The Effects of Respiratory Training in Parkinson’s Disease: A Systematic Review
Abstract
Background:
Signs of respiratory dysfunction can be present already early in the course of Parkinson’s disease (PD). Respiratory training could alleviate this, but its effectiveness is not well understood.
Objective:
The purpose of this systematic review is to review the efficacy of different respiratory training interventions in PD.
Methods:
A search strategy was performed in four databases: PubMed, Physiotherapy Evidence Database (PEDro), Cochrane Library, and Cumulative Index to Nursing and Allied Health Literature (CINAHL). Methodological quality of original full-text articles was assessed using the Cochrane Risk of Bias tool for randomized controlled trials (RCTs) and the Risk Of Bias In Non-randomized Studies of Interventions (ROBINS-I) tool for the controlled trials (CTs). Levels of evidence were rated by the Grading of Recommendation Assessment, Development and Evaluation (GRADE) approach.
Results:
Six papers reporting on four randomized controlled trials and another four controlled trials were included. Positive effects were reported for inspiratory muscle strength training (IMST), expiratory muscle strength training (EMST), air stacking, breath-stacking, incentive spirometry and postural training on respiratory muscle strength, swallowing safety, phonatory aspects and chest wall volumes. Best methodological quality was found for breath-stacking and incentive spirometry. Best levels of evidence were found for EMST, IMST and EMST plus air stacking.
Conclusion:
Respiratory training shows positive effects and should be considered when people with PD experience respiratory dysfunction. Future studies should focus on standardizing both training devices, instruments to measure outcomes and intervention protocols to further increase the level of evidence.
INTRODUCTION
Respiratory dysfunction is a relatively unknown feature in Parkinson’s disease (PD), even though inspiratory muscle weakness may be present already early in the course of the disease [1]. The rate of progression throughout the disease is unclear and, importantly, there is uncertainty about the optimal management approach. In the more advanced stages, a limited lung expansion and chest compliance contributes to less effective coughing, which is especially relevant for those with dysphagia. The latter causes penetration or aspiration of saliva, liquid or food [2, 3]. This could lead to the occurrence of aspiration pneumonia [4], which is among the highest risks factors for mortality in the advanced stages of PD [5, 6].
Box 1 offers an oversight of commonly used outcome measurements of respiratory training as the respiratory function tests, swallowing outcomes and phonatory aspects measures. Compared to age-matched healthy controls, both obstructive and restrictive features can be found in persons with PD, as reflected by reduced values of the FVC, FEV1, MVV, MIP and MEP [7]. Improving FVC, FEV1, MVV, MIP and MEP using respiratory training is already an established intervention in patients with a range of neuromuscular disorders [8, 9], but is relatively new in neurodegenerative disorders. For example, lung volume-orientated training improves respiratory function tests such as FVC and peak cough expiratory flow in patients with amyotrophic lateral sclerosis [10]. Also, cut-off scores to determine when respiratory training is indicated are available for neuromuscular diseases, but are so far lacking for PD [11, 12]. What sets PD apart from neuromuscular disorders is the fact that, besides muscle weakness (which is present for both types of conditions) also muscle control seems to be even more affected in persons with PD due to bradykinesia and rigidity [2, 13, 14]. From this perspective, not only respiratory muscle strength training is an option in PD, but also muscle control training. With muscle control training we mean targeting bradykinesia and rigidity in terms of improving reduced chest compliance, amplitude and pulmonary expansion [15].
The number of respiratory training studies in PD is increasing and more different modalities of respiratory training interventions have become available in the last decade. Against this background, our purpose here is to perform a systematic review presenting the efficacy of different respiratory training interventions in PD. We will also discuss the clinical implication of respiratory training in PD.
Box 1. Outcome measurements of respiratory training | |
Respiratory function tests | |
Abbreviation | |
FEV1 | Forced expiratory volume in 1 second |
FVC | Forced vital capacity |
MEP | Maximal expiratory pressure |
MIP | Maximal inspiratory pressure |
MV | Minute ventilation |
MVV | Maximum voluntary ventilation |
PDQ-39 | Parkinson disease questionnaire 39 |
PEF | Peak expiratory flow |
PmPeak | Inspiratory muscle endurance |
POD | Perception of Dyspnea |
QoL (SF-36) | Quality of Life (medical outcomes study 36-item short form health survey) |
r-PCF | Reflex peak cough flow |
SVC | Slow vital capacity |
TV | Tidal volume |
VFS | Videofluoroscopic studies |
v-PCF | Voluntary peak cough flow |
VT,rca | Abdominal ribcage tidal volume |
VT,rcp | Pulmonary rib cage tidal volume |
Swallowing outcomes | |
Abbreviation | |
PAS | Penetration-aspiration scale |
SWAL-QoL | Swallowing Quality of Life |
Questionnaire | |
Phonatory capacity | |
Abbreviation | |
MPT | Maximum phonation time |
Peak SGP | Peak subglottic pressure |
MATERIALS AND METHODS
The process of this systematic review was reported according to the guidelines for PRISMA (Preferred Reporting Items for Systematic Reviews and Meta-Analyses) [16].
Data sources and searches
A broad literature search was performed for four databases: PubMed, Physiotherapy Evidence Database (PEDro), Cochrane Library and Cumulative Index to Nursing and Allied Health Literature (CINAHL). From inception of the databases until December 31 2019, a search strategy was created using ‘Parkinson’s disease’ as the patient, ‘respiratory training’ and related search terms as the intervention, and ‘other training interventions or control group’ as comparison. Because the pathophysiology of respiratory dysfunction is not fully understood, we decided to search without limitations with respect to the outcome measures, respiratory training interventions and control group. The PubMed search is presented in the Supplementary Material.
Study selection
Two authors (VvdW, JK) independently screened the articles identified by the search strategy on title and abstract. Inclusion criteria were randomized controlled trials (RCTs) or controlled trials (CTs). Exclusion criteria were non-controlled studies, case reports, reviews and abstracts. In addition, the reference lists of each of the selected publications were screened for title and abstract for additional relevant articles. The included publications, relevant by domain and determinant, were read in full text.
Data extraction
The extracted data from the articles includes the following characteristics: study design, participants (number of participants, sex, age and Hoehn & Yahr stage), training protocol, outcome measures, summary of results and effect size. The data were extracted by the first author (VvdW) and checked by the second author (MN).
Methodological quality assessment
The methodological quality of the included articles was rated independently by two reviewers (VvdW, MN). To assess the risk of bias of the RCTs, the Cochrane Risk of Bias tool was used.[17] Risk of bias was assessed within seven domains: 1) random sequence generation, 2) allocation concealment, 3) blinding of participants and personnel, 4) blinding of outcome assessment, 5) incomplete outcome data, 6) selective reporting, 7) other sources of bias. A summary of the methodological quality was given; a green plus symbol corresponds with a ‘low risk of bias’ (+), a red minus symbol corresponds with a ‘high risk of bias’ (–) and a yellow question mark symbol corresponds with an ‘unclear risk of bias’ (?).
The Risk Of Bias In Non-randomized Studies of Interventions (ROBINS-I) tool was used to assess the risk of bias for the CTs [18]. The ROBINS-I tool evaluated the risk of bias for quantitative studies that compare the efficacy of an intervention in two or more groups of individuals. This tool includes seven domains: 1) bias due to confounding, 2) bias in selection of participants into the study, 3) bias in classification of interventions, 4) bias due to deviations from intended interventions, 5) bias due to missing data, 6) bias in measurement of outcomes, and 7) bias in selection of the reported results. Each of these domains could be rated as: ‘low risk’, ‘moderate risk’, ‘serious risk’, ‘critical risk’, or ‘no information’. A rating of the overall risk of bias was given as described in Table 1 [18].
