Gene Therapy to Modulate Alpha-Synuclein in Synucleinopathies
Abstract
The protein alpha-Synuclein (α-Syn) is a key contributor to the etiology of Parkinson’s disease (PD) with aggregation, trans-neuronal spread, and/or depletion of α-Syn being viewed as crucial events in the molecular processes that result in neurodegeneration. The exact succession of pathological occurrences that lead to neuronal death are still largely unknown and are likely to be multifactorial in nature. Despite this unknown, α-Syn dose and stability, autophagy-lysosomal dysfunction, and inflammation, amongst other cellular impairments, have all been described as participatory events in the neurodegenerative process. To that end, in this review we discuss the logical points for gene therapy to intervene in α-Syn-mediated disease and review the preclinical body of work where gene therapy has been used, or could conceptually be used, to ameliorate α-Syn induced neurotoxicity. We discuss gene therapy in the traditional sense of modulating gene expression, as well as the use of viral vectors and nanoparticles as methods to deliver other therapeutic modalities.
BACKGROUND
Parkinson’s disease (PD) is a neurodegenerative disorder clinically characterized by cardinal motor symptoms which can be attributed to the loss of striatal dopaminergic tone and subsequent loss of dopaminergic neurons in the substantia nigra. Postmortem evaluation of PD patient brains has revealed the presence of proteinaceous cytosolic inclusions, termed Lewy bodies (LB), and thread-like fibrils in cellular processes, termed Lewy neurites (LN), in neurons throughout various brain regions. Genetic studies linked the protein alpha-Synuclein (α-Syn) to familial forms of the disease and subsequent studies identified α-Syn as a major component of LBs [1]. Moreover, point mutations and gene multiplications of SNCA alter the aggregation potential of α-Syn and cause PD in a dose-dependent manner [2, 3], thus, indisputably linking α-Syn to the disease process.
The exact function(s) of α-Syn is still largely unknown. α-Syn, located in synaptic terminals and neuronal nuclei in the central and peripheral nervous systems is typically viewed as a neuronal protein involved in neurotransmission. However, the protein is also expressed in a variety of non-neuronal tissues including cells from a hematopoietic origin [4], suggesting a function that extends beyond the nervous system. Under normal conditions α-Syn is a natively unfolded and soluble monomer, with its existence as a tetramer debated [5, 6]. However, during the process of pathogenesis, α-Syn misfolds and forms aggregates along with other proteins, forming LN and LB, collectively referred to as Lewy pathology (LP). Aberrant neuronal accumulation of α-Syn has also been identified in dementia with Lewy bodies (DLB), and oligodendroglial accumulation of α-Syn is seen in multiple system atrophy (MSA) (reviewed further in [7, 8]). How the same protein is able to cause different diseases in different cell types still remains unclear; however, the link of α-Syn aggregation has collectively united these diseases under the umbrella term synucleinopathies and highlights α-Syn as a central therapeutic target [9].
α-SYNUCLEIN IN PD PATHOLOGY
By comparing the brains from patients presenting a spectrum of PD-associated symptoms and LP, Braak and colleagues conceived a model for the etiology of PD, in which the LP begins in the brainstem and/or olfactory bulb and extends rostral or caudally to other brain regions over time [10]. This model led to the creation of the Braak hypothesis, which in its latest iteration proposes that α-Syn pathology of PD starts in the gut (possibly initiated by an unknown pathogen) or the olfactory bulb and spreads towards, and within, the CNS in a prescribed temporal and spatial distribution. As pathology progresses, neuronal dysfunction, possibly related to α-Syn pathology per se, gives result to prodromal symptoms, and eventually classical parkinsonism ensues following the involvement of the basal ganglia. This idea has given rise to several lines of research: 1) PD prodrome; or the presence of premotor disease (i.e., Braak Stages 1 and 2); 2) PD co-pathology; the extent to which α-Syn pathology is informed by pathologies other than LP (e.g., tau [11]), and 3) PD as a prion-like disorder. Nonetheless, one commonality that ties these areas of research together is the presence of α-Syn pathology in one form or another.
RATIONALE FOR GENE THERAPY-MEDIATED TARGETING α-SYN IN DISEASE: TOXIC GAIN-OF-FUNCTION
A prion-like misfolding [12] and propagation of α-Syn non-monomeric species that eventually become insoluble aggregates is proposed as an event necessary for neurodegeneration [13]. The idea of active propagation has been supported by studies showing that injections of α-Syn fibrils can recruit adjacent neurons along the olfactory tract [14] or vagus nerve [15, 16]. With propagation proposed to occur through exosomes or via neuronal release and endocytosis, directly between neurons, as well as through the involvement of microglia [17]. However, the results as it relates to the propagation of α-Syn from the periphery per se have been mixed [18–20], with some studies reporting absent or transient pathology, and most studies demonstrating sustained CNS pathology following a peripheral inoculation requiring an additional “hit” such as a mutant α-syn transgenic background [21].To this end, it is important to note the limitations of these animal models, as they do not reflect “normal” PD pathophysiology in the sense that an injection of supraphysiological concentrations of α-Syn is required [17].
Although the exact role of α-Syn in disease remains largely unknown, the chief presumption is that α-Syn aggregation causes neurodegeneration via a direct toxic gain-of function. Recent work has highlighted that not fibrils per se, but the entire process of LB formation-including fibrilization, posttranslational modifications, and interaction with membranous organelles is the key driver of toxicity, by disrupting essential cellular functions and inducing synaptic dysfunction, as well as mitochondrial toxicity [22]. As such, a variety of therapeutic modalities have been conceived to target α-Syn protein levels and aggregate formation.
GENE THERAPY INTERVENTIONS BASED ON α-SYN
Based on the assumption that α-Syn pathology is a cause and not a consequence of disease, anti-Synuclein strategies have emerged as the indisputable disease-modifying therapeutic strategy, akin to a similar framework of anti-amyloid interventions in Alzheimer’s disease. The overarching goal aims to reduce the load of α-Syn pathology through a variety of means-either by targeting the process of aggregation or by targeting the consequences of aggregation. Thus far, the only clinical anti-Synuclein strategies have utilized active or passive immunization [23, 24]. Although available results to date have failed to meet the primary objective, the analysis of secondary outcome measures have signaled improvement. Nonetheless, numerous modalities aimed at utilizing gene therapy to target α-Syn pathology are in various stages of preclinical investigation.
In the strictest sense, gene therapy is defined as technique that modifies an individual’s genetic makeup to treat or cure disease. Below we discuss α-Syn gene therapy strategies based on existing preclinical gene therapy studies, as well as considering conceptual strategies based on basic research into α-Syn biology.
