NR; Chosen individually at the threshold of paresthesia in lower limbs | 300 | 100 Hz | + SCS alone by 23.22%; SCS+L-DOPA by 36.8% | + SWS SCS alone (steps by 12.4%; duration 23.6%), SWS SCS+L-DOPA (steps 20%; duration 29.8%), axial symptoms SCS (29.8%), axial symptoms SCS + L-DOPA (42.5%), PDQ-39 mobility sub-score (29.6 to 22.6) 0 FoG questionnaire | + no side effects, PDQ-39 (72.2 to 57) |
3 | Samotus et al., 2018 | Octopolar | T8-T10 | NR | 300–5400 | 30–130 Hz | + UPDRS Part III by 33.4% | + ABC (71.4%), mean number of FoG episodes (14.8 ep. to 0.2 ep. at 6m) 0 duration of FoG episodes, FoG questionnaire score | + no side effect/ adverse events 0 PDQ-8 |
4 | De Lima Pardini et al., 2018/ De Souza et al., 2017 | Paddle electrodes with 3 columns of contacts | T2-T4 | 4.6±1.9 V (standing position); 2.0±0.5 V (supine position) | 90 | 60/300 Hz | + by 48.7% (3m); 38.3% (6m) | + TUG (by 63.2%), 20-m walk test (no. of steps: 65.7%; duration: 58%), Berg balance scale (8%), FoG questionnaire (17.8%), APA duration at 300 Hz, FoG duration using wireless accelerometer | + PDQ-39 (44.7%) |
5 | Rohani et al., 2017 | Quadripolar | T10-T11 | 2.5–7 V | 60–90 | 60–70 | NR | + FoG, gait parameters (GAITRite Electronic Walkway) | NR |
6 | Cécile et al., 2017 | Octopolar | NR | NR | 300 | 100 Hz | + UPDRS Part III | + SWS | NR |
7 | Nishioka and Nakajima, 2015 | 5-6-5 electrodes | T8–L1 | NR; Optimal amplitude range determined individually at different time points | NR; Optimal pulse width range determined individually at different time points | NR; Optimal stimulation frequency range determined individually at different time points | + UPDRS Part III by 33.6% | NR | + VAS score, MMSE (patient 1 + 2), HDS-R (patient 1 + 2) 0 WPI, FAB |
8 | Ichikawa et al., 2013 | Octopolar | T6-T8 (five patients); C4 (one patient) | NR | 100–200 | 60–90 bps | + UPDRS Part III by 32.3% (items for lower limb function + gait: by 46.6%) | NR | NR |
9 | Mitsuyama et al., 2013 | NR | NR; Location where test stimulation indicated paresthesia over painful location | NR | NR | NR | NR | + walking posture 0 FoG | NR |
Table 2B
Table listing the stimulation parameters and outcome measures in individual studies (+ indicates outcome improvement following SCS, – indicates outcome worsening following SCS, 0 indicates no effect on outcome following SCS)
Series | Study ID, Year | Type of Electrode | SCS Spinal Level | Amplitude Range (V/mA) | Pulse Width (μs) | Frequency (Hz/bps) | MDS-UPDRS Outcome | Other Gait and Postural Measures | QoL Measures |
10 | Agari and Date, 2012 | Quadripolar/Octopolar | T7-T12 | 0–4 V | 210–330 | 5–20 Hz | + by 19.6% (3m), 9.4% (12m) | + Gait (40% at 3m; 12% at 12m), postural stability (34.8%; 21.7%), TUG (27.8%; 15.7%), T 10-M walk test (13.6%; 9.5%), walking (24%; 16%) 0 freezing, falling, rigidity, leg agility, arising from chair | + ADL score (21.2%; 15%), VAS score (8.9 preop; 2 postop) 0 hygiene |
11 | Thevathasan et al., 2010 | Quadripolar/Octopolar | High cervical level | Sub + Supra-threshold stimulation: 2-3 V(P1); 3-4 V(P2) | Sub + Supra-threshold stimulation: 240(P1); 200(P2) | Sub + Supra-threshold stimulation: 130Hz(P1); 300 Hz(P2) | 0 | 0 T 10-M test, timed hand arm movement, timed foot tapping | 0 VAS score |
