A Randomized, Double-Blinded Crossover Trial of Short Versus Conventional Pulse Width Subthalamic Deep Brain Stimulation in Parkinson’s Disease
Abstract
Background:
Subthalamic nucleus deep brain stimulation (STN-DBS) is a well-established treatment for patients with Parkinson’s disease. Previous acute challenge studies suggested that short pulse widths might increase the therapeutic window while maintaining motor symptom control with a decrease in energy consumption. However, only little is known about the effect of short pulse width stimulation beyond the setting of an acute challenge.
Objective:
To compare 4 weeks of STN-DBS with conventional pulse width stimulation (60 μs) to 4 weeks of STN-DBS with short pulse width stimulation (30 μs) regarding motor symptom control.
Methods:
This study was a monocentric, double-blinded, randomized crossover non-inferiority trial investigating whether short pulse width stimulation with 30 μs maintains equal motor control as conventional 60 μs stimulation over a period of 4 weeks (German Clinical Trials Register No. DRKS00017528). Primary outcome was the difference in motor symptom control as assessed by a motor diary. Secondary outcomes included energy consumption measures, non-motor effects, side-effects, and quality of life.
Results:
Due to a high dropout rate, the calculated sample size of 27 patients was not met and 24 patients with Parkinson’s disease and STN-DBS were included in the final analysis. However, there were no differences in any investigated outcome parameter between the two treatment conditions.
Conclusion:
This study demonstrates that short pulse width settings (30 μs) provide non-inferior motor symptom control as conventional (60 μs) stimulation without significant differences in energy consumption. Future studies are warranted to evaluate a potential benefit of short pulse width settings in patients with pronounced dyskinesia.
INTRODUCTION
Subthalamic nucleus deep brain stimulation (STN-DBS) is a well-established treatment for patients with advanced Parkinson’s disease (PD) improving quality of life, motor, and non-motor symptoms [1–3]. However, postoperative outcomes critically depend on lead locations and stimulation settings [4–7]. While titration of the stimulation amplitude and choice of the active contact are usually individual, stimulation frequency and pulse width are typically set to standard values of 130 Hz and 60 μs. This standard value emerged from a previous study, demonstrating that a pulse width of 60 μs enlarges the therapeutic window as compared to larger pulse widths [8, 9].
Several recent studies suggested that shorter pulse widths, i.e., below 60 μs, might lead to an additional widening of the therapeutic window, defined as the amplitude range between threshold amplitudes for rigidity control and occurrence of side-effects [10–14]. Additionally, some authors suggested that DBS with shorter pulse widths might reduce energy consumption while maintaining equal symptom control compared to standard pulse width as investigated during an acute challenge design [10–12]. Furthermore, DBS with 30 μs resulted in an improvement of stimulation-induced dysarthria, dyskinesia, and gait in an acute challenge [14, 15]. However, this beneficial effect remains controversial as it could not be replicated in a recent study investigating the effect of short pulse width stimulation on objective gait parameters in an acute challenge and neither in a double-blinded randomized crossover trial investigating a period of 4 weeks in a cohort of PD patients with stimulation-induced dysarthria [13, 16]. As a secondary outcome of this study by Dayal et al., there was no difference in motor symptom control between short and conventional pulse width stimulation, captured as a snapshot by the Movement Disorder Society-Unified Parkinson’s Disease Rating Scale [13].
To summarize, shorter pulse widths were so far associated with the potential to reduce side-effects and energy consumption while maintaining the therapeutic effect. Whether these effects can be reconfirmed beyond the setting of an acute challenge, remains to be elucidated. Therefore, in this double-blind, randomized, crossover trial, we investigate whether short pulse width stimulation with 30 μs maintains motor symptom control, as measured by a patient-reported motor diary, in comparison to 60 μs for 4 weeks. This is of special importance for everyday clinical care, as unnecessary reprogramming by switching pulse widths in a trial-and-error fashion should be avoided, when there is no evidence for any benefit for the patient.
METHODS
Participants and ethical approval
Eligible patients were aged 18 to 80 years with a clinical diagnosis of PD following the UK Brain Bank criteria, who underwent bilateral STN-DBS at least 3 months before study inclusion. Patients had been selected for STN-DBS according to the guidelines of the International Parkinson and Movement Disorders Society [17]. Additionally, the implanted pulse generator required the possibility of providing stimulation with a pulse width of 30 μs as per clinical routine. The ethics committee of the University of Cologne approved the trial (vote: 19-1233) conducted under the declaration of Helsinki, which was registered with the German Clinical Trials Register (registration No. DRKS00017528). Data was collected at the Department of Neurology of the University Hospital Cologne. All patients gave written informed consent prior to any study activity.
Study design
This study was a single-center, randomized, double-blind, crossover, non-inferiority trial of deep brain stimulation with a short pulse width (30 μs) versus conventional pulse width (60 μs) in PD patients with bilateral STN-DBS. A non-inferiority design was chosen as previous trials, comparing motor symptom control between short and conventional pulse width stimulation did not yield towards superiority of either pulse width [12, 13]. The trial protocol is illustrated in Fig. 1A and consisted of 3 visits per participant. At baseline, medical and surgical history, as well as medication, was recorded. Stimulation settings with a pulse width of 60 μs (PW60) and 30 μs respectively (PW30) were established based on the stimulation setting at study inclusion in the “medication ON” state, as optimized per clinical routine. Of note, the respective active contact and frequency were equal for both trial programs, whereas only the applied current was titrated for motor symptom control and side effects. Patients were trained to titrate the current with the handheld patient programmer to perform adjustments of current within individual ranges if needed. The clinician tested the range of the applicable current to exclude acute stimulation-induced side effects. Then patients were randomized blockwise (3 Blocks, 1:1 randomization using http://www.randomizer.at) to begin with PW60 followed by four weeks with PW30 or vice versa. A routine telephone call was done after 14 days of each period and 5 days prior to each follow-up visit to support the patient in self-adjustment of the applied current. Of note, patients were instructed not to titrate the current during the last three days of each period to ensure a constant stimulation setting while assessing the motor diary and no changes in medication were allowed throughout the trial.
