Blood and Cerebrospinal Fluid Biomarkers of Inflammation in Parkinson’s Disease
Abstract
Given the clear role of inflammation in the pathogenesis of Parkinson’s disease (PD) and its impact on incidence and phenotypical characteristics, this review provides an overview with focus on inflammatory biofluid markers in blood and cerebrospinal fluid (CSF) in PD patient cohorts. In preparation for clinical trials targeting the immune system, we specifically address the following questions: 1) What evidence do we have for pro-inflammatory profiles in blood and in CSF of sporadic and genetic PD patients? 2) Is there a role of anti-inflammatory mediators in blood/CSF? 3) Do inflammatory profiles in blood reflect those in CSF indicative of a cross-talk between periphery and brain? 4) Do blood/CSF inflammatory profiles change over the disease course as assessed in repeatedly taken biosamples? 5) Are blood/CSF inflammatory profiles associated with phenotypical trajectories in PD? 6) Are blood/CSF inflammatory profiles associated with CSF levels of neurodegenerative/PD-specific biomarkers? Knowledge on these questions will inform future strategies for patient stratification and cohort enrichment as well as suitable outcome measures for clinical trials.
Abbreviations:
AFP | Alpha Fetoprotein |
NAP-3 (CXCL1) | Autonomic Nervous System |
BDNF | Anti-Neutrophil Activating Protein 3 |
C3 | Complement component 3 |
C4 | Complement component 4 |
CA-125 | Cancer Antigen 125 |
CEA | Carcinoembryonic Antigen |
CRP | C-reactive Protein |
CKMB | Creatine Kinase Muscle Brain type |
CCL11 | Eotaxin |
ENA-78 (CXCL5) | AEpithelial-derived neutrophil-activating peptide 78 |
FactorVII | FactorVII |
FABP | Fatty Acid Binding Protein |
CX3CL1 | Fractalkine |
GH | Growth Hormone |
IgE | Immunoglobulin E |
ICAM-1 | Intercellular Adhesion Molecule 1 |
IFN-γ | Interferon-Gamma |
IP-9 (CXCL11) | Interferon-Gamma Induced Protein-9 |
IP-10 (CXCL10) | Interferon-Gamma Induced Protein-10 |
IL-1α | Interleukin-1 alpha |
IL-1β | Interleukin-1 beta |
IL-1RA | Interleukin-1 Receptor Antagonist |
IL-2 | Interleukin-2 |
IL-3 | Interleukin-3 |
IL-4 | Interleukin-4 |
IL-5 | Interleukin-5 |
IL-6 | Interleukin-6 |
IL-7 | Interleukin-7 |
IL-8 | Interleukin-8 |
IL-9 | Interleukin-9 |
IL-10 | Interleukin-10 |
IL-12p40 | Interleukin-12p40 |
IL-12p70 | Interleukin-12p70 |
IL-13 | Interleukin-13 |
IL-15 | Interleukin-15 |
IL-16 | Interleukin-16 |
IL-17A | Interleukin-17 A |
IL-18 | Interleukin-18 |
IL-21 | Interleukin-21 |
IL-22 | Interleukin-22 |
IL-27 | Interleukin-27 |
Leptin | Leptin |
Lymphotactin | Lymphotactin |
CSF-1 | Macrophage Colony CSF-1 Stimulating Factor 1 |
MDC | Macrophage Derived MDC Chemokine |
MIP-1β | Macrophage Inflammatory Protein 1 Alpha |
MIP-1α | Macrophage Inflammatory Protein 1 Beta |
MIP-3α (CCL20) | Autonomic Nervous System |
MIP-3 (CCL23) | Macrophage Inflammatory Protein 3 Alpha |
MMP3 | Matrix Metallopeptidase 3 |
MMP9 | Matrix Metallopeptidase 9 |
MMP10 | Matrix Metallopeptidase 9 |
MCP-1(CCL2) | Monocyte Chemoattractant Protein 1 |
MCP-2 (CCL8) | Monocyte Chemoattractant Protein 2 |
MCP-4 (CCL13) | Monocyte Chemoattractant Protein 4 |
MIG (CXCL9) | Monokine Induced by Interferon-Gamma |
MEC (CCL28) | Mucosae Associated Epithelial Chemokine |
NGAL | Neutrophil Gelatinase-Associated Lipocalin |
NLRP3 | NLR Family Pyrin Domain Containing 3 |
PDGF-BB | Platelet-Derived Growth Factor BB |
Prolactin | Prolactin |
PSA-f | Prostate Specific Antigen (free) |
CCL5 | RANTES |
S100A8/9 (Calprotectin) | S100 Calcium-Binding Protein A8/9 |
S100B | S100 Calcium-Binding Protein B |
SAA | Serum Amyloid A |
sIL-2R | Soluble Interleukin-2 Receptor |
sTNFR1 | Soluble Tumor Necrosis Factor Receptor 1 |
sTNFR2 | Soluble Tumor Necrosis Factor Receptor 2 |
sVCAM1 | Soluble Vascular Cell Adhesion Molecule 1 |
SCF | Stem Cell Factor |
SDF-1 | Stromal Cell-Derived Factor-1 |
TPO | Thyreoperoxidase |
TSH | Thyroid Stimulating Hormone |
TF | Tissue Factor |
TIMP-2 | Tissue Inhibitor of Metalloproteinases 2 |
TGF-α | Transforming Growth Factor Alpha |
TGF-β1 | Transforming Growth Factor Beta1 |
TNF-α | Tumor Necrosis Factor beta Factor beta |
YKL-40 | Chitinase-3-like 1 |
INTRODUCTION
In recent years, a growing number of epidemiological and genetic studies as well as post-mortem and biofluid marker analyses including unbiased proteomic approaches provide evidence for a relevant influence of inflammation on both incidence and progression in Parkinson’s disease (PD) [1–8]. In this context, the cerebral and peripheral as well as the innate and adaptive immune system seem involved [9]. The activation of microglia as representative of the cerebral innate immune system was shown postmortem and in vivo in PD patients by positron emission tomography (PET) studies ([11C]PK11195, [18F]FEPPA, [11C]PBR28) and by increased levels of cytokines in cerebrospinal fluid (CSF) [2, 10]. Microglia is activated by damage-associated molecular patterns (DAMPs), which are generated by damaged cells, misfolded proteins, and protein aggregates. In PD, α-synuclein acts as DAMP resulting in microglia activation with induction of neuroinflammation and release of cytokines/chemokines [11]. Moreover, there is increasing evidence for the involvement of the peripheral innate and adaptive immune system in the pathophysiology of PD. In this context, α-synuclein promotes inflammasome-related cytokine production in the periphery and specific α-synuclein peptides act as antigenic epitopes resulting in helper and cytotoxic T cell responses in peripheral blood mononuclear cells from patients with PD [12, 13].
Postmortem and biofluid (blood, CSF) studies reported that increased inflammatory profiles are associated with clinical subtypes of PD, promoting an accelerated motor and non-motor phenotype [3, 14–17]. Recent evidence highlights that the involvement of inflammation in PD is maximized in the early disease stages and maintains a chronic profile during the course of the disease [18, 19] (Fig. 1).
Fig. 1
Inflammatory markers in blood and CSF that are associated with clinical trajectories, disease progression, and neurodegenerative/disease-specific protein levels. In line with evidence from post-mortem and cell studies, blood and CSF levels of inflammatory markers indicate a contribution of the innate and adaptive immune system in both CNS and periphery. Of these, the most frequently reported inflammatory markers in blood and CSF that are associated with clinical trajectories, disease progression and neurodegenerative/disease-specific protein levels are IL-1β, IL-6, INF-γ, MCP-1 and TNF-α. Notably, inflammation is maximized in the early disease stages and maintains a chronic profile during the course of the disease. Figure created in BioRender.com.
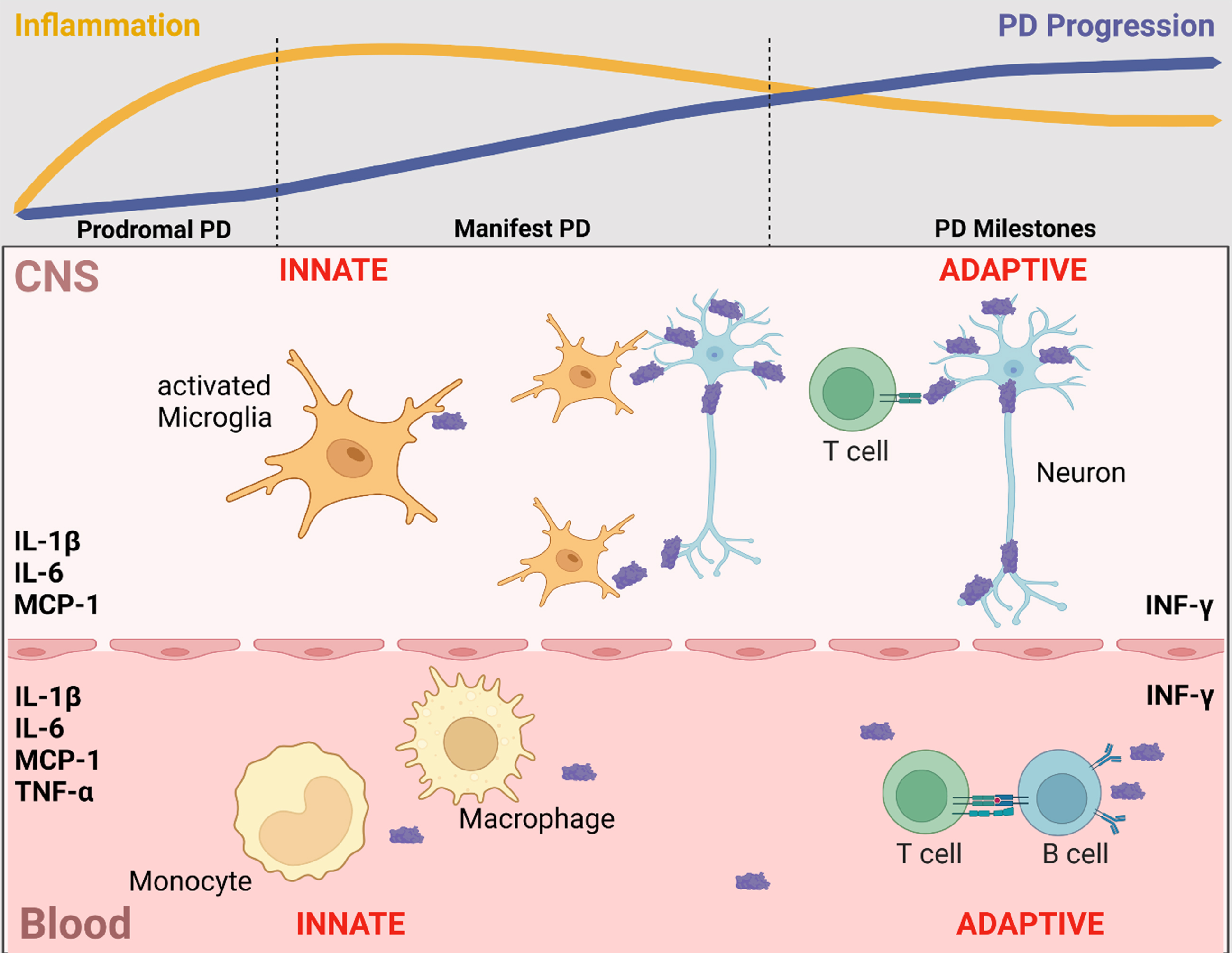
Despite this clear role for inflammation in the pathogenesis of PD, several open questions remain to be answered in preparation of clinical trials aiming at disease-modification by targeting the immune system: 1) What evidence do we have for pro-inflammatory profiles in blood and in CSF of sporadic and genetic PD patients? 2) Is there a role of anti-inflammatory mediators in blood/CSF? 3) Do inflammatory profiles in blood reflect those in CSF indicative of a cross-talk between periphery and brain? 4) Do blood/CSF inflammatory profiles change over the disease course as assessed in repeatedly taken biosamples? 5) Are blood/CSF inflammatory profiles associated with phenotypical trajectories in PD? 6) Are blood/CSF inflammatory profiles associated with CSF levels of neurodegenerative/PD-specific biomarkers? 7) Which inflammatory markers in blood/CSF are the most promising candidates for clinical trials with regard to patient stratification, cohort enrichment and outcome measures?