12 | Lai et al., 2020 | 1st SCS: percutaneous octopolar; 2nd SCS: paddle lead (5–6–5) | 1st SCS: T8-T10; 2nd SCS: T8-T9 | 1st SCS: 1.5–2.5V;2nd SCS: 3.6–4 V | 1st SCS: 180–210; 2nd SCS: 270–390 | 1st SCS: 30 Hz;2nd SCS: 60 Hz | + from 45 (2m post 1st SCS) to 42 (2m post 2nd SCS) | + TUG (29s to 22s) | + VAS score (7 to 3), KPSS (12 to 4), PDQ-8 (by 40%), SF-36 (4 items), BDI-II (18 to 6) 0 GFQ-58, EQ-5D |
13 | Kobayashi et al., 2018 | Octopolar | T6-T8 | 0.6–0.8 mA | 1 ms | 40-Hz burst with 5 spikes at the rate of 500 Hz | + 20 (pre Burst stimulation); 4 (post Burst stimulation) | + 20m walk (32s 56 steps pre Burst; 25s 45 steps post Burst), posture sagittal vertical axis (127.68mm to 94.55mm) | + SF-MPQ-2 total pain (47 to 18), SF-MPQ-2 affective pain (5 to 0), SF-36 (31.1 to 43.6) |
14 | Akiyama et al., 2017 | Octopolar | T8-L3 (right side), T8-L4 (left side) | 2.5 V (program 1); 3.5 V (program 2) | 450 (program 1); 250 (program 2) | 7 Hz (both program 1 + 2) | + 48 at D0 (I, 4; II, 25; III, 15; IV, 4), 33 at D11 (I, 5; II, 10; III, 15; IV, 3), 34 at D29 (I, 3; II, 12; III, 15; IV, 4) | + TUG (D0 : 15s, D11 : 8s, D29 : 7s) | + VAS score (10 to 2), EuroQoL (0.101 to 0.208), Numerical Rating Scale (9 to 7), Leeds Assessment of Neuropathic Symptoms and Signs Pain Scale (11 to 5), Hospital Anxiety and Depression Scale (4 to 9), Pain Disability Assessment Scale (39 to 36) |
15 | Hassan et al., 2013 | Octopolar | C2 | 0.3–1.1 mA | 500 | 40 Hz | + 28 (early postop), 22 (1y), 16 (2y) | + T 10-M test (17s baseline, 11s at 2y), tremor, shuffling gait, rigidity, posture | NR |
16 | Landi et al., 2013 | Quadripolar | T9–T10 | 1.8–2.5 V | 250 | 30 Hz | 0 | + 20m walk (duration by 20%), coordination, stability, direction change | + bladder control, HDRS (21 to 9), EuroQoL (60%) |
17 | Soltani and Lalkhen, 2013 | Octopolar | T9-T11 | 1.5 V | 300 | 60 Hz | NR | NR | + Parkinsonian symptoms (resting tremor), leg pain |
18 | Fénelon et al., 2011 | Quadripolar | T9-T10 | 3.5 V | 410 | 100–130 Hz | + UPDRS part III by 47.6%, axial sub-score (30.5%), tremor (60%), bradykinesia (40.1%), rigidity (65.2%), upper limbs (44.1%), lower limbs (64.1%) | + 7m walk (29.3s to 23s) | + VAS score (6.9 to 1.9) |
19 | Weise et al., 2010 | NR | Cervicothoracic level | NR | NR | NR | 0 | NR | NR |
SCS, spinal cord stimulation; V, volt; mA, milliampere; μs, microseconds; Hz, Hertz; bps, bits per second; MDS-UPDRS, Movement Disorder Society-Sponsored Unified Parkinson’s Disease Rating Scale; TUG, Timed Up-and-Go test; T 10-M walk, Timed 10-Meter walk; ADL, Activities of Daily Living; VAS, Visual Analog Scale; SWS, Stand-Walk-Sit; PDQ-39, Parkinson’s Disease Questionnaire - 39; FoG, freezing of gait; PD, Parkinson’s disease; APA, Anticipatory Postural Adjustment; L-DOPA, levodopa; SF-MPQ-2, Short-form McGill Pain Questionnaire 2; SF-36, 36-Item Short Form Survey; KPSS, King’s Parkinson’s Disease Pain Scale; PDQ-8, 8-item Parkinson’s Disease questionnaire; BDI-II, Beck’s Depression Inventory; GFQ, General Functioning Questionnaire; EQ-5D, EuroQoL-5 Dimension; HDRS, Hamilton’s Depression Rating Scale; SS, stop-signal; MMSE, Mini-Mental State Exam; HDS-R, Hierarchic Dementia Scale - Revised; WPI, Widespread Pain Index; FAB, Frontal Assessment Battery; ABC, Activities-specific Balance Confidence.
