Subthalamic Stimulation for Camptocormia in Parkinson’s Disease: Association of Volume of Tissue Activated and Structural Connectivity with Clinical Effectiveness
Abstract
Background:
Deep brain stimulation of the subthalamic nucleus (STN-DBS) has been reported to be effective for camptocormia in Parkinson’s disease (PD). However, the association between clinical effectiveness and the stimulated volumes or structural connectivity remains unexplored.
Objective:
To investigate the effectiveness of STN-DBS for treating camptocormia in PD and its association with volumes of tissue activated (VTA) and structural connectivity.
Methods:
We reviewed video recordings of patients who had undergone STN-DBS. The total and upper camptocormia (TCC and UCC) angles were measured to quantify changes in camptocormia. The Movement Disorders Society Unified Parkinson’s Disease Rating Scale III (MDS-UPDRS III) was used to assess motor symptoms. Pre- and postoperative brain images were collected for modeling volume of VTA and structural connectivity using Lead-DBS software.
Results:
Participants included 36 patients with PD (8 with TCC-camptocormia and 2 with UCC-camptocormia) treated with bilateral STN-DBS. After surgery, patients showed a significant improvement in postural alignment at follow-up (mean follow-up duration: 6.0±2.2 months). In the entire sample, higher structural connectivity to the right supplementary motor area (SMA) and right lateral premotor cortex along the dorsal plane (PMd) was associated with larger postsurgical improvements in axial signs and TCC angles after stimulation was turned on. In patients diagnosed with camptocormia, larger improvement in camptocormia angles after STN-DBS was associated with a larger VTA overlap with STN (R = 0.75, p = 0.032).
Conclusion:
This study suggests that both VTA overlap with STN and structural connectivity to cortical motor regions are associated with the effectiveness of STN-DBS for managing camptocormia in PD.
INTRODUCTION
Camptocormia is an abnormal posture with uncontrollable forward flexion of the spine while standing or walking, which affects 5–19% of patients with Parkinson’s disease (PD) [1–3]. This postural abnormality is a debilitating condition that can hinder patients from walking or performing activities of daily living [4]. Recently, an increasing number of retrospective studies have reported clinical effectiveness of subthalamic nucleus deep brain stimulation (STN-DBS) for treating PD postural abnormalities [5–8]. These findings showed that STN-DBS had a relatively small but significant effect on postural abnormalities in patients with PD [6, 8]. Furthermore, in a subgroup of PD patients who met diagnostic criteria for camptocormia, STN-DBS produced marked improvements (about 50–70%) in postural angle abnormalities [8], suggesting that STN-DBS may be particularly beneficial for PD patients with preexisting camptocormia.
However, despite the observed clinical value of STN-DBS in treating camptocormia, there are large differences between patients in the treatment effects [8]. These differences in treatment effects probably stem from methodological differences between studies (e.g., methods for camptocormia measurement), as well as by various patient sample characteristics (e.g., age, sex, medication status, nature/severity of clinical symptoms, illness onset/duration) and stimulation settings (e.g., DBS parameters, lead location) that may modify DBS treatment effects. In an effort to disentangle the complex effects of various covariates and disease modifiers on clinical outcome, the mapping of clinical improvements to stimulated brain areas is an intriguing concept and method with both a firm anatomical basis and an increasing research interest [9–13]. For example, overlaps between each patient’s volume of tissue activated (VTA) and STN volumes were found to be predictive of overall motor improvement, as measured by the Unified Parkinson’s Disease Rating Scale III [14]. Structural connectivity, which is measured by the estimated number of white tract fibers connecting two regions, plays a crucial role in the transmission of neural signals between brain regions, and was similarly found to be associated with clinical outcome [11, 15]. In previous studies of camptocormia in PD, univariate and multivariate regression analyses have been employed to investigate the association between clinical outcome and various candidate covariates, including the baseline severity of camptocormia, duration of camptocormia, and symptom response to levodopa treatment [6, 8, 16]. These analyses revealed that some demographic and clinical variables, including levodopa responsiveness, were associated with the DBS effect on camptocormia. However, no analyses were performed to assess whether the clinical outcome was associated with the volume of stimulated areas of the brain, or the integrity of structural connections between the VTA and brain areas of the cerebral cortex.
Here, we intended to shed more light on these issues by examining changes in camptocomia and its associated covariates in a retrospective cohort of PD patients before and after they received STN-DBS surgery. We assessed whether the effectiveness of STN-DBS for managing camptocormia was associated with: (a) the volume of stimulated areas of the brain, represented by the overlap between the patients’ VTAs and STN volumes, and (b) the VTA-motor cortex structural connectivity, namely the estmated number of fibers traversing through the VTA and projecting to motor cortices. Previous studies on camptocormia employed diverse methods for measurement of the severity of symptoms, and the results are therefore not easy to compare [17]. Fortunately, a consensus statement on camptocormia angle measurement has been released recently, which promotes unified assessment for camptocormia [3]. As this method was not yet routinely used in clinical PD evaluation and only a few studies reported outcome based on it [8, 18], we also present detailed assessment data at four states (presurgical med-OFF and med-ON, postsurgical med-OFF/DBS-OFF and med-OFF/DBS-ON) based on this published consensus [3].
MATERIALS AND METHODS
Study sample
Patients with PD who were treated with bilateral STN-DBS at Ruijin Hospital from January 2018 to December 2019 and with available videotaped pre- and post-surgical assessment data were retrospectively analyzed. PD patients were in Hoehn and Yahr stages 2–4, aged between 40 and 75 years, and had been assessed using videotaped motor examinations during presurgical (med-ON and med-OFF) and postsurgical (med-OFF/DBS-ON) conditions within 1 year after surgery. None of the patients included presented with other neurological disease or brain injuries or a history of CNS lesions. To reconstruct the lead location, presurgical T1- and T2-weighted MRI images, and postsurgical CT image were collected.
This study was carried out in accordance with the Code of Ethics of the World Medical Association (Declaration of Helsinki). The study was approved by the ethics committee of the Ruijin Hospital Shanghai Jiao Tong University School of Medicine. Informed consent was obtained from all patients.
Symptom assessment and computational methods
Presurgical evaluation was conducted 1–2 days before surgery; postsurgical evaluation was conducted at the corresponding follow-up. The follow-up assessment had to be performed within 12 months after surgery, a follow-up period during which a marked disease progression would be unlikely to occur. The mean follow-up period was 6.0±2.2 months (range: 1–12, median: 6 months).
(a) Posture analysis: Based on the method recommended in the consensus statement by Margraf et al. [3], posture angles were determined by two blinded physicians using the lateral view pictures of each patient standing still with the camera lens at approximately waist level; discrepancies between the two clinical judgements were solved during a consensus meeting. The camptocormia angles were calculated as follows [3]: (a) total camptocormia angle (TCC angle) = the angle between the line from the LM (lateral malleolus) to L5 (spinous process of L5 vertebra) and the line between L5 and C7 (spinous process of C7 vertebra), and (b) upper camptocormia angle (UCC angle) = the angle between the line from L5 to FC (vertebral fulcrum, point with the greatest distance from the spine) and the line from FC to C7. An online tool was used to calculate the angles (https://www.neurologie.uni-kiel.de/de/axial-posturale-stoerungen/camptoapp) [3]. Using the cutoff defined in previous studies (TCC angle≥30° for lower camptocormia or TCC-camptocormia, and UCC angle≥45° for upper camptocormia or UCC-camptocormia) [3, 19], it was determined whether TCC-camptocormia and UCC-camptocormia was present.