As depicted in Fig. 1, there are several logical points whereby one could utilize gene therapy to target α-Syn pathology: 1) Target extracellular α-Syn (the presumptive prion pathogen) using immunotherapy; 2) Blocking or reducing expression of receptors that may facilitate cell-to-cell propagation; 3) Use RNAi or similar technologies to decrease overall levels of α-Syn; 4) Utilize strategies that stabilize the monomeric (functional) form of the protein or enhance clearance of aggregated protein; 5) Promote cellular processes that are impaired due to α-Syn aggregation; 6) Target inflammation; 7) A toxic loss-of function hypothesis will be discussed in detail below, but with this idea in mind, one therapeutic approach may be to maintain monomeric forms of the protein.
Fig. 1
Conceptual points of intervention in α-Syn gene therapy. There are numerous conceptual points of gene therapy intervention aimed at preventing or ameliorating toxic effects that arise as a result of α-Syn oligomerization or depletion in disease. 1) A growing body of data suggests a peripheral origin of Lewy pathology which spreads rostrally to the CNS, and thereafter throughout the PD brain. Although the exact mechanism by which this occurs is still unknown, it is thought to involve extracellular α-Syn which would serve as a substrate for immunotherapy. 2) The surface proteins LAG3, TLR2, and Cx32 can interact with α-Syn and may mediate neuronal uptake of pathological forms of the protein. Accordingly, targeting these receptors either via immunotherapy or genetically (e.g., via RNAi) is a potential means to prevent trans-neuronal spread of pathology. 3) A chief strategy thus far has been to utilize various genetic means such as anti-sense oligonucleotides, RNA interference, or CRISPR-based technology to lower the overall dose of the protein and thus reducing the ability of α-Syn to aggregate. 4) A second approach to reduce aggregation is to directly stabilize the monomeric, soluble, form of α-Syn using chaperones or intrabodies. Along the same lines, enhancing the clearance of intracellular α-Syn aggregates via the enhancement of autophagy/lysosomal function, can also serve as to minimize the degree of aggregation. 5) Finally, in the process of aggregation the soluble pool of α-Syn is depleted, resulting in a potentially toxic loss-of function. Supplementation of non-aggregatable forms of the protein can then be introduced to restore crucial protein function. Used with permission from Barrow Neurological Institute, Phoenix, Arizona.
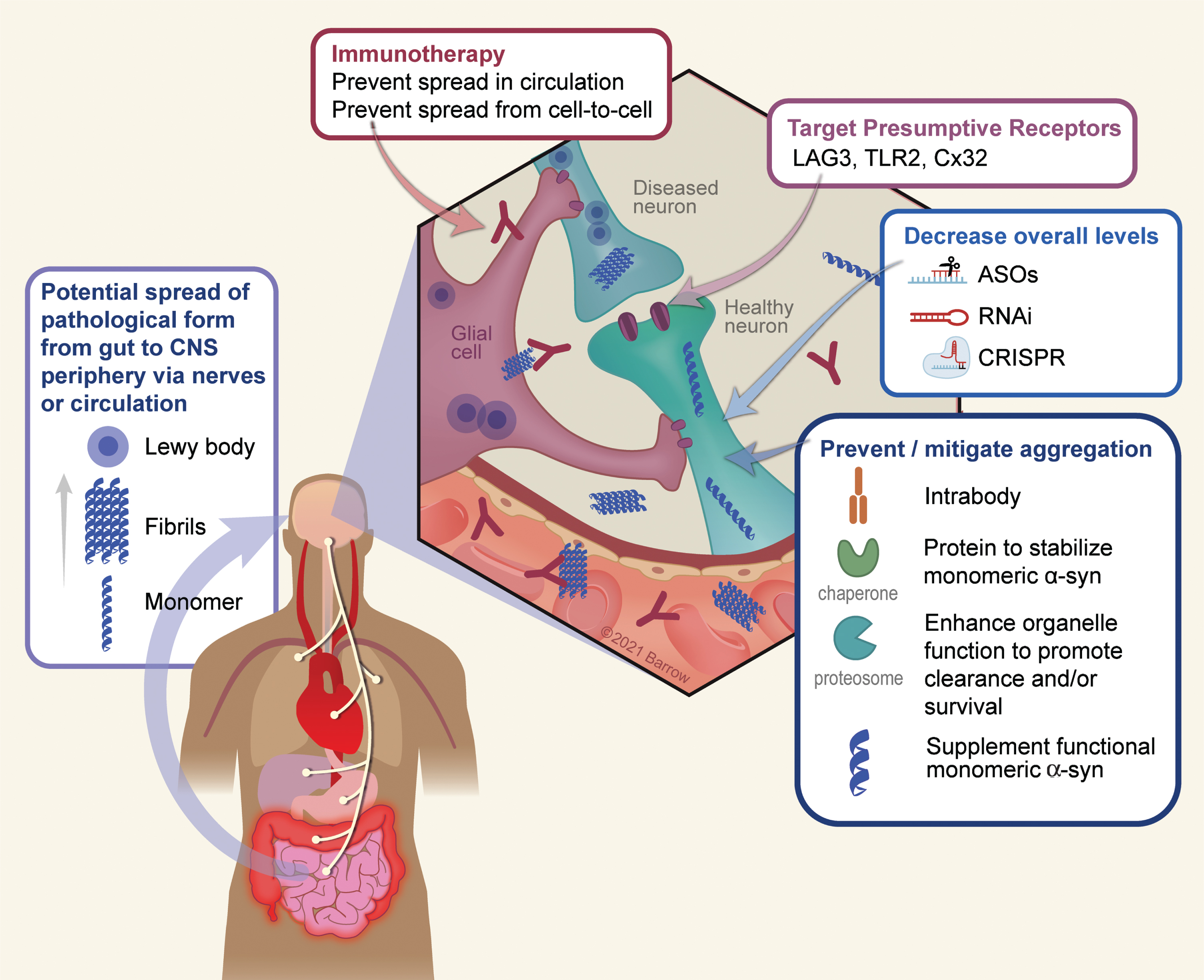
GENE THERAPY TO PREVENT α-SYN PATHOLOGY PROPAGATION
As mentioned above, the main approach to target extracellular α-Syn, and thus cell-to-cell spread, has taken place via classic immunotherapy [23]. However, vectorized immunotherapy, in which antibodies are directly delivered to the CNS using viral vectors, is being explored in attempts to enhance target engagement within the parenchyma. This approach is under development by Voyager Therapeutics, although the status of these studies is unknown. Nonetheless, with the advent and success of mRNA vaccines in the era of COVID-19, similar immune-based gene therapy approaches targeting α-Syn are likely to follow. Moreover, the use of gene therapy to directly produce intrabodies within target cells holds significant promise in reducing α-Syn related pathology [25, 26].