Sample and outcome evaluation
All relevant literature in this review consists solely of case series (11) and case reports (8), making the risk of bias assessment challenging. There is no gold standard for evaluating the quality of case series/reports as it is the case for randomized controlled trials (such as the Cochrane Collaboration tool) [46]. According to a systematic review by Zeng et al., where they investigated all existing methodological quality assessment tools for every type of study design, the only commercially available tool to qualitatively assess case reports was the 8-item Joanna Briggs Institute (JBI) 2017 Critical Appraisal Checklist for Case Reports. We used the same source for quality check of our case series (10-item Joanna Briggs Institute 2017 Critical Appraisal Checklist for Case Series) [47–49]. Checklists and scores for individual studies can be found in Supplementary Figures 6 and 7.
Using the primary measurement scale for motor deficits shared by most of the studies (16 out of 19 studies), the UPDRS-III, 13 studies reported a degree of improvement in motor symptoms following the spinal cord stimulation procedure. 11 studies reported quantitative changes in motor symptoms; on average, the motor symptoms improved by 36.3±19.0% on the UPDRS-III scale. Following the removal of the
Fig. 1
PRISMA Flow diagram of study selection. Three databases (Medline, Embase, Web of Sciences) were screened for eligible studies with a focus on SCS for gait disorders/freezing and balance/falls in PD patients.
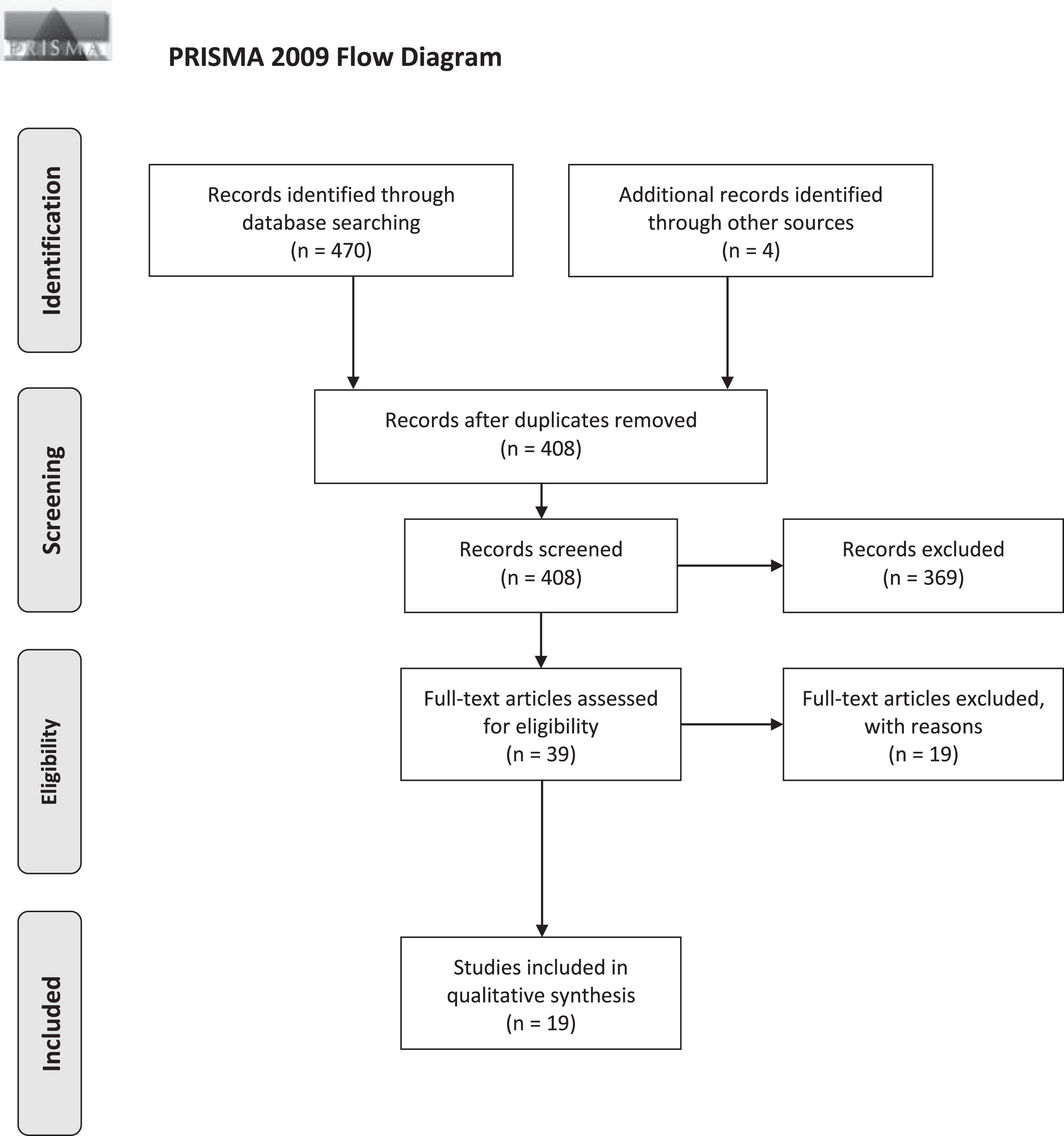
Further research should prioritize appropriately quantifying their observations, reporting significance levels where available and specifying which individual UPDRS-III items were the overall improvements in score driven by.