Fig. 1
Study Protocol and Participant Flow. A) Patients were randomized to 4 weeks of stimulation with PW60 followed by 4 weeks of stimulation with PW30 and vice versa. Stimulation settings only differed in their pulse widths while the active contact and frequency were identical. To ensure optimal symptom control during assessment of the motor diary (MD) patients were allowed for self-adjustment of the applied current. B) Reasons for dropout were: Withdrawal of consent due to COVID-19 pandemic (N = 3; 1 A1, 1 A2, 1 B2), insufficient symptom control (N = 2; 1 A2, 1 B1), withdrawal of consent without specific reason (N = 3; 2 A1, 1 B2), DBS system infection (N = 1; B2), exclusion due to violation of study protocol (unauthorized switching of stimulation programs during the trial, N = 2; 1 A2, 1 B2)).
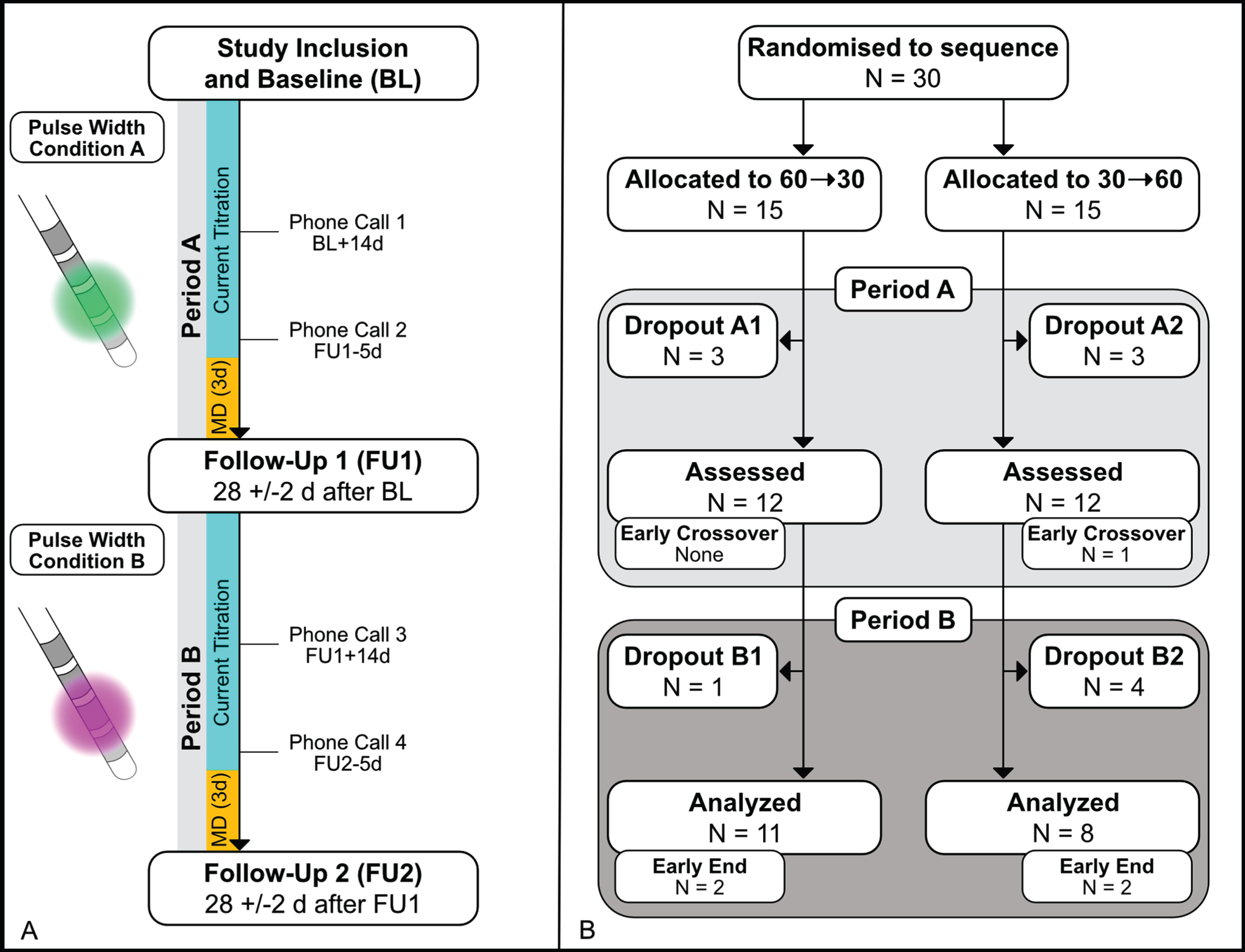
After 4 weeks (Period A), patients returned for a clinical assessment after at least overnight withdrawal of dopaminergic medications (follow up visit 1:28±2 days from baseline). Adverse events and stimulation parameters, including impedances, were recorded. Secondary outcomes were assessed, and stimulation was then switched to the other treatment condition. If necessary, current was again optimized before discharge. Patients returned after 4 weeks (Period B) to repeat the assessment in “medication OFF” state (follow up visit 2:56±2 days from baseline). By the end of the trial, patients were asked for their preferred setting. If one treatment condition was not well tolerated despite current adjustment, patients could choose between an early cross-over or end of study after filling the motor diary over three days, or an instant termination of the trial. All participants and raters remained blinded to the treatment condition throughout the trial. Blinding was ensured by i) non-visibility of the chosen pulse width on the patient programmer and ii) by exclusion of raters for the programming process.
