Evaluating the Use of Digital Biomarkers to Test Treatment Effects on Cognition and Movement in Patients with Lewy Body Dementia
Abstract
Background:
PRESENCE was a Phase 2 trial assessing mevidalen for symptomatic treatment of Lewy body dementia (LBD). Participants received daily doses (10, 30, or 75 mg) of mevidalen (LY3154207) or placebo for 12 weeks.
Objective:
To evaluate if frequent cognitive and motor tests using an iPad app and wrist-worn actigraphy to track activity and sleep could detect mevidalen treatment effects in LBD.
Methods:
Of 340 participants enrolled in PRESENCE, 238 wore actigraphy for three 2-week periods: pre-, during, and post-intervention. A subset of participants (n = 160) enrolled in a sub-study using an iPad trial app with 3 tests: digital symbol substitution (DSST), spatial working memory (SWM), and finger-tapping. Compliance was defined as daily test completion or watch-wearing ≥23 h/day. Change from baseline to week 12 (app) or week 8 (actigraphy) was used to assess treatment effects using Mixed Model Repeated Measures analysis. Pearson correlations between sensor-derived features and clinical endpoints were assessed.
Results:
Actigraphy and trial app compliance was > 90% and > 60%, respectively. At baseline, daytime sleep positively correlated with Epworth Sleepiness Scale score (p < 0.01). Physical activity correlated with improvement on Movement Disorder Society –Unified Parkinson Disease Rating Scale (MDS-UPDRS) part II (p < 0.001). Better scores of DSST and SWM correlated with lower Alzheimer Disease Assessment Scale –Cognitive 13-Item Scale (ADAS-Cog13) (p < 0.001). Mevidalen treatment (30 mg) improved SWM (p < 0.01), while dose-dependent decreases in daytime sleep (10 mg: p < 0.01, 30 mg: p < 0.05, 75 mg: p < 0.001), and an increase in walking minutes (75 mg dose: p < 0.001) were observed, returning to baseline post-intervention.
Conclusion:
Devices used in the LBD population achieved adequate compliance and digital metrics detected statistically significant treatment effects.
INTRODUCTION
Lewy body dementia (LBD), which encompasses both Parkinson’s disease dementia (PDD) and dementia with Lewy bodies (DLB), is a neurodegenerative disorder that predominantly affects dopaminergic and cholinergic neurons [1, 2]. The formation and propagation of Lewy bodies accompanied by progressive neurodegeneration lead to an overlap of motor and cognitive impairments in LBD and a resultant range of symptoms, including dementia, parkinsonism, and behavioral and sleep disturbances [2–4]. Given the multifaceted nature of LBD, it is a challenging disease to manage therapeutically, and balancing symptomatic efficacy can often lead to worsening of other symptoms or an increased burden of side effects [5–7].
Utilizing wearable sensors or applications to assess motor function, cognition, and behavior opens the possibility of including these to develop digital biomarkers and apply them in both clinical trial and community settings. These devices have shown great promise in discriminating individuals with Parkinson’s disease (PD) from healthy controls and measuring PD symptom severity [8] as well as discriminating between healthy individuals and individuals at risk of progressing to dementia within 3 years [9]. Within clinical trials, digital devices allow for continuous and objective data monitoring for several weeks or months at a time, and once validated could potentially alleviate reliance on clinical scales or questionnaires that depend on a patient’s ability to recall information or complete diaries [10, 11]. In addition, digital devices may be less disruptive to a patient’s lifestyle or normal behavior than traditional clinical visits, less influenced by “white coat syndrome”, and, with adequate compliance, allow for long-term symptom monitoring or treatment tracking in patients’ natural environments [12, 13].
Mevidalen (LY3154207) is a selective D1 receptor positive allosteric modulator (D1PAM) which enhances the affinity of the D1 receptor for endogenous and exogenous dopamine thereby amplifying its response to dopamine [14, 15]. Because of its novel mechanism, mevidalen has the potential to improve cognitive performance by enhancing frontal dopaminergic neurotransmission, activating cortical neurons, and enhancing synaptic plasticity and D1-mediated enhanced acetylcholine release and is hypothesized to also improve motor function and daytime sleepiness [15, 16]. Mevidalen was recently assessed in a Phase 2 randomized, placebo-controlled trial (PRESENCE; NCT03305809) designed to test the safety and efficacy of mevidalen in patients with mild-to-moderate LBD. In PRESENCE, mevidalen did not meet its primary endpoint of improvement in cognition measured by change in the Continuity of Attention (CoA) composite score of the Cognitive Drug Research Computerized Cognition Battery (CDR-CCB) from baseline to Week 12; however, it did produce robust global improvement as measured by the Alzheimer’s Disease Cooperative Study-Clinical Global Impression of Change (ADCS-CGIC) [17]. Mevidalen treatment also resulted in statistically significant and clinically meaningful benefits in motor function on top of stable treatment while improving or not worsening non-motor complications commonly reported in current therapies [17].
In the PRESENCE trial, which assessed the impact of mevidalen for the symptomatic treatment of LBD, participants were asked to wear an actigraphy watch and were also invited to partake in a sub-study using an iPad trial app. This work aimed to evaluate whether the wrist-worn actigraphy watch for continuous activity and sleep tracking and frequent cognitive digital symbol substitution (DSST), spatial working memory (SWM), and finger tapping tests using the iPad app could detect mevidalen treatment effects in patients with mild-to-moderate dementia due to LBD.
METHODS
Patient population and trial design
PRESENCE (NCT03305809: ClinicalTrials.gov) was a Phase 2, multicenter, randomized, double-blind, parallel-group, placebo-controlled, 12-week clinical trial assessing mevidalen (LY3154207) for symptomatic treatment of LBD (PDD and DLB). Participants (n = 344) were randomized 1:1:1:1 to receive daily doses (10, 30, or 75 mg) of mevidalen or placebo. Digital devices were used in a subpopulation (all US-based and English-speaking participants (n = 238) for actigraphy and as an optional sub-study for US-based participants (n = 160) for the iPad app) to evaluate the cognitive, motor, activity, and sleep effects of mevidalen. The trial design can be visualized in Fig. 1. The study included a Screening Period of 7 to 14 days (Visit 1–2), a Pre-intervention Period of ≥11 days and ≤17 days (Visit 2–3), a 12-week Intervention Period (Visits 3–11), and a 14-day Safety Follow-up period that included a 2-week follow-up visit (Visit 801). Actigraphy participants wore the actigraphy devices continuously for three separate 2-week periods: pre-intervention, during intervention, post- intervention. iPad trial app participants performed 2 out of 3 tests (DSST, SWM, and finger tapping) on an alternating basis twice daily during pre- and post- intervention, and once daily during intervention.
Fig. 1
Schematic showing the PRESENCE trial period and the use of digital devices. Wk, week.

The main inclusion/exclusion criteria have been described in full elsewhere [17]. Enrolled participants were aged 40 to 85 years (inclusive) with dementia, as defined by a decline in cognitive function and with a Montreal Cognitive Assessment (MoCA) score of 10–23 (inclusive) [18]. Enrolled participants met the diagnostic criteria for PD [19] or DLB [20] with categorized Modified Hoehn and Yahr Stages 0–4 [21].
Oversight
The trial was conducted at 69 sites in the US including Puerto Rico, and Canada. The trial received approval from the relevant ethics committees and participants provided written informed consent for trial participation. The trial was conducted following the Declaration of Helsinki and the Council for International Organizations of Medical Sciences International Ethical Guidelines. The sponsor held regularly scheduled trial-level safety reviews on blinded data. An internal assessment committee (IAC) held unblinded safety data reviews to ensure the continuing safety of enrolled participants.