The effects of SCS on freezing of gait were more controversial when relying on patient reported FoG questionnaires (1 out of 19 studies reports improvement of 56.2%; 2 no effect) and generalized freezing parameters with no specific tool mentioned (2/19 improved; 2/19 no effect; IMP not quantified). Nevertheless, the assessment of FoG using methods that rely on more objectively measurable, quantitative data like a walkway and wireless accelerometer (2/19) showed FoG markedly improved post SCS.
Specific parameters of postural imbalance and falling were positively affected by SCS as follows: Berg Balance Scale (1/19, IMP 8.4%), Posture Sagittal Vertical Axis (1/19, IMP 25.9%) and generalized data on postural instability and falls with no specific tool mentioned (8/19; 1 no effect on falls; IMP not quantified).
A range of different Quality of Life and affective scales was used to clinically evaluate the QoL dimension: EQ-5D (1/19 no effect, 1/19 NR, 1/19 IMP 60%), ADL (1/19, IMP 21.2%), SF-36 (1/19, IMP 40.2%), BDI-II (1/19, IMP 66.7%), PDQ-8 (1/19, IMP 40%; 1 no effect), HDRS (1/19, IMP 57.1%), PDQ-39 (2/19, AVG IMP 32.9±16.7%), GFQ-58 (1/19 no effect) and VAS score (5/19, AVG IMP 68.7±11.2%; 1/19 IMP not quantified; 1/19 no effect).
There were no mortalities associated with the intervention reported in any of the studies. One patient reported feelings of akinesia, but this issue was quickly resolved by changing the dopamine medication dose. The most common adverse event post SCS was the lead migration, which was reported in a single study, resulting in the recurrence of pain.
DISCUSSION
Despite the encouraging nature of our results being indicative of marked improvements in gait freezing and motor parameters while maintaining an acceptable side effect profile and positively affecting patients’ life quality, there is a clear need for well-designed experimental studies with a higher number of patients to establish the efficacy and safety of SCS as an alarmingly needed therapy in PD patients with gait disorders.
Existing evidence and limitations
Perhaps one of the main issues with assessing the efficacy of SCS is the placebo effect [50, 51]. The study by Lai et al. does show some underlying changes happening on a physiological level as well and that the observable pain and PIGD improvements are unlikely a result of placebo effects in their entirety [31, 52].
Several potential sources of heterogeneity within the studies included in our review were also identified, primarily concerning the study design, measurement tools and data reporting. Case reports/series can be problematic when a clear relationship between an intervention and outcome needs to be established. The findings reported by these studies are promising, but they are not as easily applicable to the general population due to the small sample size characteristic of case reports and series, baseline demographic, patient cohort without clearly defined characteristics and the high risk of bias presence from various sources [53, 54].
Further concerns stem from the lack of controlled trial environment. Not all studies clearly report if some/all patients underwent DBS prior to SCS and whether DBS induced quantitative changes from baseline in gait freezing following the procedure. Patients presenting with FoG following DBS vs. patients with FoG without prior DBS could potentially categorize separately, so this variable should be accounted for when defining study population criteria.
The outcome measures used in the studies often vary. Several studies applied measures composed of several sub-domains focusing on different disease aspects (e.g., UPDRS, PDQ-39, PDQ-8); however, only the overall scores are reported without detailing the results from the most relevant items (i.e., domains concerning freezing/balance). Moreover, these scores were assessed at specific time points at the clinic and often do not reflect the standard patient environment.
These symptoms are environment and attention-dependent, and their measurement in gait labs highly artificial. Thus, researchers often must rely on highly symptom-specific yet self-reported data [55, 56]. De Souza et al. used the wireless accelerometer to measure the occurrence of these symptoms in a more objective manner, and various other ambulatory cueing technologies can accompany patient-reported testimonies to measure gait freezing more accurately in patient’s natural settings (e.g., ankle-mounted sensor array) [57].
A further consideration for PD patients prior to undergoing SCS is the potential occurrence of paresthesia. However, not all SCS lead to paresthesia induction (e.g., high-frequency stimulation), and our review suggests that this mode of stimulation should be investigated in more depth in PD pathology [32]. Several studies included in this review attempted to identify a universal set of stimulation parameters that could promise a significant improvement in most of the clinical outcomes of interest for all patients.