The exact cell surface receptor(s) involved in fibril internalization are unknown, but some potential candidates for anti-propagation therapies have been proposed: Connexin-32 (Cx32) [27], Toll-Like receptor 2 (TLR2) [28], and Lymphocyte-activation gene 3 (LAG3) [29], although the relevance of the latter in neurodegeneration is debated [30]. These proteins represent viable gene therapy targets by either RNA interference (RNAi) or CRISPR-based approaches to ameliorate symptoms of neurodegeneration.
GENE THERAPY TO REDUCE EXPRESSION OF α-SYN
Targeted gene silencing of α-Syn has been performed with various approaches including antisense oligonucleotides (ASOs), short interfering RNA (siRNAs), short hairpin RNA (shRNA) and zinc finger nucleases, with both liposomal and viral vectors as the delivery modality [31–34]. More recently, CRISPR-based technologies have also been utilized to modulate α-Syn expression via transcriptional regulation through the endonuclease deficient dCas9-based system [35]. While, many studies suggest α-Syn silencing may prove beneficial [31, 36–38] in preventing α-Syn toxicity a number of studies from our group, in rodent and non-human primates, show nigrostriatal degeneration as a direct result of α-Syn knockdown [32, 39, 40]. The source of this discrepancy is unknown but may be attributed towards compensatory increases in β-Syn and γ-Syn levels, which may share a common redundant function with α-Syn [41]. The compensatory effect of these proteins may be facilitated by differences in the kinetics and duration of α-Syn suppression (e.g., contrasting the work by Benskey [32] with that of Zharikov [36], the former describing a much more rapid removal of nigrostriatal α-Syn and nigrostriatal degeneration). Thus, the strategy of reducing the soluble form of α-Syn should carefully consider the potential toxic effects of an excessive reduction of the physiological levels of α-Syn below a certain threshold necessary for normal function (see more below).
GENE THERAPY STRATEGIES TO STABILIZE THE MONOMERIC (FUNCTIONAL) FORM OF THE PROTEIN OR ENHANCE CLEARANCE OF AGGREGATED PROTEIN
The stabilization of monomeric species of α-Syn, or the breakdown of fibrils are possible strategies to reduce α-Syn aggregation, ultimately protecting the soluble pool of this peptide. This has been achieved by different molecules, such as catecholamines, natural phenols, or synthetic compounds [42–44]. In essence, this approach exploits the intrinsic biochemical characteristics and the complex structure of α-Syn. These include binding to the negatively charged C-terminus domain (e.g., catecholamines), or interference with the intramolecular long-range interaction between N- and C-terminus by N-terminus residues (e.g., CLR01), or binding to the hydrophobic sites of the oligomeric species (e.g., Anle138b) [45–48]. A direct effect of stabilizing the monomeric form of the protein provides an environment that thermodynamically favors soluble α-Syn and leads to the amelioration or prevention of the α-Syn nucleation process [43, 44].
Although small molecules have been in the forefront of stabilizing monomeric α-Syn, several gene therapy candidates, which may serve analogous functions, have emerged. Intrabodies (nanobodies) engineered against the non-amyloidogenic portion (NAC) of α-Syn can both inhibit misfolding, as well as enhance the clearance of the protein, reducing toxicity both in in vitro and in vivo synucleinopathy models [25, 26]. Similarly, overexpression of chaperones such as HSP-70 can ameliorate α-Syn-mediated toxicity, presumably by preventing fibril formation [49–51].
Impairments in the autophagy-lysosomal pathway (ALP) have been linked to PD. The most common genetic risk factors for PD are Gaucher disease variants (GBA), which cause the loss of function of the lysosomal glucocerebrosidase enzyme (GCase) [52]. In vitro, the accumulated GCase substrate, glycosylceramide, acts like a scaffold promoting the aggregation of α-Syn oligomers and fibrils [53]. Conversely, α-Syn pathology can inhibit GCase activity [54], thus, suggesting a feed-forward loop linking α-Syn and GCase [55]. To this end, numerous gene therapy-based approaches have been employed to improve ALP function. Overexpression of GCase itself can reduce α-Syn aggregation and toxicity [56, 57]. Similarly, overexpression of proteins aimed at strengthening ALP such as: Beclin-1 [58], the transcription factor EB (TFEB; a key regulator of ALP) [59], and LAMP2a [60] reduces nigral toxicity in various models of α-Syn pathology. Moreover, the microRNA miR-124 regulates numerous genes involved in ALP, and its expression is deregulated in PD. Accordingly, ectopic modulation of this microRNA is viewed as a potential modality in targeting α-Syn pathology [61, 62].
GENE THERAPY FOR UNIFORMITY ALLEVIATE INFLAMMATION
Several reports implicate the immune system in the pathophysiology of sporadic PD [63, 64]. Microglial activation [65, 66], T-cell activation [67], and increased inflammatory cytokine production [68], are documented in sporadic PD patients, as well as in MSA animal models and MSA patients [69]. Several factors including genetics, and infectious agents can trigger an immune-mediated inflammatory response. Activated microglia can stimulate T-cells to produce and directly release inflammatory cytokines including L-1β, IL-2, IL-6, EGF, and TGF-α and TGF-β [67]. The results of such inflammatory processes are now thought to directly contribute towards neurodegeneration. Moreover, the neurodegenerative process per se could activate microglia and further exacerbate neuronal death [66]. Once the degenerative neuroinflammatory process starts, the blood-brain barrier is weakened, becoming permeable to peripheral immune cells. This amplifies the inflammatory response and facilitates the transition toward a chronic inflammatory state. The large body of work that is immunology confers numerous potential gene therapy targets: One can envision to manipulate proteins that are 1) directly involved in α-Syn-mediated inflammatory processes, or 2) those that are general participants in inflammation. For example, overexpression of fractalkine [70] or a dominant negative form of TNF [71] attenuates microglial activation, and protects against α-Syn overexpression or treatment with the parkinsonian 6-OHDA toxin, respectively. Despite a conceivably long list of potential genetic targets in neuroinflammation, few studies have used gene therapy to manipulate these targets. One reason may reside in the difficulty in targeting immune cells in the brains using conventional vectors. For example, microglial cells remain relatively refractory to infection with common viral vectors. Furthermore, it is becoming increasingly appreciated that subpopulations of glia exist within diseased tissue, exhibiting divergent roles (e.g., neuroprotective versus neurotoxic) in the disease process. Targeting such glial subpopulations will thus require a new generation of viral vectors with much improved precision and efficacy.