Table 1
Interpretation of domain-level and overall risk of bias judgements in ROBINS-I [18]
Judgement | Within each domain | Across domains | Criterion |
Low risk of bias | The study is comparable to a well-performed randomized trial with regard to this domain | The study is comparable to a well-performed randomized trial | The study is judged to be at low risk of bias for all domains. |
Moderate risk of bias | The study is sound for a non-randomized study with regard to this domain but cannot be considered comparable to a well-performed randomized trial | The study provides sound evidence for a nonrandomized study but cannot be considered comparable to a well-performed randomized trial | The study is judged to be at low or moderate risk of bias for all domains. |
Serious risk of bias | the study has some important problems in this domain | The study has some important problems | The study is judged to be at serious risk of bias in at least one domain, but not at critical risk of bias in any domain. |
Critical risk of bias | the study is too problematic in this domain to provide any useful evidence on the effects of intervention | The study is too problematic to provide any useful evidence and should not be included in any synthesis | The study is judged to be at critical risk of bias in at least one domain |
No information | No information on which to base a judgement about risk of bias for this domain | No information on which to base a judgement about risk of bias | There is no clear indication that the study is at serious or critical risk of bias and there is a lack of information in one or more key domains of bias (a judgement is required for this). |
Data synthesis and analysis
The heterogeneity of the interventions, training protocols as well as the wide variety of primary outcome measures made it impossible to pool the results in meta-analyses. Instead, we present a narrative synthesis organized by intervention and training protocols and outcome measures. The Grading of Recommendation Assessment, Development and Evaluation (GRADE) approach was used to rate level of evidence into “high”, “moderate”, “low”, or “very low” [19]. Detailed GRADE guidance was used to evaluate the risk of bias, imprecision, inconsistency, indirectness, and publication bias. These were all reasons to downgrade the level of evidence [20, 21]. Upgrading the level of evidence was possible in case of a large effect size, evidence of dose-response gradient, or all plausible confounding factors reducing an apparent effect.
RESULTS
Study inclusion
The process of article inclusion according to PRISMA is presented in Fig. 1. The search revealed 760 records. After screening titles and abstracts, 736 records were excluded, based on duplicates and either no ‘Parkinson disease’, no ‘respiratory training’ or both. The remaining eligible 24 articles were assessed based on their full text version, resulting in 10 papers that met the inclusion criteria for this review: six papers reporting on (four) RCTs, and four papers on CTs. These were published between 1997 and 2019 and were conducted worldwide including Chile (3), the United States of America (2), Brazil (2), Israel (1), Korea (1) and Taiwan (1). The study characteristics and outcomes are presented in Table 2.
Fig. 1
Flow Diagram for article inclusion PRISMA.
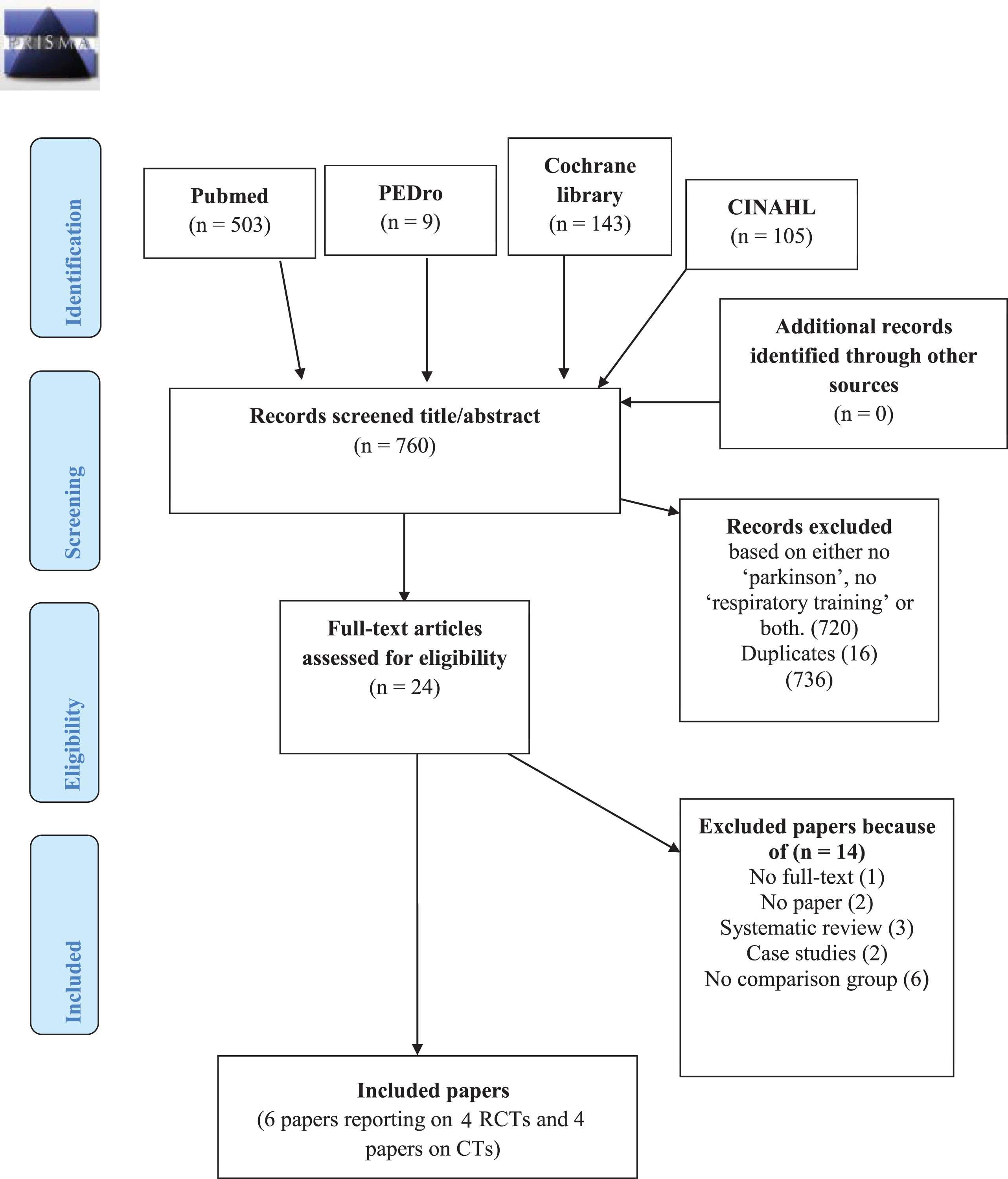
Table 2
Overall characteristics of the included studies
Study | Participants | Training protocol | Outcome measures | Results and effect size |
Inzelberg et al. 2005 [29] | Intervention: 10. | Intervention: | FVC | IMST vs control: |
RCT | 9 men | IMST | FEV1 | FVC and FEV1: NS |
Age: 59.4±4.9 years | 30 min per session | Inspiratory muscle endurance | ↑ inspiratory muscle endurance∗↑ MIP∗ | |
Control:10. | 6 d/wk | MIP | ||
9 men. | 12 wk | POD | ↓ POD∗QoL (SF-36): NS | |
Age: 65.2±3.6 years | 15% MIP first week. | QoL (SF-36) | ||
H&Y stage: II - III | Increased 5% -10% each session to reach 60% MIP at end of first month. | Correlation between improvement in MIP and endurance and decrease in | ||
Monthly reset to 60% of the MIP. | POD in IMST group (R2 = 0.571 and | |||
Control: | R2 = 0.423, p < 0.001). | |||
Same protocol with fixed resistance of 7cm H2O. | ||||
Troche et al. 2010 [22]** | Intervention: 30. | Intervention: | Primary outcome | EMST vs control: |
PAS | ↑ PAS (d = 0.55)* | |||
Secondary outcomes | Duration of hyoid elevation: NS | |||
Duration of hyoid elevation Hyoid displacement | ↑ hyoid displacement during swallowing* | |||
↑ SWAL-QoL* | ||||
RCT | 25 men | EMST | SWAL-QoL | |
Age: 66.7±8.9 years | 5 sets | |||
Control: 30. | 5 repetitions | |||
22 men | 5 d/wk | |||
Age: 68.5±10.3 years | 4 wk | |||
H&Y stage: II - IV | 75% MEP, adjusted weekly | |||
Control: | ||||
Same protocol without load | ||||
Sapienza et al. 2011 [23]** | Intervention: 30. | Intervention: | Primary outcome: | EMST vs control: |
RCT | 25 men | EMST | MEP | 27% ↑ MEP* |
Age: 66.7±8.9 years | 5 sets | Secondary outcomes: | Secondary outcomes: NS | |
Control: 30. | 5 repetitions | FEV1 | ||
22 men | 5 d/wk 4 wk 75% MEP, adjusted weekly | FEV1/FVC | ||
Age: 68.5±10.3 years | Control: | FVC | ||
H&Y stage: II - III | Same protocol without load | PEF | ||
Reyes et al. 2018 [25]*** | 31 patients, 17 men | Intervention | FVC, | EMST vs control: |
RCT | Age: 70.5±8.2 years | -Home-based IMST | MEP, | MEP (d = 1.40), v-PCF (d = 0.89), r-PCF (d = 0.27), SVC (d = 0.13), FVC (d = 0.02) |
H&Y stage: I - III | -Home-based EMST | MIP, | IMST vs control: | |
Control: home-based EMST, fixed resistance | r-PCF, | MIP (d = 0.76), v-PCF (d = 0.08) | ||
5 sets | SVC, | |||
5 repetitions | v-PCF | |||
6 d/wk | ||||
2 months | ||||
50% average MIP/MEP: adjusted until 75% last 2 wks. | ||||
Ribeiro et al. 2018 [15] | 14 patients, 9 men | Intervention | Volume variations of the chest wall before, immediately after, 15 min after and 30 min after intervention. | BS and IS: ↑ TV and MV* |
RCT | Age: 65.6±9.0 years | -Breath-Stacking (one-way valve, ventilometer) | Performed by optoelectronic plethysmography. | IS: ↑ VT,rcp and VT,rca* |
H&Y stage: I - III | -Incentive spirometer techniques (voldyne 5000). | |||