Nevertheless, the most suitable stimulation parameters always needed to be determined individually [20, 26]. Even though the studies in this review used different parameters for stimulation and failed to identify the best baseline set for everyone, their findings may provide a starting point where the most effective range could lie for most patients. The findings suggest that high burst stimulation seems to outperform when compared to tonic, low-frequency stimulation [23, 32], and burst stimulation is promising (as well as high frequency) when it comes to blinding patients and assessors to ON vs. OFF stimulation. Nonetheless, the “beneficial” frequency can range from 7–500 Hz.
In the studies reporting motor improvement, the amplitude and pulse width ranged as follows: 1.5–4.6 V/0.3–1.1 mA; 90–1000μs. The implantation levels were mainly at the thoracic spinal segments, but even patients with electrodes at cervical, lumbar, and mixed levels reported symptomatic improvements. Some of the most promising improvements were observed in patients where stimulation occurred at the mid and upper thoracic levels (T2-T9). This seems to match the conclusions stated by Fonoff et al. [52], suggesting that the area between T4 and T5 might be the most promising segment for stimulation due to the narrowness of the canal at this area and the relatively small amount of cerebrospinal fluid between the lead at the spinal cord. Patients can have implanted either a paddle lead which requires an open procedure but carries a smaller risk of migration or a percutaneous cylindrical lead, which carries fewer risks associated with the surgical procedure but a higher risk of migration and misplacement [58, 59].
Conclusion and implications for future research
Some studies suggest that the effects of SCS can be further enhanced when done in conjunction with an adequate amount of dopamine medication and DBS, even when these two therapies alone are no longer sufficient to address the refractory PD symptoms [21, 38].
In conclusion, spinal cord stimulation for PIGD in PD is a therapy that is worth investigating further. The existing evidence within this patient population, weak as it is, suggests that the procedure is relatively safe and may have beneficial effects on QoL and motor scores. There is a pressing need for an adequately powered clinical trial with clearly described statistical analysis methods, patient cohort and tools for clinical evaluation. This will aid us to draw more solid conclusions regarding the therapeutic potential of SCS in PD patients with gait complications and open new research opportunities in the field of preventative medicine or combined treatment.
ACKNOWLEDGMENTS
We would like to thank Prof. Tom Foltynie from the UCL Queen Square Institute of Neurology, Ms. Kate Brunskill from the Queen Square Library, and Ms. Linda Taib from the UCL Queen Square Institute of Neurology for their support. Harith Akram is supported by funding from the NIHR UCLH Brain Research Council and the Brain Research Trust.
CONFLICT OF INTEREST
The authors have no conflict of interest to report.
SUPPLEMENTARY MATERIAL
[1] The supplementary material is available in the electronic version of this article: https://dx.doi.org/10.3233/JPD-223284.
REFERENCES
[1] | Shulman LM ((2010) ) Understanding disability in Parkinson’s disease. Mov Disord 25: , S131–S135. |
[2] | Suarez-Cedeno G , Suescun J , Schiess MC ((2017) ) Earlier intervention with deep brain stimulation for Parkinson’s disease. Parkinsons Dis 2017: , 9358153. |
[3] | Massano J , Garrett C ((2012) ) Deep brain stimulation and cognitive decline in Parkinson’s disease: A clinical review. Front Neurol 3: , 66. |
[4] | Fasano A , Aquino CC , Krauss JK , Honey CR , Bloem BR ((2015) ) Axial disability and deep brain stimulation in patients with Parkinson disease. Nat Rev Neurol 11: , 98–110. |
[5] | Moore O , Peretz C , Giladi N ((2007) ) Freezing of gait affects quality of life of peoples with Parkinson’s disease beyond its relationships with mobility and gait. Mov Disord 22: , 2192–2195. |