This review gives an overview of the current knowledge on these questions with specific focus on blood (plasma, serum) and CSF levels of inflammatory biofluid markers in PD patient cohorts. We did a PubMed search using the search terms “Parkinson, Inflammation, Interleukin, Chemokine, Cytokine, Blood, CSF” and included publications between 01/2011-01/2022. Studies on white blood cells of the myeloid and lymphoid cell lineage as well as on postmortem tissue, imaging, and cell/animal models are not included in the present manuscript as these are reviewed in other articles from this special issue on “The immune system in Parkinson’s disease”.
Please note that the assessed cohorts largely vary in sample size and disease duration. Further, the type of assays used, differs and ranges from singleplex (ELISA) to multiplex immunoassays with different metrics of quality control (lower limit of quantification, number of replicates, variation coefficient, etc.). Additionally, it is well known that females and males differ in their immune system. They show distinct patterns in innate and adaptive immune responses which further change across the lifespan [20]. In general, females show stronger innate and adaptive immune responses than males with increased production of antibodies and anti-inflammatory species such as IL-4 and IL-10. Taking these gender differences into consideration is of importance when analysing immune marker profiles in different cohorts against disease status. However, only few studies stratified their analyses by sex. All these points pose limitations and have to be kept in mind for data interpretation and drawing congruent conclusions.
WHAT EVIDENCE DO WE HAVE FOR PRO-INFLAMMATORY PROFILES IN BLOOD AND IN CSF OF SPORADIC AND GENETIC PD PATIENTS?
In preparation of upcoming clinical trials targeting the immune system, it will be essential to translate basic research findings from cell and animal models into patient cohorts and to define markers that are representative of inflammation, that are suited for patient stratification and cohort enrichment, that are predictive/prognostic for different trajectories and that serve as read-out for target engagement. Moreover, such markers should be longitudinally accessible in a multi-centre design. While specific imaging techniques such as PET might not be readily available all over the world, blood can be repeatedly collected and stored for longitudinal biofluid marker analyses.
Most studies on inflammatory biofluid markers have been conducted cross-sectionally in blood comparing levels of cytokines between patients with sporadic PD and healthy controls.
Inflammatory markers in blood
Over 50 different pro-inflammatory biofluid markers have been assessed in serum or plasma. However, only for 7 markers (CRP, IL-1β, IL-2, IL-6, IL-8, IFN-γ, TNF-α) more than 5 studies are available. The most robust data are published for CRP [21–34] and IL-1β [25–27, 35–44]. These two show consistently higher levels in sporadic PD patients compared to healthy controls. Data for IL-2 [25, 27–29, 40, 45, 46], IL-6 [21, 25–29, 35, 37, 39–42, 45–50], IL-8 [25, 28, 29, 35, 46, 51, 52], IFN-γ [25, 26, 28, 29, 40, 46, 50, 53–55], and TNF-α [25–29, 35, 36, 39–41, 46, 50, 51, 53, 54, 56–59] are less clear with some studies reporting higher blood levels but others showing no differences or even lower levels in sporadic PD patients when compared to healthy controls. A detailed overview of all assessed blood markers along with the respective findings and references is given in Table 1.
Table 1
Findings of levels of inflammatory markers in sporadic PD patients in blood (serum, plasma) and CSF compared to healthy controls from 01/2012-12/2021
Blood (PD vs. CON) | CSF (PD vs. CON) | |
C3 | Sun et al. [96] 2019 ↓ | |
C4 | Sun et al. [96] 2019 ↓ | |
CCL2 (MCP-1) | Csencsits-Smith et al. [58] 2016 ↑ | Hall et al. [63] 2018 ⟶ |
Schröder et al. [46] 2018 ⟶ | Schröder et al. [46] 2018 ↑ | |
Usenko et al. [55] 2020 ↑ | ||
Miliukhina et al. [50] 2020 ↓ | ||
CCL3 (MIP-1α) | Schröder et al. [46] 2018 ⟶ | Schröder et al. [46] 2018 ⟶ |
Calvani et al. [52] 2020 ↓ | ||
CCL4 (MIP-1β) | Schröder et al. [46] 2018 ⟶ | |
Calvani et al. [52] 2020 ↑ | ||
CCL5 (RANTES) | Mahlknecht et al. [45] 2012 ⟶ | |
Tang et al. [47] 2014 ↑ | ||
Qin et al. [25] 2016 ↑ | ||
Schröder et al. [46] 2018 ⟶ | ||
CCL8 (MCP-2) | Santaella et al. [65] 2020 ↓ | |
CCL11 (Eotaxin) | Schröder et al. [46] 2018 ⟶ | |
CCL13 (MCP-4) | Mahlknecht et al. [45] 2012 ⟶ | |
CCL17 (TARC) | Schröder et al. [46] 2018 ⟶ | |
CCL20 (MIP-3α) | Schröder et al. [46] 2018 ⟶ | |
CCL23 (MIP-3) | Santaella et al. [65] 2020 ↓ | |
CCL28 (MEC) | Santaella et al. [65] 2020 ↑ | |
CRP | Ton et al. [21] 2012 ↓ | Hall et al. [63] 2018 ⟶ |
Andican et al. [22] 2012 ↑ | Moghaddam et al. [87] 2018 ↑ | |
Lindqvist et al. [23] 2013 ↑ | ||
Sawada et al. [24] 2014 ↑ | ||
Qin et al. [25] 2016 ↑ | ||
Wang et al. [26] 2016 ↑ | ||
Kim et al. [27] 2018 ⟶ | ||
King et al. [28, 29] 2019 ⟶ | ||
Santos-García et al. [30] 2019 ↑ | ||
Qiu et al. [31] 2019 ↑ | ||
Baran et al. [32] 2019 ↑ | ||
Jin et al. [33] 2020 ↑ | ||
Dommershuijsen et al. [34] 2022 ⟶ | ||
CSF-1 | Santaella et al. [65] 2020 ⟶ | |
CX3CL1 (Fractalkine) | Gupta et al. [78] 2021 ↑ | Santaella et al. [65] 2020 ↓ |
Hatcher-Martin et al. [89] 2021 ↓ | ||
CXCL1 (NAP-3) | Schröder et al. [46] 2018 ⟶ | Santaella et al. [65] 2020 ↓ |
CXCL5 (ENA78) | Schröder et al. [46] 2018 ⟶ | |
CXCL9 (MIG) | Schröder et al. [46] 2018 ⟶ | |
CXCL10 (IP-10) | Schröder et al. [46] 2018 ⟶ | Schröder et al. [46] 2018 ⟶ |
Csencsits-Smith et al. [58] 2016 ↑ | Hu et al. [64] 2019 ⟶ | |
CXCL11 (IP-9) | Schröder et al. [46] 2018 ⟶ | |
CXCL12 (SDF-1) | Bagheri et al. [97] 2018 ↑ | |
FABP | Brockmann et al. [38] 2016 ↑ | |
ICAM-1 | Andican et al. [22] 2012 ↑ | |
Mahlknecht et al. [45] 2012 ⟶ | ||
IL-1ß | Koziorowski et al. [35] 2012 ⟶ | Milyukhina et al. [37] 2015 ↑ |
Hu et al. [36] 2015 ↑ | Hu et al. [36] 2015 ↑ | |
Milyukhina et al. [37] 2015 ↑ | Chen et al. [60] 2018 ↑ | |
Brockmann et al. [38] 2016 ↑ | Iwaoka et al. [61] 2020 ↑ | |
Wang et al. [26] 2016 ↑ | ||
Qin et al. [25] 2016 ↑ | ||
Karpenko et al. [39] 2018 ↑ | ||
Kim et al. [27] 2018 ↑ | ||
Rocha et al. [40] 2018 ⟶ | ||
Alrafiah et al. [41] 2019 ↑ | ||
Lian et al. [42] 2019 ↑ | ||
Chatterjee et al. [43] 2020 ↑ | ||
Fan et al. [44] 2020 ↑ | ||
IL-1RA | Karpenko et al. [39] 2018 ↓ | |
IL-2 | Mahlknecht et al. [45] 2012 ⟶ | Schröder et al. [46] 2018 ↑ |
Qin et al. [25] 2016 ↑ | ||
Kim et al. [27] 2018 ↑ | ||
Rocha et al. [40] 2018 ⟶ | ||
Schröder et al. [46] 2018 ⟶ | ||
King et al. [28, 29] 2019 ⟶ | ||
sIL-2R | Wang et al. [26] 2016 ↑ | |
IL-4 | Qin et al. [25] 2016 ⟶ | Schröder et al. [46] 2018 ⟶ |
Rocha et al. [40] 2018 ↓ | ||
Schröder et al. [46] 2018 ⟶ | ||
King et al. [28, 29] 2019 ⟶ | ||
IL-5 | Schröder et al. [46] 2018 ⟶ | |
IL-6 | Ton et al. [21] 2012 ↑ | Yu et al. [62] 2014: ↑ |
Koziorowski et al. [35] 2012 ⟶ | Milyukhina et al. [37] 2015 ↑ | |
Mahlknecht et al. [45] 2012 ⟶ | Delgado-Alvarado et al. [48] 2017 ⟶ | |
Tang et al. [47] 2014 ↑ | Chen et al. [60] 2018 ↑ | |
Milyukhina et al. [37] 2015 ↑ | Hall et al. [63] 2018 ⟶ | |
Wang et al. [26] 2016 ↑ | Schröder et al. [46] 2018 ↑ | |
Qin et al. [25] 2016 ↑ | Lian et al. [42] 2019 ↑ | |
Delgado-Alvarado et al. [48] 2017 ⟶ | Iwaoka et al. [61] 2020 ⟶ | |
Karpenko et al. [39] 2018 ↑ | ||
Kim et al. [27] 2018 ↑ | ||
Rocha et al. [40] 2018 ↓ | ||
Schröder et al. [46] 2018 ⟶ | ||
Alrafiah et al. [41] 2019 ⟶ | ||
King et al. [28, 29] 2019 ↑ | ||
Lian et al. [42] 2019 ↑ | ||