Digital biomarkers
Actigraphy
The actigraphy device (AX3-axivity device: https://axivity.com/product/ax3), uses an accelerometer to measure activity and, similar to a watch, was placed on the non-dominant wrist of eligible participants (US-based and English-speaking) at specified visits for a 2-week period until the subsequent clinic visits to allow continuous tracking of sleep and activity during these periods. As shown in Fig. 1, the site provided the device to participants at Visits 2, 8, and 11, consistent with pre-intervention (baseline), during intervention (steady-state), post-intervention (off-drug) time points; following 14 days of wearing the device, participants returned it to the site at the regularly scheduled visit. Daytime walking [22] was the primary endpoint for physical activity testing for treatment effect using bi-weekly data points. Activity was measured at a sampling rate of 50 Hz, and raw trial axial acceleration signal was converted using ENMOtrunc (Euclidean norm minus one truncated to zero) approach to average acceleration (in milligravity) [23] and aggregated for hourly and daily outcomes. Daytime sleep was pre-specified as the primary measure for sleep. Sleep was measured by using an actigraphy-based algorithm to measure inactivity as a function of sleep [24, 25]. The feasibility of using actigraphy to evaluate the effects of mevidalen on various sleep parameters and daytime activity was explored.
iPad Trial app
An iPad configured with the Lilly Trial application (Lilly Trial app) was provided to participants who consented to this sub-study, who were prompted to complete twice-daily assessments starting the day following Visit 2 for 2 weeks (until Visit 3), once daily throughout the dosing period (Visit 3 until Visit 11), and twice-daily for 2 weeks after the conclusion of the intervention period (until Visit 801) as shown in Fig. 1. The purposes of the Lilly Trial app were to assess the feasibility of frequently conducting cognitive and motor function tests under real-world conditions without supervision and to measure the effect of mevidalen on the motor and cognitive outcomes in a subset of participants. The app used a series of tests that triggered iPad sensors to collect timestamped data from the participants. These tests included digital symbol substitution (DSST), spatial working memory (SWM) [26], and finger-tapping tests [8]. DSST measures a participant’s ability to shift attention quickly and precisely from one location to another, as well as measuring motor skill. In this test, participants were asked to match symbols to their corresponding digits. Nine pairs of digit-symbols were presented on the top of the screen for reference, and at the center of the screen, a set of numbers and blank boxes are presented. Participants were given 60 s to pair as many symbols to corresponding digits as possible by dragging a symbol from a pool at the bottom of the screen into the blank box. The primary performance measure was the number of correct substitutions or pairings made within the time limit. SWM measures a participant’s ability to hold information about an object’s location in short-term memory [27]. Participants viewed brief displays containing two or three dots and were asked to remember the locations of the dots. After a short delay, a probe appeared at one location on the screen and participants were asked to determine whether or not the probe appeared at the location of one of the dots presented previously. Both accuracy and reaction time were recorded. Overall accuracy was the primary measure of performance. Finger tapping was used as a digital test of hand dexterity, where a person was instructed to alternately tap two buttons on a smart device screen using the index and middle fingers. The test recorded the coordinates and timestamp of each tap, and the total number of taps (pre-specified as the primary measure for tapping) and inter tap interval were computed to assess speed, strength and consistency during the test [28].
Compliance
To assess the feasibility of using digital measurements in a patient population, adequate adherence to the use of the app and/or wearing of devices to assess digital measures was examined. Here, compliance for the app was defined as daily test completion versus required 4 daily tests (two sessions with two tests per session) in pre- and post- intervention, and two daily tests (one session with two tests) during intervention. Compliance for actigraphy was defined as watch-wearing ≥23 h/day.
Clinical measurements
A full description of clinical assessments emp-loyed in PRESENCE has been described in detail elsewhere [17]. Those relevant to the analysis in the digital biomarkers sub-study are described below.
Cognition
To assess the effect of mevidalen on cognition, the 13-item Alzheimer’s Disease Assessment Scale-Cognitive subscale (ADAS-Cog13) score was used. The ADAS-Cog13 is composed of the original 11-item ADAS-Cog, which measures memory, language, and praxis but additionally includes Delayed Recall (episodic memory) and Number Cancellation (attention) items [29]. A higher ADAS-Cog13 score indicates greater cognitive impairment.
Motor
The effect of mevidalen on PD severity was evaluated using the Movement Disorder Society–owned rating scales - Unified Parkinson Disease Rating Scale (MDS-UPDRS) [30]. The scale is composed of four parts: MDS-UPDRS Part I (non-motor aspects of experiences of daily living), Part II (motor aspects of experience of daily living), Part III (motor examination), and Part IV (motor complications). The MDS-UPDRS total score is a sum of Parts I, II, and III. A higher score indicates greater severity or impairment.
Sleep
The effect of mevidalen on daytime sleepiness was evaluated using the Epworth Sleepiness Scale (ESS), a widely used scale as a subjective measure of a participant’s sleepiness. Scores range from 0 to 24, with scores ≥10 indicating excessive daytime sleepiness [31].
Table 1
Baseline demographics and characteristics of participants at baseline
Actigraphy | Placebo (N = 63) | 10 mg LY3154207 (N = 64) | 30 mg LY3154207 (N = 59) | 75 mg LY3154207 (N = 52) | Overall p |
Male, % | 81.0 | 86.9 | 86.2 | 82.7 | 0.783 |
Age | 73.2 (7.1) | 72.7 (6.9) | 72.4 (6.7) | 73.4 (4.3) | 0.837 |
AChEI use, % | 47.6 | 42.6 | 53.5 | 50.0 | 0.693 |
Daytime sleep (min) | 85.3 (20.5) | 88.4 (58.0) | 104.7 (63.5) | 88.7 (51.7) | 0.232 |
Nighttime sleep (h) | 7.0 (1.2) | 7.1 (1.3) | 7.4 (1.3) | 7.0 (1.3) | 0.292 |
Daytime walking (h) | 0.9 (0.7) | 0.9 (0.6) | 0.8 (0.5) | 1.0 (0.7) | 0.525 |
Average acceleration (m-g) | 18.1 (5.7) | 18.5 (8.0) | 17.7 (7.7) | 18.0 (7.7) | 0.942 |
Trial app | Placebo (N = 38) | 10 mg LY3154207 (N = 43) | 30 mg LY3154207 (N = 40) | 75 mg LY3154207 (N = 39) | Overall p |
Male, % | 89.5 | 83.7 | 82.5 | 79.5 | 0.690 |
Age | 71.3 (6.7) | 73.0 (6.3) | 71.6 (6.4) | 73.0 (4.6) | 0.475 |
AChEI use, % | 50.0 | 37.2 | 47.5 | 43.6 | 0.676 |
DSST number correct | 13.3 (9.3) | 13.1 (10.9) | 11.1 (9.3) | 11.4 (9.7) | 0.660 |
SWM number correct | 15.0 (8.8) | 15.1 (9.7) | 12.6 (8.5) | 15.3 (9.1) | 0.521 |
Number of taps | 110.7 (51.8) | 120.9 (64.9) | 115.7 (52.3) | 118.4 (56.1) | 0.900 |
All data presented as mean (SD) unless otherwise indicated. AChEI, acetylcholinesterase inhibitor; DSST, digital symbol substitution; h, hours; m-g, milligravity; min, minutes; N, number of participants; SD, standard deviation; SWM, spatial working memory.
Statistical analysis
Participants were randomized, in a blinded fashion, to one of the four treatment arms (Placebo, LY3154207 10 mg, LY3154207 30 mg, LY3154207 75 mg) at a 1:1:1:1 ratio using a minimization procedure described elsewhere [17]. Digital biomarker compliance data were reported descriptively. An exploratory objective of PRESENCE was to evaluate the relationship of the digital biomarker endpoints with other clinical endpoints in terms of treatment effect. All analyses were conducted on the evaluable patient population (EPP) that partook in the digital biomarkers sub-study. The EPP included all data from all randomized participants who received at least one dose of study drug, had the baseline efficacy assessment, had at least one post-dose efficacy assessment, and partook in digital assessments. Data from digital biomarkers (iPad Trial app and actigraphy watch) were aggregated per treatment period, and change from baseline to week 12 (app) or week 8 (actigraphy) on prespecified endpoints of DSST, SWM, finger tapping, and actigraphy-measured activity and sleep were used to assess treatment effects using Mixed Model Repeated Measures analysis with treatment, time, and treatment-by-time interaction variables as fixed effects, and baseline digital biomarkers, age and acetylcholinesterase used as covariates. Time variable was defined as a repeated effect within subject. Pearson correlations between sensor-derived features and clinical endpoints were assessed. The analysis of these digital endpoints was not adjusted for multiplicity, given it was an exploratory objective in this trial.
RESULTS
Trial population and baseline characteristics
In the parent PRESENCE trial, participants’ baseline demographics and clinical characteristics were well-balanced and comparable across treatment groups [17]. Here, participants’ baseline digital endpoints were also similar with no statistically significant difference between groups across measured traits.