[6] | Lucas McKay J , Goldstein FC , Sommerfeld B , Bernhard D , Perez Parra S , Factor SA ((2019) ) Freezing of Gait can persist after an acute levodopa challenge in Parkinson’s disease. NPJ Parkinsons Dis 5: , 25. |
[7] | Palakurthi B , Burugupally SP ((2019) ) Postural Instability in Parkinson’s disease: A review. Brain Sci 9: , 239. |
[8] | Müller MLTM , Marusic U , van Emde Boas M , Weiss D , Bohnen NI ((2019) ) Treatment options for postural instability and gait difficulties in Parkinson’s disease. Expert Rev Neurother 19: , 1229–1251. |
[9] | Liu X , Zhao C , Zheng B , Guo Q , Duan X , Wulamu A , Zhang D ((2021) ) Wearable devices for gait analysis in intelligent healthcare. Front Comput Sci 3: . https://doi.org/10.3389/fcomp.2021.661676 |
[10] | di Biase L , di Santo A , Caminiti ML , de Liso A , Shah SA , Ricci L , di Lazzaro V ((2020) ) Gait analysis in Parkinson’s disease: An overview of the most accurate markers for diagnosis and symptoms monitoring. Sensors 20: , 3529. |
[11] | Han JL , Murphy KR , Hussaini SMQ , Yang S , Parente B , Xie J , Pagadala P , Lad SP ((2017) ) Explantation rates and healthcare resource utilization in spinal cord stimulation. Neuromodulation 20: , 331–339. |
[12] | Pederson A (2020) What to expect in neuromodulation this year. Medical Device and Diagnostic Industry, https://www.mddionline.com/business/what-expect-neuromodulation-year |
[13] | Moore DM , McCrory C ((2016) ) Spinal cord stimulation. BJA Educ 16: , 258–263. |
[14] | Lamy J , Varriale P , Apartis E , Mehdi S , Blancher-Meinadier A , Kosutzka Z , Degos B , Frismand S , Simonetta-Moreau M , Meunier S , Roze E , Vidailhet M ((2021) ) Trans-spinal direct current stimulation for managing primary orthostatic tremor. Mov Disord 36: , 1835–1842. |
[15] | Zhong H , Zhu C , Minegishi Y , Richter F , Zdunowski S , Roy RR , Vissel B , Gad P , Gerasimenko Y , Chesselet M-F , Edgerton VR ((2019) ) Epidural spinal cord stimulation improves motor function in rats with chemically induced parkinsonism. Neurorehabil Neural Repair 33: , 1029–1039. |
[16] | Yadav A , Borda E , Nicolelis M ((2018) ) Closed loop spinal cord stimulation restores locomotion and desynchronizes corticostriatal beta oscillations [abstract]. Mov Disord 33: (Suppl 2). https://www.mdsabstracts.org/abstract/closed-loop-spinal-cord-stimulation-restores-locomotion-and-desynchronizes-corticostriatal-beta-oscillations/ |
[17] | Shinko A , Agari T , Kameda M , Yasuhara T , Kondo A , Tayra JT , Sato K , Sasaki T , Sasada S , Takeuchi H , Wakamori T , Borlongan C v , Date I ((2014) ) Spinal cord stimulation exerts neuroprotective effects against experimental Parkinson’s disease. PLoS One 9: , e101468. |
[18] | Santana MB , Halje P , Simplicio H , Richter U , Freire MAM , Petersson P , Fuentes R , Nicolelis MAL ((2014) ) Spinal cord stimulation alleviates motor deficits in a primate model of Parkinson disease. Neuron 84: , 716–722. |
[19] | Fuentes R , Petersson P , Siesser WB , Caron MG , Nicolelis MAL ((2009) ) Spinal cord stimulation restores locomotion in animal models of Parkinson’s disease. Science 323: , 1578–1582. |
[20] | Mazzone P , Viselli F , Ferraina S , Giamundo M , Marano M , Paoloni M , Masedu F , Capozzo A ((2019) ) High cervical spinal cord stimulation: A one year follow-up study on motor and non-motor functions in Parkinson’s disease. Brain Sci 9: , 78. |
[21] | Hubsch C , D’Hardemare V , ben Maacha M , Ziegler M , Patte-Karsenti N , Thiebaut JB , Gout O ((2019) ) Tonic spinal cord stimulation as therapeutic option in Parkinson disease with axial symptoms: Effects on walking and quality of life. Parkinsonism Relat Disord 63: , 235–237. |
[22] | Samotus O , Parrent A , Jog M ((2018) ) Spinal cord stimulation therapy for gait dysfunction in advanced Parkinson’s disease patients. Mov Disord 33: , 783–792. |
[23] | de Lima-Pardini AC , Coelho DB , Souza CP , Souza CO , dos Ghilardi MGS , Garcia T , Voos M , Milosevic M , Hamani C , Teixeira LA , Fonoff ET ((2018) ) Effects of spinal cord stimulation on postural control in Parkinson’s disease patients with freezing of gait. Elife 7: , e37727. |