Outcome parameters
The primary outcome parameter was the difference in “On time” as reported by a standardized self-reported motor diary between the PW60 and the PW30 condition over the last three days in each condition [18]. A motor diary assesses the motor state in intervals of 30 min defined as 1) awake and with good symptom control (“On”), 2) awake and troublesome dyskinesia (“Dyskinesia”), 3) awake and with poor motor function (“Off”), and 4) asleep (“Sleep”). Previous studies have already used this diary to evaluate motor symptom control in PD patients with STN-DBS, beyond an acute assessment [2, 19, 20]. For further analysis the mean time of the respective motor state in each period was calculated.
Predefined secondary outcomes, as assessed in “medication OFF” state at each follow-up visit, were differences in the Unified Parkinson’s Disease Rating Scale (UPDRS-I, -II and -III), 10 m timed walk test, visual analog scale (VAS) for self-assessment of “ability to walk”, and “ability to speak”, Parkinson’s Disease questionnaire 39-Item Quality of Life Questionnaire Summary Index (PDQ-39 SI), and Non-Motor Symptoms Questionnaire (NMSQ). Additionally, differences in speech intelligibility were investigated by reading a German standard text (“Der Teppichklopfer”) rated by 13 blinded naïve listeners on a VAS. All VAS ranged from 0 to 10 (“worst possible state” to “best possible state” of the respective symptom). To investigate differences in energy consumption the battery charge index (BCI) [21], the total charge delivered per pulse (CPP = current*pulse width), and the total electrical energy delivered (TEED = ((current*impedance)2 *frequency*pulse width)/ impedance) were calculated, and the sum of both hemispheres was reported.
Statistical analysis
A modified intention-to-treat analysis, including all available data points despite the high dropout rate, was conducted for each outcome parameter employing linear mixed effects models including the grouping variable (PW60, PW30) as a fixed effect and subject as a random effect to correct for the paired nature of the data (outcome ∼ pulse width group + (1|subject)). For analysis of the primary outcome parameter the interaction between period and the grouping variable (PW60, PW30) was included as an additional fixed effect to account for a possible period effect (outcome ∼ pulse width group + period*pulse width group + (1|subject)). A carryover effect was not expected as there was a sufficient period between the two follow-up assessments in each condition. The power analysis for non-inferiority regarding the primary outcome parameter with a power of 80% and a significance level of 0.05 was based on a previous trial by Timmermann et al. [19], implementing a standard deviation of 4.3 and a non-inferiority margin of 25%, resulting in a total of 27 patients needed. When considering a dropout rate of 10%, a total of 30 patients had to be recruited. For subgroup analysis (comparison of non-preferred settings) Shapiro-Wilk-tests were conducted to test for normal distribution. Then paired t-test or Wilcoxon signed rank test was employed, respectively. The Bonferroni method was used to correct for multiple comparisons and statistical significance was set to p < 0.05. Results are reported as mean and standard deviation if not indicated otherwise. We used MATLAB R2020a (The MathWorks Inc., Natick, Massachusetts, United States) for all data analysis.
Data availability
The data and MATLAB code used for the present analysis are available via the Open Science Framework (https://osf.io/q23xj/).
RESULTS
Participants
Between August 15, 2019, and December 3, 2020, 30 patients were consecutively enrolled. A total of 24 patients were included in the intention-to-treat analysis (7 female) as 6 patients had to be excluded or dropped out of the trial during the first period of the trial. Fig. 1B depicts the participant flow and reasons for dropout. Patients included in the analysis were 61.4 years (±8.7) old, had a mean disease duration of 8.8 years (±3.8), and were included 0.9 year (±0.8) after surgery. The median Hoehn & Yahr stage was 2.5 (IQR:±1.0) and the mean levodopa equivalent daily dose at study inclusion was 443.5 mg (±214.2). Fig. 2 shows the order of the pulse width settings throughout the trial and the preferred settings at the end of the trial. All patients were implanted with the same implantable pulse generator (GeviaTM, Boston Scientific) and CartesiaTM leads (Boston Scientific) except one patient (Medtronic 3387 leads). Adverse events and stimulation settings for each pulse width condition are reported in Supplementary Tables 1 and 2 .
Fig. 2
Individual Order of Pulse Widths. Before the trial a stimulation setting with 60 μs was chosen in 21 of 24 patients. At the end of the trial 9 of 19 patients completing the trial, preferred a stimulation with 30 μs or 60 μs respectively.