Compliance
During the intervention phase, overall actigraphy compliance was > 90% (Fig. 2A). Similar actigraphy compliance was also observed in the pre-intervention and post-intervention phases (Supplementary Figure 1). Note that the reduction in compliance on days 13 and 14 in the graph can be explained by participants returning devices at different times. Trial app compliance was > 60% among those who completed the study (Fig. 2C). There was a high rate of completed tests throughout the study period with a reduction toward the end of the study due to the return of devices (Supplementary Figure 2).
Fig. 2
Digital biomarker compliance. A) Actigraphy compliance during the intervention period. The number of compliant participants on each day is shown within the bars. B) Overall iPad Trial app compliance including data by all participants, whereas compliance removing data from participants who discontinued the study is shown in C).
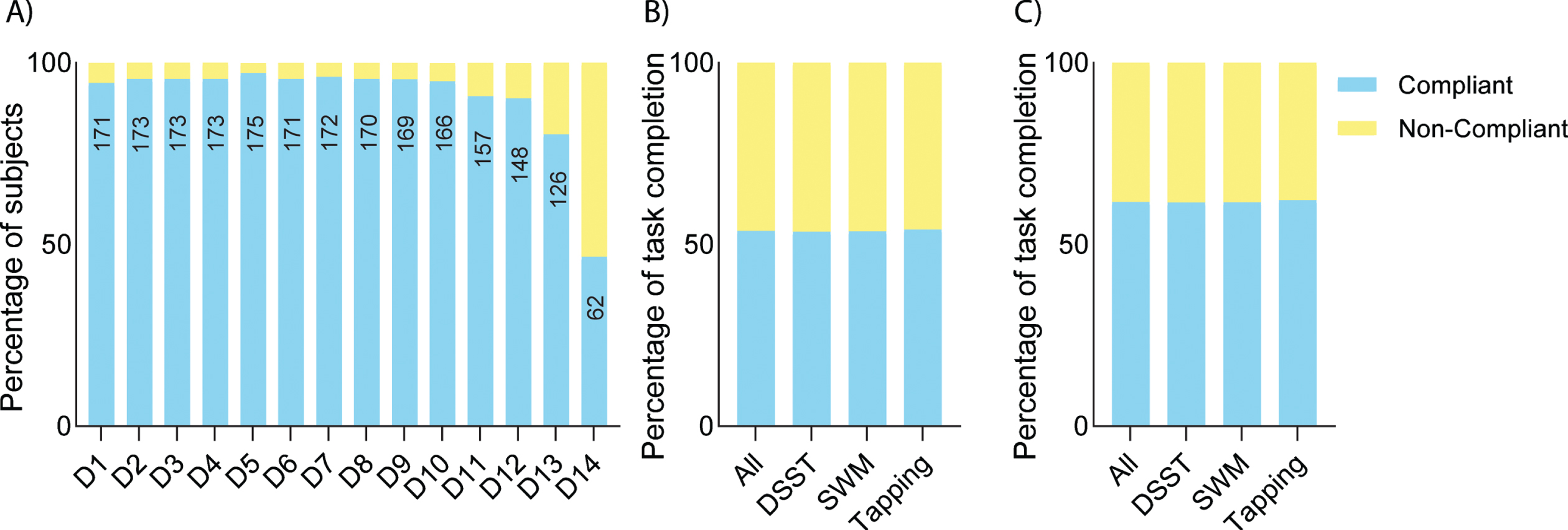
Digital biomarkers detect mevidalen treatment effects
Overall, treatment with mevidalen resulted in a statistically significant reduction in actigraphy-measured daytime sleep from baseline to week 8 compared to placebo (Fig. 3A. 10 mg: p < 0.01, effect size = 0.24; 30 mg: p < 0.05, effect size = 0.23; and 75 mg: p < 0.001, effect size = 0.39), without having significant effects on night-time sleep in the same period (Fig. 3B. 10 mg: p = 0.135, effect size = 0.14; 30 mg: p = 0.902, effect size = 0.01; and 75 mg: p = 0.618, effect size = 0.05). Treatment with 75 mg mevidalen resulted in a statistically significant increase in the walking minutes per day from baseline to week 8 compared to placebo (Fig. 3C. p < 0.001, effect size = 0.39); however, increase in the walking minutes per day was not statistically significant with 10 mg or 30 mg mevidalen (Fig. 3C. 10 mg: p = 0.059, effect size = 0.17; and 30 mg: p = 0.282, effect size = 0.10). With regard to the iPad trial app, mevidalen treatment (10, 30, and 75 mg) did not increase the number of correct responses in the DSST (Fig. 3D. 10 mg: p = 0.694, effect size = 0.04; 30 mg: p = 0.527, effect size = 0.07; and 75 mg: p = 1.000, effect size = 0.00) or performance in the finger tapping test (Fig. 3F. 10 mg: p = 0.326, effect size = 0.12; 30 mg: p = 0.808, effect size = 0.03; and 75 mg: p = 0.690, effect size = 0.05) from baseline to week 12 compared to placebo. Treatment with 30 mg mevidalen statistically significantly increased SWM from baseline to week 12 compared to placebo (Fig. 3E. 30 mg: p = 0.003, effect size = 0.35), but there were no statistically significant changes with 10 mg or 75 mg mevidalen (Fig. 3E. 10 mg: p = 0.663, effect size = 0.05; and 75 mg: p = 0.462, effect size = 0.08).
Fig. 3
Key actigraphy (A–C) and trial app (D–F) outcomes. A) Actigraphy measurements detected a dose-dependent reduction in daytime sleep using the actigraphy watch during intervention (visit 9) returning to pre-intervention levels post-intervention. B) Nighttime sleep showed minimal changes. iPad trial assessments were performed throughout the study. C) A mevidalen-induced increase in a daily activity in walking minutes per day was observed during intervention (75 mg group). D) Mevidalen treatment showed minimal changes to DSST performance. E) A mevidalen-induced increase (30 mg group) was observed in SWM. F) Minimal treatment effects were observed in the finger tapping tests. Type 1 errors were not controlled for, and p-values were not adjusted. *p < 0.05, **p < 0.01, ***p < 0.001.