SUPPLEMENTING SOLUBLE α-SYN TO RETAIN FUNCTION: THE LOSS-OF FUNCTION HYPOTHESIS
The chief preclinical focus in PD is directed towards the direct toxic effect of α-Syn aggregation, while very little attention has been given to potential toxic effects resulting from α-Syn depletion due to aggregation; namely the loss-of-function (LOF) hypothesis. As described above, multiple studies have demonstrated toxic effects due to rapid α-Syn removal in PD-susceptible populations of neurons. Moreover, several lines of evidence show that monomeric soluble α-Syn is depleted during the process of aggregation resulting in a de facto physical LOF [72, 73], where the monomers are sequestered in a non-native conformation within solid amyloid fibrils. Despite the difference in mechanism, both genetic and physical LOF will confer the same pathophysiological consequences as a result of α-Syn depletion.
Despite the lack of universal agreement on its precise function, a wealth of studies implicates a role for α-Syn in a variety of essential processes, including synaptic vesicle trafficking and neurotransmitter release [74], immune cell maturation and function [4, 75, 76], DNA repair [77], and dopamine biosynthesis [78]. Thus, it is not inconceivable that a protein with crucial function(s) and with an abundant expression pattern throughout the body, when perturbed, can have deleterious consequences to the cell, as well as the organism as a whole.
Several pathological factors can push a protein over the physical nucleation barrier via different pathways and trigger amyloid aggregation. Protein overexpression due to gene duplication and triplication can lower the nucleation barrier increasing the probability of spontaneous nucleation. Furthermore, adding preformed nuclei (seeds/prions) will catalyze amyloid transformation by bypassing the nucleation step altogether. Moreover, exogenous surfaces such as nanoparticles or microbes can catalyze nucleation by increasing local protein concentration and inducing conformational changes via heterogeneous nucleation [79]. Many proteins exposed to such catalytic pathways of nucleation will end up in the non-functional amyloid state, while not necessarily becoming more toxic in the process. Thus, although controversial, LOF needs to be considered as a potential pathogenic mechanism in PD. To that end, strategies that aim to stabilize monomeric forms of the protein, or to remove the nucleation seed (i.e., aggregated Synuclein) are ideal therapeutic candidates. Moreover, supplementation in the form of non-aggregatable forms of the protein should also be considered.
CONCLUSIONS
Without doubt, α-Syn is a key etiopathological participant in PD and other synucleinopathies, and at the center of the role of α-Syn in disease is the propensity of this protein to form stable aggregates. The structure and formation of such α-Syn aggregates confers detrimental effects on neurons and depending on the vulnerability of specific neuronal sub-population neurodegeneration occurs. Nonetheless, the specific mechanism by which the neuron is impacted, whether it is toxic gain or loss of function, and whether α-Syn-mediated toxicity is heterogenous in nature remains elusive. Still, in the last decade, numerous preclinical gene-therapy targets have emerged, representing conceptually distinct potential points of intervention as it relates to various molecular processes in neurons. At the same time, CNS gene therapy has made great strides, and PD has a rich history utilizing gene therapy, with 25 trials currently listed on clinicaltrials.gov involving PD, albeit none have been aimed at modulating α-Syn. Current and completed studies have focused on the neuroprotective effects of neurotrophic factors glial cell line-derived neurotrophic factor (GDNF, NCT01621581) and neurturin (CERE-120) [80], as well as to increase enzymatic levels such as glutamic acid decarboxylase (GAD) [81], aromatic L-amino acid decarboxylase (AADC; NCT03065192), or a combination of enzymes with lentiviral delivery of tyrosine hydroxylase, AADC, and GTP cyclohydrolase 1 (Prosavin; [82]). While clinical trials have yielded varying degrees of clinical improvements in PD motor symptoms, gene therapy studies have proven long-term safety [83]. With the concomitant development of novel, more efficient means of delivery, we are likely to see α-Syn-specific gene therapies in the years to come.
ACKNOWLEDGMENTS
Authors are supported by the Barrow Neurological Foundation.
CONFLICT OF INTEREST
The authors have no conflict of interest to report.
REFERENCES
[1] | Spillantini MG , Schmidt ML , Lee VM , Trojanowski JQ , Jakes R , Goedert M ((1997) ) Alpha-synuclein in Lewy bodies. Nature 388: , 839–840. |
[2] | Chartier-Harlin MC , Kachergus J , Roumier C , Mouroux V , Douay X , Lincoln S , Levecque C , Larvor L , Andrieux J , Hulihan M , Waucquier N , Defebvre L , Amouyel P , Farrer M , Destee A ((2004) ) Alpha-synuclein locus duplication as a cause of familial Parkinson’s disease. Lancet 364: , 1167–1169. |
[3] | Polymeropoulos MH , Lavedan C , Leroy E , Ide SE , Dehejia A , Dutra A , Pike B , Root H , Rubenstein J , Boyer R , Stenroos ES , Chandrasekharappa S , Athanassiadou A , Papapetropoulos T , Johnson WG , Lazzarini AM , Duvoisin RC , Di Iorio G , Golbe LI , Nussbaum RL ((1997) ) Mutation in the alpha-synuclein gene identified in families with Parkinson’s disease. Science 276: , 2045–2047. |