Kwiatek-Majkusiak et al. [49] 2020 ↑ | ||
Miliukhina et al. [50] 2020 ↓ | ||
IL-8 | Koziorowski et al. [35] 2012 ⟶ | Hall et al. [63] 2018 ↑ |
Gupta et al. [51] 2016 ↓ | Schröder et al. [46] 2018 ⟶ | |
Qin et al. [25] 2016 ⟶ | Hu et al. [64] 2019 ⟶ | |
Schröder et al. [46] 2018 ⟶ | Santaella et al. [65] 2020 ↓ | |
King et al. [28, 29] 2019 ⟶ | ||
Calvani et al. [52] 2020 ↑ | ||
IL-9 | Schröder et al. [46] 2018 ⟶ | Schröder et al. [46] 2018 ↓ |
Calvani et al. [52] 2020 ↓ | ||
IL-10 | Koziorowski et al. [35] 2012 ⟶ | Schröder et al. [46] 2018 ⟶ |
Brockmann et al. [38] 2016 ↑ | Hu et al. [64] 2019 ⟶ | |
Qin et al. [25] 2016 ↑ | ||
Karpenko et al. [39] 2018 ⟶ | ||
Kim et al. [27] 2018 ⟶ | ||
Rocha et al. [40] 2018 ↓ | ||
Schröder et al. [46] 2018 ⟶ | ||
King et al. [28, 29] 2019 ⟶ | ||
Rathnayake et al. [54] 2019 ↑ | ||
Martin-Ruiz et al. [57] 2020 ↑ | ||
IL-12 | Koziorowski et al. [35] 2012 ⟶ | |
IL-12-p40 | Brockmann et al. [38] 2016 ↑ | |
IL-13 | Schröder et al. [46] 2018 ⟶ | |
Lin et al. [53] 2019 ↑ | ||
IL-16 | ||
IL-17A | Rocha et al. [40] 2018 ↓ | |
Schröder et al. [46] 2018 ⟶ | Schröder et al. [46] 2018 ⟶ | Majbour et al. [68] 2020 ↓ |
IL-21 | Schröder et al. [46] 2018 ⟶ | Schröder et al. [46] 2018 ⟶ |
IL-22 | Schröder et al. [46] 2018 ⟶ | Schröder et al. [46] 2018 ⟶ |
IL-27 | Kouchaki et al. [59] 2018 ↓ | |
IFN-y | Wang et al. [26] 2016 ⟶ | Schröder et al. [46] 2018 ⟶ |
Qin et al. [25] 2016 ⟶ | Iwaoka et al. [61] 2020 ⟶ | |
Eidson et al. [56] 2017 ↑ | ||
Rocha et al. [40] 2018 ↓ | ||
Schröder et al. [46] 2018 ⟶ | ||
King et al. [28, 29] 2019 ⟶ | ||
Lin et al. [53] 2019 ↑ | ||
Rathnayake et al. [54] 2019 ↑ | ||
Miliukhina et al. [50] 2020 ↑ | ||
Usenko et al. [55] 2020 ↓ | ||
Leptin | Mahlknecht et al. [45] 2012 ⟶ | |
Rahnemayan et al. [98] 2021 ⟶ | ||
NGAL | Eidson et al. [56] 2017 ↑ | |
NLRP3 | Chatterjee et al. [43] 2020 ↑ | |
Fan et al. [44] 2020 ↑ | ||
Roy et al. [99] 2021 ↑ | ||
PDGF-BB | Mahlknecht et al. [45] 2012 ↑ | |
Prolactin | Mahlknecht et al. [45] 2012 ↑ | |
S100A8/9 (Calprotectin) | Dumitrescu et al. [100] 2021 ↑ | |
S100B | Sathe et al. [101] 2012 ↑ | |
SAA (Serum amyloid A) | Hall et al. [63] 2018 ↑ | |
SCF | Brockmann et al. [38] 2016 ↑ | Santaella et al. [65] 2020 ⟶ |
TGF-β1 | Chen et al. [60] 2018 ↑ | |
TGF-α | Santaella et al. [65] 2020 ⟶ | |
TIMP-2 | Mahlknecht et al. [45] 2012 ⟶ | |
TNF-α | Koziorowski et al. [35] 2012 ↑ | Delgado-Alvarado et al. [48] 2017 ↑ |
Hu et al. [36] 2015 ↑ | Chen et al. [60] 2018 ⟶ | |
Gupta et al. [51] 2016 ↓ | Schröder et al. [46] 2018 ↑ | |
Wang et al. [26] 2016 ↑ | Hu et al. [36, 64] 2015, 2019 ⟶ | |
Qin et al. [25] 2016 ↑ | Iwaoka et al. [61] 2020 ↑ | |
Csencsits-Smith et al. [58] 2016 ↑ | ||
Eidson et al. [56] 2017 ↑ | ||
Karpenko et al. [39] 2018 ⟶ | ||
Kim et al. [27] 2018 ⟶ | ||
Kouchaki et al. [59] 2018 ↑ | ||
Rocha et al. [40] 2018 ↓ | ||
Schröder et al. [46] 2018 ⟶ | ||
Alrafiah et al. [41] 2019 ⟶ | ||
King et al. [28, 29] 2019 ↑ | ||
Lin et al. [53] 2019 ↑ | ||
Rathnayake et al. [54] 2019 ↑ | ||
Martin-Ruiz et al. [57] 2020 ↑ | ||
Miliukhina et al. [50] 2020 ↓ | ||
sTNFR1 and sTNFR2 | Rocha et al. [83] 2014 ↑ | |
sVCAM1 | Perner et al. [80] 2019 ↑ | |
YKL-40 | Hall et al. [63] 2018 ↓ |
↑: Significantly elevated levels in comparison to controls. ⟶: No significant differences in comparison to controls. ↓: Significantly reduced levels in comparison to controls.
Inflammatory markers in CSF
Studies in CSF are less frequent. Overall, 26 pro-inflammatory markers have been assessed. However, only IL-1β, IL-6, IL-8, and TNF-α have been measured in 4 or more studies. Of these, levels of IL-1β were consistently higher in sporadic PD patients compared to healthy controls [36, 37, 60, 61] while levels of IL-6 were also mostly higher in sporadic PD patients but with less robustness across studies [37, 42, 46, 48, 60–63]. Data for IL-8 and TNF-α are less clear with some studies reporting higher CSF levels but others showing no differences or even lower levels in sporadic PD patients when compared to healthy controls [36, 46, 48, 60, 61, 63–65]. A detailed overview of all assessed CSF markers along with the respective findings and references is given in Table 1.
Genetic-associated PD
LRRK2 (Leucine-rich repeat kinase 2)
There are 3 studies available which explored inflammatory markers in PD patients with LRRK2 mutations as well as in non-manifesting LRRK2 mutation carriers.
Two studies with focus on LRRK2 used serum samples from the Michael J. Fox Foundation LRRK2 Cohort Consortium and assessed 32 and 23 inflammatory markers by multiplex assays. Both studies found similar serum levels of inflammatory markers between PD patients with LRRK2 mutations compared to those with LRRK2-wildtype status [38, 66]. In a subgroup with CSF available, higher levels of VEGF and IL-8 were detected in PD patients with LRRK2 mutations compared to those with LRRK2-wildtype status [66]. A recent study from Israel confirmed the findings of relatively similar inflammatory profiles between PD patients with vs. without LRRK2 mutations as the colleagues also did not find any differences in blood or CSF levels of cytokines [67].
Within the group of PD patients with LRRK2 mutations, higher serum levels of IL-8, MCP-1 and MIP1-β seem associated with a more severe phenotype comprising cognitive impairment, orthostatic hypotension and REM-Sleep-behaviour disorder [14].
While 1 of the 3 studies reports higher serum levels of IL-1β [66], the other 2 studies did not find differences in inflammatory profiles in blood and/or CSF between non-manifesting LRRK2 mutation carriers and healthy controls, even when clinically stratified for prodromal markers [38, 67]. A recent study in Norwegian individuals revealed higher CSF levels of TNF-α in LRRK2 mutation carriers when compared to controls [68]. Given these somewhat variable findings, one has to keep in mind that different prodromal symptoms vary in their predictive value to PD conversion and that the rate of progression from prodromal to manifest PD is age-dependent and varies among patients. Therefore, these cross-sectional results in the prodromal cohorts need to be interpreted with caution and warrant further longitudinal studies, ideally in individuals who convert to PD during study.
GBA (Glucocerebrosidase)
While one small study reports increased plasma levels of IFN-γ, IL-1β, IL-2, and TNF-α in 8 PD patients carrying heterozygous mutations in the GBA gene [50], two larger studies did not find any differences in blood and CSF levels between PD patients as well as healthy individuals with vs. without heterozygous GBA mutations [67, 69].
PRKN/PINK1 (Parkin/PINK1)
It has been shown that dysfunction in Parkin and PINK1 enhances mitochondrial stress with activation of inflammatory processes and neurodegeneration via the STING protein cascade (stimulator of interferon genes) [70]. Consequently, PD patients with bi-allelic and heterozygous mutations in PRKN/PINK1 showed increased serum levels of IL-6 in a gene-dosage manner in 3 cohorts [71].
We conclude that the role of inflammation in PD is reflected in increased inflammatory markers in blood and CSF in sporadic PD patients compared to healthy control individuals. Studies in genetically associated PD (LRRK2, GBA, PRKN/PINK1) are rare and indicate similar profiles as seen in sporadic PD. Data in at-risk and prodromal mutation carriers are sparse and conflicting and need further validation in prospectively followed longitudinal cohorts.
IS THERE A ROLE OF ANTI-INFLAMMATORY MEDIATORS IN BLOOD/CSF?