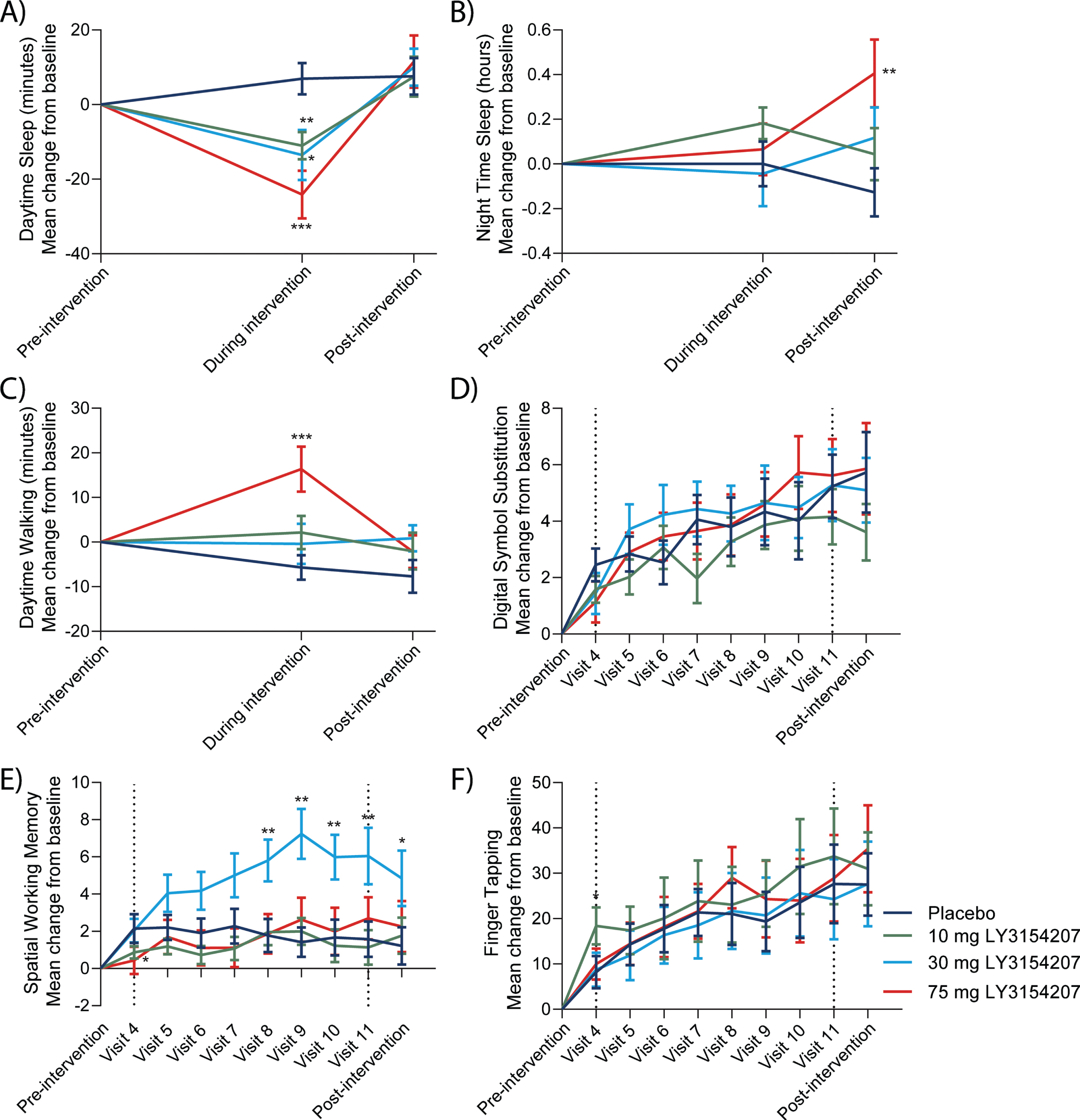
Digital biomarkers for continuous data capture
One potential advantage of utilizing digital biomarkers such as actigraphy in a clinical trial is that they offer continuous data monitoring and capture the period between study visits (actigraphy) or the entire study period (iPad trial app) rather than intermittent assessments at site visits. Continuous data capture for actigraphy allowed for both daily and hourly assessment of sleep and activity. A mevidalen-induced reduction was detected in daytime sleep from baseline throughout intervention as well as when hourly actigraphy-measured sleep was shown over a period of 24 h (when each actigraphy-measured sleep day was averaged from the full on-treatment period) (Fig. 4A and B, respectively). In the same respect, mevidalen-induced increases in daytime activity from baseline were observed throughout the intervention as well as when hourly actigraphy-measured activity was shown over a period of 24 h (when each actigraphy-measured activity day was averaged from the full on-treatment period) (Fig. 4C and D, respectively). The mevidalen-induced changes in daily daytime sleep (Fig. 4A) and daily daytime activity (Fig. 4C) returned to baseline levels in the post-intervention phase, whereas when plotting over a 24-h period, reduced daytime sleep (Fig. 4B) and increased daytime activity (Fig. 4D) can each clearly be observed in the treated groups beginning at 9am until 4pm.
Fig. 4
Digital biomarkers such as actigraphy allow for continuous data capture. A) Daily sleep. Reduction in total daytime sleep every day at all doses relative to placebo at steady state, with return to baseline post-intervention. B) Hourly sleep. Reduction in daytime sleep/inactivity from 9am to 3pm at all doses relative to placebo. C) Daily activity. Increase in average acceleration (m-g) every day at all doses relative to placebo at steady state, with return to baseline post-intervention. D) Hourly activity. Increase in average acceleration (m-g) from 9am to 7pm at all doses relative to placebo. Night in this study was defined as 7pm to 9am. X-axis break in A) and C) indicates the several weeks between intervention and post-intervention as per study schematic showing the PRESENCE trial period and the use of digital devices in Fig. 1.
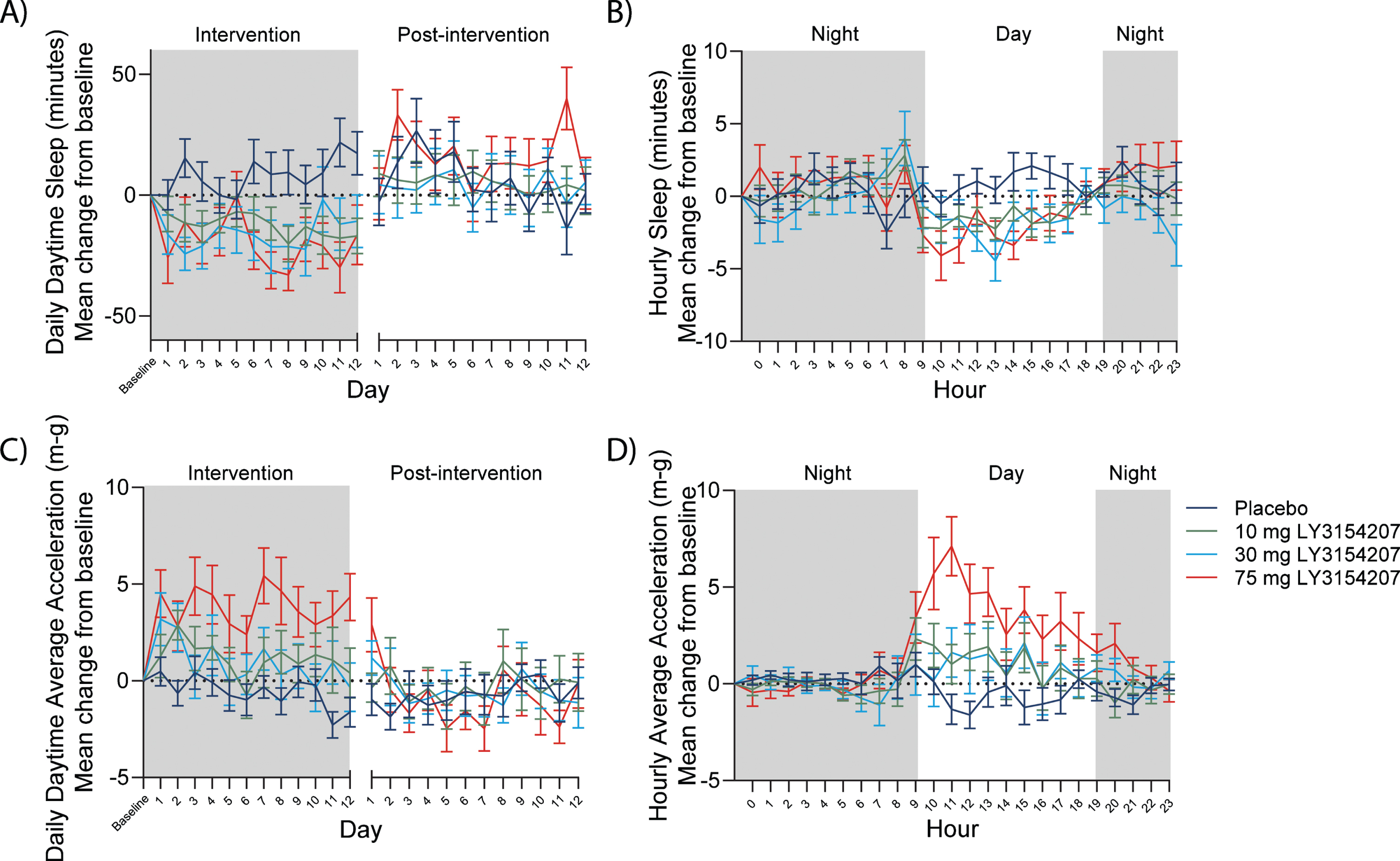
Correlation between digital and clinical measures
Although digital biomarkers can detect treatment effects of mevidalen on sleep, activity, and digital cognitive assessments such as SWM, it is important to relate these findings to clinical assessments obtained at site visits in order to establish clinical relevancy of digital biomarker both at baseline (Fig. 5) and change from baseline (Supplementary Figure 3). At baseline, actigraphy-measured daytime sleep positively correlated with the ESS score (Fig. 5A, R = 0.19, p < 0.01), whereas actigraphy-measured daytime activity correlated with lower (and, therefore, better) scores on MDS-UPDRS Part II (Fig. 5B, R = –0.31, p < 0.001) which assesses the motor experiences of daily living. Better scores of SWM and DSST correlated with lower (less cognitively impaired) scores on the ADAS-Cog13 scale (Fig. 5C, SWM: R = –0.54, p < 0.001; Fig. 5D, DSST: R = –0.52, p < 0.001). Change from baseline correlations revealed a weak but statistically significant relationship between actigraphy-measured daytime sleep and the ESS score (Supplementary Figure 3A, R = 0.2, p = 0.018), but non-significant relationship between daytime activity and MDS-UPDRS Part II Score (Supplementary Figure 3B, R = –0.13, p = 0.12), or SWM and DSST with ADAS-Cog13 scores (Supplementary Figure 3C, SWM: R = 0.073, p = 0.46; Supplementary Figure 3D, DSST: R = 0.0093, p = 0.92).