All participants confirmed both interventions and participated in control protocol. | Control: no intervention. | |||
3 sets | ||||
5 repetitions | ||||
35 sec interval between set. | ||||
4 non-consecutive days. Day1: general data. Day 2/3/4: BS/IS/control. | ||||
Reyes et al. 2019 [30]*** | 31 patients, 17 men | Intervention: | Primary outcome | IMST vs control |
RCT | Age: 70.5±8.2 years | -Home-based IMST | MPT | MPT (d = 1.26) |
H&Y stage: I - III | -Home-based EMST | Peak SGP | Peak SGP (d = 1.32)Peak sound pressure level (d = 1.27)EMST vs controlPeak SGP (d = 1.96)Peak sound pressure level (d = 1.10) | |
Control: home-based EMST, fixed resistance | Peak sound pressure level | |||
5 sets | ||||
5 repetitions | ||||
6 d/wk | ||||
2 months | ||||
50% average MIP/MEP: adjusted until 75% last 2 wks. | ||||
Reyes et al. 2019 [26] | Intervention: | Intervention: | Primary outcome | EMST vs control |
CT | Group EMST: 11, | EMST | r-PCF, | v-PCF (d = 0.77) |
7 men | 5 sets | v-PCF | r-PCF (d = 0.32) | |
Age: 69.81±6.75 years | 5 repetitions | Secondary outcome | MEP (d = 1.07) | |
Group 2 EMST+AS: 11, 6 men | 6 d/wk | SVC | MIP (d = 0.19) | |
Age: 65.81±7.35 years | 2 months | MIP | EMST+AS vs control | |
Control: | 50% average MEP: adjusted until 75% last 2 wks. | MEP | v-PCF (d = 1.00) | |
11, 5 men | EMST+AS: | r-PCF (d = 1.34) | ||
Age: 70.45±6.4 years | Same protocol for EMST | MEP (d = 0.58) | ||
H&Y stage: I-III | 10 sets | MIP (d = 0.57) | ||
3-4 consecutive lung insufflations using a manual resuscitator bag | SVC showed small effects for both interventions | |||
Control: | ||||
EMST with same protocol, fixed resistance on 9cm H2O | ||||
Frazao et al. 2014 [27] | Intervention: 15. | Intervention: | Spirometric assessment | Intervention vs quiet breathing: |
CT | 12 men | EMST with 10, 15 or 20 cm H20. | Respiratory muscle strength | ↑ TV in all PEP levels* |
Age: 59.1±9.3 years | Control: Quiet breathing | Chest wall volumes and long volume variables measured by optoelectronic plethysmography | ↑ end-inspiratory and expiratory chest wall volume in all positive expiratory pressure levels* | |
H&Y stage: II-III | Measurements at baseline, during EMST, after EMST. | |||
Control (healthy individuals): 15. | ||||
12 men | ||||
Age: 58.8±9.0 years | ||||
Byeon 2016 [28] | 33 patients. 31 men. | Intervention: | Functional | ↓ in VFS for both groups.* |
CT | EMST group: 18Age: 63.8±8.2 years | EMST (n = 18)8 repetitions | Dysphagia Scale based on video fluoroscopic studies (VFS) | Greater decrease in EMST+PT group than in the EMST-only group.* |
EMST+PT group: 15 | 30 sec rest | |||
Age: 65.1±9.5 years | 20 min/d | |||
H&Y stage: 0-V. | 5 d/wk | |||
4 wk | ||||
75% MEP | ||||
EMST+PT (n = 15): | ||||
PT: chin tucking, head rotation, head tilting, bending head back, and lying down straight | ||||
30 minutes per session | ||||
5 d/wk | ||||
4 wk | ||||
Kuo et al. 2017 [24] | Intervention: | Intervention: | MEP | Bigger improvement in MEP EMST-5DE (88% increase of MEP) compared to EMST-3DE (61% increase in MEP)*. PDQ-39: only significant increase in mobility score*. |
CT | Group 1 5DE: 4. | Group 1: EMST-5DE | PDQ-39 | |
2 men | 5 sets | |||
Age: 59.3±3.8 years | 5 repetitions | |||
Group 2 3DE: 5 | 5 d/wk | |||
3 men | 4 wk | |||
Age: 58.4±6.6 years | 75% MEP | |||
Control: 4 | 75% average MEP: weekly reset. | |||
2 men | Group 2: EMST-3DE | |||
Age: 60.5±6.1 years | 5 sets | |||
H&Y stage: I -III | 5 repetitions | |||
3 d/wk | ||||
4 wk | ||||
75% MEP | ||||
75% average MEP: weekly reset. | ||||
Control: | ||||
Same protocol | ||||
3 d/wk | ||||
EMST training without load |
RCT, randomized controlled trial; CT, controlled trail; *Significant change; NS, no significant change; PD, Parkinson disease; NA, not applicable; H&Y stage, Hoehn and Yahr stage; IMST, inspiratory muscle strength training; EMST, expiratory muscle strength training; FVC, forced vital capacity; FEV1, forced expiratory volume in 1 second; POD, Perception of Dyspnea; QoL, quality of life; SF-36, medical outcomes study 36-item short form health survey; PEF, peak expiratory flow; SGP, subglottic pressure, MPT, maximum phonation time; SWAL-QoL, Swallowing Quality of Life Questionnaire; TV, Tidal volume; MV, minute ventilation; VT,rcp, pulmonary rib cage tidal volume; VT,rca, abdominal ribcage tidal volume; MVV, maximum voluntary ventilation; SVC, slow vital capacity; MIP, maximal inspiratory pressure; MEP, maximal expiratory pressure; PAS, penetration-aspiration scale; PDQ-39 scale, Parkinson disease questionnaire 39; r-PCF, reflex peak cough flow; v-PCF, voluntary peak cough flow; PT, postural techniques. **Troche et al. [22] and Sapienza et al. [23] used the same population and intervention, but other outcome measurements. ***Two studies from Reyes et al. [25, 30] used the same population and intervention, but other outcome measurements.
Participants
The median sample size for all studies was 30.5 and ranged from nine to 60 participants, with mean ages ranging from 58.8 to 70.5 years within an overall woman-men ratio of 7:10.
Most studies included persons with PD in Hoehn & Yahr stage I to III. Information about disease duration in years or disease severity (as measured by the Unified Parkinson’s Disease Rating Scale ((MDS-)UPDRS) scores) was given in only three studies.
Intervention and training protocols
Box 2 briefly summarizes the types of interventions and training devices that have been tested in persons with PD. The interventions in the RCTs were IMST only in one study, EMST only in two studies, both IMST and EMST in two studies, and breath-stacking and incentive spirometer techniques in one study. The interventions of the four CTs were EMST only in two studies, EMST complemented by postural techniques in one study, and EMST complemented by air stacking in one study. Three different devices were used for IMST and EMST: Threshold® (Philips Respironics, USA), POWERbreathe® (Southam, Warwickshire, UK), and EMST 150 (Aspire products LLC., USA). Training protocols and parameters were all different except for three EMST reports in which the same protocol was used consisting of 5 sets, 5 repetitions, 5 days per week for 4 weeks on 75% MEP [22– 24].