(b) Motor examination: The Movement Disorders Society Unified Parkinson’s Disease Rating Scale Part III (MDS-UPDRS III) was used to assess the patients’ motor symptoms [20, 21]. Preoperatively, the evaluation was carried out in the med-OFF state (after 12-h discontinuation of levodopa and 72 h for dopaminergic antagonist) and med-ON state (1.5 times the routine drug use and 45 min after administration). After surgery, the evaluation was carried out in the med-OFF/DBS-OFF state (1h after the stimulator had been turned off) and med-OFF/DBS-ON state.
(c) Collection of demographical information: Age at surgery, sex, duration of PD, medication, and other medical information along with the stimulation parameters used were collected.
(d) Based on the postoperative CT images, the position of the electrodes in the nucleus was reconstructed using the Lead-DBS toolbox on Matlab, according to the methods described by Horn et al. [22].
Imaging parameters
Presurgical imaging was performed on a 3T MRI system (Signa HDx 3.0 T, GE Healthcare, USA). A standard dose of 0.1 mmol/kg gadobutrol was administered intravenously at the start of the procedure for enhanced imaging. The MRI protocol consisted of the following sequences: head scout (14 s), axial three-dimensional T1-weighted magnetization-prepared rapid gradient-echo (3D-T1 MPRAGE; TR/TE/TI 6.648/2.04/450 ms, voxel size 0.9×0.9×0.9 mm3, FOV 240×240 mm2), and axial T2-weighted fast spin-echo (T2 TSE; TR/TE 3000/129.5 ms, voxel size 0.7×0.7×2.0 mm3, FOV 240×240 mm2). Postoperative CT scans were also acquired. High-resolution images were acquired on a LightSpeed16 (GE Medical System, Milwaukee, WI, USA) slice CT with a spatial resolution of 0.49×0.49×0.67 mm3. Images were acquired in axial (i.e., sequential/incremental) order at 140 kV and automated mA setting. The noise index was 7.0. A large scan field of view with a 50 cm diameter was used.
Image processing
Electrode localization and stimulation of VTAs were performed using the Lead-DBS software package (http://www.lead-dbs.org) [22]. Briefly, leads were semi-automatically localized using postsurgical CT images. The VTA was estimated with Lead-DBS based on finite element models (FEM). Conductivity values for white matter were set to 0.14 S/mm and for gray matter to 0.33 S/mm. Thresholding of the potential gradient at 0.2 V/mm then determined the activated tissue [11]. STN were located on the DBS Intrinsic Template AtLas (DISTAL), which was designed for surgical targets in basal ganglia and found to yield high localization accuracy [23]. Overlaps between VTAs and the STN were calculated for both hemispheres and were added together. For structural connectivity, we used a PD-specific connectome which was obtained from 85 patients included in the Parkinson Progression Marker Initiative (PPMI) database (http://www.ppmi-info.org) [24]. This connectome has also been used in prior studies [11, 14, 25] and processing details have been reported elsewhere [23]. Structural connectivity was calculated as the amount of fibers traversing through the VTA and projecting to the volumetric space of the brain in 2 mm isotropic resolution, yielding the fiber counts (numbers of streamlines seeding from VTAs) that traversed through each voxel [11, 14, 22]. Parcellation of motor cortices was based on the Human Motor Area Template (HMAT) atlas, which defines the primary motor cortex (M1), somatosensory cortex (S1), supplementary motor area (SMA), pre-SMA, and lateral premotor cortex along the dorsal and ventral plane (PMd and PMv) [26].
Statistical analysis
Initially, the Kolmogorov-Smirnov test was used to assess whether the data distributions deviated significantly from normality. When a variable met the normality requirement, we used paired t-tests to compare the patients’ baseline clinical data with their follow-up assessments. When a variable deviated from normality, non-parametric Wilcoxon signed-rank tests were used to analyze the data. χ2 or Fisher’s exact test was used for comparisons between treatment groups when appropriate.
Additionally, the effect of presurgical medication on TCC/UCC angles was investigated by comparing the angles during the medication-OFF state (med-OFF) with angles during the medication-ON state (med-ON) prior to surgery. The therapeutic benefit of DBS was determined by comparing the TCC/UCC angles during the presurgical med-OFF state with those measures during the postsurgical med-OFF/DBS-ON state. To describe the effect of stimulation, we also made data comparisons between the med-OFF/DBS-OFF state and med-OFF/DBS-ON state. Depending on the normality of the data, we employed either Pearson’s or Spearman’s correlation coefficient to assess the association between clinical/demographic characteristics and the effect of STN-DBS on camptocormia angles. Age at surgery, gender, duration of PD, follow-up time, pre-surgical motor score (MDS-UPDRS-III) at med-OFF, relative response to levodopa in motor score or TCC/UCC angles (pre-surgical med-OFF vs. pre-surgical med-ON evaluation) were explored as covariates of interest. Two-tailed p values <0.05 were considered significant. STATA 14.0 was used to analyze the data. The data are presented using means and standard deviations for continuous variables and frequencies for categorical variables, unless indicated otherwise.
RESULTS
Patient sample characteristics
Study participants consisted of 36 patients with PD. The demographical and clinical characteristics of the patient sample are presented in Tables 1, 2. Ten out of the 36 patients (27.7%) had camptocormia: 8 patients (22.2% of all patients) were diagnosed with TCC-camptocormia based on the criterion of a TCC angle of≥30° and 2 (5.6%) patients were diagnosed with UCC-camptocormia based on the criterion of a UCC angle of ≥45°. Assessment data collected during the presurgical med-OFF and med-ON states and the postsurgical med-OFF/DBS-ON state were available for all included participants. Data obtained during the med-OFF/DBS-OFF state were available for 32 patients (32 with measurement of TCC and UCC angles and 31 with MDS-UPDRS III ratings). The patients’ presurgical levodopa equivalent daily dosage (LEDD) was 717.1±311.7 mg, which was significantly reduced at follow-up (LEDD: 425.4±204.5 mg, p < 0.001).
Table 1
Patient demographic and clinical information
Characteristics | Value |
n | 36 |
Age at surgery (years) | 58.5±11.7 |
Sex | 7 F/29 M |
Age at PD onset | 49.2±12.0 |
Duration of PD (years) | 9.4±3.8 |
Follow-up time (months) | 6.0±2.2 |
LEDD (mg) | 717.1±311.7 |
Table 2
Presurgical examinations at the med-OFF state
Items | Overall | Without camptocormia | TCC-camptocormia | UCC-camptocormia |
n | 36 | 26 | 8 | 2 |
MDS-UPDRS III | ||||
Total | 53.0±16.1 | 47.9±14.2 | 69.0±13.8 | 50, 60 |
Tremor | 9.5±7.5 | 8.0±7.0 | 14.4±7.2 | 2, 18 |
Rigidity | 11.9±4.0 | 11.3±4.2 | 13.9±3.5 | 11, 13 |
Bradykinesia | 22.4±7.9 | 20.6±6.6 | 28.4±10.2 | 20, 23 |
Axial | 9.2±3.7 | 8.1±3.2 | 12.4±3.6 | 9, 14 |
Posture | 2.2±1.2 | 1.8±1.0 | 3.5±0.8 | 3, 3 |
Camptocormia angles | ||||
TCC angle | 22.6±16.5 | 14.0±4.5 | 50.2±11.7 | 21.3, 29.0 |
UCC angle | 33.9±7.5 | 31.8±5.5 | 35.2±2.6 | 51.6, 61.0 |
TCC-camptocormia | 8 | 0 | 8 | – |
UCC-camptocormia | 2 | 0 | – | 2 |
TCC, total camptocormia; UCC, upper camptocormia; TCC-camptocormia, group of patients with a clinically diagnosed camptocormia as defined by a TCC angle ≥30°; UCC-camptocormia, group of patients with a clinically diagnosed camptocormia as defined by a UCC angle ≥45°.