While many studies focus on pro-inflammatory profiles, it is more and more established that inflammation is a balance between pro- and anti-inflammatory processes.
The best investigated anti-inflammatory marker in the context of PD is IL-10 in blood. However, reports are quite heterogenous. While some studies show higher levels in sporadic PD patients, others find no differences or lower levels in sporadic PD patients compared to healthy controls [25, 27–29, 35, 38–40, 46, 54, 57]. Two studies have been done in CSF and found no differences between sporadic PD patients and healthy controls [46, 64].
Within the group of PD patients, single studies report higher blood levels of IL-10 to be associated with motor and cognitive impairment as well as with higher CSF levels of α-synuclein [55, 57]. Whether such findings represent compensatory upregulation to keep the balance between pro-and anti-inflammatory species remains to be elucidated. A detailed overview of the respective findings in blood and CSF along with references is given in Table 1.
DO INFLAMMATORY PROFILES IN BLOOD REFLECT THOSE IN CSF INDICATIVE OF A CROSS-TALK BETWEEN PERIPHERY AND BRAIN?
When designing clinical trials, one has to decide which biomaterial should to be repeatedly collected for outcome measurements. In this context, blood is more easily accessible than CSF. However, we should know whether blood profiles are representative for profiles in CSF and can serve as proxy for central-nervous system neuroinflammation?
So far, there are 3 comprehensive studies published in sporadic PD that assessed a variety of inflammatory markers by multiplex assay in CSF/serum pairs [16, 56, 72]. The 2 smaller cohorts comprised 12 and 22 PD patients whereas our own cohort consisted of 453 sporadic PD patients. Importantly, the platform/panel (Mesoscale: V-PLEX: IFN-γ, IL-1β, IL-2, IL-4, IL-6, IL-8, IL-10, IL-12p70, IL-13, TNF-α) that was used in the 2 smaller studies was identical and fully overlapped with the inflammatory markers assessed in our own study (Myriad: AFP, BDNF, CA-125, CEA, CKMB, ENA-78, FactorVII, FABP, GH, IgE, ICAM-1, IL-1α, IL-1β IL-2, IL-3, IL-4, IL-5, IL-6, IL-7, IL-8, IL-10, IL-12p40, IL-12p70, IL-13, IL-15, IL-16, IL-18, Leptin, Lymphotactin, MDC, MIP-1β, MMP3, MMP9, MCP-1, PSA-f, SCF, TPO, TSH, TF, TNF-α, TNF-β). Results from all 3 studies are quite congruent: (I) Only a fraction of markers is reliably detectable in both CSF and serum. (II) Correlations of cytokines between blood and CSF are sparse. In the 2 smaller studies, none of the markers IL-6, IL-8, IL-10, IFN-γ, TNF-α showed a significant correlation between serum and CSF. The first study with 12 patients additionally assessed CRP which showed a good correlation between serum and CSF. In our larger study of 41 markers, ICAM-1, IL-4, IL-6, IL-12p40, MCP-1, MIP-1β, MMP3, Leptin, and TSH were correlated between CSF and serum in females and in males whereas IL-8, IL-13 and CKMB were correlated only in females.
These findings indicate that inflammatory markers in blood and CSF do not reflect one another and that they might be regulated independently of each other. This poses a challenge for clinical trials. Which biomaterial should to be collected for repeated outcome measurements –the more easily accessible blood or CSF by lumbar puncture?
DO BLOOD/CSF INFLAMMATORY PROFILES CHANGE OVER THE DISEASE COURSE AS ASSESSED IN REPEATEDLY TAKEN BIOSAMPLES?
Inflammatory profiles do not follow a linear pattern as recent evidence highlights that inflammation in PD is maximized in the early disease stages and maintains a chronic profile during the course of the disease. Knowledge of the trajectories of the different biofluid markers in relation to disease progression is important when interpreting therapeutic effects (beneficial/risk) in clinical trials targeting the immune system.
One study used a multiplex assay assessing 37 cytokines in serum of 25 sporadic PD patients every 6 months over 2 years. While levels of IL-4 increased, plasma levels of IL-2, IL-9 and IL-15 decreased over time. Other cytokines did not change significantly over time [58]. Another study reports stable blood levels of CRP over a period of 3 years in 313 PD patients with a mean disease duration of 7.9 years at baseline. Notably, the patients have been stratified by their individual CRP levels at baseline (low, mid, high) [73]. Another study in 47 PD patients showed an increase in serum levels of C3, C4, and IL-6 in 30–50% of patients over a period of 2 years [74].
So far, 1 study reports longitudinal measurements of YKL-40 in repeatedly taken CSF samples of 63 sporadic PD patients. The authors report an increase of CSF levels of YKL-40 over 2 years in the whole PD cohort as well as in subgroups when stratified by disease duration≤or>5 years disease duration [75].
We conclude that large longitudinal studies with repeatedly collected blood and CSF biosamples in de-novo patients over several years are missing. However, such data are of utmost importance and will provide information on how inflammatory profiles evolve over time and in concert with other neurodegenerative/disease-specific biomarkers.
ARE BLOOD/CSF INFLAMMATORY PROFILES ASSOCIATED WITH PHENOTYPICAL TRAJECTORIES IN PD?
In order to enrich cohorts for maximized therapeutic benefit, define clinically reasonable outcome measures and target specific clinical milestones, knowledge on the predictive/prognostic value of inflammatory profiles in relation to clinical trajectories is important.
There are several studies in blood and CSF that investigated inflammatory profiles in relation to phenotypical characteristics of motor and non-motor symptoms, the latter with focus on cognition, depression and sleep. A detailed overview of associations between inflammatory profiles in blood and CSF with phenotypical characteristics is given in Table 2 along with all references.
Table 2
Findings of levels of inflammatory markers in association with clinical characteristics in sporadic PD patients in blood (serum, plasma) and CSF from 01/2012-12/2021
Blood | CSF | |||||||||||
Mortality | Motor function | Cognitive function | Depression | Hallucination | Fatigue Sleep RBD | Mortality | Motor function | Cognitive function | Hallucination | Depression | Fatigue Sleep RBD | |
C3 | Vesely [74, 85] 2018, 2019 | |||||||||||
C4 | Vesely [74] 2018 | |||||||||||
CCL2 MCP-1 | Santaella [88] 2020 | Lerche [72] 2022 | Lindqvist [23] 2013 | Lindqvist [23] 2013 | ||||||||
CRP | Sawada [73] 2015 | Umemura [81] 2015 | Mollenhauer [84] 2019 | Sawada [24] 2014 | Hall [63] 2018 | Lindqvist [23] 2013 | Lindqvist [23] 2013 | Lindqvist [23] 2013 | ||||
Santos-García [30] 2019 | Martin-Ruiz [57] 2020 | Moghaddam [87] 2018 | Hall [63] 2018 | Hall [63] 2018 | ||||||||
Li [77] 2021 | Moghaddam [87] 2018 | Lindqvist [23] 2013 | ||||||||||
CX3CL1 Fractalkine | Gupta [78] 2021 | Hatcher-Martin [89] 2021 | ||||||||||
CXCL10 IP-10 | Lindqvist [23] 2013 | |||||||||||
FABP | Lerche [72] 2022 | Lerche [72] 2022 | ||||||||||
IL-1ß | Fan [44] 2020 | Hu [36] 2015 | ||||||||||
IL-1RA | Herlofson [102] 2018 | |||||||||||
IL-2 | King [28] 2019 | |||||||||||
IL-4 | King [28] 2019 | |||||||||||
IL-6 | Dufek [86] 2015 | Delgado-Alvarado [48] 2017 | Vesely [85] 2019 | Vesely [74] 2018 | Pereira [103] 2016 | Lian [42] 2019 | Lindqvist [23] 2013 | |||||
Green [76] 2019 | Martin-Ruiz [57] 2020 | Yu [62] 2014 | ||||||||||
Lian [42] 2019 | Usenko [55] 2020 | |||||||||||
IL -8 | Lerche [72] 2022 | Lerche [72] 2022 | ||||||||||
IL-10 | Usenko [55] 2020 | Karpenko [104] 2018 | ||||||||||
Martin-Ruiz [57] 2020 | ||||||||||||
IL-12p40 | Yilmaz [82] 2018 | Yilmaz [82] 2018 | ||||||||||
IL-15 | Lerche [72] 2022 | |||||||||||
IL-17A | Green [76] 2019 | Green [76] 2019 | ||||||||||
IL-18 | Lerche [72] 2022 | |||||||||||
IFN-y | Eidson [56] 2017 | Martin-Ruiz [57] 2020 | ||||||||||
MMP10 | Santaella [88] 2020 | |||||||||||
S100B | Carvalho [79] 2015 | Carvalho [79] 2015 | ||||||||||
SAA Serum amyloid A | Hall [63] 2018 | Hall [63] 2018 | Hall [63] 2018 | |||||||||
SCF | Lerche [72] 2022 | |||||||||||
TNF-α | Lerche [72] 2022 | Usenko [55] 2020 | ||||||||||
Martin-Ruiz [57] 2020 | ||||||||||||
Lerche [72] 2022 | ||||||||||||
sTNFR1 sTNFR2 | Rocha [83] 2013 | |||||||||||
sVCAM1 | Perner [80] 2019 | Herlofson [102] 2018 | ||||||||||
YKL-40 | Wennström [90] 2015 Hall [75] 2016 |
Straight = positive associations meaning higher levels of the respective inflammatory marker are associated with worse clinical outcome measure. Italic = Results show an inverse association meaning lower levels of the respective (anti)-inflammatory marker are associated with worse clinical outcome measure. Bold = Studies with longitudinal clinical data. Please note that due to space limitation we only name the first author with year of publication and do not refer to co-authors with “et al.”.
Inflammatory markers in blood: Phenotypical trajectories
It was repeatedly shown cross-sectionally that higher blood levels of IL-6 [42, 48, 76] and CRP [30, 77] are associated with worse motor function. Other single studies further found that higher blood levels of fractalkine [78], IL-1β [44], IL-15 [72], IFN-γ [56], S100B [79], TNF-α [72], and VCAM1 [80] are related to worse motor function. One longitudinal study could further support the finding that higher CRP levels are associated with more severe motor impairment [81]. Contrary, lower blood levels of the anti-inflammatory makers IL-4 [28] and IL-12p40 [82] were also associated with worse motor function.