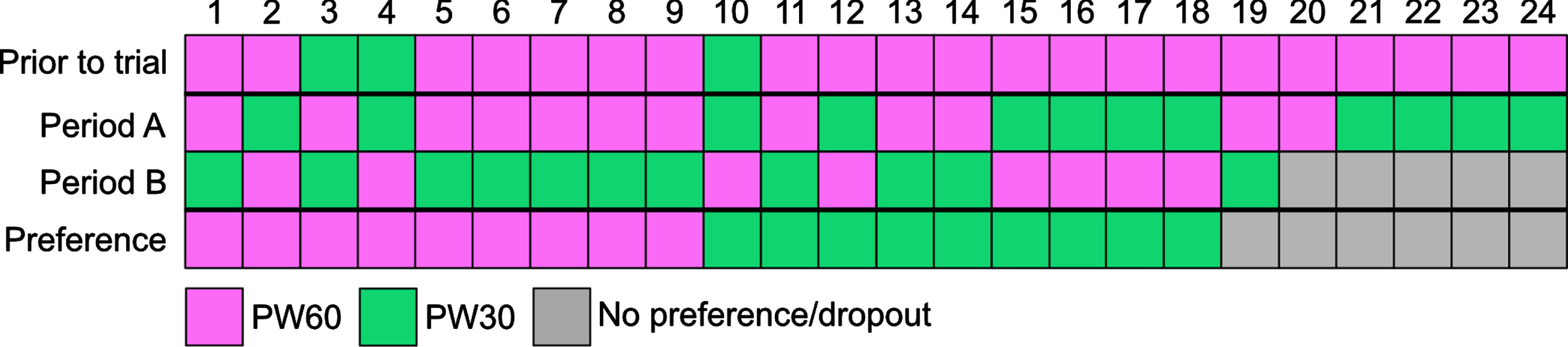
Clinical outcomes
There were no differences regarding the time spent in “On” state (PW60:11.7±4.07 h 90% -CI [10.1 to 13.3]; PW30:11.09±5.08 h, 90% -CI [9.3 to 11.9]; estimate 2.34±3.05, p = 1.0) and all other motor states as investigated by the motor diary between the PW60 and the PW30 setting (see Table 1). Of note, the lower border of the 90% -CI of time spent in “On” state in PW30 was above the non-inferiority margin (see Fig. 1). Additionally, there was no significant period-effect detected (see Supplementary Table 3, data for interaction term not shown). For secondary outcomes, there were no differences between the two treatment conditions for UPDRS-I (PW60:1.5±1.8, PW30:1.3±1.7, p = 1.00), UPDRS-II (PW60:8.7±3.4, PW30:6.6±3.6, p = 0.132), UPDRS-III (PW60:16.9±6.5, PW30:16.1±6.6, p = 1.0), PDQ-39 SI (PW60:64.1±47.3, PW30:59.1±47.7, p = 1.00), and NMSQ (PW60:7.6±3.6, PW30:6.7±4.0, p = 1.00). Regarding side-effects there were no differences for VAS “ability to speak” (PW60:4.8±2.9, PW30:5.7±2.7, p = 1.00), intelligibility ratings (PW60:8.0±3.6, PW30:6.7±4.0, p = 1.00), VAS “ability to walk” (PW60:5.4±2.8, PW30:5.4±2.6, p = 1.00), and 10-m timed walk test (PW60:8.6±1.6 s, PW30:9.3±1.9 s, p = 0.179). Furthermore, to check for a probable bias by the chosen modified intention-to-treat approach, a supplemental analysis only including patients completing both treatment conditions (N = 19) was conducted, demonstrating the same results (see Supplementary Table 4).
Table 1
Motor Diary Outcomes
Motor States [h] | PW60 N = 20 Mean (SD), [90% -CI] | PW30 N = 23 Mean (SD), [90% -CI] | LME PW60 vs. PW 30 N = 24 | PW60 vs. PW30 N = 19 Mean of difference (SD), [90% -CI] | |
Estimate (SE) | p | ||||
“On” | 11.7 (4.07) [10.1 to 13.3] | 11.09 (5.08) [9.3 to 12.9] | 2.34 (3.05) | 1.0 | 0.63 (3.35) [–1.0 to 2.2] |
“Off” | 1.92 (3.6) [1.0 to 3.0] | 1.73 (3.29) [1.4 to 4.2] | –0.26 (2.33) | 1.0 | –0.54 (2.49) [–1.7 to 0.7] |
“Dyskinesia” | 2.02 (2.55) [0.5 to 3.3] | 2.8 (3.83) [0.6 to 2.9] | –1.02 (1.58) | 1.0 | 0.09 (1.73) [–0.7 to 0.9] |
“Sleep” | 8.31 (1.35) [7.8 to 8.8] | 8.48 (1.53) [7.9 to 9.0] | –0.15 (0.75) | 1.0 | –0.23 (0.78) [–0.6 to 0.14] |
Comparison of motor diary outcomes between PW60 and PW 30 condition. For direct comparison of mean of differences (last column) only datasets with assessments in both conditions were included. 90% -CI, 90% confidence interval; SD, standard deviation; SE, standard error; PW30, trial condition with 30 μs; PW60, trial condition with 60 μs.
Fig. 3
Motor Diary Outcomes. A) There were no differences in motor states as on the group level between treatment conditions. B) On the subject level, individual differences between the treatment conditions were observed. Relation of the respective 90% -CI and the non-inferiority margin (dashed line) for the “On” state, the primary outcome parameter, is indicated in the respective subfigure. C) Patients preferring 30 μs (N = 9) seemed to experience more dyskinesia in their non-preferred 60 μs setting (PW60), whereas patients preferring 60 μs (N = 9) tended to have more Off-time in their non-preferred 30 μs setting (PW30). PW30, trial condition with 30 μs; PW60, trial condition with 60 μs.