Effect of levodopa treatment on posture angles in the overall sample and in patients with/without camptocormia
Before STN-DBS surgery, a small but significant overall improvement was observed in both the TCC angle (from 22.6°±16.5° to 15.7°±8.2°, p = 0.0039; Fig. 1A) and the UCC angle (from 33.9°±7.5° to 31.5°±6.5°, p = 0.0002; Fig. 1B) in response to levodopa treatment. Similar levodopa-related improvements were detected in axial symptom scores (from 9.2±3.7 to 5.1±2.7, p < 0.0001; Fig. 1C). Particularly, significant improvements were observed in the TCC angle (from 50.2°±11.7° to 23.9°±12.2°, p = 0.0047; Fig. 2) in patients with TCC-camptocormia. In patients with UCC-camptocormia, levodopa-related improvements were also observed in the UCC angle (from 61° to 52° and from 51.6° to 44.7°). Additionally, significant improvements were seen in patients without camptocormia, with the TCC angle decreasing to 12.7±4.0°(p = 0.0129) and the UCC angle decreasing to 30.0±4.9°(p = 0.0012), following levodopa treatment.
Fig. 1
Pre- and postsurgical assessment of total (A) and upper (B) camptocormia angles (TCC/UCC angles), and axial subscores of MDS-UPDRS III in the whole population that underwent subthalamic (STN) deep brain stimulation (DBS). **p < 0.01; ***p < 0.001; ns: not significant.
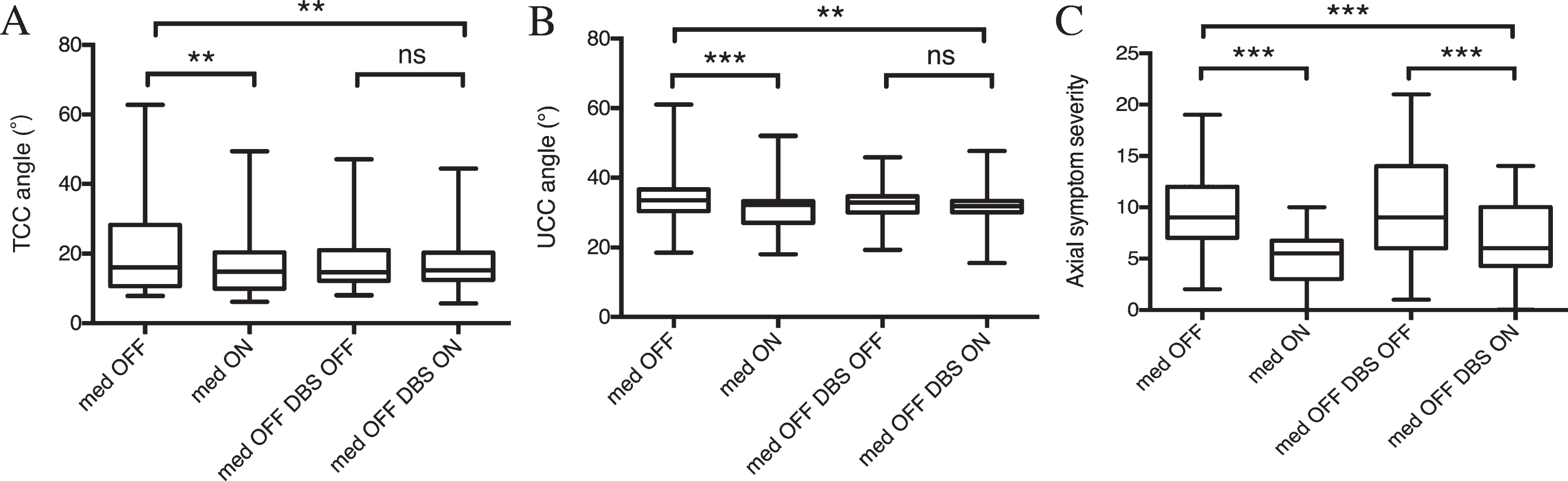
Fig. 2
Pre- and postsurgical assessment of total camptocormia (TCC) angles in patients with TCC-camptocormia. **p < 0.01; ***p < 0.001; ns: not significant.
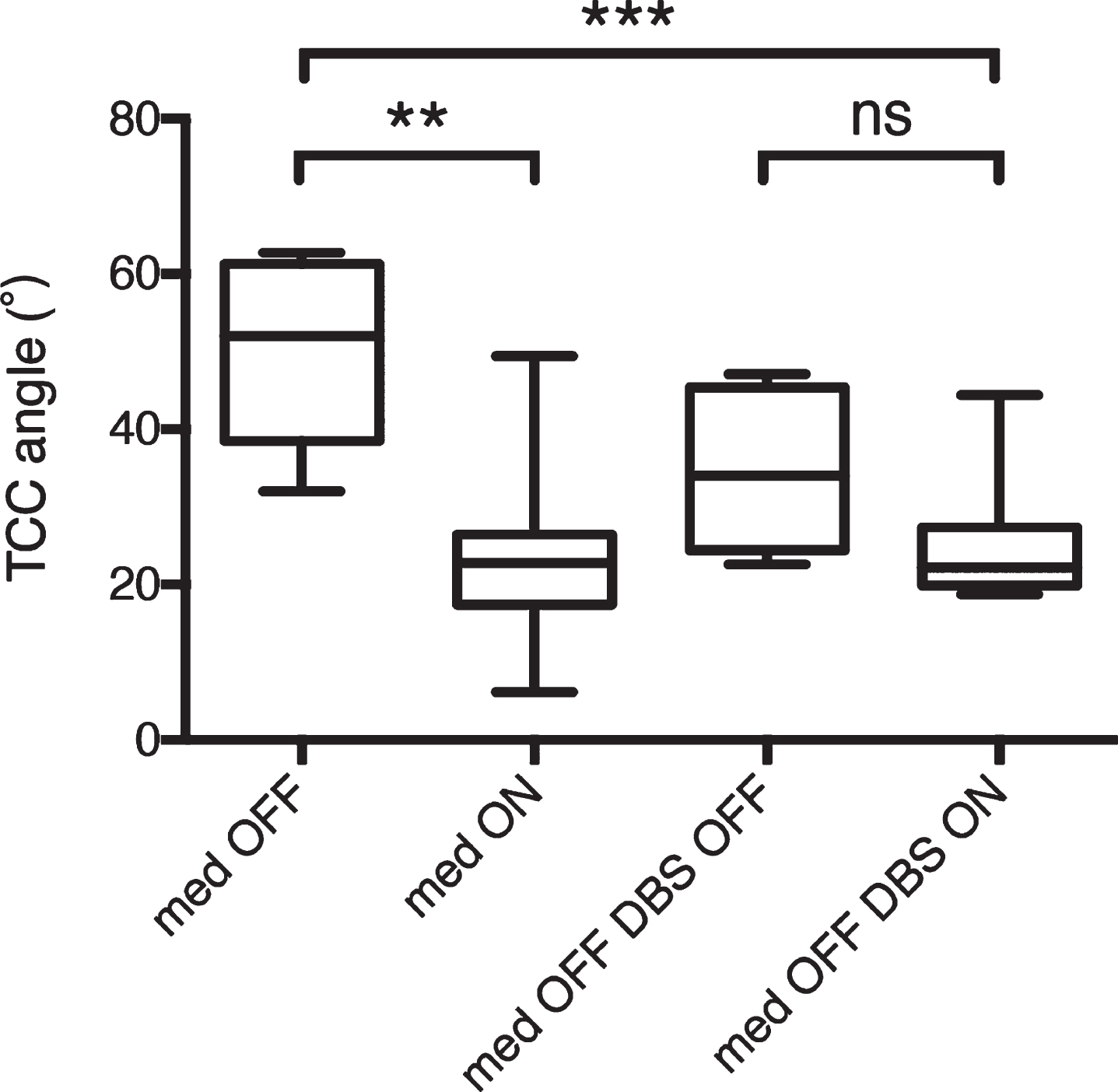
Effect of STN-DBS on posture angles in the overall sample and in patients with/without camptocormia
After bilateral STN-DBS surgery, all patients in the med-OFF/DBS-ON condition displayed a significant decrease in both the TCC angle (on average, from 22.6°±16.5° to 16.8°±7.0°; p = 0.0069; Fig. 1A) and the UCC angle (from 33.9°±7.5° to 31.3°±6.0°, p = 0.0056; Fig. 1B) at follow-up when compared with baseline med-OFF data. The decrease in TCC and UCC angles was accompanied by a marked improvement in axial symptoms (from 9.2±3.7 to 6.9±3.7, p = 0.0002; Fig. 1C). When these follow-up data were compared with the postsurgical data from the med-OFF/DBS-OFF condition, a non-significant reduction was seen in both the TCC angle (n = 32, p = 0.0692; Fig. 1A) and the UCC angle (n = 32, p = 0.1548; Fig. 1B).