Box 2. Types of respiratory training interventions and training devices | |||
Abbreviation | Written in full | Aim intervention | Performance and devices |
AS | Air stacking | Increase the inspiratory phase to improve cough effectiveness. | Stacking air behind the glottis by performing consecutive lung insufflations using a manual resuscitator bag. |
BS | Breath-stacking | Increase long volumes, target lung expansion and prevent atelectasis. | Stacking air behind the glottis by performing successive breaths until no inspiratory volume was observed using a ventilometer (Ferraris Mark Wright ® 8, Middlesex, England). |
EMST | Expiratory muscle strength training | Improve respiratory muscle strength, lung volumes, swallowing function and phonatory capacity. | Perform maximum expiratory muscle flows against resistance using a training device as EMST 150 (Aspire products LLC., USA), Threshold® (Philips Respironics, USA) or PEP valve (Vital Signs Inc., Totowa, NJ, United States) |
IMST | Inspiratory muscle strength training | Improve respiratory muscle strength and phonatory capacity. | Perform maximum inspiratory muscle flows against resistance using a training device as POWERbreathe® (Southam, Warwickshire, UK) or Threshold® (Philips Respironics, USA). Aims to improve respiratory muscle strength. |
IS | Incentive spirometer techniques | Increase lung volumes, target lung expansion and prevent atelectasis. | Performing a slow and deep breathing to total lung capacity has been reached, using an incentive spirometry (Voldyne 5000 ®; Sherwood Medical, St Louis, USA). |
PT | Postural training | Conduct as swallowing intervention. | General postural compensation techniques were conducted being chin tuck, head rotation, head tilting, bending head back, and lying down. |
Outcome measurement
Outcome measures used in the reviewed studies were several respiratory function tests (MEP was most frequently used), swallow function tests, quality of life scales and different optoelectronic plethysmography variables. All 10 studies showed a statistically significant positive effect of the intervention on the primary outcome compared to the control group (Table 2). Two different instruments were used to measure respiratory muscle strength: a pressure manometer (FLUKE 713– 30 G [Fluke Corp., Everett, WA]) and the MICRO RPM respiratory pressure meter (MicroRPM; Micro Medical-Care Fusion, UK).
Expiratory muscle strength training
Three RCTs reported positive effects of EMST on the primary outcome compared to control group. Three RCTs, with different training protocols, showed a Cohen’s d effect size between EMST and control group. A large effect was found for peak subglottic pressure (d = 1.96), MEP (d = 1.4), peak sound pressure level (d = 1.10) and voluntary PCF (d = 0.89). EMST and control showed a moderate effect on penetration aspiration score (PAS) (d = 0.55)and reflex PCF (d = 0.27) [22, 23, 25].
Four CTs reported positive effects of EMST compared to control group. The Cohen’s d effect size between EMST and control group was large for MEP (d = 1.07) moderate for voluntary PCF (d = 0.77) and small for reflex PCF (d = 0.32) [26]. An 88% increase was found on MEP for EMST-5DE compared to the control group [24]. Compared to quiet breathing, EMST with different resistance settings (10, 15 or 20 cm H2O) improved tidal volume and end-inspiratory chest wall volume (p < 0.001) [27]. Both EMST only and EMST combined with postural techniques enhanced the swallowing safety in persons with dysphagia caused by PD (p < 0.05) [28].
Inspiratory muscle strength training
Three RCTs performed IMST with significant positive effects on the primary outcome. In the first RCT, twelve weeks of IMST training significantly increased MIP by 25.8%, inspiratory muscle endurance by 45% and perception of dyspnea decreased by 27.8% compared to the control group (n = 20) [29]. The second RCT showed a moderate Cohen’s d effect size between IMST and control group on MIP (d = 0.76) after two months of IMST training [25]. In the same RCT, two months of IMST (5 sets, 5 repetitions, 6 days/week on 50% MEP) showed large effect sizes for peak subglottic pressure (d = 1.32), peak sound pressure level (d = 1.27) and maximum phonation time (d = 1.26) when compared to the control group.[30]
Other interventions
Breath-stacking and incentive spirometer techniques were found to directly increase tidal volume and minute ventilation measured by optoelectronic plethysomography [15]. EMST complemented by air stacking showed a large Cohen’s d effect size compared with the control group on r-PCF (d = 1.34) and v-PCF (d = 1.00) [26].
Quality assessment
Table 3 shows the quality assessments of the Cochrane risk of bias tool for the RCTs. Ribeiro et al. [15] showed a low risk of bias with a sample size of 14 persons with PD. Troche et al. [22] and Sapienza et al. [23] included 30 persons with PD and showed a low risk of bias except for unclear generation of a randomized sequence (selection bias). Inzelberg et al. [29] included 20 participants with an inadequate concealment of allocations prior to assignment (selection bias) and data from two persons from the training group with a low compliance to the training regime were left out of the analysis. Attempts were made to keep the participants blinded (by using sham devices) for at least the intervention. Blinding of outcome assessment was guaranteed in all studies, except for the two papers of Reyes et al. [25, 30]. Although in both papers of Reyes et al., the attempts to ascertain blinding of the personnel that provided instructions for training was unclear, we decided to rate all six RCTs for low risk of bias on the criterion ‘blinding of participants and personnel’. We downgraded both papers of Reyes et al. for criteria 4 ‘blinding of outcome assessment’ as the researchers were not blinded for group allocation. Both papers of Reyes et al. showed also a risk of bias because no information about the compliance of the training regime (home-based exercise program) was reported, and some participants were excluded from the analysis for unknown reasons.
Table 3
Cochrane Handbook for Systematic Reviews of Interventions quality assessment RCTs
![]() |
Table 4
ROBINS-I tool quality assessment non-randomized controlled intervention studies (CTs)
![]() |
Assessment of the methodological quality by both authors (VvdW, MN) showed an agreement for risk of bias assessment in five of the six RCTs. Disagreement for one study was caused by the lack of information about compliance to the training regime, but consensus was reached that this could lead to a high risk of performance bias.
Table 4 showed the results of the ROBIN-I checklist for the four included CTs. Both authors (VvdW, MN) indicated a serious risk of bias in three of the studies, and a critical risk of bias in one study. Frazao et al. showed a serious risk of bias because there was no blinding of outcome assessors.[27] Next to that, the aim and conclusion of this study suggested that PD patients were compared to healthy subjects. However, the methods and results section showed a comparison between three different levels of expiratory resistance, compared to no resistance. The study of Haewon Byeon scored a serious risk of bias because the intervention protocol for the postural techniques and the measurement procedure of MEP were not described [28], limiting the reproducibility of this study. Reyes et al. showed a serious risk of bias because there was no blinding for outcome assessment as the researchers were not blinded for group allocation [26]. Kuo et al. showed a critical risk of bias because of a lack of randomization and reporting incomplete data of four of the thirteen participants [24].
Levels of evidence
Table 5 shows the levels of evidence per intervention and specified outcomes according to the GRADE approach. Out of 26 outcomes included in this study, 22 were measured in only one study. For these 22 outcomes, inconsistency in results could not be determined. Detecting risk of publication bias was complicated due to the limited number of studies for each outcome. This made it impossible to calculate funnel plots. However, nine out of the ten included studies presented both significant and non-significant results.