[4] | Pei Y , Maitta RW ((2019) ) Alpha synuclein in hematopoiesis and immunity. Heliyon 5: , e02590. |
[5] | Bartels T , Choi JG , Selkoe DJ ((2011) ) alpha-Synuclein occurs physiologically as a helically folded tetramer that resists aggregation. Nature 477: , 107–110. |
[6] | Fauvet B , Mbefo MK , Fares MB , Desobry C , Michael S , Ardah MT , Tsika E , Coune P , Prudent M , Lion N , Eliezer D , Moore DJ , Schneider B , Aebischer P , El-Agnaf OM , Masliah E , Lashuel HA ((2012) ) alpha-Synuclein in central nervous system and from erythrocytes, mammalian cells, and Escherichia coli exists predominantly as disordered monomer. J Biol Chem 287: , 15345–15364. |
[7] | Halliday GM , Holton JL , Revesz T , Dickson DW ((2011) ) Neuropathology underlying clinical variability in patients with synucleinopathies. Acta Neuropathol 122: , 187–204. |
[8] | McCann H , Stevens CH , Cartwright H , Halliday GM ((2014) ) alpha-Synucleinopathy phenotypes.S. Parkinsonism Relat Disord 20: (Suppl 1), 62–67. |
[9] | Brundin P , Dave KD , Kordower JH ((2017) ) Therapeutic approaches to target alpha-synuclein pathology. Exp Neurol 298: , 225–235. |
[10] | Braak H , Del Tredici K , Rub U , de Vos RA , Jansen Steur EN , Braak E ((2003) ) Staging of brain pathology related to sporadic Parkinson’s disease. Neurobiol Aging 24: , 197–211. |
[11] | Moussaud S , Jones DR , Moussaud-Lamodiere EL , Delenclos M , Ross OA , McLean PJ ((2014) ) Alpha-synuclein and tau: Teammates in neurodegeneration? Mol Neurodegener 9: , 43. |
[12] | Prusiner SB ((1982) ) Novel proteinaceous infectious particles cause scrapie. Science 216: , 136–144. |
[13] | Hijaz BA , Volpicelli-Daley LA ((2020) ) Initiation and propagation of alpha-synuclein aggregation in the nervous system. Mol Neurodegener 15: , 19. |
[14] | Rey NL , Steiner JA , Maroof N , Luk KC , Madaj Z , Trojanowski JQ , Lee VM , Brundin P ((2016) ) Widespread transneuronal propagation of alpha-synucleinopathy triggered in olfactory bulb mimics prodromal Parkinson’s disease. J Exp Med 213: , 1759–1778. |
[15] | Holmqvist S , Chutna O , Bousset L , Aldrin-Kirk P , Li W , Bjorklund T , Wang ZY , Roybon L , Melki R , Li JY ((2014) ) Direct evidence of Parkinson pathology spread from the gastrointestinal tract to the brain in rats. Acta Neuropathol 128: , 805–820. |
[16] | Kim S , Kwon SH , Kam TI , Panicker N , Karuppagounder SS , Lee S , Lee JH , Kim WR , Kook M , Foss CA , Shen C , Lee H , Kulkarni S , Pasricha PJ , Lee G , Pomper MG , Dawson VL , Dawson TM , Ko HS ((2019) ) Transneuronal propagation of pathologic alpha-synuclein from the gut to the brain models Parkinson’s disease. Neuron 103: , 627–641 e627. |
[17] | Brundin P , Ma J , Kordower JH ((2016) ) How strong is the evidence that Parkinson’s disease is a prion disorder? Curr Opin Neurol 29: , 459–466. |
[18] | Manfredsson FP , Luk KC , Benskey MJ , Gezer A , Garcia J , Kuhn NC , Sandoval IM , Patterson JR , O’Mara A , Yonkers R , Kordower JH ((2018) ) Induction of alpha-synuclein pathology in the enteric nervous system of the rat and non-human primate results in gastrointestinal dysmotility and transient CNS pathology. Neurobiol Dis 112: , 106–118. |
[19] | Uemura N , Yagi H , Uemura MT , Hatanaka Y , Yamakado H , Takahashi R ((2018) ) Inoculation of alpha-synuclein preformed fibrils into the mouse gastrointestinal tract induces Lewy body-like aggregates in the brainstem via the vagus nerve. Mol Neurodegener 13: , 21. |
[20] | Uemura N , Yagi H , Uemura MT , Yamakado H , Takahashi R ((2020) ) Limited spread of pathology within the brainstem of alpha-synuclein BAC transgenic mice inoculated with preformed fibrils into the gastrointestinal tract. Neurosci Lett 716: , 134651. |
[21] | Ayers JI , Riffe CJ , Sorrentino ZA , Diamond J , Fagerli E , Brooks M , Galaleldeen A , Hart PJ , Giasson BI ((2018) ) Localized induction of wild-type and mutant alpha-synuclein aggregation reveals propagation along neuroanatomical tracts. J Virol 92: , e00586–18. |
[22] | Mahul-Mellier AL , Burtscher J , Maharjan N , Weerens L , Croisier M , Kuttler F , Leleu M , Knott GW , Lashuel HA ((2020) ) The process of Lewy body formation, rather than simply alpha-synuclein fibrillization, is one of the major drivers of neurodegeneration. Proc Natl Acad Sci U S A 117: , 4971–4982. |
[23] | Castonguay AM , Gravel C , Levesque M ((2021) ) Treating Parkinson’s disease with antibodies: Previous studies and future directions. J Parkinsons Dis 11: , 71–92. |
[24] | Volc D , Poewe W , Kutzelnigg A , Luhrs P , Thun-Hohenstein C , Schneeberger A , Galabova G , Majbour N , Vaikath N , El-Agnaf O , Winter D , Mihailovska E , Mairhofer A , Schwenke C , Staffler G , Medori R ((2020) ) Safety and immunogenicity of the alpha-synuclein active immunotherapeutic PD01A in patients with Parkinson’s disease: A randomised, single-blinded, phase 1 trial. Lancet Neurol 19: , 591–600. |
[25] | Butler DC , Joshi SN , Genst E , Baghel AS , Dobson CM , Messer A ((2016) ) Bifunctional anti-non-amyloid component alpha-synuclein nanobodies are protective in situ. PLoS One 11: , e0165964. |
[26] | Chatterjee D , Bhatt M , Butler D , De Genst E , Dobson CM , Messer A , Kordower JH ((2018) ) Proteasome-targeted nanobodies alleviate pathology and functional decline in an alpha-synuclein-based Parkinson’s disease model. NPJ Parkinsons Dis 4: , 25. |