In patients with TCC-camptocormia, it showed a significant decrease in the TCC angle at follow-up relative to the TCC data from the baseline med-OFF condition (50.2°±11.7° vs. 25.3°±8.3°, p = 0.0004; Fig. 2). However, the difference in the TCC angle between the postsurgical med-OFF/DBS-OFF state and med-OFF/DBS-ON state did not reach statistical significance (n = 6, 33.4°±10.1° vs. 22.7°±3.6°, p = 0.0504). The 2 patients with UCC-camptocormia similarly showed an improvement in the UCC angle at follow-up (from 61.0° to 33.4° and from 51.6° to 47.7°, presurgical med-OFF compared with postsurgical med-OFF/DBS-ON). Additionally, patients without camptocormia displayed a slight, nonsignificant increase in the TCC angle (from 14.0°±4.5° to 14.6°±3.9°, p = 0.2823) along with a significant decrease in the UCC angle (from 31.8°±5.5° to 29.9°±4.9°, p = 0.0018) in the med-OFF/DBS-ON state relative to the baseline med-OFF state. The TCC/UCC angles in patients without camptocormia did not differ significantly between the med-OFF/DBS-ON state and med-OFF/DBS-OFF state (n = 32, p = 0.6795/0.9067).
In 18 patients (4 with TCC-camptocormia and 2 with UCC-camptocormia) with available follow-up data at med-ON/DBS-ON state, we found the camptocormia angles were similar to those at presurgical med-ON state (15.6°±10.0° vs. 15.7°±7.8° in TCC angles, p = 0.8866; 32.2°±8.3° vs. 31.6°±6.4° in UCC angles, p = 0.7284), and the response rates (improvement > 15° or > 50% [5]) did not differ greatly (3 TCC/1 UCC responders at med-ON/DBS-ON state and 3 TCC/ 0 UCC responders at presurgical med-ON state) in either TCC or UCC angles (Fisher’s exact p = 1.000/1.000). Though the responders to DBS all respond well to levodopa in terms of TCC angle, one patient with UCC-camptocormia who responded poorly to levodopa (UCC angle from 61.0° at med-OFF state to 52.0° at med-ON state) improved greatly after surgery (UCC angle being 30.0° at med-ON/DBS-ON state).
Prior to the VTA and structural connectivity analyses, correlation analysis was performed to assess the relations between the demographical and clinical covariates to the clinical improvements at med-OFF/DBS-ON state observed at follow-up compared to presurgical med-OFF state. The results revealed that greater postsurgical improvements in the TCC/UCC angles were associated with greater presurgical TCC/UCC angles during the med-OFF state (TCC angle: R = 0.74, p < 0.0001; UCC angle: R = 0.43 p =0.0094), as well as with greater presurgical responsiveness to levodopa (R = 0.68, p < 0.0001; R = 0.39, p = 0.0174).
Effects of stimulation
To model the effects of stimulation, we first reconstructed the electrode location in Lead-DBS (Fig. 3). Subsequently, therapeutic effects were quantified in terms of: (a) the percent change between the presurgical med-OFF state and the postsurgical med-OFF/DBS-ON state, and (b) the percent change between the postsurgical med-OFF/DBS-OFF and med-OFF/DBS-ON states. We then assessed the correlations between the therapeutic effects and (1) the percentage of VTA overlap with STN, and (2) measures of structural connectivity from VTA to motor cortices (M1, S1, SMA, pre-SMA, PMd and PMv). The results showed that, for the whole patient sample, no significant correlation was found between VTA-STN overlap and the percent changes of axial subscores and TCC/UCC angles at postsurgical med-OFF/DBS-ON state compared to presurgical med-OFF values (all ps > 0.05). In terms of the postsurgical stimulation-related improvements, the percentage of VTA overlap with STN was found positively correlated with improvements in the subscores for axial symptoms (R = 0.46, p = 0.002; Fig. 4A). However, the postsurgical stimulation-related improvements in neither TCC angles nor UCC angles were significantly correlated with the percentage overlap with STN (both p > 0.05; Fig. 4B, 4C). On the other hand, in the subgroup of 7 patients who presented with TCC-camptocormia (n = 6) or UCC-camptocormia (n = 1), the postsurgical stimulation-related improvement in TCC/UCC angles did show a significant correlation with the percentage of VTA overlap with STN (R = 0.75, p = 0.032; Fig. 5).
Fig. 3
The deep brain stimulation (DBS) leads of included patients were merged on the T1-weighted Montreal Neurological Institute (MNI) templates, with active contacts marked in red. Masses with yellow describe the location of the STN, and red indicates the red nucleus.
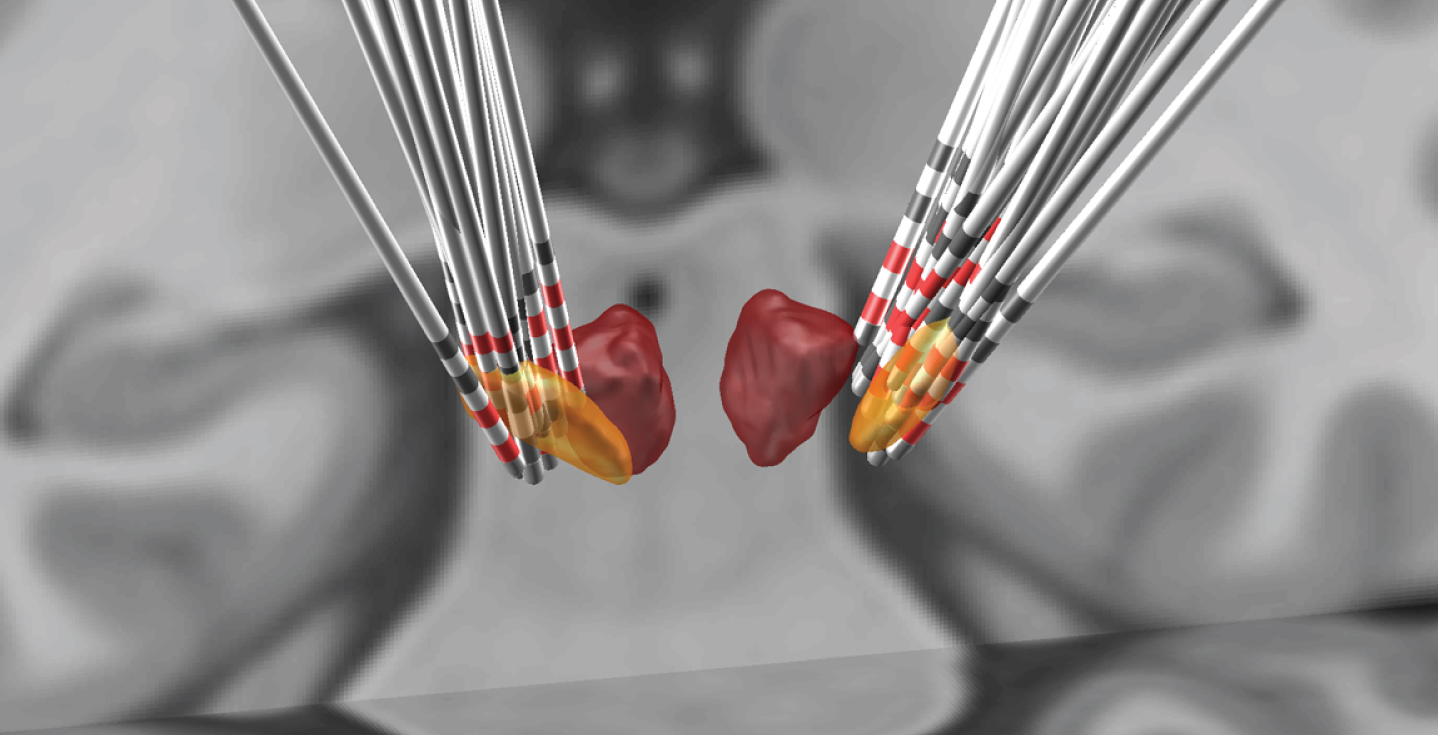
Fig. 4
Percentage of volume of tissue activated (VTA) overlap with subthalamic nucleus (STN) correlated with percent improvements in MDS-UPDRS III subscores for axial symptom (A), but not with the percent improvement in total camptocormia (TCC) angles (B) or upper camptocormia (UCC) angles (C). Grey areas represent the 95% confidence intervals (CIs).