Table 5
GRADE evidence profile per outcome measurement
Intervention | Outcome | No. of participants (studies) | Results | Risk of biasa | Inconsistencyb | Indirectnessc | Imprecisiond | Publication Biase | Large effect | Dose-response | Residual bias | Levels of evidence (GRADE) |
EMST | MEP | 115 (4) | Reyes’18 (RCT): ↑ MEP (d = 1.4) Reyes’19 (CT): ↑ MEP (d = 1.07) Sapienza’11 (RCT): ↑ MEP (F = 3.214, P < 0.01) Kuo’17 (CT): 88% ↑ of 5 days EMST vs 3 days EMST | Serious risk of bias | Serious inconsistency | Serious indirectness | Very serious imprecision | Not detected | Detected | Not detected | Not detected | (+)()()() Very low |
v-PCF | 42 (2) | Reyes’19 (CT): ↑ v-PCF (d = 0.77) Reyes’18 (RCT): ↑ v-PCF (d = 0.89) | Serious risk of bias | No serious inconsistency | No serious indirectness | Very serious imprecision | Not detected | Not detected | Not detected | Not detected | (+)(+)()() Low | |
r-PCF | 42 (2) | Reyes’19 (CT): ↑ r-PCF (d = 0.32) Reyes’18 (RCT): ↑ r-PCF (d = 0.27) | Serious risk of bias | No serious inconsistency | No serious indirectness | Serious imprecision | Not detected | Not detected | Not detected | Not detected | (+)(+)()() Low | |
PAS | 60 (1) | Troche’10 (RCT): ↑ PAS (d = 0.55) | No serious risk of bias | NA | Serious indirectness | No serious imprecision | Not detected | Not detected | Not detected | Not detected | (+)(+)(+)() Moderate | |
SVC | 31 (1) | Reyes’18 (RCT): ↑ SVC (d = 0.13) | Serious risk of bias | NA | No serious indirectness | Very serious imprecision | Not detected | Not detected | Not detected | Not detected | (+)()()() Very low | |
FVC | 31 (1) | Reyes’18 (RCT): ↑ FVC (d = 0.02) | Serious risk of bias | NA | No serious indirectness | Very serious imprecision | Not detected | Not detected | Not detected | Not detected | (+)()()() Very low | |
Peak SGP | 31 (1) | Reyes’19 (RCT): ↑ Peak SGP (d = 1.32) | Serious risk of bias | NA | No serious indirectness | Serious imprecision | Not detected | Detected | Not detected | Not detected | (+)(+)(+)() Moderate | |
Peak sound pressure level | 31 (1) | Reyes’19 (RCT): ↑ Peak sound pressure level (d = 1.27) | Serious risk of bias | NA | No serious indirectness | Serious imprecision | Not detected | Detected | Not detected | Not detected | (+)(+)(+)() Moderate | |
Chest wall volumes | 30 (1) | Frazao’14 (CT): ↑ TV in all EMST levels* ↑ end-inspiratory and expiratory chest wall volume in all EMST levels* | ||||||||||
Serious risk of bias | NA | No serious indirectness | Serious imprecision | Not detected | Not detected | Not detected | Not detected | (+)(+)()() Low | ||||
Functional dysphagia scale by VFS | 33 (1) | Byeon’16 (CT): ↓ in VFS for both groups.* | Serious risk of bias | NA | Very serious indirectness | Serious imprecision | Detected | Not detected | Not detected | Not detected | (+)()()() Very low | |
PDQ-39 | 13 (1) | Kuo’17 (CT): Only significant increase in mobility score*. | Very serious risk of bias | NA | Very serious indirectness | Very serious imprecision | Not detected | Not detected | Not detected | Not detected | (+)()()() Very low | |
EMST+AS | v-PCF | 33 (1) | Reyes’19 (CT): ↑ v-PCF (d = 1.00) | Serious risk of bias | NA | No serious indirectness | Serious imprecision | Not detected | Not detected | Not detected | Not detected | (+)(+)()() Low |
r-PCF | 33 (1) | Reyes’19 (CT): ↑ r-PCF (d = 1.34) | Serious risk of bias | NA | No serious indirectness | Serious imprecision | Not detected | Detected | Not detected | Not detected | (+)(+)(+)() Moderate | |
MEP | 33 (1) | Reyes’19 (CT): ↑ MEP (d = 0.58) | Serious risk of bias | NA | No serious indirectness | Serious imprecision | Not detected | Not detected | Not detected | Not detected | (+)(+)()() Low | |
MIP | 33 (1) | Reyes’19 (CT): ↑ MIP (d = 0.57) | Serious risk of bias | NA | Serious indirectness | Serious imprecision | Not detected | Not detected | Not detected | Not detected | (+)()()() Very low | |
IMST | MIP | 41 (2) | Reyes’18 (RCT): ↑ MIP (d = 0.76) ↑ 2 cm H2O for MIP after IMST. Inzelberg’05 (RCT): ↑ 16 cm H2O in MIP | High risk of bias | Very serious inconsistency | Very serious indirectness | Very serious imprecision | Not detected | Not detected | Not detected | Not detected | (+)()()() Very low |
v-PCF | 31 (1) | Reyes’18 (RCT): ↑ v-PCF (d = 0.08) | Serious risk of bias | NA | No serious indirectness | Very serious imprecision | Not detected | Not detected | Not detected | Not detected | (+)()()() Very low | |
MPT | 31 (1) | Reyes’19 (RCT): ↑ MPT (d = 1.26) | Serious risk of bias | NA | No serious indirectness | Serious imprecision | Not detected | Detected | Not detected | Not detected | (+)(+)(+)() Moderate | |
Peak SGP | 31 (1) | Reyes’19 (RCT): Peak SGP (d = 1.32) | Serious risk of bias | NA | No serious indirectness | Serious imprecision | Not detected | Detected | Not detected | Not detected | (+)(+)(+)() Moderate | |
Peak sound pressure level | 31 (1) | Reyes’19 (RCT): Peak sound pressure level (d = 1.27) | Serious risk of bias | NA | No serious indirectness | Serious imprecision | Not detected | Detected | Not detected | Not detected | (+)(+)(+)() Moderate | |
FVC | 20 (1) | Inzelberg’05 (RCT): FVC: NS | Serious risk of bias | NA | Very serious indirectness | Very serious imprecision | Not detected | Not detected | Not detected | Not detected | (+)()()() Very low | |
FEV1 | 20 (1) | Inzelberg’05 (RCT): FEV1: NS | Serious risk of bias | NA | Very serious indirectness | Very serious imprecision | Not detected | Not detected | Not detected | Not detected | (+)()()() Very low | |
PmPeak | 20 (1) | Inzelberg’05 (RCT): ↑ inspiratory muscle endurance* | Serious risk of bias | NA | Very serious indirectness | Serious imprecision | Not detected | Not detected | Not detected | Not detected | (+)()()() Very low | |
POD | 20 (1) | Inzelberg’05 (RCT): ↓ POD* | Serious risk of bias | NA | Very serious indirectness | Very serious imprecision | Not detected | Not detected | Not detected | Not detected | (+)()()() Very low | |
QoL (SF-36) | 20 (1) | Inzelberg’05 (RCT): QoL (SF-36): NS | Serious risk of bias | NA | Very serious indirectness | Very serious imprecision | Not detected | Not detected | Not detected | Not detected | (+)()()() Very low | |
BS + IS | Chest wall volume | 14 (1) | Ribeiro’18 (RCT): BS and IS: ↑ TV and MV* IS: ↑ VT,rcp and VT,rca* | Low risk of bias | NA | Serious indirectness | Serious imprecision | Not detected | Not detected | Not detected | Not detected | (+)(+)()() Low |
a issues related to inadequate allocation concealment, incomplete outcome data, blinding of outcome assessors and missing data; b issues related to differences in trainings protocol, follow-up time, intervention in control group, disease severity, outcome values, effect sizes; c issues related to indirect comparison of outcomes and use of control group, limit generalizability, only before-after measurements; d issues related to small sample sizes, different effect sizes and confidence intervals. e no funnel plots, because limit number of studies per outcome; No., number; GRADE, Grading of Recommendation Assessment, Development and Evaluation; NA, not applicable; EMST, expiratory muscle strength training; EMST + AS, expiratory muscle strength training + air stacking; IMST, inspiratory muscle strength training; BS + IS, breath stacking + Incentive spirometer techniques; MEP, maximal expiratory pressure; v-PCF, voluntary peak cough flow; r-PCF, reflex peak cough flow; PAS, penetration-aspiration scale; SVC, slow vital capacity; FVC, forced vital capacity; SGP, subglottic pressure; VFS, videofluoroscopic studies; PDQ-39 scale, Parkinson disease questionnaire 39; MIP, maximal inspiratory pressure; MPT, maximum phonation time; FEV1, forced expiratory volume in 1 s; PmPeak, inspiratory muscle endurance; POD, Perception of Dyspnea; QoL, quality of life; SF-36, medical outcomes study 36-item short form health survey; TV, Tidal volume; MV, minute ventilation; VT,rcp, pulmonary rib cage tidal volume; VT,rca, abdominal ribcage tidal volume.