[27] | Reyes JF , Sackmann C , Hoffmann A , Svenningsson P , Winkler J , Ingelsson M , Hallbeck M ((2019) ) Binding of alpha-synuclein oligomers to Cx32 facilitates protein uptake and transfer in neurons and oligodendrocytes. Acta Neuropathol 138: , 23–47. |
[28] | Kim C , Spencer B , Rockenstein E , Yamakado H , Mante M , Adame A , Fields JA , Masliah D , Iba M , Lee HJ , Rissman RA , Lee SJ , Masliah E ((2018) ) Immunotherapy targeting toll-like receptor 2 alleviates neurodegeneration in models of synucleinopathy by modulating alpha-synuclein transmission and neuroinflammation. Mol Neurodegener 13: , 43. |
[29] | Mao X , Ou MT , Karuppagounder SS , Kam TI , Yin X , Xiong Y , Ge P , Umanah GE , Brahmachari S , Shin JH , Kang HC , Zhang J , Xu J , Chen R , Park H , Andrabi SA , Kang SU , Goncalves RA , Liang Y , Zhang S , Qi C , Lam S , Keiler JA , Tyson J , Kim D , Panicker N , Yun SP , Workman CJ , Vignali DA , Dawson VL , Ko HS , Dawson TM ((2016) ) Pathological alpha-synuclein transmission initiated by binding lymphocyte-activation gene 3. Science 353: , aah3374. |
[30] | Emmenegger M , De Cecco E , Hruska-Plochan M , Eninger T , Schneider MM , Barth M , Tantardini E , de Rossi P , Bacioglu M , Langston RG , Kaganovich A , Bengoa-Vergniory N , Gonzalez-Guerra A , Avar M , Heinzer D , Reimann R , Häsler LM , Herling TW , Matharu NS , Landeck N , Luk K , Melki R , Kahle PJ , Hornemann S , Knowles TPJ , Cookson MR , Polymenidou M , Jucker M , Aguzzi A (2021) LAG3 is not expressed in human and murine neurons and does not modulate α-synucleinopathies. bioRxiv, 2021.2004.2025.441302. |
[31] | Cole TA , Zhao H , Collier TJ , Sandoval I , Sortwell CE , Steece-Collier K , Daley BF , Booms A , Lipton J , Welch M , Berman M , Jandreski L , Graham D , Weihofen A , Celano S , Schulz E , Cole-Strauss A , Luna E , Quach D , Mohan A , Bennett CF , Swayze EE , Kordasiewicz HB , Luk KC , Paumier KL ((2021) ) alpha-Synuclein antisense oligonucleotides as a disease-modifying therapy for Parkinson’s disease. JCI Insight 6: , e135633. |
[32] | Benskey MJ , Sellnow RC , Sandoval IM , Sortwell CE , Lipton JW , Manfredsson FP ((2018) ) Silencing alpha synuclein in mature nigral neurons results in rapid neuroinflammation and subsequent toxicity. Front Mol Neurosci 11: , 36. |
[33] | Gaj T , Gersbach CA , Barbas CF , 3rd ((2013) ) ZFN, TALEN, and CRISPR/Cas-based methods for genome engineering. Trends Biotechnol 31: , 397–405. |
[34] | Liu L , Li Y , Peng H , Liu R , Ji W , Shi Z , Shen J , Ma G , Zhang X ((2020) ) Targeted exosome coating gene-chem nanocomplex as “nanoscavenger” for clearing alpha-synuclein and immune activation of Parkinson’s disease. Sci Adv 6: , eaba3967. |
[35] | Kantor B , Tagliafierro L , Gu J , Zamora ME , Ilich E , Grenier C , Huang ZY , Murphy S , Chiba-Falek O ((2018) ) Downregulation of SNCA expression by targeted editing of DNA methylation: A potential strategy for precision therapy in PD. Mol Ther 26: , 2638–2649. |
[36] | Zharikov AD , Cannon JR , Tapias V , Bai Q , Horowitz MP , Shah V , El Ayadi A , Hastings TG , Greenamyre JT , Burton EA ((2015) ) shRNA targeting alpha-synuclein prevents neurodegeneration in a Parkinson’s disease model. J Clin Invest 125: , 2721–2735. |
[37] | Wang YC , Feng GY , Xia QJ , Hu Y , Xu Y , Xiong LL , Chen ZW , Wang HP , Wang TH , Zhou X ((2016) ) Knockdown of alpha-synuclein in cerebral cortex improves neural behavior associated with apoptotic inhibition and neurotrophin expression in spinal cord transected rats. Apoptosis 21: , 404–420. |
[38] | Alarcon-Aris D , Recasens A , Galofre M , Carballo-Carbajal I , Zacchi N , Ruiz-Bronchal E , Pavia-Collado R , Chica R , Ferres-Coy A , Santos M , Revilla R , Montefeltro A , Farinas I , Artigas F , Vila M , Bortolozzi A ((2018) ) Selective alpha-synuclein knockdown in monoamine neurons by intranasal oligonucleotide delivery: Potential therapy for Parkinson’s disease. Mol Ther 26: , 550–567. |
[39] | Collier TJ , Redmond DE Jr. , Steece-Collier K , Lipton JW , Manfredsson FP ((2016) ) Is alpha-synuclein loss-of-function a contributor to parkinsonian pathology? Evidence from non-human primates. Front Neurosci 10: , 12. |
[40] | Gorbatyuk OS , Li S , Nash K , Gorbatyuk M , Lewin AS , Sullivan LF , Mandel RJ , Chen W , Meyers C , Manfredsson FP , Muzyczka N ((2010) ) In vivo RNAi-mediated alpha-synuclein silencing induces nigrostriatal degeneration. Mol Ther 18: , 1450–1457. |
[41] | Greten-Harrison B , Polydoro M , Morimoto-Tomita M , Diao L , Williams AM , Nie EH , Makani S , Tian N , Castillo PE , Buchman VL , Chandra SS ((2010) ) alphabetagamma-Synuclein triple knockout mice reveal age-dependent neuronal dysfunction. Proc Natl Acad Sci U S A 107: , 19573–19578. |
[42] | Kujawska M , Jodynis-Liebert J ((2018) ) Polyphenols in Parkinson’s disease: A systematic review of in vivo studies. Nutrients 10: , 642. |
[43] | Wrasidlo W , Tsigelny IF , Price DL , Dutta G , Rockenstein E , Schwarz TC , Ledolter K , Bonhaus D , Paulino A , Eleuteri S , Skjevik AA , Kouznetsova VL , Spencer B , Desplats P , Gonzalez-Ruelas T , Trejo-Morales M , Overk CR , Winter S , Zhu C , Chesselet MF , Meier D , Moessler H , Konrat R , Masliah E ((2016) ) A de novo compound targeting alpha-synuclein improves deficits in models of Parkinson’s disease. Brain 139: , 3217–3236. |
[44] | Price DL , Koike MA , Khan A , Wrasidlo W , Rockenstein E , Masliah E , Bonhaus D ((2018) ) The small molecule alpha-synuclein misfolding inhibitor, NPT200-11, produces multiple benefits in an animal model of Parkinson’s disease. Sci Rep 8: , 16165. |