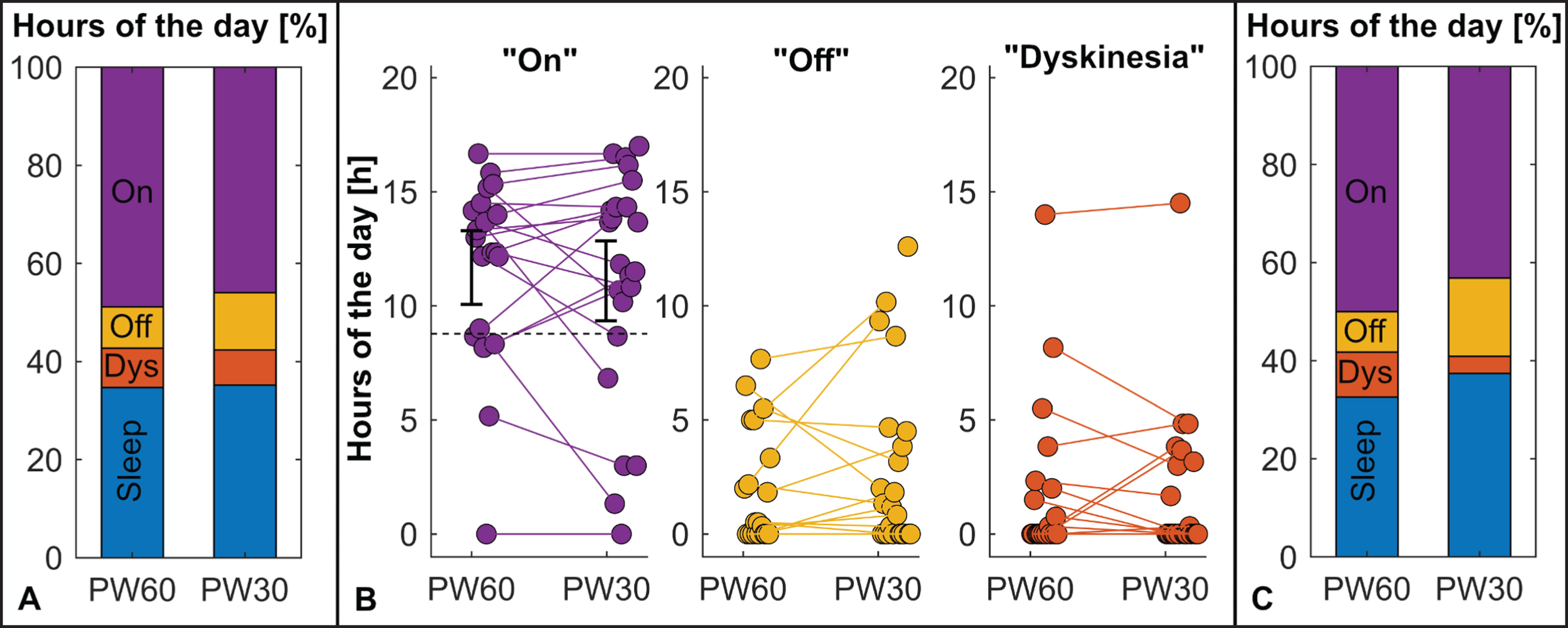
Energy consumption
The applied current was lower in PW60 than in PW30 settings (PW60:4.5±2.0 mA, PW30:6.9±3.5 mA, p < 0.001). As shown in Fig. 4, there were differences between the PW60 and PW30 setting, concerning CPP (PW60:271.80±118.86 nC, PW30:206.87±103.67 nC, p < 0.001) but neither for TEED (PW60:73.56±45.17 μJ/s, PW30:92.22±66.46 μJ/s, p = 0.052) nor BCI (PW60:38.88±20.98 μJ, PW30:47.05±31.25 μJ, p = 0.124).
Fig. 4
Energy Indices. A) In PW30 condition higher amplitudes were applied than in PW60 condition. B) The PW30 condition resulted in a lower charge per pulse, whereas the total electrical energy delivered (C), and the battery drain were not different between the two conditions (D). (*p < 0.001) BCI, Battery Charge Index; CPP, charge per pulse; PW30, trial condition with 30 μs; PW60, trial condition with 60 μs; TEED, total electrical energy delivered.
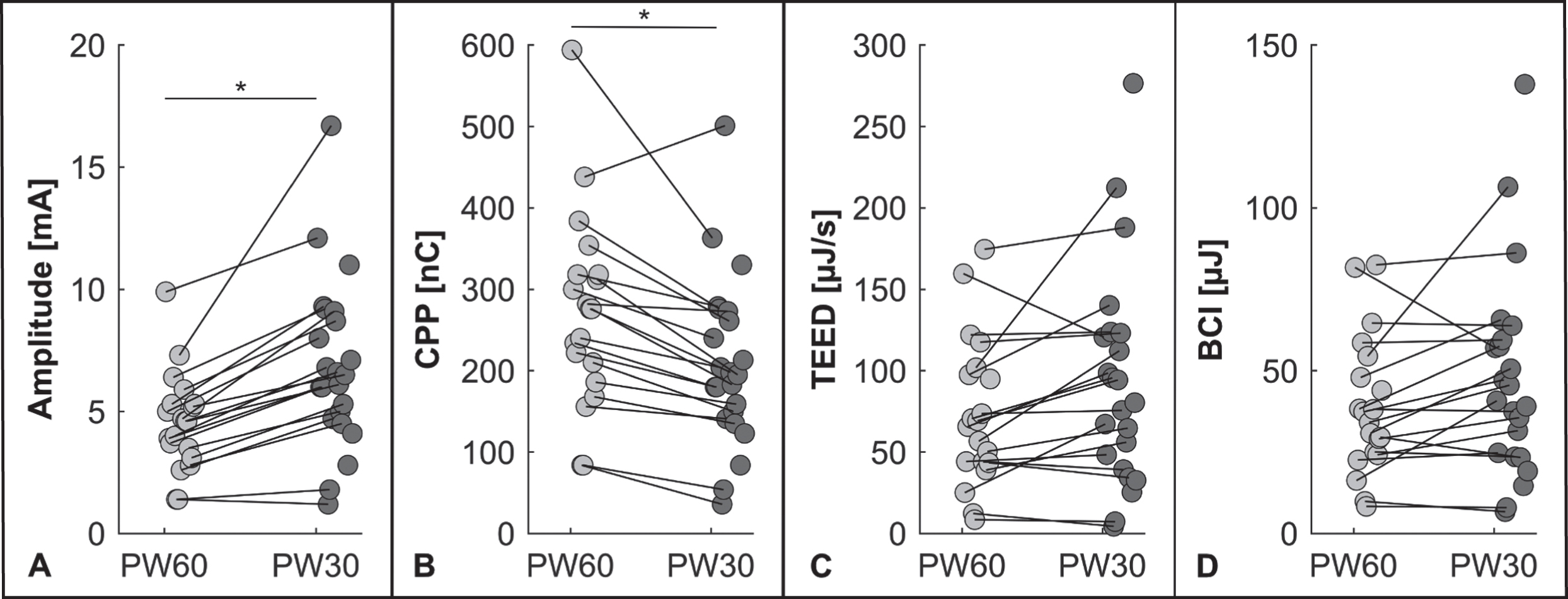
DISCUSSION
In this double-blind, crossover trial in patients with PD and bilateral STN-DBS, motor symptom control was not significantly different for short and conventional pulse widths. Additionally, there were no differences regarding any investigated side-effect, non-motor symptoms, quality-of-life, or energy consumption measures.