For EMST, 11 different outcomes were included in the studies. The MEP was measured in four studies and was scored as “very low”. Reasons for downgrading were a serious risk of bias, inconsistency because of different outcome values and no comparison of outcomes between intervention and control group, indirectness by differences in follow-up time, a limited generalizability (severe PD was not included), and imprecision by small sample sizes. Despite an upgrade of evidence because of the large effect sizes in the four studies, the “very low” score remains. V-PCF and r-PCF were measured in two studies and scored as “low” due to serious risk of bias, small sample sizes and wide confidence intervals. All other outcomes were only measured in one study. The PAS, peak SGP and peak sound pressure level were scored as “moderate” as they only showed limitations in terms of small sample sizes and serious risk of bias caused by reporting incomplete outcome data and an unclear allocation concealment. Large effect sizes upgraded the level of evidence for peak SGP and peak sound pressure level. One study combined EMST with AS and scored “moderate” for r-PCF because of limitations due to a small sample size and an unclear recruitment of participants.
For IMST, ten different outcomes were included. The MIP was measured in two studies and was scored as “very low” due to high risk of bias, inconsistency because of different outcome values and effect sizes, indirectness caused by differences in the population for disease severity, differences in trainings protocols, the control group had a different intervention, and imprecision due to small sample sizes and unknown confidence intervals. All other IMST outcomes were measured in only one study. The MPT, Peak SGP and peak sound pressure level was scored as “moderate” showing limitations in terms of a serious risk of bias and small sample sizes. Large effect sizes upgraded the level of evidence for these outcomes.
One study combined BS with IS and was ranked as “low” due to the small sample size and serious indirectness and imprecision (the latter because the study only measured the effects directly after the intervention, without a follow-up).
All other outcomes scored “very low” or “low”. The most important reasons for downgrading were: 1) a high risk of bias, 2) indirectness caused by heterogeneity in the population (disease severity), intervention, and treatment duration, and 3) imprecision because of small sample sizes (n = 10 per group). Overseeing all results of the included studies we had to much heterogeneities to do meta-analysis.
DISCUSSION
The overall conclusion of this systematic review is that all respiratory training interventions show positive effects in people with PD, underlining that respiratory training should be considered as a possible treatment option for people with PD. Our two main findings are: (1) EMST significantly improves swallowing safety (PAS score) and phonatory aspects (Peak SGP and peak sound pressure level) and, when EMST is combined with air stacking, improves coughing (r-PCF), with large effect sizes and a moderate level of evidence; and (2) IMST improves phonatory aspects (MPT, peak SGP and peak sound pressure) with large effect sizes and a moderate level of evidence.
This review provides good methodological quality scores for two different modalities of respiratory training: respiratory strength training (EMST more than IMST) and ‘volume- orientated’ training in which volume variations of the chest wall increase directly after breath-stacking combined with incentive spirometer techniques.[15] The methodological quality of the study about breath-stacking plus incentive spirometer techniques was superior followed by good to moderate quality for the five RCTs about IMST and EMST, and a serious risk of bias for the four CTs. Methodological limitations of all studies included an unclear randomization and allocation concealment, reporting of incomplete data, no blinding of outcome measurement, unclear intervention protocol and an unclear intervention for the control group.
The level of evidence measured by the GRADE approach showed different reasons for downgrading for most outcomes due to: 1) a serious risk of bias, 2) indirectness caused by heterogeneity in the population, intervention (for control group) and treatment duration and 3) imprecision because of small sample sizes (n = 10 per group).
Pre-post intervention studies (that had been excluded from the analyses) showed similar positive effects for either strength training (EMST and IMST) and other respiratory training techniques like air stacking, or deep breathing exercises (plus upper extremity exercises) and (global) postural training to improve respiratory function tests, swallowing safety and phonatory aspects.[24, 26– 28, 31– 37] This confirms that the positive results are robust, but also indicates that more high-quality studies for both respiratory strength training and volume-oriented training techniques remain needed. The included studies all showed positive effects, but the magnitude of the effects was different. For example, Sapienza et al. [23] showed a 27% increase of MEP from pre- to post-EMST after four weeks of training. In contrast, Reyes et al. found an increase of MEP of only 8.5% after 8 weeks EMST [25]. There are several possible explanations for the differences in the magnitude of the effects in the included studies. First, differences might result from a lack of homogeneity of the training devices as two different devices were used for IMST [25, 29] and two different devices for EMST [22, 27]. Second, four out of the five included papers who used a respiratory function tests as outcome [24– 26, 29] describe to measure according to the statement of the American Thoracic Society (ATS) and European Respiratory Society (ERS) [38]. This procedure included the performance of respiratory function tests. The ATS/ERS statement described the performance of MIP and MEP as: the maximum value of three inspiratory or expiratory maneuvers that vary by less than 10%. However, this procedure seems to attenuate the learning effect of repeated measurements insufficiently [39]. A ‘warm up session’ prior to measuring is recommended to improve the reliability, and this procedure should be incorporated in future studies having either MIP or MEP as primary outcome [40]. However, the number of repeating measurements differ in the five studies and the starting position and use of a nose clip while testing was different. The study of Sapienza et al. didn’t describe to follow this statement and used another measurement device to capture the MEP [23]. Third, heterogeneity was found for the training protocol as training duration varied from 4 weeks to 12 weeks.[22, 29] For EMST, training frequency of 5 sets, 5 repetitions, 6 days a weeks within an intensity of 75% of MEP showed the greatest effects on MEP, PAS, and voice production.[22, 25, 30] Finally, in four studies, EMST was provided as a combined intervention with either IMST, air stacking and postural training.
Except improving respiratory (muscle) function tests, there are also indications that respiratory training improves coughing (PCF), swallowing safety (PAS score, VFS) and phonatory aspects (MPT, Peak SGP and peak sound pressure level). Having these positive outcomes the important question remains where, in the course of the disease, should respiratory training be considered? In almost all included studies, the disease severity ranged between H&Y I to III. Respiratory dysfunction measured with the MIP is already an early feature in PD. Also, notice that when treatment with levodopa is started in the early phase of PD, the MIP seems to improve, at least initially [1]. So, it can be questioned if respiratory training should be started that early in the disease. The lack of evidence for the effects of respiratory training in H&Y stages IV and V is clinically relevant, because coughing, speech and swallowing difficulties are more prevalent in advanced PD, and more often lead to serious complications such as aspiration pneumonia [5, 41]. In amyotrophic lateral sclerosis (ALS), patient also experience speech, swallowing and coughing problems [42]. We do know from ALS, that respiratory training in terms of lung volume-orientated training strongly enhances cough efficacy [10][43]. In ALS, lung volume-orientated training is indicated if the peak cough flow is less than 270 liters per minute [44]. For that reason, future respiratory intervention studies in PD should include more advanced PD patients and perhaps should consider to start training based on the criteria of ALS.
The impact of respiratory dysfunction on daily life is unknown, which makes it almost impossible to answer the question whether amelioration in MIP and MEP by respiratory training leads to meaningful improvements for the patient in daily life. A better understanding how experienced signs and symptoms relate to abnormal respiratory function tests is therefore needed. In addition, qualitative research enquiring the impact of respiratory training on daily functioning and respiratory symptoms could provide more information about the clinical impact of respiratory dysfunction in PD.
Despite the progressive and typically longstanding course of PD (which can extend to decades for some), the effects of respiratory training have not been investigated beyond a period of 3 months. Two studies included in this review only measured the effect of an intervention without a training period [15, 27]. Next to that, any possible changes resulting from discontinuation of training are not well established, so it remains unclear whether prolonged maintenance therapy (perhaps in the form of boost sessions) is needed to ascertain a long-term efficacy [45]. The suggested pathophysiology underlying respiratory dysfunction in PD is diffuse and still somewhat unclear [46]. Cardinal motor features of PD such as bradykinesia, hypokinesia, rigidity and dystonia can influence muscle control in the limbs, but can also influence muscle strength and control in the respiratory system [13, 14]. Although the motor control of respiratory muscles differs from that of skeletal muscles, the respiratory training effects found in our review do show similarities with, e.g., the effects of gait interventions in PD [47]. These gait interventions in PD have been studied in more detail, yielding strong evidence that strength training of the skeletal leg muscles as well as amplitude-oriented training (by using compensatory strategies) can improve gait functions [48]. The rationale behind these finding is that a good strength in mainly the upper legs is a prerequisite to walk, and that stressing these muscles by training improves gait function [49]. Similar results are found for respiratory strength training in terms of IMST and EMST. However, solely strength training does not improve the decreased chest amplitudes due to bradykinesia, hypokinesia or akinesia [50]. For gait, compensatory cueing strategies which are applied consciously with the aim to improve step length improve gait function as well [47]. Volume-oriented respiratory training interventions like breath-stacking, air stacking or deep breathing exercises (which are performed consciously) seem to improve muscle control just as they do for gait. From this viewpoint, conscious and deep breathing exercises or existing techniques need to be considered and studied into more detail in future studies. These future studies should particularly examine how long the immediate effects found for volume-oriented techniques like incentive spirometry persist.