[24] | Rohani M , Kalsi-Ryan S , Lozano AM , Fasano A ((2017) ) Spinal cord stimulation in primary progressive freezing of gait. Mov Disord 32: , 1336–1337. |
[25] | Cecile H , Vincent DH , Malek BM , Nathalie PK , Jean-Batiste T , Marc Z , Jean-Philippe B ((2017) ) Spinal cord stimulation as therapeutic option in gait disorder in Parkinson’s disease. Mov Disord 32: , 940–941. |
[26] | Nishioka K , Nakajima M ((2015) ) Beneficial therapeutic effects of spinal cord stimulation in advanced cases of Parkinson’s disease with intractable chronic pain: A case series. Neuromodulation 18: , 751–753. |
[27] | Ichikawa T , Oshima H , Nishida Y ((2013) ) Spinal cord stimulation is effective to gait disturbances with Parkinson’s disease. J Neurol Sci 333: , e72. |
[28] | Mitsuyama T , Goto S , Sasaki T , Taira T ((2013) ) Spinal cord stimulation for chronic lumbar pain in patients with Parkinson’s disease. Stereotact Funct Neurosurg 91: , 273. |
[29] | Agari T , Date I ((2012) ) Spinal cord stimulation for the treatment of abnormal posture and gait disorder in patients with Parkinson’s disease. Neurol Med Chir (Tokyo) 52: , 470–474. |
[30] | Thevathasan W , Mazzone P , Jha A , Djamshidian A , Dileone M , Di Lazzaro V , Brown P ((2010) ) Spinal cord stimulation failed to relieve akinesia or restore locomotion in Parkinson disease. Neurology 74: , 1325–1327. |
[31] | Lai Y , Pan Y , Wang L , Zhang C , Sun B , Li D ((2020) ) Spinal cord stimulation with surgical lead improves pain and gait in Parkinson’s disease after a dislocation of percutaneous lead: A case report. Stereotact Funct Neurosurg 98: , 104–109. |
[32] | Kobayashi R , Kenji S , Taketomi A , Murakami H , Ono K , Otake H ((2018) ) New mode of burst spinal cord stimulation improved mental status as well as motor function in a patient with Parkinson’s disease. Parkinsonism Relat Disord 57: , 82–83. |
[33] | Akiyama H , Nukui S , Akamatu M , Hasegawa Y , Nishikido O , Inoue S ((2017) ) Effectiveness of spinal cord stimulation for painful camptocormia with Pisa syndrome in Parkinson’s disease: A case report. BMC Neurol 17: , 148. |
[34] | Hassan S , Amer S , Alwaki A , Elborno A ((2013) ) A patient with Parkinson’s disease benefits from spinal cord stimulation. J Clin Neurosci 20: , 1155–1156. |
[35] | Landi A , Trezza A , Pirillo D , Vimercati A , Antonini A , Sganzerla pietro E ((2013) ) Spinal cord stimulation for the treatment of sensory symptoms in advanced Parkinson’s disease. Neuromodulation 16: , 276–279. |
[36] | Soltani F , Lalkhen A ((2013) ) Improvement of parkinsonian symptoms with spinal cord stimulation: Consequence or coincidence? J Neurol Neurosurg Psychiatry 84: , e2.74–e2. |
[37] | Fénelon G , Goujon C , Gurruchaga J-M , Cesaro P , Jarraya B , Palfi S , Lefaucheur J-P ((2011) ) Spinal cord stimulation for chronic pain improved motor function in a patient with Parkinson’s disease. Parkinsonism Relat Disord 18: , 213–214. |
[38] | Weise D , Winkler D , Meixensberger J , Classen J ((2010) ) Effects of spinal cord stimulation in a patient with Parkinson’s disease and chronic back pain. J Neurol 257: , S217. |
[39] | Tieppo Francio V , Polston KF , Murphy MT , Hagedorn JM , Sayed D ((2021) ) Management of chronic and neuropathic pain with 10 kHz spinal cord stimulation technology: Summary of findings from preclinical and clinical studies. Biomedicines 9: , 644. |
[40] | Kapural L , Yu C , Doust MW , Gliner BE , Vallejo R , Sitzman BT , Amirdelfan K , Morgan DM , Yearwood TL , Bundschu R , Yang T , Benyamin R , Burgher AH ((2016) ) Comparison of 10-kHz high-frequency and traditional low-frequency spinal cord stimulation for the treatment of chronic back and leg pain. Neurosurgery 79: , 667–677. |