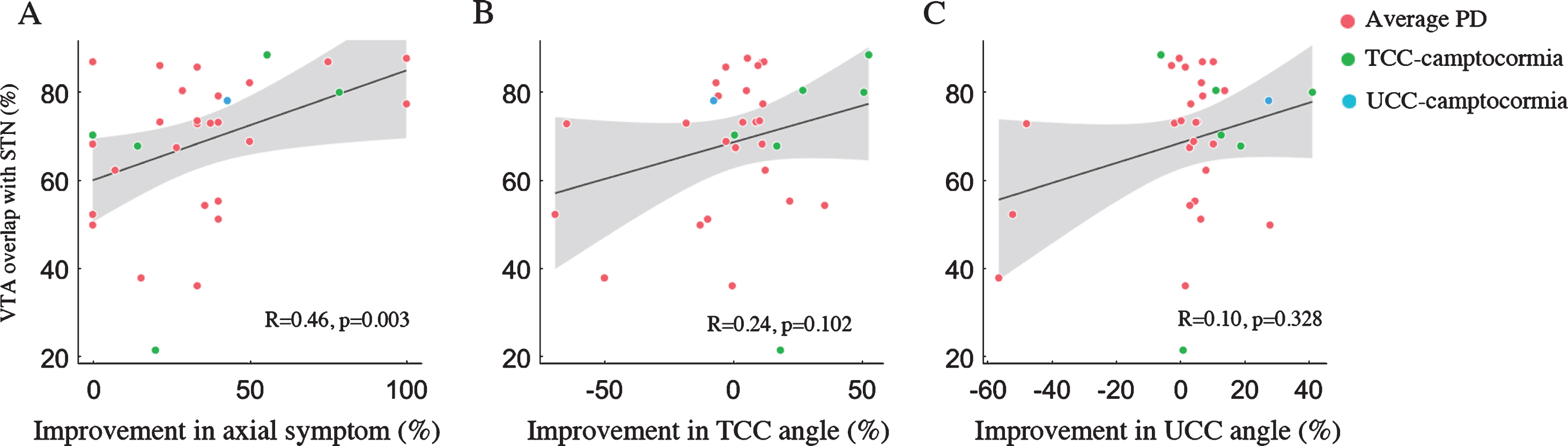
Fig. 5
Percentage of volume of tissue activated (VTA) overlap with subthalamic nucleus (STN) correlated with percent improvements in camptocormia angles [total camptocormia (TCC) angles in 6 patients and upper camptocormia (UCC) angle in 1 patient] in patients with camptocormia. Grey areas represent the 95% confidence intervals (CIs).
![Percentage of volume of tissue activated (VTA) overlap with subthalamic nucleus (STN) correlated with percent improvements in camptocormia angles [total camptocormia (TCC) angles in 6 patients and upper camptocormia (UCC) angle in 1 patient] in patients with camptocormia. Grey areas represent the 95% confidence intervals (CIs).](https://content.iospress.com:443/media/jpd/2021/11-1/jpd-11-1-jpd202259/jpd-11-jpd202259-g005.jpg)
Using VTA as the seed, estimates of structural connectivity to the right SMA (R = 0.41, p = 0.019; Fig. 6A) and the right PMd (R = 0.43, p = 0.015; Fig. 6B) showed significant correlations with postsurgical DBS-ON compared to DBS-OFF improvements at med-OFF state in TCC angles. Structural connectivity to these two brain areas was also associated with postsurgical improvements in subscores for axial symptoms (right SMA: R = 0.45, p = 0.011, Fig. 6C; right PMd: R = 0.52, p = 0.003, Fig. 6D). In addition, estmates of structural connectivity to the PMv were related to improvements in axial subscores observed at the postsurgical med-OFF/DBS-ON state when compared with the postsurgical med-OFF/DBS-OFF state (left PMv: R = 0.66, p < 0.001; right PMv: R = 0.50, p = 0.005).Structural connectivity to the left PMv (R = 0.40, p = 0.018) and right preSMA (R = 0.35, p = 0.037) was associated with improvements in axial subscores at the postsurgical med-OFF/DBS-ON state relative to the presurgical med-OFF state. No significant correlations were obtained between the remaining fiber connections and therapeutic effects under study.
Fig. 6
Structrual connectivity from volume of tissue activated (VTA) to supplementary motor area (SMA) and lateral premotor cortex along the dorsal plane (PMd) correlated with improvements in axial symptom (A, C) and total camptocormia (TCC) angles (B, D). Grey areas represent the 95% confidence intervals (CIs).