Several cross-sectional studies report higher levels of CRP, FABP, IL-6, IL-10, IL-17A, TNF-α, and TNFR to be related with worse cognitive function and dementia [55, 72, 76, 83]. One longitudinal study supports the finding that higher levels of CRP are associated with cognitive decline over time [84]. Similarly, it was shown longitudinally that higher levels of C3 and C4 are related to reduced memory function [74, 85]. Another longitudinal study created an inflammatory composite score based on Patients’ individual blood levels of CRP, IFN-γ, IL-6, IL-10, and TNF-α. While a higher composite score predicted worse MoCA scores over 3 years, no association was found with phenoconversion to manifest dementia [57].
Two longitudinal studies report an association between higher levels of CRP and IL-6 with overall mortality in PD [73, 86].
Inflammatory markers in CSF: Phenotypical trajectories
Two studies report higher CSF levels of CRP to be associated with worse motor function [63, 87]. Other single studies found that higher CSF levels of IL-6 [42], IL-8 [72], and SAA [63] are related to worse motor function. One longitudinal study reports that higher CSF levels of MCP-1 and MMP-10 are associated with more severe motor impairment [88]. Contrary, lower levels of the anti-inflammatory marker fractalkine and IL-18 were associated with worse motor function [72, 89].
Several cross-sectional studies report higher CSF levels of CRP and IL-6 to be related with worse cognitive function [23, 63, 87]. Other single studies further found that higher CSF levels of MCP-1, FABP, IL-8, SAA, SCF, and YKL-40 are associated with cognitive impairment [63, 72, 90]. One longitudinal study supports that higher CSF levels of YKL-40 are associated with cognitive decline over time [75].
Higher CSF levels of CRP and MCP-1 were associated with depression and fatigue [23, 63].
We conclude that higher blood and CSF levels of inflammatory markers are associated with more severe clinical trajectories of motor and non-motor symptoms cross-sectionally as well as longitudinally (Fig. 1).
ARE BLOOD/CSF INFLAMMATORY PROFILES ASSOCIATED WITH CSF LEVELS OF NEURODEGENERATIVE/PD-SPECIFIC BIOMARKERS?
In order to map the complex picture of neurodegeneration with its different pathway-related endpoints (α-synuclein aggregation, concomitant amyloid-beta (Aβ) and tau pathology) and (neuro)inflammation, comprehensive biomarker analyses are needed.
The 3 studies that assessed inflammatory markers by multiplex assay in CSF/serum pairs (described above) also performed additional analyses in relation to CSF levels of neurodegenerative/PD-biomarkers [16, 56, 72].
Inflammatory markers in blood: CSF levels of neurodegenerative/PD-specific biomarkers
In our own study, we could show that higher blood levels of ICAM-1, IL-18, and MIP-1β are associated with higher CSF levels of t-Tau and p181-Tau. Higher blood levels of FABP, MMP3, TF, and TNF-α are correlated with higher CSF levels of NFL. Higher blood levels of IL-10, IL-12p40, IL-13, IL-16, IL-18, MCP-1, and MIP-1β are associated with higher CSF levels of α-synuclein. Notably, the majority of these associations were only found in female PD patients [72].
Inflammatory markers in CSF: CSF levels of neurodegenerative/PD-specific biomarkers
In the study by Wijeyekoon, higher CSF levels of IL-1β and IL-8 were associated with higher CSF levels of t-Tau whereas no correlation was seen between CSF inflammatory markers with p-Tau, α-synuclein or Aβ142 [16]. Our own study revealed that higher CSF levels of FABP, ICAM-1, MMP3, SCF, and TF were associated with higher CSF levels of t-Tau, p181-Tau and α-synuclein in both, males and in females whereas the same association was detected with Aβ142 only on males [72].
There is only 1 longitudinal study with repeatedly taken CSF samples over 2 years available. Here, higher baseline CSF levels of YKL-40 were associated with higher baseline CSF levels of t-Tau, p-Tau and α-synuclein but not with Aβ142. Similarly, an increase in CSF levels of YKL-40 over 2 years was associated with an increase in CSF levels of t-Tau, p-Tau, and α-synuclein [75].
In conclusion, the studies indicate that associations between inflammatory markers with CSF levels of neurodegenerative/PD-biomarkers are primarily seen with t-Tau, p-Tau, and α-synuclein but not with Aβ142.
CONCLUSION
Based on findings of the studies discussed in this review we conclude: (I) The role of inflammation in PD pathogenesis is reflected in increased inflammatory markers in blood and CSF in sporadic PD patients compared to healthy control individuals. (II) Within the group of PD patients, higher blood and CSF levels of inflammatory markers are associated with more severe clinical trajectories of motor and non-motor symptoms cross-sectionally as well as longitudinally. (III) In line with evidence from post-mortem and cell studies, the kind of blood and CSF levels of inflammatory markers indicate a contribution of the innate and adaptive immune system in both CNS and periphery. (IV) Correlations of inflammatory markers in blood and CSF are sparse indicating that they do not reflect one another. This poses a challenge for clinical trials as one has to decide which biomaterial should to be collected for repeated outcome measurements— the more easily accessible blood or CSF by lumbar puncture.
Inflammation is also relevant in the pathogenic mechanisms in other neurodegenerative diseases, among them also Alzheimer’s disease (AD). Interestingly, similar metabolites as reported relevant in PD patients are also elevated in blood and/or CSF in AD patients when compared to healthy controls: CRP, IL-1ß, IL-2, IL-4, IL-6, IL-10, IL-12, IL-18, MCP-1, MCP-3, IL-8, IFN-γ, TGF-β, TNA-α, and YKL-40 [60, 91–95]. Thus, inflammatory processes seem to be somewhat disease-unspecific and triggered by different disease-related protein species such as α-synuclein, Aβ, and tau. These findings in turn offer the chance to accumulate knowledge on inflammation across different neurodegenerative diseases. We acknowledge the following shortcomings: (I) Longitudinal studies with repeatedly collected blood and CSF biosamples in de-novo patients over several years, ideally accompanied by other disease-related biomarkers are missing. This will provide information on how inflammatory profiles evolve over time and in concert with other neurodegenerative/disease-specific protein levels and on the predictive value for the development of disease-related milestones such as dementia. (II) Studies in (genetically) at-risk and prodromal individuals are just beginning to emerge. So far, no robust conclusions can be drawn as longitudinal data in phenoconverters are missing. (III) The type of assays used (single vs. multiplex, platforms), of cytokines assessed and the cohorts’ characteristics (samples size, disease duration) are quite variable. This limits the informative value of each individual marker and comparability across studies. (IV) There is not the one single inflammatory marker in blood and CSF that is associated with clinical trajectories, disease progression and neurodegenerative/disease-specific protein levels. The most frequently reported markers in this context are IL-1β, IL-6, IL-8, MCP-1, and TNF-α (Fig. 1). However, these are also the most investigated ones so that there might be a bias in interpretation. An elegant way might be a composite score out of the 5–10 top markers and thereby stratifying patients by their intra-individual inflammatory load.
ACKNOWLEDGMENTS
KB received funding from The Michael J. Fox Foundation for Parkinson’s Research (MJFF) within the grant “Influence of Inflammatory profiles on PD Phenotype and Progression” and within the PD-Strat project (FKZ 031L0137B) which was supported by the German Federal Ministry of Education and Research (BMBF) in the frame of ERACoSysMed2.
CONFLICT OF INTEREST
The authors declare that the research was conducted in the absence of any commercial or financial relationships that could be construed as a potential conflict of interest.
REFERENCES
[1] | Nalls MA , Pankratz N , Lill CM , Do CB , Hernandez DG , Saad M , DeStefano AL , Kara E , Bras J , Sharma M , Schulte C , Keller MF , Arepalli S , Letson C , Edsall C , Stefansson H , Liu X , Pliner H , Lee JH , Cheng R; , International Parkinson’s Disease Genomics Consortium (IPDGC); Parkinson’s Study Group (PSG) Parkinson’s Research: The Organized GENetics Initiative (PROGENI); 23andMe; GenePD; NeuroGenetics Research Consortium (NGRC); Hussman Institute of Human Genomics (HIHG); Ashkenazi Jewish Dataset Investigator; Cohorts for Health and Aging Research in Genetic Epidemiology (CHARGE); North American Brain Expression Consortium (NABEC); United Kingdom Brain Expression Consortium (UKBEC); Greek Parkinson’s Disease Consortium; Alzheimer Genetic Analysis Group Ikram MA , Ioannidis JP , Hadjigeorgiou GM , Bis JC , Martinez M , Perlmutter JS , Goate A , Marder K , Fiske B , Sutherland M , Xiromerisiou G , Myers RH , Clark LN , Stefansson K , Hardy JA , Heutink P , Chen H , Wood NW , Houlden H , Payami H , Brice A , Scott WK , Gasser T , Bertram L , Eriksson N , Foroud T , Singleton AB ((2014) ) Large-scale meta-analysis of genome-wide association data identifies six new risk loci for Parkinson’s disease. Nat Genet 46: , 989–993. |