Fig. 5
Clinical relevance of digital measures at baseline. Correlations between clinical assessments and Actigraphy (A and B) and iPad trial (C and D) assessments. A) Actigraphy-measured daytime sleep positively correlates with Epworth Sleepiness Scale score. B) Physical activity correlates with improvement on MDS-UPDRS Part II. C) Better scores of SWM correlate with lower ADAS-Cog13. D) Better scores of DSST correlate with lower ADAS-Cog13. ADAS-Cog13, Alzheimer Disease Assessment Scale –Cognitive 13-Item Scale; DSST, digital symbol substitution; MDS, Movement Disorder Society–owned rating scales; SWM, spatial working memory; UPDRS, Unified Parkinson Disease Rating Scale.
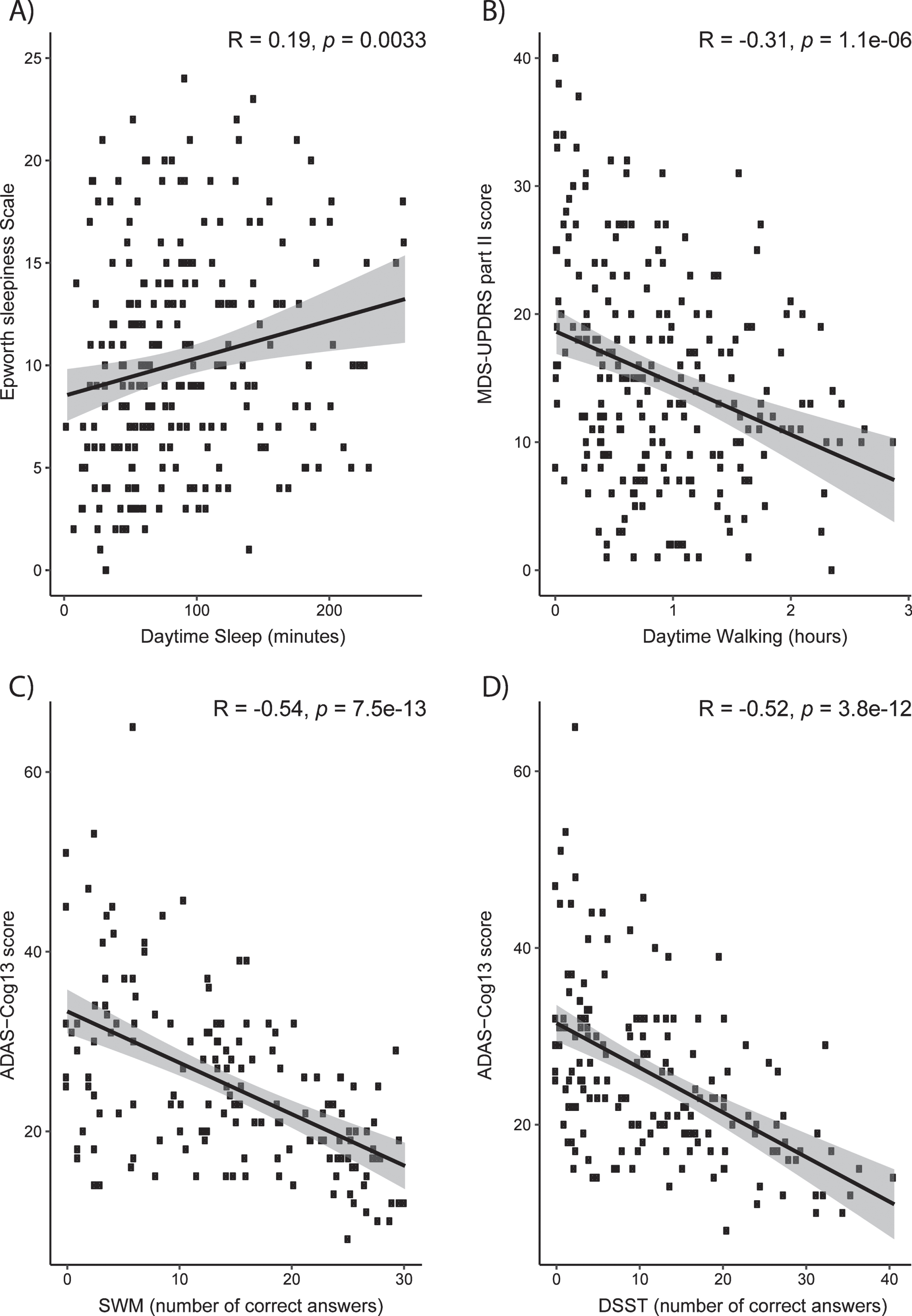
DISCUSSION
The results of the digital assessments included in the PRESENCE trial indicate that the use of iPad apps and wearable devices is feasible and results in adequate compliance in participants with LBD. Digital metrics were sensitive to detecting treatment-related changes in cognition, motor activity and daytime sleep. Further supporting the symptomatic benefits of mevidalen that were assessed with standard outcomes, digital assessments may be a meaningful addition to traditional clinical assessments of activity and sleep.
The high rate of compliance to digital measures throughout this study indicates that the use of digital measures is feasible even in a cognitively and motorically impaired population, though compliance was less for the trial app than the actigraphy device. Regarding the trial app, compliance was similar across all tasks, likely given that the app was designed so that each task required completion before one could proceed to subsequent tasks, and the duration of tasks was limited to 5 min to minimize patient burden. Elements of the actigraphy device that may have increased compliance include that the device was largely passive, did not require charging and was both durable and water-resistant allowing for continuous wear during the 2-week period of use.
At baseline, scores of SWM and DSST correlated with ADAS-Cog13 scores, actigraphy-measured daytime sleep correlated with the ESS score, and actigraphy-measured daytime activity correlated with MDS-UPDRS Part II scores. These baseline correlations between clinical measures of cognition, sleep and motor function and relevant digital biomarkers support the clinical validation of these digital measures. In contrast, change from baseline correlations between digital measures and clinical measures revealed mixed results. For example, we observed a non-significant relationship of SWM and DSST with ADAS-Cog13 scores, a modest but statistically significant relationship between actigraphy-measured daytime sleep and ESS score, and a non-significant relationship between daytime activity and MDS-UPDRS Part II Score. Such discrepancy underscores the disparity between digital and clinical measurements in terms of assessment timing, cadence, sensitivity and that they likely measure slightly different disease status. The daytime activity used for change correlation was summarized from two weeks of continuous actigraphy data, while the MDS-UPDRS Part II Score was from one in-clinic. In addition, continuous activity from actigraphy sensors captures different aspects of physiological state from physician rated activity of daily living associated with motor impairment. For broader adoption of digital measures in clinical development or practice, it will be valuable for the community to align on standardized ways to compare digital and clinical measures.
The primary endpoint of mevidalen treatment in the PRESENCE trial was improvement in cognition assessed by the CDR-CoA, which was not met, nor was a secondary cognitive endpoint of improvement in ADAS-Cog13 from baseline [17]. Supportive of the apparent lack of mevidalen-induced improvements in cognition were the limited treatment-related improvements in digital measures of cognition; however, treatment with 30 mg mevidalen did result in a statistically significant improvement in the SWM from baseline to Week 12 compared to placebo and the improvement during the last 6 weeks of treatment averages 50% from baseline. Although preclinical data did indicate that mevidalen treatment had the potential for improvement in SWM [15], the lack of cognitive benefit observed in clinical measures prompts caution in the interpretation of the improvement in SWM here; furthermore, given that analyses were exploratory and no correction for multiple comparisons was made, some results (e.g., improvement in SWM) may be spurious. Indeed, the improvement in SWM in the 30 mg mevidalen group may be partially driven by a 3-point lower score in this group at baseline.
Using traditional clinical assessments, mevidalen treatment (at the 30 and 75 mg doses) significantly improved MDS-UPDRS Part II scores and therefore motor function [17]. Mevidalen treatment (at the 75 mg dose) significantly increased actigraphy-measured daytime activity from baseline to week 8 compared to placebo, indicating that the actigraphy-measured increases in activity can predict the treatment-induced motor improvements. There was also a lack of noted mevidalen effect on finger tapping despite published evidence of its sensitivity [28]; however, the test was modified in this trial to use an iPad, and not a smartphone that was used in validating this task in other studies. Despite the inclusion of multiple daily tests and two weeks of pre-intervention data collection in an effort to overcome the potential learning effect, there still appears to be a continued learning effect for the duration of the trial. Indeed, it was observed in an observational study using mPower iPhone app that patients with PD required more finger tapping tests to reach a steady-state performance than healthy volunteers [28]. The participants with LBD in the current study were at an advanced stage and likely had more performance variability therefore two weeks of baseline period may not be sufficient to reach a steady state.