Another viewpoint is that intensive exercise stimulates a deeper ventilation [48]. The effects of aerobic exercise in PD have been studied extensively for outcomes related to balance, gait, functional mobility or motor function (UPDRS) but none of these studies looked at the impact of exercise on respiratory parameters such as FVC, MIP or MEP so far.
In summary, this review shows positive effects of respiratory training in PD. EMST significantly improves swallowing safety and phonatory aspects and IMST improves phonatory aspects. Volume-orientated respiratory training seems to improve chest amplitude, lung expansion and also the ability to produce an effective cough, the latter being a clinically important mechanism that can help to prevent pneumonia.
This review also reveals important research questions that need to be answered to better understand the implications for clinical practice. Future studies should: 1) standardize training devices, instruments to measure outcomes and protocols in respiratory training; 2) investigate volume oriented techniques and consciously performed breathing exercises; 3) explore the determinants of respiratory dysfunction, but also the impact of respiratory dysfunction on daily life functioning people with PD; and 4) include people with advanced PD as well. This is important to better understand the optimal timing of when to start respiratory training, with the overall aim of preventing respiratory complications such as aspiration pneumonia [6].
CONFLICT OF INTEREST
The authors have no financial conflicts of interest related to this publication. Prof. Bloem currently serves as co-Editor in Chief for the Journal of Parkinson’s disease, serves on the editorial of Practical Neurology and Digital Biomarkers, has received honoraria from serving on the scientific advisory board for Abbvie, Biogen and UCB, has received fees for speaking at conferences from AbbVie, Zambon, Roche, GE Healthcare and Bial, and has received research support from the Netherlands Organization for Scientific Research, the Michael J Fox Foundation, UCB, Abbvie, the Stichting Parkinson Fonds, the Hersenstichting Nederland, the Parkinson’s Foundation, Verily Life Sciences, Horizon 2020 and the Parkinson Vereniging.
ACKNOWLEDGMENTS
This work was supported by funding from the Principal Clinician 2019 of Maarten, J. Nijkrake, Radboudumc Nijmegen. The Center of Expertise for Parkinson & Movement Disorders was supported by a center of excellence grant by the Parkinson Foundation.
SUPPLEMENTARY MATERIAL
[1] The supplementary material is available in the electronic version of this article: https://dx.doi.org/10.3233/JPD-202223.
REFERENCES
[1] | Baille G , Perez T , Devos D , Deken V , Defebvre L , Moreau C ((2018) ) Early occurrence of inspiratory muscle weakness in Parkinson’s disease. PLoS One 13: , e0190400. |
[2] | Gross RD , Atwood CW , Ross SB , Eichhorn KA , Olszewski JW , Doyle PJ ((2008) ) The coordination of breathing and swallowing in Parkinson’s disease. Dysphagia 23: , 136–145. |
[3] | Monteiro L , Souza-Machado A , Pinho P , Sampaio M , Nobrega AC , Melo A ((2014) ) Swallowing impairment and pulmonary dysfunction in Parkinson’s disease: The silent threats. J Neurol Sci 339: , 149–152. |
[4] | Pitts T , Bolser D , Rosenbek J , Troche M , Sapienza C ((2008) ) Voluntary cough production and swallow dysfunction in Parkinson’s disease. Dysphagia 23: , 297–301. |
[5] | Fernandez HH , Lapane KL ((2002) ) Predictors of mortality among nursing home residents with a diagnosis of Parkinson’s disease. Med Sci Monit 8: , Cr241–246. |
[6] | Hegland KW , Okun MS , Troche MS ((2014) ) Sequential voluntary cough and aspiration or aspiration risk in Parkinson’s disease. Lung 192: , 601–608. |
[7] | Pal PK , Sathyaprabha TN , Tuhina P , Thennarasu K ((2007) ) Pattern of subclinical pulmonary dysfunctions in Parkinson’s disease and the effect of levodopa. Mov Disord 22: , 420–424. |
[8] | Reyes A , Ziman M , Nosaka K ((2013) ) Respiratory muscle training for respiratory deficits in neurodegenerative disorders: A systematic review. Chest 143: , 1386–1394. |
[9] | Pollock RD , Rafferty GF , Moxham J , Kalra L ((2013) ) Respiratory muscle strength and training in stroke and neurology: A systematic review. Int J Stroke 8: , 124–130. |
[10] | Macpherson CE , Bassile CC ((2016) ) Pulmonary physical therapy techniques to enhance survival in amyotrophic lateral sclerosis: A systematic review. J Neurol Phys Ther 40: , 165–175. |
[11] | Fromageot C , Lofaso F , Annane D , Falaize L , Lejaille M , Clair B , Gajdos P , Raphael JC ((2001) ) Supine fall in lung volumes in the assessment of diaphragmatic weakness in neuromuscular disorders. Arch Phys Med Rehabil 82: , 123–128. |
[12] | Mendoza M , Gelinas DF , Moore DH , Miller RG ((2007) ) A comparison of maximal inspiratory pressure and forced vital capacity as potential criteria for initiating non-invasive ventilation in amyotrophic lateral sclerosis. Amyotroph Lateral Scler 8: , 106–111. |
[13] | Polatli M , Akyol A , Cildag O , Bayulkem K ((2001) ) Pulmonary function tests in Parkinson’s disease. Eur J Neurol 8: , 341–345. |
[14] | Sathyaprabha TN , Kapavarapu PK , Pall PK , Thennarasu K , Raju TR ((2005) ) Pulmonary functions in Parkinson’s disease. Indian J Chest Dis Allied Sci 47: , 251–257. |
[15] | Ribeiro R , Brandao D , Noronha J , Lima C , Fregonezi G , Resqueti V , Dornelas de Andrade A ((2018) ) Breath-stacking and incentive spirometry in Parkinson’s disease: Randomized crossover clinical trial. Respir Physiol Neurobiol 255: , 11–16. |
[16] | Moher D , Liberati A , Tetzlaff J , Altman DG ((2009) ) Preferred reporting items for systematic reviews and meta-analyses: The PRISMA statement. PLoS Med 6: , e1000097. |
[17] | Higgins JPT , Green S ((2011) ) Cochrane Handbook for Systematic Reviews of Interventions, The Cochrane Collaboration. |
[18] | Sterne JA , Hernan MA , Reeves BC , Savovic J , Berkman ND , Viswanathan M , Henry D , Altman DG , Ansari MT , Boutron I , Carpenter JR , Chan AW , Churchill R , Deeks JJ , Hrobjartsson A , Kirkham J , Juni P , Loke YK , Pigott TD , Ramsay CR , Regidor D , Rothstein HR , Sandhu L , Santaguida PL , Schunemann HJ , Shea B , Shrier I , Tugwell P , Turner L , Valentine JC , Waddington H , Waters E , Wells GA , Whiting PF , Higgins JP ((2016) ) ROBINS-I: A tool for assessing risk of bias in non-randomised studies of interventions. BMJ 355: , i4919. |
[19] | Guyatt GH , Oxman AD , Vist GE , Kunz R , Falck-Ytter Y , Alonso-Coello P , Schunemann HJ ((2008) ) GRADE: An emerging consensus on rating quality of evidence and strength of recommendations. BMJ 336: , 924–926. |
[20] | Schünemann HJ , Mustafa RA , Brozek J , Steingart KR , Leeflang M , Murad MH , Bossuyt P , Glasziou P , Jaeschke R , Lange S , Meerpohl J , Langendam M , Hultcrantz M , Vist GE , Akl EA , Helfand M , Santesso N , Hooft L , Scholten R , Rosen M , Rutjes A , Crowther M , Muti P , Raatz H , Ansari MT , Williams J , Kunz R , Harris J , Rodriguez IA , Kohli M , Guyatt GH ((2020) ) GRADE guidelines: 21 part 1. Study design, risk of bias, and indirectness in rating the certainty across a body of evidence for test accuracy. J Clin Epidemiol 122: , 129–141. |