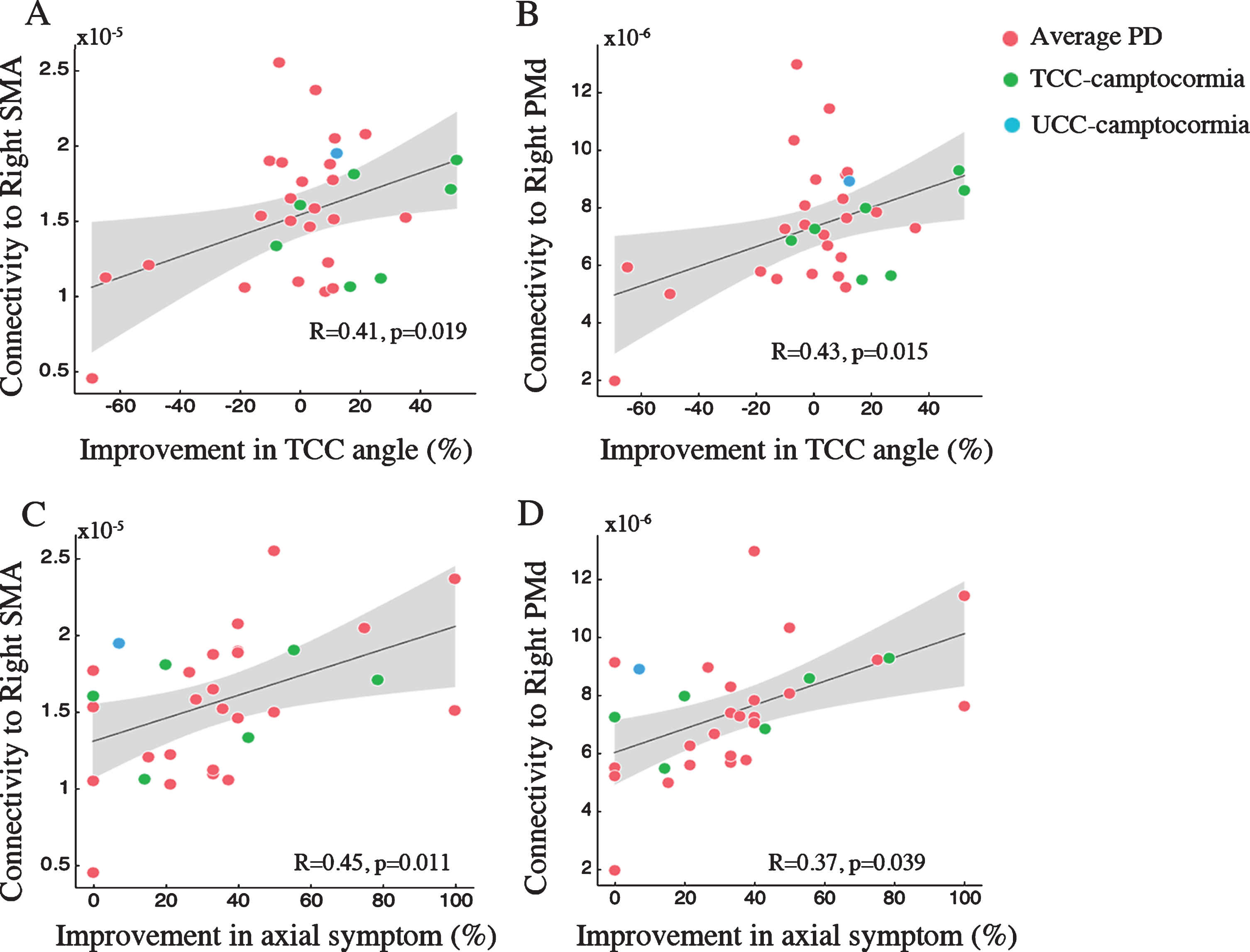
DISCUSSION
The basic results of this STN-DBS treatment study for PD camptocormia provides analysis of both the clinical effectiveness and stimulation related effect: (1) STN-DBS was associated with a significant improvement in patients’ postural abnormalities, as measured by reduced TCC and UCC angles at follow-up; (2) STN-DBS yielded evident postsurgical improvements in TCC/UCC angles in patients with camptocormia (28% of all patients); (3) patients with larger presurgical camptocormia angles and/or responsiveness to levodopa tended to achieve the greatest clinical benefits from STN-DBS treatment; and (4) the effectiveness of STN-DBS in managing the patients’ postural angle abnormalities was associated with both the extent of VTA overlap with STN and the density of structural connections to motor cortices, e.g. a larger VTA intersection of the STN and an increased connectivity to the right SMA and right PMd co-occurring with a larger postsurgical stimulation-related improvement in postural TCC angles. The finding that STN-DBS improved postural angle abnormalities confirms the results of previous DBS treatment studies of patients with PD [6, 8]. The present findings extend previous results by indicating that the effects of STN-DBS on postural angle abnormalities are most distinct in a subgroup of PD patients with camptocormia. Also, the results provide putative evidence indicating that the effect of STN-DBS on postural angle abnormalities in PD patients is not only locally mediated but instead, involves modulation of a widely distributed network of regions that include basal ganglia structures and cortical motor areas, specifically the SMA and PM. Indeed, animal and human studies have demonstrated that the SMA is crucial for the planning and execution of voluntary, self-initiated movement sequences [27].
In line with previous findings [8, 16], our re-sults showed that levodopa-related improvements in patients’ postural angle abnormalities were corr-elated with the improvements in postural abnormalities after STN-DBS treatment. This finding indicates that the patient’s responsiveness to levodopa could serve as predictor of the effectiveness of STN-DBS for managing camptocormia in patients with PD [5, 6]. According to some clinicians, camptocormia in PD could be a form of off-period dystonia. On this view, it was expected that camptocormia would respond well to levodopa and STN-DBS treatment [16, 28, 29]. Besides off-period dystonia which may respond well to levodopa treatment [28, 29], camptocormia can also be a form of peak-dose dystonic dyskinesia or a side effect of antiparkinsonian medications, and while it would not respond to medical therapy, it may be ameliorated after STN-DBS by an adequate drug reduction [30, 31]. In our only patient with poor response to levodopa but good response to DBS, though not drastically, the presurgical LEDD reduced from 300 mg to 200 mg, which may help understand the outcome of DBS. Compared to dopa-responsiveness, the PD disease duration, another important factor for surgery candidate selection, was found not associated with the STN-DBS treatment effect. Interestingly, the patients’ TCC/UCC angles at baseline were positively correlated with postsurgical improvements in postural angles, which suggests that the presence of camptocormia among patients with PD could also serve as a useful predictor for the clinical benefit of STN-DBS treatment.
Additionally, we observed a reduction in the patients’ posture angles in the postsurgical med-OFF/DBS-OFF state compared with the posture angles measured in the presurgical med-OFF state. This effect was also seen in a previous study [8]. According to the authors, this may reflect that STN-DBS exerts an additional effect to medication over time for this particular subgroup. This interpretation seems plausible because the action of DBS on axial symptoms is believed to take hours to weeks to occur [32]. The carryover effect from one experimental condition to the other experimental condition needs to be considered as a possible explanation because the time interval between the two tested states (med-OFF/DBS-ON and med-OFF/DBS-OFF) was relatively short (30–60 min) in both the current study and the study that implicated the presence of a carryover effect [8]. Alternatively, this reduction is suggested be related to the lesion caused by the STN-DBS surgery, which may still be evident at 6 months or longer postoperatively [33]. Therefore, med-OFF/DBS-ON data in comparison to presurgical med-OFF data gives relatively adequate time for improvements in axial symptoms to occur after programming but may assemble effects of both lesion and stimulation. On the other hand, though affected by the carryover effect, motor assessment in the med-OFF/DBS-ON condition in comparison to the med-OFF/DBS-OFF condition may provide a good, reasonably objective assessment of the effect of stimulation on parkinsonian motor signs [34].
In this study, the estimated overlap between VTA and STN volumes was predictive of postsurgical improvements not only for the patients’ cardinal PD motor symptoms, as reported by other studies [22, 35], but also for their axial symptoms. Although STN-DBS does not directly act on the striatum, this treatment is believed to impact other key structures in the basal ganglia circuit and hence, modulates the output of the pallidum [32]. The present results support the notion that the coverage of the STN and the amount of tissue activated by the stimulation play important roles in the clinical effectiveness of STN-DBS treatment for PD.
In general, the pathophysiology of postural abnormalities in PD is not well understood [2]. The activity of the SMA, a part of a larger supplementary motor complex (SMC), seems to be modulated by the interactive effects of proprioceptive and efference copy based information [36, 37]. The SMC has efferent projections to the putamen and the caudate, which then project to the internal segment of the globus pallidus (GPi) and, through the hyperdirect pathway, to the STN [27]. Recently, the SMA has been linked to postural control [38–41]. In our study, higher structural connectivity between the VTA and right SMA was similarly associated with a larger improvement in postural angle abnormalities after STN-DBS surgery. The present findings may indicate that stimulation over the fibers to the SMA could affect basal ganglia activity, thereby modulating the flow of information in corticostriatal circuitry. Due to the intrinsic positive connectivity between the SMA and STN [42], future research with patient-specific fiber tracking and direct stimulation of the SMA may help disentangle the impact of STN intersection from the influence of SMA structural connectivity on postural improvements in PD.