In the PRESENCE trial, potentially clinically-meaningful improvements in the ESS were observed with mevidalen treatment (75 mg mevidalen dose) [17], paralleled by statistically significant reductions in actigraphy-measured daytime sleep from baseline to week 8 compared to placebo at all doses of mevidalen (10, 30, and 75 mg). This reduction indicates that the actigraphy-measured reduction in daytime sleep can not only predict treatment-induced increases in wakefulness but can also detect changes in wakefulness earlier and with a smaller sample size than its clinical assessment counterpart. Although the actigraphy-measured sleep appeared to be more sensitive than clinical measurements, it is important to note that sleep was measured using an actigraphy-based algorithm [22, 32, 33]; in future studies, the use of devices that measure other physiological measures related to sleep, such as heart rate variability, may be helpful to further differentiate and improve the accuracy of sleep detection.
Taken together, the results suggest that digital biomarkers may allow the identification of domain-specific treatment effects to inform future development using smaller sample sizes and over shorter durations than traditional clinical measures; however, there were several limitations associated with this study. First, the data here do not represent a full data set and rely on convenience sampling regarding participants and timepoints utilized. The population examined was limited by geographical location for actigraphy availability and the optional aspect of the iPad trial app sub-study, which may result in bias and limit the generalization of these findings to the broader PRESENCE trial cohort. While no clear differences in demographic or disease specific measures were observed between the digital biomarker cohort relative to the entire PRESENCE trial cohort nor across treatment arms in the digital biomarker cohort, it remains possible that unmeasured factors may have further biased these findings. Second, there were a disproportionate number of male versus female participants included in the digital component of the trial, which, although representative of the parent trial population and not unusual in clinical research [34], may also highlight a sex difference in the ease and willingness to use digital devices. In addition, the trial participants also lacked diversity in race [17]; additionally, given the English-speaking requirement for actigraphy participants which would result in a less-diverse cohort, these results would need to be further validated in patients from a more diverse background. Third, correlations between digital measurements and clinical measurements are often weak, and, although clinical validation is critical, the multitude of variables that each measure can have on the accuracy of results of clinical scales and associated correlations with digital measurements must be recognized (e.g., subjective versus objective assessment, real time versus recall, continuous versus sporadic assessments as well as the known limitations of current clinical measurements). In addition, despite the correlation of digital and clinical measurements related to activity and sleep shown here, further research would be required to determine if the changes observed are clinically meaningful to participants. To address these evolving challenges, numerous groups and efforts, such as the Parkinson’s Disease Digital Biomarker DREAM Challenge [35], the Critical Path for Parkinson’s Consortium, the Michael J Fox Foundation, the Digital Medical society, the Clinical Trials Transformation Initiative [36] and the open wearable initiatives, are collaboratively evaluating algorithms and developing benchmark datasets to facilitate wearable device and sensor signal verification, digital biomarker development, and clinical validation [37].
Finally, given the ongoing coronavirus pandemic and associated travel limitations imposed around the world, the use of telemedicine and digital measures has dramatically increased and has been suggested as a rare silver lining of the pandemic [38–40]. Over the course of the pandemic, digital devices have allowed for the continuity of care and clinical research and have perhaps accelerated the adoption of decentralized clinical trial (DCT) approaches. The use of decentralized intervention strategies using high-frequency, unsupervised, home-based assessments may shape the future of clinical trials, and digital devices play an integral role in these innovative clinical trials [39]. DCT and the associated use of digital devices may also provide increased diversity, inclusion, and representation in terms of sex, race, and socioeconomics in clinical trial populations.
Clinical measures for PD have largely remained unchanged and are limited by their episodic and subjective nature. These traditional assessments may not accurately capture symptoms that occur between visits, or the regular fluctuations associated with disease. Digital measures are objective, often passive, or minimally burdensome, and may have greater sensitivity than traditional measures and allow for more frequent assessments; therefore, they may allow for detection of drug effect in small sample sizes to inform drug development. Although the digital biomarker results in this Phase 2 study should be validated with an independent sample of patients either through another Phase 2 or a Phase 3 study, these results highlight the unique potential of digital devices to detect treatment effects over shorter durations and smaller sample sizes than traditional clinical outcomes. These results indicate that digital biomarkers offer great promise to empower smaller, more efficient, and better patient-centric clinical trial designs with a view of overall improved patient care.
ACKNOWLEDGMENTS
The authors would like to acknowledge Deirdre Hoban, PhD, an employee of Eli Lilly and Company, for project management support, and strategic and scientific communication expertise. We thank all the patients and their families and caregivers who participated in this trial. We would like to acknowledge the site staff and site investigators, including, but not limited to, the following: Aaron Ellenbogen (Michigan Institute for Neurological Disorders (M.I.N.D.), Farmington Hills, Michigan, USA), Adolfo Ramirez-Zamora (Norman Fixel Institute For Neurological Diseases (FIND), Gainesville, Florida, USA), Alicia Cabrera (Clincloud, Llc., Maitland, Florida, USA), Amy Amara (University of Alabama Birmingham, Birmingham, Alabama, USA), Andrew Feigin (NYU Langone, New York, New York, USA), Andrew Garner (Adirondack Medical Research, Glen Falls, New York, USA), Andrew Lerman (Visionary Investigators Network, Miami, Florida, USA), Anna Morenkova (University of California, Irvine, Irvine, California, USA), Arifulla Khan (Northwest Clinical Research Center, Bellevue, Washington, USA), Arjun Tarakad (Baylor College Of Medicine, Houston, Texas, USA), Ashok Patel (Bio Behavioral Health, Toms River, New Jersey, USA), Bruno Gallo (Suncoast Research Group, Miami, Florida, USA), Carmen Serrano (University of Puerto Rico, San Juan, Puerto Rico), Christian Schenk (Cortex, Psc., Las Piedras, Puerto Rico), Daniel Truong (Parkinson’s & Movement Disorder Institute, Fountain Valley, California, USA), Danielle Feigenbaum (University of Southern California School of Medicine, Los Angeles, California, USA), David Grimes (Ottawa Hospital Research Institute, Parkinson’s Disease and Movement Disorders Clinic, Ottawa, Ontario, Canada), David Shprecher (Banner Sun Health Research Institute, Sun City, Arizona, USA), David Weisman (Abington Neurological Associates, Ltd., Willow Grove, Pennsylvania, USA), Federico Rodriguez-Porcel (Medical University of South Carolina, Charleston, South Carolina, USA), Fernando Pagan (Georgetown University Hospital, Washington, DC, USA), Horacio Capote (Dent Neurological Institute, Amherst, New York, USA), J. Antonelle de Marcaida (Hartford Healthcare Chase Movement Disorders Center, Vernon, Connecticut, USA), Jay Schneider (Thomas Jefferson University, Philadelphia, Philadelphia, USA), Julie Schwartzbard (Visionary Investigators Network, Aventura, Florida USA), Kara Wyant (University of Michigan, Ann Arbor, Michigan, USA), Kathleen Shannon (University Of Wisconsin-Madison Hospital and Health Clinic, Madison, Wisconsin, USA), Kinga Szigeti (Alzheimer’s Disease and Memory Disorders Center, Buffalo, New York, USA), Kristi George (Josephson Wallack Munshower Neurology, Indianapolis, Indiana, USA), Laurice Yang (Stanford Neuroscience Health Center, Palo Alto, California, USA), Lin Zhang (University of California, Davis, Sacramento, California, USA), Margarita Almeida El-Ramey (Visionary Investigators Network, Pembroke Pines, Florida, USA), Mark A. Goldstein (Jem Research Institute, Atlantis, Florida, USA), Michael Kleinman (Maine Medical Center-Maine Medical Partners Neurology, Maine, USA), Nabila Dahodwala (Pennsylvania Hospital, Philadelphia, Pennsylvania, USA), Nirav Pavasia (Neurology Consultants Of Dallas, Dallas, Texas, USA), Oleg Tcheremissine (Carolinas Healthcare System, North Charlotte, North Carolina, USA), Omid Omidvar (Collaborative Neuroscience Research, Llc., Long Beach, California, USA), Peter McAllister (New England Institute For Clinical Research, Stamford, Connecticut, USA), Pinky Agarwal (Evergreen Professional Plaza, Kirkland, Washington, USA), Rajeev Kumar (Rocky Mountain Movement Disorders Center, Englewood, Colorado, USA), Rajesh Pahwa (University of Kansas School of Medicine, Parkinson’s Disease & Movement Disorders Center, Kansas City, Kansas, USA), Robert Riesenberg (Atlanta Center of Medical Research, Atlanta, Georgia, USA), Ryan Walsh (Barrow Neurological Institute, Barrow Neurology Clinic, Phoenix, Arizona, USA), Samantha Holden (University of Colorado Hospital, Aurora, Colorado, USA), Scott Sherman (University of Arizona Health Sciences, Department of Neurology, Tucson, Arizona, USA), Sharon Cohen (Toronto Memory Program, Toronto, Ontario, Canada), Shyamal Mehta (Mayo Clinic of Scottsdale, Scottsdale, Arizona, USA), Siddharth Kaul (Clinical Research Professionals, Chesterfield, Missouri, USA), Stephen Lee (Dartmouth Hitchcock Medical Center, Lebanon, New Hampshire, USA), Stewart Factor (Emory University, Atlanta, Georgia USA), Stuart Isaacson (Parkinson’s Disease and Movement Disorders, Boca Raton, Florida, USA), Susan Steen (Axiom Clinical Research of Florida, Tampa, Florida, USA), Thyagarajan Subramanian (Penn State University Milton S. Hershey Medical Center, Pennsylvania, USA), Victor Faradji (Visionary Investigators Network, Miami, Florida, USA), Waleed El-Feky (Texas Neurology, Dallas, Texas, USA), William Julio (Santa Cruz Behavioral Psc., Bayamón, Puerto Rico), Zoltan Mari (Cleveland Clinic of Las Vegas, Las Vegas, Nevada, USA), and Zoran Grujic (Central Dupage Hospital, Winfield, Illinois, USA).