[21] | Schunemann HJ , Mustafa RA , Brozek J , Steingart KR , Leeflang M , Murad MH , Bossuyt P , Glasziou P , Jaeschke R , Lange S , Meerpohl J , Langendam M , Hultcrantz M , Vist GE , Akl EA , Helfand M , Santesso N , Hooft L , Scholten R , Rosen M , Rutjes A , Crowther M , Muti P , Raatz H , Ansari MT , Williams J , Kunz R , Harris J , Rodriguez IA , Kohli M , Guyatt GH ((2020) ) GRADE guidelines: 21 part 2. Test accuracy: Inconsistency, imprecision, publication bias, and other domains for rating the certainty of evidence and presenting it in evidence profiles and summary of findings tables. J Clin Epidemiol 122: , 142–152. |
[22] | Troche MS , Okun MS , Rosenbek JC , Musson N , Fernandez HH , Rodriguez R , Romrell J , Pitts T , Wheeler-Hegland KM , Sapienza CM ((2010) ) Aspiration and swallowing in Parkinson disease and rehabilitation with EMST: A randomized trial. Neurology 75: , 1912–1919. |
[23] | Sapienza C , Troche M , Pitts T , Davenport P ((2011) ) Respiratory strength training: Concept and intervention outcomes. Semin Speech Lang 32: , 21–30. |
[24] | Kuo YC , Chan J , Wu YP , Bernard JR , Liao YH ((2017) ) Effect of expiratory muscle strength training intervention on the maximum expiratory pressure and quality of life of patients with Parkinson disease. Neurorehabilitation 41: , 219–226. |
[25] | Reyes A , Castillo A , Castillo J , Cornejo I ((2018) ) The effects of respiratory muscle training on peak cough flow in patients with Parkinson’s disease: A randomized controlled study. Clin Rehabil 32: , 1317–1327. |
[26] | Reyes A , Castillo A , Castillo J ((2020) ) Effects of expiratory muscle training and air stacking on peak cough flow in individuals with Parkinson’s disease. Lung 198: , 207–211. |
[27] | Frazao M , Cabral E , Lima I , Resqueti V , Florencio R , Aliverti A , Fregonezi G ((2014) ) Assessment of the acute effects of different PEP levels on respiratory pattern and operational volumes in patients with Parkinson’s disease. Respir Physiol Neurobiol 198: , 42–47. |
[28] | Haewon B ((2016) ) Effect of simultaneous application of postural techniques and expiratory muscle strength training on the enhancement of the swallowing function of patients with dysphagia caused by parkinson’s disease. J Phys Ther Sci 28: , 1840–1843. |
[29] | Inzelberg R , Peleg N , Nisipeanu P , Magadle R , Carasso RL , Weiner P ((2005) ) Inspiratory muscle training and the perception of dyspnea in Parkinson’s disease. Can J Neurol Sci 32: , 213–217. |
[30] | Reyes A , Castillo A , Castillo J , Cornejo I , Cruickshank T ((2019) ) The effects of respiratory muscle training on phonatory measures in individuals with Parkinson’s disease. J Voice, doi: 10.1016/j.jvoice.2019.05.001. |
[31] | Darling-White M , Huber JE ((2017) ) The impact of expiratory muscle strength training on speech breathing in individuals with Parkinson’s disease: A preliminary study. Am J Speech Lang Pathol 26: , 1159–1166. |
[32] | Montero Ferro A , P Basso-Vanelli R , Moreira Mello RL , Sanches Garcia-Araujo A , Gonçalves Mendes R , Costa D , Gianlorenço AC ((2019) ) Effects of inspiratory muscle training on respiratory muscle strength, lung function, functional capacity and cardiac autonomic function in Parkinson’s disease: Randomized controlled clinical trial protocol. Physiother Res Int 24: , e1777. |
[33] | Genç A , Dönmez Çolakoğlu B , Kara B , çakmur R , Makalesi A ((2012) ) Evaluation of the effects of home-based deep breathing exercises in Parkinson’s disease patients. Arch Neuropsychiatry 49: , 59–62. |
[34] | Koseoglu F , Inan L , Ozel S , Deviren SD , Karabiyikoglu G , Yorgancioglu R , Atasoy T , Ozturk A ((1997) ) The effects of a pulmonary rehabilitation program on pulmonary function tests and exercise tolerance in patients with Parkinson’s disease. Funct Neurol 12: , 319–325. |
[35] | Pitts T , Bolser D , Rosenbek J , Troche M , Okun MS , Sapienza C ((2009) ) Impact of expiratory muscle strength training on voluntary cough and swallow function in Parkinson disease. Chest 135: , 1301–1308. |
[36] | Clementino Salgado de Oliveira Rocha L , Natsumi Hosoda Mineshita L , Lobato Sobral L , Dias Magno L , Clementino de Souza Santos M , Santiago Barbosa Rocha R ((2017) ) Influence of global postural reeducation method on respiratory muscle strength and parkinsonian quality of life. Man Ther Posturol Rehabil J 15: , 1–5. |
[37] | Silverman EP , Sapienza CM , Saleem A , Carmichael C , Davenport PW , Hoffman-Ruddy B , Okun MS ((2006) ) Tutorial on maximum inspiratory and expiratory mouth pressures in individuals with idiopathic Parkinson disease (IPD) and the preliminary results of an expiratory muscle strength training program. Neurorehabilitation 21: , 71–79. |
[38] | ((2002) ) ATS/ERS Statement on respiratory muscle testing. Am J Respir Crit Care Med 166: , 518–624. |
[39] | Dimitriadis Z , Kapreli E , Konstantinidou I , Oldham J , Strimpakos N ((2011) ) Test/retest reliability of maximum mouth pressure measurements with the microRPM in healthy volunteers. Respiratory Care 56: , 776–782. |
[40] | Volianitis S , McConnell AK , Jones DA ((2001) ) Assessment of maximum inspiratory pressure. Prior submaximal respiratory muscle activity (’warm-up’) enhances maximum inspiratory activity and attenuates the learning effect of repeated measurement. Respiration 68: , 22–27. |
[41] | Santos RBD , Fraga AS , Coriolano M , Tiburtino BF , Lins OG , Esteves ACF , Asano NMJ ((2019) ) Respiratory muscle strength and lung function in the stages of Parkinson’s disease. J Bras Pneumol 45: , e20180148. |
[42] | Plowman EK , Watts SA , Robison R , Tabor L , Dion C , Gaziano J , Vu T , Gooch C ((2016) ) Voluntary cough airflow differentiates safe versus unsafe swallowing in amyotrophic lateral sclerosis. Dysphagia 31: , 383–390. |
[43] | Senent C , Golmard JL , Salachas F , Chiner E , Morelot-Panzini C , Meninger V , Lamouroux C , Similowski T , Gonzalez-Bermejo J ((2011) ) A comparison of assisted cough techniques in stable patients with severe respiratory insufficiency due to amyotrophic lateral sclerosis. Amyotroph Lateral Scler 12: , 26–32. |
[44] | Motor Neurone Disease: Assessment and Management. National Institue for Health and Care Excellence: Clinical Guidelines 2016. |
[45] | Troche MS , Rosenbek JC , Okun MS , Sapienza CM ((2014) ) Detraining outcomes with expiratory muscle strength training in Parkinson disease. J Rehabil Res Dev 51: , 305–310. |
[46] | Torsney KM , Forsyth D ((2017) ) Respiratory dysfunction in Parkinson’s disease. J R Coll Physicians Edinb 47: , 35–39. |
[47] | Nonnekes J , Ruzicka E , Nieuwboer A , Hallett M , Fasano A , Bloem BR ((2019) ) Compensation strategies for gait impairments in Parkinson disease: A review. JAMA Neurol 76: , 718–725. |
[48] | Uhrbrand A , Stenager E , Pedersen MS , Dalgas U ((2015) ) Parkinson’s disease and intensive exercise therapy – a systematic review and meta-analysis of randomized controlled trials. J Neurol Sci 353: , 9–19. |
[49] | Nonnekes J , Snijders AH , Nutt JG , Deuschl G , Giladi N , Bloem BR ((2015) ) Freezing of gait: A practical approach to management. Lancet Neurol 14: , 768–778. |
[50] | Tamaki A , Matsuo Y , Yanagihara T , Abe K ((2000) ) Influence of thoracoabdominal movement on pulmonary function in patients with Parkinson’s disease: Comparison with healthy subjects. Neurorehabil Neural Repair 14: , 43–47. |