While most of the previous studies focused on an acute challenge— and often limited their analysis to differences in amplitude thresholds— the present study reports a comparison of motor symptom control after 4 weeks of continuous stimulation between the two pulse width conditions [10–12]. In line with a previous study by Dayal et al. [13], our study provides evidence for a non-inferiority regarding motor symptom control and an acceptable safety profile of STN-DBS with a pulse width of 30 μs. Unlike the present study, Dayal et al. only included patients with stimulation-induced dysarthria and thereby stimulation settings had already been adapted to this troublesome side-effect, which can lead to suboptimal motor symptom control. Additionally, in the present study, motor symptom control in each condition was evaluated over three days using a standardized motor diary, providing a home-based overall impression of motor symptom control under everyday conditions [18]. This might especially be important in patients with advanced PD, as motor fluctuations throughout the day could influence subjective motor outcomes and might not be captured by frequently used scales as, e.g., the UPDRS in a single visit [2, 18, 19].
Subjectively, 47.5% of the patients (N = 9/19), tested in both conditions, preferred either the short (30 μs) or the conventional (60 μs) setting (see Fig. 2). This individual preference has also been reported by Seger et al. regarding gait outcomes in short versus conventional pulse width settings [16]. Although the group size did not allow for distinct subgroup analysis, the results presented here carefully point towards different patient profiles regarding preferences of pulse width settings. While patients preferring 30 μs spent more time with troublesome dyskinesia in their non-preferred setting with 60 μs, patients preferring 60 μs experienced more time with bradykinesia throughout the day in their non-preferred 30 μs setting (see Fig. 3C and Supplementary Table 5). This concept is supported by a recent study by Dayal et al. demonstrating an alleviation of dyskinesia by stimulation with 30 μs in an acute challenge [15]. Of note, there was no difference in percentual change of current applied in the respective pulse width setting when comparing both preference groups (see Supplementary Table 5). Therefore, it seems unlikely that this effect is solely based on an inadvertently insufficient current titration during the trial and indeed might be related to the applied pulse width.
An often-discussed benefit of short pulse width stimulation is its reduced charge per pulse. Previous studies hypothesized, that this might result in fewer side-effects and increased battery life [10–12]. The potential benefit of preventing stimulation-induced side effects has mainly been derived from an increase of the therapeutic window in terms of a widening of the amplitude range between side-effect and efficacy threshold [11–14, 22]. Beneficial effects of short pulse width stimulation on side-effects have only been reported in DBS for essential tremor patients [23], but not in patients with PD beyond the setting of an acute challenge [13, 14]. Although Reich et al. concluded that a wider therapeutic window might result from selectivity of short pulse widths for smaller, beneficial axons over larger, side-effect associated fibers [10], this hypothesis has been falsified in prior and current studies [22, 24]. Instead, stimulation with shorter pulse widths might lead to a preferential activation of larger axons with shorter chronaxies, as demonstrated by Anderson et al. [22]. Regarding battery life, it has to be clarified that it critically depends on energy consumption and not only charge. Therefore we additionally calculated the TEED and the BCI, an energy index also used to indicate energy consumption of stimulation settings on the programming device per clinical routine [21]. Both energy consumption measures were equal for the two conditions. This demonstrates that stimulating with 30 μs has no beneficial effect on battery life and is in line with a previous study reporting equivalency in TEED when comparing both settings at the efficacy threshold in an acute challenge [14]. It also highlights the important fact, that when only taking one of those parameters into account, e.g., current, charge, TEED or BCI, different results regarding its energy consumption can be obtained by definition when comparing 30 μs to 60 μs stimulation.
The main limitation of the present trial is that the calculated sample size of N = 27 to show non-inferiority of short pulse width stimulation with a power of 80% has not been reached due to an unexpected high number of dropouts during the trial. These dropouts reflect the difficulty of conducting a clinical trial during the COVID-19 pandemic, which is why visits in between the follow-up visits were kept to a minimum and patients were mainly supervised via telephone. Additionally, the possibility to change stimulation settings via the patient programmer was another source of dropouts, as two patients switched back to the previous condition’s setting instead of titrating the current. However, in line with our clinical routine, we discharged patients with a well-established “backup” stimulation setting for patient safety reasons. Nevertheless, the study size seems sufficient as not even a trend for a difference in motor symptom control between the study conditions was observed despite inclusion of round about 80% of the calculated sample size (all p = 1.0, see Table 1). Of note, the sample size is one of the biggest in studies investigating the effect of short pulse widths in STN-DBS. Another consequence of the dropouts was that the planned 1:1 randomization could not be achieved. However, this seems not to influence the results as no period-effect was observed. Secondly, the choice of the stimulation settings was based on a single non-standardized clinical programming session instead of a standardized monopolar review as it has been part of a similar trial by Dayal et al. [13] As mentioned before, Dayal et al. included patients with stimulation-induced dysarthria in contrast to the present study. Therefore, every patient needed an elaborated adjustment of the stimulation setting before study inclusion, which was not the case in the present cohort. Another difference to prior studies is that mainly patients themselves titrated the current. To prevent potentially suboptimal stimulation settings [25], patients were well trained at baseline and reinstructed as per protocol telephone calls if motor symptom control was insufficient or side-effects were reported. Overall real-life outcomes may even be better reflected by this practice, as it is unlikely that patients present to an outpatient clinic for stimulation adjustment regularly within such a short time frame.