This work is sponsored by Eli Lilly and Company.
CONFLICT OF INTEREST
All authors are full-time employees, salary recipients and minor shareholders at Eli Lilly and Company.
AVAILABILITY OF DATA AND MATERIALS
Lilly provides access to all individual participant data collected during the trial, after anonymization, with the exception of pharmacokinetic or genetic data. Data are available to request 6 months after the indication studied has been approved in the US and EU and after primary publication acceptance, whichever is later. No expiration date of data requests is currently set once data are made available. Access is provided after a proposal has been approved by an independent review committee identified for this purpose and after receipt of a signed data sharing agreement. Data and documents, including the study protocol, statistical analysis plan, clinical study report, blank or annotated case report forms, will be provided in a secure data sharing environment. For details on submitting a request, see the instructions provided at http://www.vivli.org.
SUPPLEMENTARY MATERIAL
[1] The supplementary material is available in the electronic version of this article: https://dx.doi.org/10.3233/JPD-213126.
REFERENCES
[1] | Colloby SJ , McKeith IG , Burn DJ , Wyper DJ , O’Brien JT , Taylor JP ((2016) ) Cholinergic and perfusion brain networks in Parkinson disease dementia. Neurology 87: , 178–185. |
[2] | Klein JC , Eggers C , Kalbe E , Weisenbach S , Hohmann C , Vollmar S , Baudrexel S , Diederich NJ , Heiss WD , Hilker R ((2010) ) Neurotransmitter changes in dementia with Lewy bodies and Parkinson disease dementia in vivo. Neurology 74: , 885–892. |
[3] | Colloby SJ , McParland S , O’Brien JT , Attems J ((2012) ) Neuropathological correlates of dopaminergic imaging in Alzheimer’s disease and Lewy body dementias. Brain 135: , 2798–2808. |
[4] | Harding AJ , Halliday GM ((2001) ) Cortical Lewy body pathology in the diagnosis of dementia. Acta Neuropathol 102: , 355–363. |
[5] | Emre M , Aarsland D , Albanese A , Byrne EJ , Deuschl G , De Deyn PP , Durif F , Kulisevsky J , van Laar T , Lees A , Poewe W , Robillard A , Rosa MM , Wolters E , Quarg P , Tekin S , Lane R ((2004) ) Rivastigmine for dementia associated with Parkinson’s disease. N Engl J Med 351: , 2509–2518. |
[6] | Taylor JP , McKeith IG , Burn DJ , Boeve BF , Weintraub D , Bamford C , Allan LM , Thomas AJ , O’Brien JT ((2020) ) New evidence on the management of Lewy body dementia. Lancet Neurol 19: , 157–169. |
[7] | Walker Z , Possin KL , Boeve BF , Aarsland D ((2015) ) Lewy body dementias. Lancet 386: , 1683–1697. |
[8] | Bot BM , Suver C , Neto EC , Kellen M , Klein A , Bare C , Doerr M , Pratap A , Wilbanks J , Dorsey ER , Friend SH , Trister AD ((2016) ) The mPower study, Parkinson disease mobile data collected using ResearchKit. Sci Data 3: , 160011. |
[9] | Buegler M , Harms RL , Balasa M , Meier IB , Exarchos T , Rai L , Boyle R , Tort A , Kozori M , Lazarou E , Rampini M , Cavaliere C , Vlamos P , Tsolaki M , Babiloni C , Soricelli A , Frisoni G , Sanchez-Valle R , Whelan R , Merlo-Pich E , Tarnanas I ((2020) ) Digital biomarker-based individualized prognosis for people at risk of dementia. Alzheimers Dement (Amst) 12: , e12073. |
[10] | Ancoli-Israel S , Cole R , Alessi C , Chambers M , Moorcroft W , Pollak CP ((2003) ) The role of actigraphy in the study of sleep and circadian rhythms. Sleep 26: , 342–392. |
[11] | Erb MK , Karlin DR , Ho BK , Thomas KC , Parisi F , Vergara-Diaz GP , Daneault JF , Wacnik PW , Zhang H , Kangarloo T , Demanuele C , Brooks CR , Detheridge CN , Shaafi Kabiri N , Bhangu JS , Bonato P ((2020) ) mHealth and wearable technology should replace motor diaries to track motor fluctuations in Parkinson’s disease. NPJ Digit Med 3: , 6. |
[12] | Cohen S , Waks Z , Elm JJ , Gordon MF , Grachev ID , Navon-Perry L , Fine S , Grossman I , Papapetropoulos S , Savola JM ((2018) ) Characterizing patient compliance over six months in remote digital trials of Parkinson’s and Huntington disease. BMC Med Inform Decis Mak 18: , 138. |
[13] | Silva de Lima AL , Hahn T , Evers LJW , de Vries NM , Cohen E , Afek M , Bataille L , Daeschler M , Claes K , Boroojerdi B , Terricabras D , Little MA , Baldus H , Bloem BR , Faber MJ ((2017) ) Feasibility of large-scale deployment of multiple wearable sensors in Parkinson’s disease. PLoS One 12: , e0189161. |
[14] | Hao J , Beck JP , Schaus JM , Krushinski JH , Chen Q , Beadle CD , Vidal P , Reinhard MR , Dressman BA , Massey SM , Boulet SL , Cohen MP , Watson BM , Tupper D , Gardinier KM , Myers J , Johansson AM , Richardson J , Richards DS , Hembre EJ , Remick DM , Coates DA , Bhardwaj RM , Diseroad BA , Bender D , Stephenson G , Wolfangel CD , Diaz N , Getman BG , Wang XS , Heinz BA , Cramer JW , Zhou X , Maren DL , Falcone JF , Wright RA , Mitchell SN , Carter G , Yang CR , Bruns RF , Svensson KA ((2019) ) Synthesis and pharmacological characterization of 2-(2,6-Dichlorophenyl)-1-((1S,3R)-5-(3-hydroxy-3-methylbutyl)-3-(hydroxymethyl)-1-methyl-3,4-dihydroisoquinolin-2(1H)-yl)ethan-1-one (LY3154207), a potent, subtype sele-ctive, and orally available positive allosteric modulator of the human dopamine D1 receptor. J Med Chem 62: , 8711–8732. |
[15] | Svensson KA , Hao J , Bruns RF ((2019) ) Positive allosteric modulators of the dopamine D1 receptor: A new mechanism for the treatment of neuropsychiatric disorders. Adv Pharmacol 86: , 273–305. |
[16] | Wilbraham D , Biglan KM , Svensson KA , Tsai M , Kielbasa W ((2020) ) Safety, tolerability, and pharmacokinetics of mevidalen (LY3154207), a centrally acting dopamine D1 receptor-positive allosteric modulator (D1PAM), in healthy subjects. Clin Pharmacol Drug Dev 10: , 393–403. |
[17] | Biglan K , Munsie L , Svensson KA , Ardayfio P , Pugh M , Sims JR , Brys M ((2022) ) Safety and efficacy of mevidalen in Lewy body dementia: A phase 2, randomized, placebo-controlled trial. Mov Disord 37: , 513–524. |
[18] | Nasreddine ZS , Phillips NA , Bedirian V , Charbonneau S , Whitehead V , Collin I , Cummings JL , Chertkow H ((2005) ) The Montreal Cognitive Assessment, MoCA: A brief screening tool for mild cognitive impairment. J Am Geriatr Soc 53: , 695–699. |