Another issue that should be addressed is whether either the STN or GPi can be considered as the clinically most optimal DBS target for PD. So far, however, there is no strong evidence supporting one target over the other [43]. We chose the STN as the target because of its common use and recent evidence suggesting its better performance in improving off-drug motor symptoms and functioning compared with GPi-DBS [44]. Comparative DBS studies, however, are required to evaluate the relative efficacy of these two targets in managing camptocormia in PD [5, 17].
Study limitations
The main limitations to our study were its retrospective design and observational nature. Therefore, prospective and controlled studies are needed to confirm or qualify the present results. Second, the relatively small camptocormia patient sample size posed limitations to the statistical power and precluded a formal analysis of the effects of STN-DBS on UCC angles [45]. The involvement of large number of patients with normal posture or mild postural abnormalities make the improvements in camptocormia angles quite fluctuant and random, which may weaken the correlation between investigated variables and outcomes. Future studies with larger number of PD patients with camptocormia are necessary to identify robust predictors of clinical outcome of STN-DBS treatment. Third, the follow-up duration was relatively short (12 months or shorter) in our study. It remains to be determined, therefore, whether the beneficial effects of DBS-STN on camptocormia persist over the long-term treatment and disease course [46].
CONCLUSIONS
Our study demonstrates that the size of the overlap between the VTA and STN volumes, as well as the density of the white matter fibers from the VTA to the right SMA and right PMd, was associated with the clinical improvement in camptocormia angles of DBS-STN treatment in a cohort of PD patients. Specifically, patients who displayed larger VTA-STN overlap or higher structural connectivity were likely to experience greater improvements in PD motor symptoms and camptocormia after DBS-STN surgery. The observed treatment effectiveness compared to presurgical evaluation also suggests that camptocormia is not a contraindication for DBS and that this postural abnormality may even improve following STN-DBS. Large randomized controlled studies of PD patients with camptocormia are needed to determine the long-term effects of STN-DBS.
CONFLICT OF INTEREST
All authors reported no conflict of interest related to this study.
Dr. Sun received research support from DBS industry SceneRay and PINS (donated devices); Dr. D. Li and Dr. C. Zhang received honoraria and travel expenses from Medtronic, SceneRay, and PINS.
ACKNOWLEDGMENTS
We thank Ms.Lu Xu for data collection
This research did not receive any specific grant from funding agencies in the public, commercial, or not-for-profit sectors.
REFERENCES
[1] | Tiple D , Fabbrini G , Colosimo C , Ottaviani D , Camerota F , Defazio G , Berardelli A ((2009) ) Camptocormia in Parkinson disease: An epidemiological and clinical study. J Neurol Neurosurg Psychiatry 80: , 145–148. |
[2] | Doherty KM , van de Warrenburg BP , Peralta MC , Silveira-Moriyama L , Azulay JP , Gershanik OS , Bloem BR ((2011) ) Postural deformities in Parkinson’s disease. Lancet Neurol 10: , 538–549. |
[3] | Margraf NG , Wolke R , Granert O , Berardelli A , Bloem BR , Djaldetti R , Espay AJ , Fasano A , Furusawa Y , Giladi N , Hallett M , Jankovic J , Murata M , Tinazzi M , Volkmann J , Berg D , Deuschl G ((2018) ) Consensus for the measurement of the camptocormia angle in the standing patient. Parkinsonism Relat Disord 52: , 1–5. |
[4] | Upadhyaya CD , Starr PA , Mummaneni PV ((2010) ) Spinal deformity and Parkinson disease: A treatment algorithm. Neurosurg Focus 28: , E5. |
[5] | Chan AK , Chan AY , Lau D , Durcanova B , Miller CA , Larson PS , Starr PA , Mummaneni PV ((2018) ) Surgical management of camptocormia in Parkinson’s disease: Systematic review and meta-analysis. J Neurosurg 131: , 368–375. |
[6] | Roediger J , Artusi CA , Romagnolo A , Boyne P , Zibetti M , Lopiano L , Espay AJ , Fasano A , Merola A ((2019) ) Effect of subthalamic deep brain stimulation on posture in Parkinson’s disease: A blind computerized analysis. Parkinsonism Relat Disord 62: , 122–127. |
[7] | Fasano A , Aquino CC , Krauss JK , Honey CR , Bloem BR ((2015) ) Axial disability and deep brain stimulation in patients with Parkinson disease. Nat Rev Neurol 11: , 98–110. |
[8] | Schlenstedt C , Gavriliuc O , Bosse K , Wolke R , Granert O , Deuschl G , Margraf NG ((2019) ) The effect of medication and deep brain stimulation on posture in Parkinson’s disease. Front Neurol 10: , 1254. |
[9] | Fox MD ((2018) ) Mapping symptoms to brain networks with the human connectome. N Engl J Med 379: , 2237–2245. |
[10] | Kahan J , Urner M , Moran R , Flandin G , Marreiros A , Mancini L , White M , Thornton J , Yousry T , Zrinzo L , Hariz M , Limousin P , Friston K , Foltynie T ((2014) ) Resting state functional MRI in Parkinson’s disease: The impact of deep brain stimulation on ‘effective’ connectivity. Brain 137: , 1130–1144. |
[11] | Horn A , Reich M , Vorwerk J , Li N , Wenzel G , Fang Q , Schmitz-Hubsch T , Nickl R , Kupsch A , Volkmann J , Kuhn AA , Fox MD ((2017) ) Connectivity predicts deep brain stimulation outcome in Parkinson disease. Ann Neurol 82: , 67–78. |
[12] | Horn A , Wenzel G , Irmen F , Huebl J , Li N , Neumann WJ , Krause P , Bohner G , Scheel M , Kuhn AA ((2019) ) Deep brain stimulation induced normalization of the human functional connectome in Parkinson’s disease. Brain 142: , 3129–3143. |
[13] | Coenen VA , Schlaepfer TE , Varkuti B , Schuurman PR , Reinacher PC , Voges J , Zrinzo L , Blomstedt P , Fenoy AJ , Hariz M ((2019) ) Surgical decision making for deep brain stimulation should not be based on aggregated normative data mining. Brain Stimul 12: , 1345–1348. |
[14] | Treu S , Strange B , Oxenford S , Neumann W-J , Kühn A , Li N , Horn A ((2020) ) Deep brain stimulation: Imaging on a group level. Neuroimage 219: , 117018. |
[15] | Coenen VA , Allert N , Paus S , Kronenburger M , Urbach H , Madler B ((2014) ) Modulation of the cerebello-thalamo-cortical network in thalamic deep brain stimulation for tremor: A diffusion tensor imaging study. Neurosurgery 75: , 657–669; discussion 669-670. |
[16] | Yamada K , Shinojima N , Hamasaki T , Kuratsu J ((2016) ) Subthalamic nucleus stimulation improves Parkinson’s disease-associated camptocormia in parallel to its preoperative levodopa responsiveness. J Neurol Neurosurg Psychiatry 87: , 703–709. |
[17] | Lizarraga KJ , Fasano A ((2019) ) Effects of deep brain stimulation on postural trunk deformities: A systematic review. Mov Disord Clin Pract 6: , 627–638. |