To conclude, the present study provides evidence that there is no difference concerning motor symptom control, the occurrence of side-effects or energy consumption between short and conventional pulse width stimulation in STN-DBS for patients with PD on the group level when tested under real-life conditions. However, future studies are warranted with larger patient samples to investigate whether patients with distinct symptom burden profiles, especially dyskinesia, might benefit from shorter pulse width stimulation as an individualized treatment option.
ACKNOWLEDGMENTS
We thank our patients for contributing to this study. JNPS and TAD were supported by the Cologne Clinician Scientist Program (CCSP)/ Faculty of Medicine/ University of Cologne. Funded by the German Research Foundation (DFG, FI 773/15-1). JCB and VVV were funded by the Deutsche Forschungsgemeinschaft, German Research Foundation (Project-ID: 431549029 –SFB 1451).
CONFLICT OF INTEREST
The authors have no conflict of interest to report.
SUPPLEMENTARY MATERIAL
[1] The supplementary material is available in the electronic version of this article: https://dx.doi.org/10.3233/JPD-213119.
REFERENCES
[1] | Jost ST , Sauerbier A , Visser-Vandewalle V , Ashkan K , Silverdale M , Evans J , Loehrer PA , Rizos A , Petry-Schmelzer JN , Reker P , Fink GR , Franklin J , Samuel M , Schnitzler A , Barbe MT , Antonini A , Martinez-Martin P , Timmermann L , Ray-Chaudhuri K , Dafsari HS , EUROPAR and the International Parkinson and Movement Disorders Society Non-Motor Parkinson’s Disease Study Group ((2020) ) A prospective, controlled study of non-motor effects of subthalamic stimulation in Parkinson’s disease: Results at the 36-month follow-up. J Neurol Neurosurg Psychiatry 91: , 687–694. |
[2] | Schuepbach WMM , Rau J , Knudsen K , Volkmann J , Krack P , Timmermann L , Hälbig TD , Hesekamp H , Navarro SM , Meier N , Falk D , Mehdorn M , Paschen S , Maarouf M , Barbe MT , Fink GR , Kupsch A , Gruber D , Schneider G-H , Seigneuret E , Kistner A , Chaynes P , Ory-Magne F , Brefel Courbon C , Vesper J , Schnitzler A , Wojtecki L , Houeto J-L , Bataille B , Maltête D , Damier P , Raoul S , Sixel-Doering F , Hellwig D , Gharabaghi A , Krüger R , Pinsker MO , Amtage F , Régis J-M , Witjas T , Thobois S , Mertens P , Kloss M , Hartmann A , Oertel WH , Post B , Speelman H , Agid Y , Schade-Brittinger C , Deuschl G ((2013) ) Neurostimulation for Parkinson’s disease with early motor complications. N Engl J Med 368: , 610–622. |
[3] | Kleiner-Fisman G , Herzog J , Fisman DN , Tamma F , Lyons KE , Pahwa R , Lang AE , Deuschl G ((2006) ) Subthalamic nucleus deep brain stimulation: Summary and meta-analysis of outcomes. Mov Disord 21: , S290–S304. |
[4] | Petry-Schmelzer JN , Krause M , Dembek TA , Horn A , Evans J , Ashkan K , Rizos A , Silverdale M , Schumacher W , Sack C , Loehrer PA , Fink GR , Fonoff ET , Martinez-Martin P , Antonini A , Barbe MT , Visser-Vandewalle V , Ray-Chaudhuri K , Timmermann L , Dafsari HS ;EUROPAR and the IPMDS Non-Motor PD Study Group ((2019) ) Non-motor outcomes depend on location of neurostimulation in Parkinson’s disease. Brain 142: , 3592–3604. |
[5] | Dembek T , Baldermann JC , Petry-Schmelzer J , Jergas H , Treuer H , Visser-Vandewalle V , Dafsari H , Barbe M (2020) Sweetspot mapping in deep brain stimulation: Strengths and limitations of current approaches. Neuromodulation, doi: 10.1111/ner.13356. |
[6] | Horn A , Reich M , Vorwerk J , Li N , Wenzel G , Fang Q , Schmitz-Hübsch T , Nickl R , Kupsch A , Volkmann J , Kühn AA , Fox MD ((2017) ) Connectivity predicts deep brain stimulation outcome in Parkinson disease. Ann Neurol 82: , 67–78. |
[7] | Irmen F , Horn A , Mosley P , Perry A , Petry-Schmelzer JN , Dafsari HS , Barbe M , Visser-Vandewalle V , Schneider G-H , Li N , Kübler D , Wenzel G , Kühn AA ((2020) ) Left prefrontal connectivity links subthalamic stimulation with depressive symptoms. Ann Neurol 87: , 962–975. |
[8] | Moro E , Esselink RJA , Xie J , Hommel M , Benabid AL , Pollak P ((2002) ) The impact on Parkinson’s disease of electrical parameter settings in STN stimulation. Neurology 59: , 706–713. |
[9] | Rizzone M , Lanotte M , Bergamasco B , Tavella A , Torre E , Faccani G , Melcarne A , Lopiano L ((2001) ) Deep brain stimulation of the subthalamic nucleus in Parkinson’s disease: Effects of variation in stimulation parameters. J Neurol Neurosurg Psychiatry 71: , 215–219. |