[2] | Halliday GM , Stevens CH ((2011) ) Glia: Initiators and progressors of pathology in Parkinson’s disease. Mov Disord 26: , 6–17. |
[3] | Williams-Gray CH , Wijeyekoon R , Yarnall AJ , Lawson RA , Breen DP , Evans JR , Cummins GA , Duncan GW , Khoo TK , Burn DJ , Barker RA , ICICLE-PD study group ((2016) ) Serum immune markers and disease progression in an incident Parkinson’s disease cohort (ICICLE-PD). Mov Disord 31: , 995–1003. |
[4] | Witoelar A , Jansen IE , Wang Y , Desikan RS , Gibbs JR , Blauwendraat C , Thompson WK , Hernandez DG , Djurovic S , Schork AJ , Bettella F , Ellinghaus D , Franke A , Lie BA , McEvoy LK , Karlsen TH , Lesage S , Morris HR , Brice A , Wood NW , Heutink P , Hardy J , Singleton AB , Dale AM , Gasser T , Andreassen OA , Sharma M , International Parkinson’s Disease Genomics Consortium (IPDGC) North American Brain Expression Consortium (NABEC) and United Kingdom Brain Expression Consortium (UKBEC) Investigators ((2017) ) Genome-wide pleiotropy between Parkinson disease and autoimmune diseases. JAMA Neurol 74: , 780–792. |
[5] | Caldi Gomes L , Galhoz A , Jain G , Roser AE , Maass F , Carboni E , Barski E , Lenz C , Lohmann K , Klein C , Bahr M , Fischer A , Menden MP , Lingor P ((2022) ) Multi-omic landscaping of human midbrains identifies disease-relevant molecular targets and pathways in advanced-stage Parkinson’s disease. Clin Transl Med 12: , e692. |
[6] | Nayak A , Salt G , Verma SK , Kishore U ((2015) ) Proteomics approach to identify biomarkers in neurodegenerative diseases. Int Rev Neurobiol 121: , 59–86. |
[7] | Dixit A , Mehta R , Singh AK ((2019) ) Proteomics in human Parkinson’s disease: Present scenario and future directions. Cell Mol Neurobiol 39: , 901–915. |
[8] | Magdalinou NK , Noyce AJ , Pinto R , Lindstrom E , Holmen-Larsson J , Holtta M , Blennow K , Morris HR , Skillback T , Warner TT , Lees AJ , Pike I , Ward M , Zetterberg H , Gobom J ((2017) ) Identification of candidate cerebrospinal fluid biomarkers in parkinsonism using quantitative proteomics. Parkinsonism Relat Disord 37: , 65–71. |
[9] | Harms AS , Ferreira SA , Romero-Ramos M ((2021) ) Periphery and brain, innate and adaptive immunity in Parkinson’s disease. Acta Neuropathol 141: , 527–545. |
[10] | Mogi M , Harada M , Riederer P , Narabayashi H , Fujita K , Nagatsu T ((1994) ) Tumor necrosis factor-alpha (TNF-alpha) increases both in the brain and in the cerebrospinal fluid from parkinsonian patients. Neurosci Lett 165: , 208–210. |
[11] | de Araujo FM , Cuenca-Bermejo L , Fernandez-Villalba E , Costa SL , Silva VDA , Herrero MT (2021) Role of microgliosis and NLRP3 inflammasome in Parkinson’s disease pathogenesis and therapy. Cell Mol Neurobiol , doi: 10.1007/s10571-020-01027-6. |
[12] | Sulzer D , Alcalay RN , Garretti F , Cote L , Kanter E , Agin-Liebes J , Liong C , McMurtrey C , Hildebrand WH , Mao X , Dawson VL , Dawson TM , Oseroff C , Pham J , Sidney J , Dillon MB , Carpenter C , Weiskopf D , Phillips E , Mallal S , Peters B , Frazier A , Lindestam Arlehamn CS , Sette A ((2017) ) T cells from patients with Parkinson’s disease recognize alpha-synuclein peptides. Nature 546: , 656–661. |
[13] | White AJ , Wijeyekoon RS , Scott KM , Gunawardana NP , Hayat S , Solim IH , McMahon HT , Barker RA , Williams-Gray CH ((2018) ) The peripheral inflammatory response to alpha-synuclein and endotoxin in Parkinson’s disease. Front Neurol 9: , 946. |
[14] | Brockmann K , Schulte C , Schneiderhan-Marra N , Apel A , Pont-Sunyer C , Vilas D , Ruiz-Martinez J , Langkamp M , Corvol JC , Cormier F , Knorpp T , Joos TO , Bernard A , Gasser T , Marras C , Schule B , Aasly JO , Foroud T , Marti-Masso JF , Brice A , Tolosa E , Berg D , Maetzler W ((2017) ) Inflammatory profile discriminates clinical subtypes in LRRK2-associated Parkinson’s disease. Eur J Neurol 24: , 427–e426. |
[15] | Kouli A , Camacho M , Allinson K , Williams-Gray CH ((2020) ) Neuroinflammation and protein pathology in Parkinson’s disease dementia. Acta Neuropathol Commun 8: , 211. |
[16] | Wijeyekoon RS , Moore SF , Farrell K , Breen DP , Barker RA , Williams-Gray CH ((2020) ) Cerebrospinal fluid cytokines and neurodegeneration-associated proteins in Parkinson’s disease. Mov Disord 35: , 1062–1066. |
[17] | Wijeyekoon RS , Kronenberg-Versteeg D , Scott KM , Hayat S , Kuan WL , Evans JR , Breen DP , Cummins G , Jones JL , Clatworthy MR , Floto RA , Barker RA , Williams-Gray CH ((2020) ) Peripheral innate immune and bacterial signals relate to clinical heterogeneity in Parkinson’s disease. Brain Behav Immun 87: , 473–488. |
[18] | Lindestam Arlehamn CS , Dhanwani R , Pham J , Kuan R , Frazier A , Rezende Dutra J , Phillips E , Mallal S , Roederer M , Marder KS , Amara AW , Standaert DG , Goldman JG , Litvan I , Peters B , Sulzer D , Sette A ((2020) ) alpha-Synuclein-specific T cell reactivity is associated with preclinical and early Parkinson’s disease. Nat Commun 11: , 1875. |
[19] | Kouli A , Williams-Gray CH ((2020) ) Timing is everything: The T-cell response to alpha-synuclein is maximal in early Parkinson’s. Mov Disord 35: , 1137. |
[20] | Klein SL , Flanagan KL ((2016) ) Sex differences in immune responses. Nat Rev Immunol 16: , 626–638. |
[21] | Ton TG , Jain S , Biggs ML , Thacker EL , Strotmeyer ES , Boudreau R , Newman AB , Longstreth WT Jr , Checkoway H ((2012) ) Markers of inflammation in prevalent and incident Parkinson’s disease in the Cardiovascular Health Study. Parkinsonism Relat Disord 18: , 274–278. |
[22] | Andican G , Konukoglu D , Bozluolcay M , Bayulkem K , Firtiina S , Burcak G ((2012) ) Plasma oxidative and inflammatory markers in patients with idiopathic Parkinson’s disease. Acta Neurol Belg 112: , 155–159. |
[23] | Lindqvist D , Hall S , Surova Y , Nielsen HM , Janelidze S , Brundin L , Hansson O ((2013) ) Cerebrospinal fluid inflammatory markers in Parkinson’s disease–associations with depression, fatigue, and cognitive impairment. Brain Behav Immun 33: , 183–189. |
[24] | Sawada H , Oeda T , Umemura A , Tomita S , Hayashi R , Kohsaka M , Yamamoto K , Sudoh S , Sugiyama H ((2014) ) Subclinical elevation of plasma C-reactive protein and illusions/hallucinations in subjects with Parkinson’s disease: Case-control study. PLoS One 9: , e85886. |
[25] | Qin XY , Zhang SP , Cao C , Loh YP , Cheng Y ((2016) ) Aberrations in peripheral inflammatory cytokine levels in Parkinson disease: A systematic review and meta-analysis. JAMA Neurol 73: , 1316–1324. |
[26] | Wang XM , Zhang YG , Li AL , Long ZH , Wang D , Li XX , Xia JH , Luo SY , Shan YH ((2016) ) Relationship between levels of inflammatory cytokines in the peripheral blood and the severity of depression and anxiety in patients with Parkinson’s disease. Eur Rev Med Pharmacol Sci 20: , 3853–3856. |
[27] | Kim R , Kim HJ , Kim A , Jang M , Kim A , Kim Y , Yoo D , Im JH , Choi JH , Jeon B ((2018) ) Peripheral blood inflammatory markers in early Parkinson’s disease. J Clin Neurosci 58: , 30–33. |
[28] | King E , O’Brien J , Donaghy P , Williams-Gray CH , Lawson RA , Morris CM , Barnett N , Olsen K , Martin-Ruiz C , Burn D , Yarnall AJ , Taylor JP , Duncan G , Khoo TK , Thomas A ((2019) ) Inflammation in mild cognitive impairment due to Parkinson’s disease, Lewy body disease, and Alzheimer’s disease. Int J Geriatr Psychiatry 34: , 1244–1250. |
[29] | King E , O’Brien JT , Donaghy P , Morris C , Barnett N , Olsen K , Martin-Ruiz C , Taylor JP , Thomas AJ ((2019) ) Peripheral inflammationin mild cognitive impairment with possible and probable Lewy bodydisease and Alzheimer’s disease. Int Psychogeriatr 31: , 551–560. |
[30] | Santos-Garcia D , de Deus Fonticoba T , Suarez Castro E , Aneiros Diaz A , Paz Gonzalez JM , Feal Panceiras MJ , Garcia Sancho C , Jesus S , Mir P , Aguilar M , Pastor P , Hernandez Vara J , de Fabregues-Boixar O , Puente V , Crespo Cuevas A , Gonzalez-Aramburu I , Infante J , CarrilloPadilla F , Pueyo M , Escalante S , Bernardo N , Solano B , Cots Foraster A , Martinez-Martin P , Group CS ((2019) ) High ultrasensitive serumC-reactive protein may be related to freezing of gait in Parkinson’sdisease patients. J Neural Transm (Vienna) 126: , 1599–1608. |
[31] | Qiu X , Xiao Y , Wu J , Gan L , Huang Y , Wang J ((2019) ) C-reactive protein and risk of Parkinson’s disease: A systematic review and meta-analysis. Front Neurol 10: , 384. |
[32] | Baran A , Bulut M , Kaya MC , Demirpence O , Sevim B , Akil E , Varol S ((2019) ) High-sensitivity C-reactive protein and high mobility group box-1 levels in Parkinson’s disease. Neurol Sci 40: , 167–173. |
[33] | Jin H , Gu HY , Mao CJ , Chen J , Liu CF ((2020) ) Association of inflammatory factors and aging in Parkinson’s disease. Neurosci Lett 736: , 135259. |
[34] | Dommershuijsen LJ , Ruiter R , Erler NS , Rizopoulos D , Ikram MA , Ikram MK ((2022) ) Peripheral immune cell numbers and C-reactive protein in Parkinson’s disease: Results from a population-based study. J Parkinsons Dis 12: , 667–678. |