[18] | Schlenstedt C , Bosse K , Gavriliuc O , Wolke R , Granert O , Deuschl G , Margraf NG ((2020) ) Quantitative assessment of posture in healthy controls and patients with Parkinson’s disease. Parkinsonism Relat Disord 76: , 85–90. |
[19] | Fasano A , Geroin C , Berardelli A , Bloem BR , Espay AJ , Hallett M , Lang AE , Tinazzi M ((2018) ) Diagnostic criteria for camptocormia in Parkinson’s disease: A consensus-based proposal. Parkinsonism Relat Disord 53: , 53–57. |
[20] | Li X , Xing Y , Martin-Bastida A , Piccini P , Auer DP ((2018) ) Patterns of grey matter loss associated with motor subscores in early Parkinson’s disease. Neuroimage Clin 17: , 498–504. |
[21] | Goetz CG , Tilley BC , Shaftman SR , Stebbins GT , Fahn S , Martinez-Martin P , Poewe W , Sampaio C , Stern MB , Dodel R , Dubois B , Holloway R , Jankovic J , Kulisevsky J , Lang AE , Lees A , Leurgans S , LeWitt PA , Nyenhuis D , Olanow CW , Rascol O , Schrag A , Teresi JA , van Hilten JJ , LaPelle N , Movement Disorder Society UPDRS Revision Task Force ((2008) ) Movement Disorder Society-Sponsored Revision of the Unified Parkinson’s Disease Rating Scale (MDS-UPDRS): Scale Presentation and Clinimetric Testing Results. Mov Disord 23: , 2129–2170. |
[22] | Horn A , Li N , Dembek TA , Kappel A , Boulay C , Ewert S , Tietze A , Husch A , Perera T , Neumann WJ , Reisert M , Si H , Oostenveld R , Rorden C , Yeh FC , Fang Q , Herrington TM , Vorwerk J , Kuhn AA ((2019) ) Lead-DBS v2: Towards a comprehensive pipeline for deep brain stimulation imaging. Neuroimage 184: , 293–316. |
[23] | Ewert S , Plettig P , Li N , Chakravarty MM , Collins DL , Herrington TM , Kühn AA , Horn A ((2018) ) Toward defining deep brain stimulation targets in MNI space: A subcortical atlas based on multimodal MRI, histology and structural connectivity. Neuroimage 170: , 271–282. |
[24] | Marek K , Chowdhury S , Siderowf A , Lasch S , Coffey CS , Caspell-Garcia C , Simuni T , Jennings D , Tanner CM , Trojanowski JQ , Shaw LM , Seibyl J , Schuff N , Singleton A , Kieburtz K , Toga AW , Mollenhauer B , Galasko D , Chahine LM , Weintraub D , Foroud T , Tosun-Turgut D , Poston K , Arnedo V , Frasier M , Sherer T ((2018) ) The Parkinson’s progression markers initiative (PPMI) - establishing a PD biomarker cohort. Ann Clin Transl Neurol 5: , 1460–1477. |
[25] | Wang Q , Akram H , Muthuraman M , Gonzalez-Escamilla G , Sheth SA , Oxenford S , Yeh F-C , Groppa S , Vanegas-Arroyave N , Zrinzo L , Li N , Kühn A , Horn A ((2020) ) Normative vs. patient-specific brain connectivity in deep brain stimulation. Neuroimage 224: , 117307. |
[26] | Mayka MA , Corcos DM , Leurgans SE , Vaillancourt DE ((2006) ) Three-dimensional locations and boundaries of motor and premotor cortices as defined by functional brain imaging: A meta-analysis. Neuroimage 31: , 1453–1474. |
[27] | Nachev P , Kennard C , Husain M ((2008) ) Functional role of the supplementary and pre-supplementary motor areas. Nat Rev Neurosci 9: , 856–869. |
[28] | Van Gerpen JA ((2006) ) Dopa-responsive dystonic camptocormia. Neurology 66: , 1779. |
[29] | Ho B , Prakash R , Morgan JC , Sethi KD ((2007) ) A case of levodopa-responsive camptocormia associated with advanced Parkinson’s disease. Nat Clin Pract Neurol 3: , 526–530. |
[30] | Yamada K , Goto S , Matsuzaki K , Tamura T , Murase N , Shimazu H , Nagahiro S , Kuratsu J , Kaji R ((2006) ) Alleviation of camptocormia by bilateral subthalamic nucleus stimulation in a patient with Parkinson’s disease. Parkinsonism Relat Disord 12: , 372–375. |
[31] | Lyons M , Boucher O , Patel N , Birch B , Evidente V ((2012) ) Long-term benefit of bilateral subthalamic deep brain stimulation on camptocormia in Parkinson’s disease. Turk Neurosurg 22: , 489–492. |
[32] | Herrington TM , Cheng JJ , Eskandar EN ((2016) ) Mechanisms of deep brain stimulation. J Neurophysiol 115: , 19–38. |
[33] | Mestre TA , Lang AE , Okun MS ((2016) ) Factors influencing the outcome of deep brain stimulation: Placebo, nocebo, lessebo, and lesion effects. Mov Disord 31: , 290–296. |
[34] | Vitek JL , Lyons KE , Bakay R , Benabid AL , Deuschl G , Hallett M , Kurlan R , Pancrazio JJ , Rezai A , Walter BL , Lang AE ((2010) ) Standard guidelines for publication of deep brain stimulation studies in Parkinson’s disease (Guide4DBS-PD). Mov Disord 25: , 1530–1537. |
[35] | Horn A ((2019) ) The impact of modern-day neuroimaging on the field of deep brain stimulation. Curr Opin Neurol 32: , 511–520. |
[36] | Stock AK , Wascher E , Beste C ((2013) ) Differential effects of motor efference copies and proprioceptive information on response evaluation processes. PLoS One 8: , e62335. |
[37] | Kenzie JM , Findlater SE , Pittman DJ , Goodyear BG , Dukelow SP ((2019) ) Errors in proprioceptive matching post-stroke are associated with impaired recruitment of parietal, supplementary motor, and temporal cortices. Brain Imaging Behav 13: , 1635–1649. |
[38] | Massion J , Viallet F , Massarino R , Khalil R ((1989) ) [The supplementary motor area is implicated in the coordination between posture and movement in man]. C R Acad Sci III 308: , 417–423. |
[39] | Fujita H , Kasubuchi K , Wakata S , Hiyamizu M , Morioka S ((2016) ) Role of the frontal cortex in standing postural sway tasks while dual-tasking: A functional near-infrared spectroscopy study examining working memory capacity. Biomed Res Int 2016: , 7053867. |
[40] | de Lima-Pardini AC , Coelho DB , Souza CP , Souza CO , Ghilardi M , Garcia T , Voos M , Milosevic M , Hamani C , Teixeira LA , Fonoff ET ((2018) ) Effects of spinal cord stimulation on postural control in Parkinson’s disease patients with freezing of gait. Elife 7: , e37727. |
[41] | Tsuru D , Watanabe T , Chen X , Kubo N , Sunagawa T , Mima T , Kirimoto H ((2020) ) The effects of transcranial static magnetic fields stimulation over the supplementary motor area on anticipatory postural adjustments. Neurosci Lett 723: , 134863. |
[42] | Akram H , Dayal V , Mahlknecht P , Georgiev D , Hyam J , Foltynie T , Limousin P , De Vita E , Jahanshahi M , Ashburner J , Behrens T , Hariz M , Zrinzo L ((2018) ) Connectivity derived thalamic segmentation in deep brain stimulation for tremor. Neuroimage Clin 18: , 130–142. |
[43] | Tagliati M ((2012) ) Turning tables: Should GPi become the preferred DBS target for Parkinson disease? Neurology 79: , 19–20. |
[44] | Odekerken VJ , Boel JA , Schmand BA , de Haan RJ , Figee M , van den Munckhof P , Schuurman PR , de Bie RM ; NSTAPS study group ((2016) ) GPi vs STN deep brain stimulation for Parkinson disease: Three-year follow-up. Neurology 86: , 755–761. |
[45] | Riley RD , Snell KIE , Ensor J , Burke DL , Harrell FE Jr. , Moons KGM , Collins GS ((2019) ) Minimum sample size for developing a multivariable prediction model: Part I - Continuous outcomes. Stat Med 38: , 1262–1275. |
[46] | Limousin P , Foltynie T ((2019) ) Long-term outcomes of deep brain stimulation in Parkinson disease. Nat Rev Neurol 15: , 234–242. |