[45] | Herrera FE , Chesi A , Paleologou KE , Schmid A , Munoz A , Vendruscolo M , Gustincich S , Lashuel HA , Carloni P ((2008) ) Inhibition of alpha-synuclein fibrillization by dopamine is mediated by interactions with five C-terminal residues and with E83 in the NAC region. PLoS One 3: , e3394. |
[46] | Schrader T , Bitan G , Klarner FG ((2016) ) Molecular tweezers for lysine and arginine - powerful inhibitors of pathologic protein aggregation. Chem Commun (Camb) 52: , 11318–11334. |
[47] | Deeg AA , Reiner AM , Schmidt F , Schueder F , Ryazanov S , Ruf VC , Giller K , Becker S , Leonov A , Griesinger C , Giese A , Zinth W ((2015) ) Anle138b and related compounds are aggregation specific fluorescence markers and reveal high affinity binding to alpha-synuclein aggregates. Biochim Biophys Acta 1850: , 1884–1890. |
[48] | Tatenhorst L , Eckermann K , Dambeck V , Fonseca-Ornelas L , Walle H , Lopes da Fonseca T , Koch JC , Becker S , Tonges L , Bahr M , Outeiro TF , Zweckstetter M , Lingor P ((2016) ) Fasudil attenuates aggregation of alpha-synuclein in models of Parkinson’s disease. Acta Neuropathol Commun 4: , 39. |
[49] | Moloney TC , Hyland R , O’Toole D , Paucard A , Kirik D , O’Doherty A , Gorman AM , Dowd E ((2014) ) Heat shock protein 70 reduces alpha-synuclein-induced predegenerative neuronal dystrophy in the alpha-synuclein viral gene transfer rat model of Parkinson’s disease. CNS Neurosci Ther 20: , 50–58. |
[50] | Dedmon MM , Christodoulou J , Wilson MR , Dobson CM ((2005) ) Heat shock protein 70 inhibits alpha-synuclein fibril formation via preferential binding to prefibrillar species. J Biol Chem 280: , 14733–14740. |
[51] | Huang C , Cheng H , Hao S , Zhou H , Zhang X , Gao J , Sun QH , Hu H , Wang CC ((2006) ) Heat shock protein 70 inhibits alpha-synuclein fibril formation via interactions with diverse intermediates. J Mol Biol 364: , 323–336. |
[52] | Sidransky E , Nalls MA , Aasly JO , Aharon-Peretz J , Annesi G , Barbosa ER , Bar-Shira A , Berg D , Bras J , Brice A , Chen CM , Clark LN , Condroyer C , De Marco EV , Durr A , Eblan MJ , Fahn S , Farrer MJ , Fung HC , Gan-Or Z , Gasser T , Gershoni-Baruch R , Giladi N , Griffith A , Gurevich T , Januario C , Kropp P , Lang AE , Lee-Chen GJ , Lesage S , Marder K , Mata IF , Mirelman A , Mitsui J , Mizuta I , Nicoletti G , Oliveira C , Ottman R , Orr-Urtreger A , Pereira LV , Quattrone A , Rogaeva E , Rolfs A , Rosenbaum H , Rozenberg R , Samii A , Samaddar T , Schulte C , Sharma M , Singleton A , Spitz M , Tan EK , Tayebi N , Toda T , Troiano AR , Tsuji S , Wittstock M , Wolfsberg TG , Wu YR , Zabetian CP , Zhao Y , Ziegler SG ((2009) ) Multicenter analysis of glucocerebrosidase mutations in Parkinson’s disease. N Engl J Med 361: , 1651–1661. |
[53] | Mazzulli JR , Xu YH , Sun Y , Knight AL , McLean PJ , Caldwell GA , Sidransky E , Grabowski GA , Krainc D ((2011) ) Gaucher disease glucocerebrosidase and alpha-synuclein form a bidirectional pathogenic loop in synucleinopathies. Cell 146: , 37–52. |
[54] | Yap TL , Velayati A , Sidransky E , Lee JC ((2013) ) Membrane-bound alpha-synuclein interacts with glucocerebrosidase and inhibits enzyme activity. Mol Genet Metab 108: , 56–64. |
[55] | Cookson MR ((2011) ) A feedforward loop links Gaucher and Parkinson’s diseases? Cell 146: , 9–11. |
[56] | Rocha EM , Smith GA , Park E , Cao H , Brown E , Hayes MA , Beagan J , McLean JR , Izen SC , Perez-Torres E , Hallett PJ , Isacson O ((2015) ) Glucocerebrosidase gene therapy prevents alpha-synucleinopathy of midbrain dopamine neurons. Neurobiol Dis 82: , 495–503. |
[57] | Sardi SP , Clarke J , Kinnecom C , Tamsett TJ , Li L , Stanek LM , Passini MA , Grabowski GA , Schlossmacher MG , Sidman RL , Cheng SH , Shihabuddin LS ((2011) ) CNS expression of glucocerebrosidase corrects alpha-synuclein pathology and memory in a mouse model of Gaucher-related synucleinopathy. Proc Natl Acad Sci U S A 108: , 12101–12106. |
[58] | Spencer B , Potkar R , Trejo M , Rockenstein E , Patrick C , Gindi R , Adame A , Wyss-Coray T , Masliah E ((2009) ) Beclin 1 gene transfer activates autophagy and ameliorates the neurodegenerative pathology in alpha-synuclein models of Parkinson’s and Lewy body diseases. J Neurosci 29: , 13578–13588. |
[59] | Decressac M , Mattsson B , Weikop P , Lundblad M , Jakobsson J , Bjorklund A ((2013) ) TFEB-mediated autophagy rescues midbrain dopamine neurons from alpha-synuclein toxicity. Proc Natl Acad Sci U S A 110: , E1817–1826. |
[60] | Xilouri M , Brekk OR , Landeck N , Pitychoutis PM , Papasilekas T , Papadopoulou-Daifoti Z , Kirik D , Stefanis L ((2013) ) Boosting chaperone-mediated autophagy in vivo mitigates alpha-synuclein-induced neurodegeneration. Brain 136: , 2130–2146. |
[61] | Alvarez-Erviti L , Seow Y , Schapira AH , Rodriguez-Oroz MC , Obeso JA , Cooper JM ((2013) ) Influence of microRNA deregulation on chaperone-mediated autophagy and alpha-synuclein pathology in Parkinson’s disease. Cell Death Dis 4: , e545. |
[62] | Angelopoulou E , Paudel YN , Piperi C ((2019) ) miR-124 and Parkinson’s disease: A biomarker with therapeutic potential. Pharmacol Res 150: , 104515. |
[63] | Caggiu E , Arru G , Hosseini S , Niegowska M , Sechi G , Zarbo IR , Sechi LA ((2019) ) Inflammation, infectious triggers, and Parkinson’s disease. Front Neurol 10: , 122. |