[19] | Postuma RB , Berg D , Stern M , Poewe W , Olanow CW , Oertel W , Obeso J , Marek K , Litvan I , Lang AE , Halliday G , Goetz CG , Gasser T , Dubois B , Chan P , Bloem BR , Adler CH , Deuschl G ((2015) ) MDS clinical diagnostic criteria for Parkinson’s disease. Mov Disord 30: , 1591–1601. |
[20] | McKeith IG , Boeve BF , Dickson DW , Halliday G , Taylor JP , Weintraub D , Aarsland D , Galvin J , Attems J , Ballard CG , Bayston A , Beach TG , Blanc F , Bohnen N , Bonanni L , Bras J , Brundin P , Burn D , Chen-Plotkin A , Duda JE , El-Agnaf O , Feldman H , Ferman TJ , Ffytche D , Fujishiro H , Galasko D , Goldman JG , Gomperts SN , Graff-Radford NR , Honig LS , Iranzo A , Kantarci K , Kaufer D , Kukull W , Lee VMY , Leverenz JB , Lewis S , Lippa C , Lunde A , Masellis M , Masliah E , McLean P , Mollenhauer B , Montine TJ , Moreno E , Mori E , Murray M , O’Brien JT , Orimo S , Postuma RB , Ramaswamy S , Ross OA , Salmon DP , Singleton A , Taylor A , Thomas A , Tiraboschi P , Toledo JB , Trojanowski JQ , Tsuang D , Walker Z , Yamada M , Kosaka K ((2017) ) Diagnosis and management of dementia with Lewy bodies: Fourth consensus report of the DLB Consortium. Neurology 89: , 88–100. |
[21] | Hoehn MM , Yahr MD ((1967) ) Parkinsonism: Onset, progression and mortality. Neurology 17: , 427–442. |
[22] | Doherty A , Smith-Byrne K , Ferreira T , Holmes MV , Holmes C , Pulit SL , Lindgren CM ((2018) ) GWAS identifies 14 loci for device-measured physical activity and sleep duration. Nat Commun 9: , 5257. |
[23] | Doherty A , Jackson D , Hammerla N , Plotz T , Olivier P , Granat MH , White T , van Hees VT , Trenell MI , Owen CG , Preece SJ , Gillions R , Sheard S , Peakman T , Brage S , Wareham NJ ((2017) ) Large scale population assessment of physical activity using wrist worn accelerometers: The UK Biobank Study. PLoS One 12: , e0169649. |
[24] | Peraza LR , Joules R , Dauvilliers Y , Wolz R (2020) Device agnostic sleep-wake segment classification from wrist-worn accelerometry. 2020 IEEE International Conference on Healthcare Informatics (ICHI), pp. 1–3. |
[25] | van Hees VT , Sabia S , Anderson KN , Denton SJ , Oliver J , Catt M , Abell JG , Kivimaki M , Trenell MI , Singh-Manoux A ((2015) ) A novel, open access method to assess sleep duration using a wrist-worn accelerometer. PLoS One 10: , e0142533. |
[26] | Brain Baseline https://www.brainbaseline.com/, |
[27] | Awh E , Jonides J , Reuter-Lorenz PA ((1998) ) Rehearsal in spatial working memory. J Exp Psychol Hum Percept Perform 24: , 780–790. |
[28] | Prince J , Arora S , de Vos M ((2018) ) Big data in Parkinson’s disease: Using smartphones to remotely detect longitudinal disease phenotypes. Physiol Meas 39: , 044005. |
[29] | Mohs RC , Knopman D , Petersen RC , Ferris SH , Ernesto C , Grundman M , Sano M , Bieliauskas L , Geldmacher D , Clark C , Thal LJ ((1997) ) Development of cognitive instruments for use in clinical trials of antidementia drugs: Additions to the Alzheimer’s Disease Assessment Scale that broaden its scope. The Alzheimer’s Disease Cooperative Study. Alzheimer Dis Assoc Disord 11: (Suppl 2), S13–S21. |
[30] | Goetz CG , Tilley BC , Shaftman SR , Stebbins GT , Fahn S , Martinez-Martin P , Poewe W , Sampaio C , Stern MB , Dodel R , Dubois B , Holloway R , Jankovic J , Kulisevsky J , Lang AE , Lees A , Leurgans S , LeWitt PA , Nyenhuis D , Olanow CW , Rascol O , Schrag A , Teresi JA , van Hilten JJ , LaPelle N , Movement Disorder Society URTF ((2008) ) Movement Disorder Society-sponsored revision of the Unified Parkinson’s Disease Rating Scale (MDS-UPDRS): Scale presentation and clinimetric testing results. Mov Disord 23: , 2129–2170. |
[31] | Johns MW ((1991) ) A new method for measuring daytime sleepiness: The Epworth sleepiness scale. Sleep 14: , 540–545. |
[32] | Walmsley R , Chan S , Smith-Byrne K , Ramakrishnan R , Woodward M , Rahimi K , Dwyer T , Bennett D , Doherty A (2020) Reallocating time from device-measured sleep, sedentary behaviour or light physical activity to moderateto-vigorous physical activity is associated with lower cardiovascular disease risk. medRxiv, 2020.2011.2010.202 27769. |
[33] | Willetts M , Hollowell S , Aslett L , Holmes C , Doherty A ((2018) ) Statistical machine learning of sleep and physical activity phenotypes from sensor data in 96,220 UK Biobank participants. Sci Rep 8: , 7961. |
[34] | Feldman S , Ammar W , Lo K , Trepman E , van Zuylen M , Etzioni O ((2019) ) Quantifying sex bias in clinical studies at scale with automated data extraction. JAMA Netw Open 2: , e196700. |
[35] | Parkinsons Disease Digital Biomarker DREAM Challenge, https://www.synapse.org/#!Synapse:syn8717496/wiki/422884. |
[36] | Coran P , Goldsack JC , Grandinetti CA , Bakker JP , Bolognese M , Dorsey ER , Vasisht K , Amdur A , Dell C , Helfgott J , Kirchoff M , Miller CJ , Narayan A , Patel D , Peterson B , Ramirez E , Schiller D , Switzer T , Wing L , Forrest A , Doherty A ((2019) ) Advancing the use of mobile technologies in clinical trials: Recommendations from the clinical trials transformation initiative. Digit Biomark 3: , 145–154. |
[37] | Stephenson D , Badawy R , Mathur S , Tome M , Rochester L ((2021) ) Digital progression biomarkers as novel endpoints in clinical trials: A multistakeholder perspective. J Parkinsons Dis 11: , S103–S109. |
[38] | Bloem BR , Dorsey ER , Okun MS ((2020) ) The coronavirus disease 2019 crisis as catalyst for telemedicine for chronic neurological disorders. JAMA Neurol 77: , 927–928. |
[39] | Dorsey ER , Okun MS , Bloem BR ((2020) ) Care, convenience, comfort, confidentiality, and contagion: The 5 C’s that will shape the future of telemedicine. J Parkinsons Dis 10: , 893–897. |
[40] | Miele G , Straccia G , Moccia M , Leocani L , Tedeschi G , Bonavita S , Lavorgna L , Digital Technologies, Web and Social Media Study Group of the Italian Society of Neurology ((2020) ) Telemedicine in Parkinson’s disease: How to ensure patient needs and continuity of care at the time of COVID-19 pandemic. Telemed J E Health 26: , 1533–1536. |