[10] | Reich MM , Steigerwald F , Sawalhe AD , Reese R , Gunalan K , Johannes S , Nickl R , Matthies C , McIntyre CC , Volkmann J ((2015) ) Short pulse width widens the therapeutic window of subthalamic neurostimulation. Ann Clin Transl Neurol 2: , 427–432. |
[11] | Bouthour W , Wegrzyk J , Momjian S , Péron J , Fleury V , Tomkova Chaoui E , Horvath J , Boëx C , Lüscher C , Burkhard PR , Krack P , Zacharia A ((2018) ) Short pulse width in subthalamic stimulation in Parkinson’s disease: A randomized, double-blind study. Mov Disord 33: , 169–173. |
[12] | Steigerwald F , Timmermann L , Kühn A , Schnitzler A , Reich MM , Kirsch AD , Barbe MT , Visser-Vandewalle V , Hübl J , van Riesen C , Groiss SJ , Moldovan A-S , Lin S , Carcieri S , Manola L , Volkmann J ((2018) ) Pulse duration settings in subthalamic stimulation for Parkinson’s disease. Mov Disord 33: , 165–169. |
[13] | Dayal V , Grover T , Tripoliti E , Milabo C , Salazar M , Candelario-McKeown J , Athauda D , Zrinzo L , Akram H , Hariz M , Limousin P , Foltynie T ((2020) ) Short versus conventional pulse-width deep brain stimulation in Parkinson’s disease: A randomized crossover comparison. Mov Disord 35: , 101–108. |
[14] | Dayal V , Grover T , Limousin P , Akram H , Cappon D , Candelario J , Salazar M , Tripoliti E , Zrinzo L , Hyam J , Jahanshahi M , Hariz M , Foltynie T ((2018) ) The effect of short pulse width settings on the therapeutic window in subthalamic nucleus deep brain stimulation for Parkinson’s disease. J Parkinsons Dis 8: , 273–279. |
[15] | Dayal V , De Roquemaurel A , Grover T , Ferreira F , Salazar M , Milabo C , Candelario-McKeown J , Zrinzo L , Akram H , Limousin P , Foltynie T ((2020) ) Novel programming features help alleviate subthalamic nucleus stimulation-induced side effects. Mov Disord 35: , 2261–2269. |
[16] | Seger A , Gulberti A , Vettorazzi E , Braa H , Buhmann C , Gerloff C , Hamel W , Moll CKE , Pötter-Nerger M ((2021) ) Short pulse and conventional deep brain stimulation equally improve the parkinsonian gait disorder. J Parkinsons Dis 11: , 1455–1464. |
[17] | Lang AE , Houeto J-L , Krack P , Kubu C , Lyons KE , Moro E , Ondo W , Pahwa R , Poewe W , Tröster AI , Uitti R , Voon V ((2006) ) Deep brain stimulation: Preoperative issues. Mov Disord 21: , S171–S196. |
[18] | Hauser RA , Friedlander J , Zesiewicz TA , Adler CH , Seeberger LC , O’Brien CF , Molho ES , Factor SA ((2000) ) A home diary to assess functional status in patients with Parkinson’s disease with motor fluctuations and dyskinesia. Clin Neuropharmacol 23: , 75–81. |
[19] | Timmermann L , Jain R , Brücke T , Seijo F , Martin ESS , Haegelen C , Verin M , Maarouf M , Barbe M , Gill S , Whone A , Porta M , Servello D , Alesch F ((2015) ) VANTAGE Trial: A prospective, multi-center trial evaluating deep brain stimulation with a new multiple-source, constant-current rechargeable system in Parkinson’s disease (P1.173). Neurology 84: (14 Suppl), P1.173.. |
[20] | Deuschl G , Schade-Brittinger C , Krack P , Volkmann J , Schäfer H , Bötzel K , Daniels C , Deutschländer A , Dillmann U , Eisner W , Gruber D , Hamel W , Herzog J , Hilker R , Klebe S , Kloss M , Koy J , Krause M , Kupsch A , Lorenz D , Lorenzl S , Mehdorn HM , Moringlane JR , Oertel W , Pinsker MO , Reichmann H , Reuss A , Schneider G-H , Schnitzler A , Steude U , Sturm V , Timmermann L , Tronnier V , Trottenberg T , Wojtecki L , Wolf E , Poewe W , Voges J , German Parkinson Study Group Neurostimulation Section ((2006) ) A randomized trial of deep-brain stimulation for Parkinson’s disease. N Engl J Med 355: , 896–908. |
[21] | Juárez-Paz LM ((2020) ) In silico accuracy and energy efficiency of two steering paradigms in directional deep brain stimulation. Front Neurol 11: , 593798. |
[22] | Anderson CJ , Anderson DN , Pulst SM , Butson CR , Dorval AD ((2020) ) Neural selectivity, efficiency, and dose equivalence in deep brain stimulation through pulse width tuning and segmented electrodes. Brain Stimul 13: , 1040–1050. |
[23] | Kroneberg D , Ewert S , Meyer A-C , Kühn AA ((2019) ) Shorter pulse width reduces gait disturbances following deep brain stimulation for essential tremor. J Neurol Neurosurg Psychiatry 90: , 1046–1050. |
[24] | Gorman PH , Mortimer JT ((1983) ) The effect of stimulus parameters on the recruitment characteristics of direct nerve stimulation. IEEE Trans Biomed Eng 30: , 407–414. |
[25] | Oliveira LM , Ruiz-Lopez M , Boutet A , Elias GJB , Kalia SK , Hodaie M , Lozano AM , Munhoz RP , Fasano A ((2021) ) Self-adjustment of deep brain stimulation delays optimization in Parkinson’s disease. Brain Stimul 14: , 676–681. |