[64] | Kline EM , Houser MC , Herrick MK , Seibler P , Klein C , West A , Tansey MG ((2021) ) Genetic and environmental factors in Parkinson’s disease converge on immune function and inflammation. Mov Disord 36: , 25–36. |
[65] | Ferreira SA , Romero-Ramos M ((2018) ) Microglia response during Parkinson’s disease: Alpha-synuclein intervention. Front Cell Neurosci 12: , 247. |
[66] | Rocha EM , De Miranda B , Sanders LH ((2018) ) Alphasynuclein: Pathology, mitochondrial dysfunction and neuroinflammation in Parkinson’s disease. Neurobiol Dis 109: , 249–257. |
[67] | Lindestam Arlehamn CS , Dhanwani R , Pham J , Kuan R , Frazier A , Rezende Dutra J , Phillips E , Mallal S , Roederer M , Marder KS , Amara AW , Standaert DG , Goldman JG , Litvan I , Peters B , Sulzer D , Sette A ((2020) ) alpha-Synuclein-specific T cell reactivity is associated with preclinical and early Parkinson’s disease. Nat Commun 11: , 1875. |
[68] | Qin XY , Zhang SP , Cao C , Loh YP , Cheng Y ((2016) ) Aberrations in peripheral inflammatory cytokine levels in Parkinson disease: A systematic review and meta-analysis. JAMA Neurol 73: , 1316–1324. |
[69] | Williams GP , Marmion DJ , Schonhoff AM , Jurkuvenaite A , Won WJ , Standaert DG , Kordower JH , Harms AS ((2020) ) T cell infiltration in both human multiple system atrophy and a novel mouse model of the disease. Acta Neuropathol 139: , 855–874. |
[70] | Nash KR , Moran P , Finneran DJ , Hudson C , Robinson J , Morgan D , Bickford PC ((2015) ) Fractalkine over expression suppresses alpha-synuclein-mediated neurodegeneration. Mol Ther 23: , 17–23. |
[71] | Harms AS , Barnum CJ , Ruhn KA , Varghese S , Trevino I , Blesch A , Tansey MG ((2011) ) Delayed dominant-negative TNF gene therapy halts progressive loss of nigral dopaminergic neurons in a rat model of Parkinson’s disease. Mol Ther 19: , 46–52. |
[72] | Cali T , Ottolini D , Negro A , Brini M ((2012) ) alpha-Synuclein controls mitochondrial calcium homeostasis by enhancing endoplasmic reticulum-mitochondria interactions. J Biol Chem 287: , 17914–17929. |
[73] | Osterberg VR , Spinelli KJ , Weston LJ , Luk KC , Woltjer RL , Unni VK ((2015) ) Progressive aggregation of alpha-synuclein and selective degeneration of lewy inclusion-bearing neurons in a mouse model of parkinsonism. Cell Rep 10: , 1252–1260. |
[74] | Lautenschlager J , Kaminski CF , Kaminski Schierle GS ((2017) ) alpha-Synuclein - regulator of exocytosis, endocytosis, or both? Trends Cell Biol 27: , 468–479. |
[75] | Tashkandi H , Shameli A , Harding CV , Maitta RW ((2018) ) Ultrastructural changes in peripheral blood leukocytes in alpha-synuclein knockout mice. Blood Cells Mol Dis 73: , 33–37. |
[76] | Xiao W , Shameli A , Harding CV , Meyerson HJ , Maitta RW ((2014) ) Late stages of hematopoiesis and B cell lymphopoiesis are regulated by alpha-synuclein, a key player in Parkinson’s disease. Immunobiology 219: , 836–844. |
[77] | Schaser AJ , Osterberg VR , Dent SE , Stackhouse TL , Wakeham CM , Boutros SW , Weston LJ , Owen N , Weissman TA , Luna E , Raber J , Luk KC , McCullough AK , Woltjer RL , Unni VK ((2019) ) Alpha-synuclein is a DNA binding protein that modulates DNA repair with implications for Lewy body disorders. Sci Rep 9: , 10919. |
[78] | Perez RG , Waymire JC , Lin E , Liu JJ , Guo F , Zigmond MJ ((2002) ) A role for alpha-synuclein in the regulation of dopamine biosynthesis. J Neurosci 22: , 3090–3099. |
[79] | Ezzat K , Pernemalm M , Palsson S , Roberts TC , Jarver P , Dondalska A , Bestas B , Sobkowiak MJ , Levanen B , Skold M , Thompson EA , Saher O , Kari OK , Lajunen T , Sverremark Ekstrom E , Nilsson C , Ishchenko Y , Malm T , Wood MJA , Power UF , Masich S , Linden A , Sandberg JK , Lehtio J , Spetz AL , El Andaloussi S ((2019) ) The viral protein corona directs viral pathogenesis and amyloid aggregation. Nat Commun 10: , 2331. |
[80] | Marks WJ Jr. , Ostrem JL , Verhagen L , Starr PA , Larson PS , Bakay RA , Taylor R , Cahn-Weiner DA , Stoessl AJ , Olanow CW , Bartus RT ((2008) ) Safety and tolerability of intraputaminal delivery of CERE-120 (adeno-associated virus serotype 2-neurturin) to patients with idiopathic Parkinson’s disease: An open-label, phase I trial. Lancet Neurol 7: , 400–408. |
[81] | Kaplitt MG , Feigin A , Tang C , Fitzsimons HL , Mattis P , Lawlor PA , Bland RJ , Young D , Strybing K , Eidelberg D , During MJ ((2007) ) Safety and tolerability of gene therapy with an adeno-associated virus (AAV) borne GAD gene for Parkinson’s disease: An open label, phase I trial. Lancet 369: , 2097–2105. |
[82] | Palfi S , Gurruchaga JM , Ralph GS , Lepetit H , Lavisse S , Buttery PC , Watts C , Miskin J , Kelleher M , Deeley S , Iwamuro H , Lefaucheur JP , Thiriez C , Fenelon G , Lucas C , Brugieres P , Gabriel I , Abhay K , Drouot X , Tani N , Kas A , Ghaleh B , Le Corvoisier P , Dolphin P , Breen DP , Mason S , Guzman NV , Mazarakis ND , Radcliffe PA , Harrop R , Kingsman SM , Rascol O , Naylor S , Barker RA , Hantraye P , Remy P , Cesaro P , Mitrophanous KA ((2014) ) Long-term safety and tolerability of ProSavin, a lentiviral vector-based gene therapy for Parkinson’s disease: A dose escalation, open-label, phase 1/2 trial. Lancet 383: , 1138–1146. |
[83] | Chu Y , Bartus RT , Manfredsson FP , Olanow CW , Kordower JH ((2020) ) Long-term post-mortem studies following neurturin gene therapy in patients with advanced Parkinson’s disease. Brain 143: , 960–975. |