[35] | Koziorowski D , Tomasiuk R , Szlufik S , Friedman A ((2012) ) Inflammatory cytokines and NT-proCNP in Parkinson’s disease patients. Cytokine 60: , 762–766. |
[36] | Hu Y , Yu SY , Zuo LJ , Cao CJ , Wang F , Chen ZJ , Du Y , Lian TH , Wang YJ , Chan P , Chen SD , Wang XM , Zhang W ((2015) ) Parkinson disease with REM sleep behavior disorder: Features, alpha-synuclein, and inflammation. Neurology 84: , 888–894. |
[37] | Milyukhina IV , Karpenko MN , Klimenko VM ((2015) ) [Clinical parametersand the level of certain cytokines in blood and cerebrospinal fluidof patients with Parkinson’s disease]. Klin Med (Mosk) 93: , 51–55. |
[38] | Brockmann K , Apel A , Schulte C , Schneiderhan-Marra N , Pont-Sunyer C , Vilas D , Ruiz-Martinez J , Langkamp M , Corvol JC , Cormier F , Knorpp T , Joos TO , Gasser T , Schule B , Aasly JO , Foroud T , Marti-Masso JF , Brice A , Tolosa E , Marras C , Berg D , Maetzler W ((2016) ) Inflammatory profile in LRRK2-associated prodromal and clinical PD. J Neuroinflammation 13: , 122. |
[39] | Karpenko MN , Vasilishina AA , Gromova EA , Muruzheva ZM , Miliukhina IV , Bernadotte A ((2018) ) Interleukin-1beta, interleukin-1 receptorantagonist, interleukin-6, interleukin-10, and tumor necrosisfactor-alpha levels in CSF and serum in relation to the clinicaldiversity of Parkinson’s disease. Cell Immunol 327: , 77–82. |
[40] | Rocha NP , Assis F , Scalzo PL , Vieira ELM , Barbosa IG , de Souza MS , Christo PP , Reis HJ , Teixeira AL ((2018) ) Reduced activated T lymphocytes (CD4+CD25+) and plasma levels of cytokines in Parkinson’s disease. Mol Neurobiol 55: , 1488–1497. |
[41] | Alrafiah A , Al-Ofi E , Obaid MT , Alsomali N ((2019) ) Assessment of thelevels of level of biomarkers of bone matrix glycoproteins andinflammatory cytokines from Saudi Parkinson patients. BiomedRes Int 2019: , 2690205. |
[42] | Lian TH , Guo P , Zuo LJ , Hu Y , Yu SY , Yu QJ , Jin Z , Wang RD , Li LX , Zhang W ((2019) ) Tremor-dominant in Parkinson disease: The relevance to iron metabolism and inflammation. Front Neurosci 13: , 255. |
[43] | Chatterjee K , Roy A , Banerjee R , Choudhury S , Mondal B , Halder S , Basu P , Shubham S , Dey S , Kumar H ((2020) ) Inflammasome and alpha-synuclein in Parkinson’s disease: A cross-sectional study. J Neuroimmunol 338: , 577089. |
[44] | Fan Z , Pan YT , Zhang ZY , Yang H , Yu SY , Zheng Y , Ma JH , Wang XM ((2020) ) Systemic activation of NLRP3 inflammasome and plasma alpha-synuclein levels are correlated with motor severity and progression in Parkinson’s disease. J Neuroinflammation 17: , 11. |
[45] | Mahlknecht P , Stemberger S , Sprenger F , Rainer J , Hametner E , Kirchmair R , Grabmer C , Scherfler C , Wenning GK , Seppi K , Poewe W , Reindl M ((2012) ) An antibody microarray analysis of serum cytokinesin neurodegenerative Parkinsonian syndromes. ProteomeSci 10: , 71. |
[46] | Schroder JB , Pawlowski M , Meyer Zu Horste G , Gross CC , Wiendl H , Meuth SG , Ruck T , Warnecke T ((2018) ) Immune cell activation in thecerebrospinal fluid of patients with Parkinson’s disease. FrontNeurol 9: , 1081. |
[47] | Tang P , Chong L , Li X , Liu Y , Liu P , Hou C , Li R ((2014) ) Correlation between serum RANTES levels and the severity of Parkinson’s disease. Oxid Med Cell Longev 2014: , 208408. |
[48] | Delgado-Alvarado M , Gago B , Gorostidi A , Jimenez-Urbieta H , Dacosta-Aguayo R , Navalpotro-Gomez I , Ruiz-Martinez J , Bergareche A , Marti-Masso JF , Martinez-Lage P , Izagirre A , Rodriguez-Oroz MC ((2017) ) Tau/alpha-synuclein ratio and inflammatory proteins in Parkinson’s disease: An exploratory study. Mov Disord 32: , 1066–1073. |
[49] | Kwiatek-Majkusiak J , Geremek M , Koziorowski D , Tomasiuk R , Szlufik S , Friedman A ((2020) ) Serum levels of hepcidin and interleukin 6 in Parkinson’s disease. Acta Neurobiol Exp (Wars) 80: , 297–304. |
[50] | Miliukhina IV , Usenko TS , Senkevich KA , Nikolaev MA , Timofeeva AA , Agapova EA , Semenov AV , Lubimova NE , Totolyan AA , Pchelina SN ((2020) ) Plasma cytokines profile in patients with Parkinson’s diseaseassociated with mutations in GBA gene. Bull Exp Biol Med 168: , 423–426. |
[51] | Gupta V , Garg RK , Khattri S ((2016) ) Levels of IL-8 and TNF-alpha decrease in Parkinson’s disease. Neurol Res 38: , 98–102. |
[52] | Calvani R , Picca A , Landi G , Marini F , Biancolillo A , Coelho-Junior HJ , Gervasoni J , Persichilli S , Primiano A , Arcidiacono A , Urbani A , Bossola M , Bentivoglio AR , Cesari M , Bernabei R , Monaco MRL , Marzetti E ((2020) ) A novel multi-marker discovery approach identifies new serum biomarkers for Parkinson’s disease in older people: An EXosomes in PArkiNson Disease (EXPAND) ancillary study. Geroscience 42: , 1323–1334. |
[53] | Lin CH , Chen CC , Chiang HL , Liou JM , Chang CM , Lu TP , Chuang EY , Tai YC , Cheng C , Lin HY , Wu MS ((2019) ) Altered gut microbiota and inflammatory cytokine responses in patients with Parkinson’s disease. J Neuroinflammation 16: , 129. |
[54] | Rathnayake D , Chang T , Udagama P ((2019) ) Selected serum cytokines andnitric oxide as potential multi-marker biosignature panels forParkinson disease of varying durations: A case-control study. BMC Neurol 19: , 56. |
[55] | Usenko TS , Nikolaev MA , Miliukhina IV , Bezrukova AI , Senkevich KA , Gomzyakova NA , Beltceva YA , Zalutskaya NM , Gracheva EV , Timofeeva AA , Petrova OA , Semenov AV , Lubimova NE , Totolyan AA , Pchelina SN ((2020) ) Plasma cytokine profile in synucleinophaties with dementia. J Clin Neurosci 78: , 323–326. |
[56] | Eidson LN , Kannarkat GT , Barnum CJ , Chang J , Chung J , Caspell-Garcia C , Taylor P , Mollenhauer B , Schlossmacher MG , Ereshefsky L , Yen M , Kopil C , Frasier M , Marek K , Hertzberg VS , Tansey MG ((2017) ) Candidate inflammatory biomarkers display unique relationships withalpha-synuclein and correlate with measures of disease severity insubjects with Parkinson’s disease. J Neuroinflammation 14: , 164. |
[57] | Martin-Ruiz C , Williams-Gray CH , Yarnall AJ , Boucher JJ , Lawson RA , Wijeyekoon RS , Barker RA , Kolenda C , Parker C , Burn DJ , Von Zglinicki T , Saretzki G ((2020) ) Senescence and Inflammatory Markers for Predicting Clinical Progression in Parkinson’s Disease: The ICICLE-PD Study. J Parkinsons Dis 10: , 193–206. |
[58] | Csencsits-Smith K , Suescun J , Li K , Luo S , Bick DL , Schiess M ((2016) ) Serum lymphocyte-associated cytokine concentrations change more rapidly over time in multiple system atrophy compared to Parkinson disease. Neuroimmunomodulation 23: , 301–308. |
[59] | Kouchaki E , Kakhaki RD , Tamtaji OR , Dadgostar E , Behnam M , Nikoueinejad H , Akbari H ((2018) ) Increased serum levels of TNF-alpha and decreased serum levels of IL-27 in patients with Parkinson disease and their correlation with disease severity. Clin Neurol Neurosurg 166: , 76–79. |
[60] | Chen X , Hu Y , Cao Z , Liu Q , Cheng Y ((2018) ) Cerebrospinal fluid inflammatory cytokine aberrations in Alzheimer’s disease, Parkinson’s disease and amyotrophic lateral sclerosis: A systematic review and meta-analysis. Front Immunol 9: , 2122. |
[61] | Iwaoka K , Otsuka C , Maeda T , Yamahara K , Kato K , Takahashi K , Takahashi K , Terayama Y ((2020) ) Impaired metabolism of kynurenine and its metabolites in CSF of Parkinson’s disease. Neurosci Lett 714: , 134576. |
[62] | Yu SY , Zuo LJ , Wang F , Chen ZJ , Hu Y , Wang YJ , Wang XM , Zhang W ((2014) ) Potential biomarkers relating pathological proteins, neuroinflammatory factors and free radicals in PD patients with cognitive impairment: A cross-sectional study. BMC Neurol 14: , 113. |
[63] | Hall S , Janelidze S , Surova Y , Widner H , Zetterberg H , Hansson O ((2018) ) Cerebrospinal fluid concentrations of inflammatory markers in Parkinson’s disease and atypical parkinsonian disorders. Sci Rep 8: , 13276. |
[64] | Hu WT , Howell JC , Ozturk T , Gangishetti U , Kollhoff AL , Hatcher-Martin JM , Anderson AM , Tyor WR ((2019) ) CSF cytokines in aging, multiple sclerosis, and dementia. Front Immunol 10: , 480. |
[65] | Santaella A , Kuiperij HB , van Rumund A , Esselink RAJ , van Gool AJ , Bloem BR , Verbeek MM ((2020) ) Inflammation biomarker discovery in Parkinson’s disease and atypical parkinsonisms. BMC Neurol 20: , 26. |
[66] | Dzamko N , Rowe DB , Halliday GM ((2016) ) Increased peripheral inflammation in asymptomatic leucine-rich repeat kinase 2 mutation carriers. Mov Disord 31: , 889–897. |
[67] | Thaler A , Omer N , Giladi N , Gurevich T , Bar-Shira A , Gana-Weisz M , Goldstein O , Kestenbaum M , Shirvan JC , Cedarbaum JM , Orr-Urtreger A , Regev K , Shenhar-Tsarfaty S , Mirelman A ((2021) ) Mutations in GBA and LRRK2 are not associated with increased inflammatory markers. J Parkinsons Dis 11: , 1285–1296. |
[68] | Majbour NK , Aasly JO , Hustad E , Thomas MA , Vaikath NN , Elkum N , van de Berg WDJ , Tokuda T , Mollenhauer B , Berendse HW , El-Agnaf OMA ((2020) ) CSF total and oligomeric alpha-Synuclein along with TNF-alpha as risk biomarkers for Parkinson’s disease: A study in LRRK2 mutation carriers. Transl Neurodegener 9: , 15. |