[41] | Petersen EA , Stauss TG , Scowcroft JA , Brooks ES , White JL , Sills SM , Amirdelfan K , Guirguis MN , Xu J , Yu C , Nairizi A , Patterson DG , Tsoulfas KC , Creamer MJ , Galan V , Bundschu RH , Paul CA , Mehta ND , Choi H , Sayed D , Lad SP , DiBenedetto DJ , Sethi KA , Goree JH , Bennett MT , Harrison NJ , Israel AF , Chang P , Wu PW , Gekht G , Argoff CE , Nasr CE , Taylor RS , Subbaroyan J , Gliner BE , Caraway DL , Mekhail NA ((2021) ) Effect of high-frequency (10-kHz) spinal cord stimulation in patients with painful diabetic neuropathy. JAMA Neurol 78: , 687. |
[42] | de Carolis G , Paroli M , Tollapi L , Doust MW , Burgher AH , Yu C , Yang T , Morgan DM , Amirdelfan K , Kapural L , Sitzman BT , Bundschu R , Vallejo R , Benyamin RM , Yearwood TL , Gliner BE , Powell AA , Bradley K ((2017) ) Paresthesia-independence: An assessment of technical factors related to 10 kHz paresthesia-free spinal cord stimulation. Pain Physician 20: , 331–341. |
[43] | Duarte RV , McNicol E , Colloca L , Taylor RS , North RB , Eldabe S ((2020) ) Randomized placebo-/sham-controlled trials of spinal cord stimulation: A systematic review and methodological appraisal. Neuromodulation 23: , 10–18. |
[44] | Moher D , Liberati A , Tetzlaff J , Altman DG , RISMA Group ((2009) ) Preferred reporting items for systematic reviews and meta-analyses: The PRISMA statement. PLoS Med 6: , e1000097. |
[45] | Bettany-Saltikov J ((2010) ) Learning how to undertake a systematic review: Part 1. Nurs Stand 24: , 47–55. |
[46] | RoB 2:Arevised Cochrane risk-of-bias tool for randomized trials. https://methods.cochrane.org/bias/resources/rob-2-revised-cochrane-risk-bias-tool-randomized-trials |
[47] | Zeng X , Zhang Y , Kwong JSW , Zhang C , Li S , Sun F , Niu Y , Du L ((2015) ) The methodological quality assessment tools for preclinical and clinical studies, systematic review and meta-analysis, and clinical practice guideline: A systematic review. J Evid Based Med 8: , 2–10. |
[48] | JBI Critical Appraisal Checklist for Case Reports. https://jbi.global/sites/default/files/2019-05/JBI_Critical_Appraisal-Checklist_for_Case_Reports2017_0.pdf |
[49] | JBI Critical Appraisal Checklist for Case Series. https://jbi.global/sites/default/files/2019-05/JBI_Critical_Appraisal-Checklist_for_Case_Series2017_0.pdf |
[50] | Duarte RV , Nevitt S , McNicol E , Taylor RS , Buchser E , North RB , Eldabe S ((2020) ) Systematic review and meta-analysis of placebo/sham controlled randomised trials of spinal cord stimulation for neuropathic pain. Pain 161: , 24–35. |
[51] | de Souza CP , dos Santos MGG , Hamani C , Fonoff ET ((2018) ) Spinal cord stimulation for gait dysfunction in Parkinson’s disease: Essential questions to discuss. Mov Disord 33: , 1828–1829. |
[52] | Fonoff ET , de Lima-Pardini AC , Coelho DB , Monaco BA , Machado B , Pinto de Souza C , dos Santos Ghilardi MG , Hamani C ((2019) ) Spinal cord stimulation for freezing of gait: From bench to bedside. Front Neurol 10: , 905. |
[53] | Nissen T , Wynn R ((2014) ) The clinical case report: A review of its merits and limitations. BMC Res Notes 7: , 264. |
[54] | Chan K , Bhandari M ((2011) ) Three-minute critical appraisal of a case series article. Indian J Orthop 45: , 103–104. |
[55] | Borzì L , Varrecchia M , Olmo G , Artusi CA , Fabbri M , Rizzone MG , Romagnolo A , Zibetti M , Lopiano L ((2019) ) Home monitoring of motor fluctuations in Parkinson’s disease patients. J Reliab Intell Environ 5: , 145–162. |
[56] | Beck EN , Ehgoetz Martens KA , Almeida QJ ((2015) ) Freezing of gait in Parkinson’s disease: An overload problem? PLoS One 10: , e0144986. |
[57] | Moore ST , MacDougall HG , Ondo WG ((2008) ) Ambulatory monitoring of freezing of gait in Parkinson’s disease. J Neurosci Methods 167: , 340–348. |
[58] | Babu R , Hazzard MA , Huang KT , Ugiliweneza B , Patil CG , Boakye M , Lad SP ((2013) ) Outcomes of percutaneous and paddle lead implantation for spinal cord stimulation: A comparative analysis of complications, reoperation rates, and health-care costs. Neuromodulation 16: , 418–427. |
[59] | Kim TH , Lee PB , Son HM , Choi JB , Moon JY ((2010) ) Spontaneous lead breakage in implanted spinal cord stimulation systems. Korean J Pain 23: , 78–81. |