[69] | Galper J , Balwani M , Fahn S , Waters C , Krohn L , Gan-Or Z , Dzamko N , Alcalay RN ((2021) ) Cytokines and Gaucher biomarkers in glucocerebrosidase carriers with and without Parkinson disease. Mov Disord 36: , 1451–1455. |
[70] | Sliter DA , Martinez J , Hao L , Chen X , Sun N , Fischer TD , Burman JL , Li Y , Zhang Z , Narendra DP , Cai H , Borsche M , Klein C , Youle RJ ((2018) ) Parkin and PINK1 mitigate STING-induced inflammation. Nature 561: , 258–262. |
[71] | Borsche M , Konig IR , Delcambre S , Petrucci S , Balck A , Bruggemann N , Zimprich A , Wasner K , Pereira SL , Avenali M , Deuschle C , Badanjak K , Ghelfi J , Gasser T , Kasten M , Rosenstiel P , Lohmann K , Brockmann K , Valente EM , Youle RJ , Grunewald A , Klein C ((2020) ) Mitochondrial damage-associated inflammation highlights biomarkers in PRKN/PINK1 parkinsonism. Brain 143: , 3041–3051. |
[72] | Lerche S , Zimmermann M , Wurster I , Roeben B , Fries FL , Deuschle C , Waniek K , Lachmann I , Gasser T , Jakobi M , Joos TO , Schneiderhan-Marra N , Brockmann K ((2022) ) CSF and serum levels of inflammatory markers in PD: Sparse correlation, sex differences and association with neurodegenerative biomarkers. Front Neurol 13: , 834580. |
[73] | Sawada H , Oeda T , Umemura A , Tomita S , Kohsaka M , Park K , Yamamoto K , Sugiyama H ((2015) ) Baseline C-reactive protein levels and life prognosis in Parkinson disease. PLoS One 10: , e0134118. |
[74] | Vesely B , Dufek M , Thon V , Brozman M , Kiralova S , Halaszova T , Koritakova E , Rektor I ((2018) ) Interleukin 6 and complement serum level study in Parkinson’s disease. J Neural Transm (Vienna) 125: , 875–881. |
[75] | Hall S , Surova Y , Ohrfelt A , Swedish Bio FS , Blennow K , Zetterberg H , Hansson O ((2016) ) Longitudinal measurements of cerebrospinal fluid biomarkers in Parkinson’s disease. Mov Disord 31: , 898–905. |
[76] | Green HF , Khosousi S , Svenningsson P ((2019) ) Plasma IL-6 and IL-17A correlate with severity of motor and non-motor symptoms in Parkinson’s disease. J Parkinsons Dis 9: , 705–709. |
[77] | Li S , Zhang Q , Gao Y , Nie K , Liang Y , Zhang Y , Wang L ((2021) ) Serum folate, vitamin B12 levels, and systemic immune-inflammation index correlate with motor performance in Parkinson’s disease: A cross-sectional study. Front Neurol 12: , 665075. |
[78] | Gupta M , Paliwal VK , Babu GN ((2022) ) Serum fractalkine and 3-nitrotyrosine levels correlate with disease severity in Parkinson’s disease: A pilot study. Metab Brain Dis 37: , 209–217. |
[79] | Carvalho DZ , Schonwald SV , Schumacher-Schuh AF , Braga CW , Souza DO , Oses JP , Donis KC , Rieder CR ((2015) ) Overnight S100B in Parkinson’s Disease: A glimpse into sleep-related neuroinflammation. Neurosci Lett 608: , 57–63. |
[80] | Perner C , Perner F , Gaur N , Zimmermann S , Witte OW , Heidel FH , Grosskreutz J , Prell T ((2019) ) Plasma VCAM1 levels correlate with disease severity in Parkinson’s disease. J Neuroinflammation 16: , 94. |
[81] | Umemura A , Oeda T , Yamamoto K , Tomita S , Kohsaka M , Park K , SugiyamaH , Sawada H ((2015) ) Baseline plasma C-reactive protein concentrationsand motor prognosis in Parkinson disease. PLoS One 10: , e0136722. |
[82] | Yilmaz R , Strafella AP , Bernard A , Schulte C , van den Heuvel L , Schneiderhan-Marra N , Knorpp T , Joos TO , Leypoldt F , Geritz J , Hansen C , Heinzel S , Apel A , Gasser T , Lang AE , Berg D , Maetzler W , Marras C ((2018) ) Serum inflammatory profile for the discrimination ofclinical subtypes in Parkinson’s disease. Front Neurol 9: , 1123. |
[83] | Rocha NP , Teixeira AL , Scalzo PL , Barbosa IG , de Sousa MS , Morato IB , Vieira EL , Christo PP , Palotas A , Reis HJ ((2014) ) Plasma levelsof soluble tumor necrosis factor receptors are associated withcognitive performance in Parkinson’s disease. Mov Disord 29: , 527–531. |
[84] | Mollenhauer B , Zimmermann J , Sixel-Doring F , Focke NK , Wicke T , Ebentheuer J , Schaumburg M , Lang E , Friede T , Trenkwalder C , DeNoPa Study Group ((2019) ) Baseline predictors for progression 4 years after Parkinson’s disease diagnosis in the De Novo Parkinson Cohort (DeNoPa). Mov Disord 34: , 67–77. |
[85] | Vesely B , Koritakova E , Bohnen NI , Viszlayova D , Kiralova S , Valkovic P , Kurca E , Rektor I ((2019) ) The contribution of cerebrovascular risk factors, metabolic and inflammatory changes to cognitive decline in Parkinson’s disease: Preliminary observations. J Neural Transm (Vienna) 126: , 1303–1312. |
[86] | Dufek M , Rektorova I , Thon V , Lokaj J , Rektor I ((2015) ) Interleukin-6 may contribute to mortality in Parkinson’s disease patients: A 4-year prospective study. Parkinsons Dis 2015: , 898192. |
[87] | Sanjari Moghaddam H , Valitabar Z , Ashraf-Ganjouei A , Mojtahed Zadeh M , Ghazi Sherbaf F , Aarabi MH ((2018) ) Cerebrospinal fluid C-reactive protein in Parkinson’s disease: Associations with motor and non-motor symptoms. Neuromolecular Med 20: , 376–385. |
[88] | Santaella A , Kuiperij HB , van Rumund A , Esselink RAJ , van Gool AJ , Bloem BR , Verbeek MM ((2020) ) Cerebrospinal factaluid monocyte chemoattrnt protein 1 correlates with progression of Parkinson’s disease. NPJ Parkinsons Dis 6: , 21. |
[89] | Hatcher-Martin JM , McKay JL , Pybus AF , Sommerfeld B , Howell JC , Goldstein FC , Wood L , Hu WT , Factor SA ((2021) ) Cerebrospinal fluid biomarkers in Parkinson’s disease with freezing of gait: An exploratory analysis. NPJ Parkinsons Dis 7: , 105. |
[90] | Wennstrom M , Surova Y , Hall S , Nilsson C , Minthon L , Hansson O , Nielsen HM ((2015) ) The inflammatory marker YKL-40 is elevated in cerebrospinal fluid from patients with Alzheimer’s but not Parkinson’s disease or dementia with Lewy bodies. PLoS One 10: , e0135458. |
[91] | Park JC , Han SH , Mook-Jung I ((2020) ) Peripheral inflammatory biomarkers in Alzheimer’s disease: A brief review. BMB Rep 53: , 10–19. |
[92] | Su C , Zhao K , Xia H , Xu Y ((2019) ) Peripheral inflammatory biomarkers in Alzheimer’s disease and mild cognitive impairment: A systematic review and meta-analysis. Psychogeriatrics 19: , 300–309. |
[93] | Xie J , Van Hoecke L , Vandenbroucke RE ((2021) ) The impact of systemic inflammation on Alzheimer’s disease pathology. Front Immunol 12: , 796867. |
[94] | Dhapola R , Hota SS , Sarma P , Bhattacharyya A , Medhi B , Reddy DH ((2021) ) Recent advances in molecular pathways and therapeutic implications targeting neuroinflammation for Alzheimer’s disease. Inflammopharmacology 29: , 1669–1681. |
[95] | de Oliveira J , Kucharska E , Garcez ML , Rodrigues MS , Quevedo J , Moreno-Gonzalez I , Budni J ((2021) ) Inflammatory cascade in Alzheimer’s disease pathogenesis: A review of experimental findings. Cells 10: , 2581. |
[96] | Sun C , Yu W , Zhao Z , Song C , Liu Y , Jia G , Wang X , Liu Y ((2019) ) Peripheral humoral immune response is associated with the non-motor symptoms of Parkinson’s disease. Front Neurosci 13: , 1057. |
[97] | Bagheri V , Khorramdelazad H , Hassanshahi G , Moghadam-Ahmadi A , Vakilian A ((2018) ) CXCL12 and CXCR4 in the peripheral blood of patients with Parkinson’s disease. Neuroimmunomodulation 25: , 201–205. |
[98] | Rahnemayan S , Mirghafourvand M , Fathalizadeh A , Faramarzi E , Reyhanifard A , Mahmoodpoor A , Sanaie S ((2021) ) Leptin levels in patients with Parkinson’s disease: A systematic review and meta-analysis. Clin Nutr ESPEN 41: , 104–109. |
[99] | Roy A , Choudhury S , Banerjee R , Basu P , Kumar H ((2021) ) Soluble LAG-3 and Toll-interacting protein: Novel upstream neuro-inflammatory markers in Parkinson’s disease.Parkinsonism Relat Disord 91: , 121–123. |
[100] | Dumitrescu L , Marta D , Danau A , Lefter A , Tulba D , Cozma L , Manole E , Gherghiceanu M , Ceafalan LC , Popescu BO ((2021) ) Serum and fecalmarkers of intestinal inflammation and intestinal barrierpermeability are elevated in Parkinson’s disease. FrontNeurosci 15: , 689723. |
[101] | Sathe K , Maetzler W , Lang JD , Mounsey RB , Fleckenstein C , Martin HL , Schulte C , Mustafa S , Synofzik M , Vukovic Z , Itohara S , Berg D , Teismann P ((2012) ) S100B is increased in Parkinson’s disease andablation protects against MPTP-induced toxicity through the RAGE andTNF-alpha pathway. Brain 135: , 3336–3347. |
[102] | Herlofson K , Heijnen CJ , Lange J , Alves G , Tysnes OB , Friedman JH , Fagundes CP ((2018) ) Inflammation and fatigue in early, untreated Parkinson’s disease. Acta Neurol Scand 138: , 394–399. |
[103] | Pereira JR , Santos LVD , Santos RMS , Campos ALF , Pimenta AL , de Oliveira MS , Bacheti GG , Rocha NP , Teixeira AL , Christo PP , Scalzo PL ((2016) ) IL-6 serum levels are elevated in Parkinson’s disease patients with fatigue compared to patients without fatigue. J Neurol Sci 370: , 153–156. |
[104] | Karpenko MN , Vasilishina AA , Gromova EA , Muruzheva ZM , Bernadotte A ((2018) ) Corrigendum to “Interleukin-1beta, interleukin-1 receptor antagonist, interleukin-6, interleukin-10, and tumor necrosis factor-alpha levels in CSF and serum in relation to the clinical diversity of Parkinson’s disease” [Cell. Immunol. 327 (2018) 77-82]. Cell Immunol 334: , 99. |