Systematic Review of the Relationship between Vitamin D and Parkinson’s Disease
Abstract
Background: Although vitamin D may have both protective and symptomatic effects in Parkinson’s disease (PD), the evidence is scarce and not well understood. Also, 25-hydroxyvitamin D (vitamin D) is suggested to play a neuroprotective and neurotrophic role in the brain. Therefore, this review investigates the relationship between vitamin D and PD.
Objective: Investigate the evidence for a relationship between vitamin D and PD by summarizing observational and interventional studies in humans, as well as relevant experimental studies.
Methods: A systematic search was made in the Medline, Cochrane and Embase databases (from inception to March 2014). All identified titles were independently evaluated by two reviewers. Articles were selected based on the presence of PD-related outcome data. Included were observational studies (including genetic studies) and interventional studies in humans, as well as relevant animal studies.
Results: A total of 20 studies (14 observational, 1 interventional and 5 rodent studies) were selected for analysis. Eight observational studies showed that serum 25(OH) D levels tend to be low in PD. One observational study indicated that low serum 25(OH) D may worsen automatic postural responses and one interventional study suggested that vitamin D supplementation can prevent worsening (based on the Hoehn and Yahr rating scale). Studies in rodent models of PD showed a protective effect of vitamin D treatment on dopaminergic neurons in the substantia nigra. Results of genetic studies on the association between vitamin D receptor polymorphisms and the risk of PD were contradictory.
Conclusion: The literature supports possible protective and symptomatic effects of vitamin D in PD. However, more observational and interventional studies in humans are needed to confirm and further elucidate the suggested beneficial effect of vitamin D on PD.
BACKGROUND
There is accumulating evidence that 25-hydroxyvitamin D (25-(OH)D) is not only involved in bone health, but may also play a role in non-skeletal systems via the vitamin D receptor (VDR) [1, 2]. Vitamin D receptors, and the enzyme that converts 25-hydroxyvitamin D into active vitamin D, are found throughout the brain [1, 2]. It is also suggested that vitamin D plays a role in brain development and in neurodegenerative diseases as Parkinson’s disease (PD) and dementia [1, 2]; however, this relationship is not well understood.
Vitamin D can be ingested or synthesized in the human skin as the pre-vitamin D, (25-(OH)D). Thereafter, 25-(OH)D has to be hydroxylated twice to become the active form 1,25-dihydroxyvitamin D (1,25-(OH)2D); these hydroxylations occur first in the liver and then in the kidney. 1,25-(OH)2D binds to the VDR, thereby activating this receptor and regulating gene transcription [3].
The VDR and the enzyme 1a-hydroxylase are also expressed in other tissues, including neuronal and glial cells [1]. There is evidence that vitamin D has a neuroprotective effect on brain function. Firstly, 1,25-(OH)2D inhibits the synthesis of inducible nitric oxide synthase (iNOS), which catalyzes nitric oxide, a free radical that can damage cells [4]. Secondly, 1,25-(OH)2D stimulates γ-glutamyl transpeptidase activity. The enzyme γ-glutamyl transpeptidase is important in the synthesis of glutathione. Glutathione is an antioxidant that neutralizes free radicals and in this way protects cells from damage [4]. In addition, vitamin D may act as a neurotrophic factor. Neveu et al. showed that vitamin D stimulates brain cells to produce several growth factors, like nerve growth factor (NGF), glial cell line-derived neurotrophic factor (GDNF) and neurotrophin 3 (NT3) [5–7]. These neutrophic and neuroprotective actions of vitamin D suggest that this vitamin can stimulate the protection and growth of neuronal cells. Therefore, vitamin D may slow down the progression of neurodegenerative diseases, such as PD.
OBJECTIVE
The aim of this study was to investigate the evidence for a relationship between vitamin D and PD by summarizing observational and interventional studies in humans, as well as relevant experimental studies.
METHODS
A literature search was performed in Medline, Embase and Cochrane Library databases from inception until March 2014. The following (MESH) terms were used: ‘Vitamin D’ OR cholecalciferol OR ‘ergocalciferol’ AND ‘Neurodegenerative Diseases’ OR ‘Parkinson’ OR ‘Huntington’ OR ‘dementia’ OR ‘ALS’ OR ‘lewy body’ OR ‘multiple system atrophy’.
Excluded were articles not in the English language and studies not investigating PD or vitamin D.
All identified titles (n = 5184) were independently evaluated by two reviewers. First, a search within the neurodegenerative diseases revealed that, contrary to our expectations, more studies were found that addressed vitamin D than PD. We selected only those studies that were related to both PD and vitamin D (i.e. correlation studies, case-control and interventional studies in humans, and experimental studies) that examined the relationship between vitamin D and PD. This yielded 107 studies on PD, of which 75 were excluded based on the abstract alone, because vitamin D or PD was not investigated. After scrutinizing the remaining 32 articles 12 articles were excluded because: i) vitamin D levels, vitamin D supplementation or vitamin D intake were not reported, ii) PD was not established, and iii) the article was not in the English language. Finally, 20 studies were included in the present review. Figure 1 shows the inclusion process of the studies.
RevMan 5 was used to perform a meta-analysis and create forest plots.
RESULTS
Serum 25(OH)D concentration in PD vs. healthy controls: Observational studies
Table 1 lists the eight studies in which vitamin D status was compared between patients with PD and a healthy control group [8–15]. With the exception of one study [14], all showed that serum 25(OH)D in patients is significantly lower than in healthy controls. Our meta-analysis (Table 2) confirmed this relationship with a significant mean difference of 11.6 ug/ml (SD 12.2–10.9) in the PD group as compared with the healthy controls. Of these eight studies, only six were included in the meta-analysis [9–11, 13–15] because two studies did not report the standard deviation of the level of vitamin D [8, 12]. However, these two latter studies were in line with the results of the meta-analysis and showed a significantly higher vitamin D level in the control group compared with patients with PD [8, 12]. Sato et al. made a separate analysis of vitamin D status in patients with Hoehn & Yahr (H&Y) stage 1–2 and with stage 3–5 [9]; serum 25(OH)D was lower in the group with H&Y stage 3–5 compared with patients with stage 1–2 [9]. Our meta-analysis included the group with H&Y stage 3–5; however, when including only results from the group with H&Y stage 1–2, mean serum 25(OH)D level in patients with PD was still lower (10.72 ug/m; –11.49–9.95) than in healthy controls.
Serum 25(OH)D concentration and relation with balance in PD: Observational study
Peterson et al. investigated the relationship between serum 25(OH) D level and balance in a cross-sectional, observational pilot study including 40 patients with PD. Balance was examined using five tests: the motor control test, sensory organization test, sit and stand test, walk and turn test, and the unilateral stance eyes open and closed test. The authors found a significant positive correlation between serum 25(OH)D and automatic postural responses [16].
Vitamin D intake/exposure and PD: Observational studies
Miyake et al. studied the ingestion of vitamin D and the risk of PD; no significantly lower vitamin D intake was found in patients versus controls[17].
Relationship between VDR polymorphisms and PD: Observational studies
Five observational studies investigated VDR polymorphisms in PD [16, 18–21]. Four compared VDR polymorphism in PD with healthy controls (Table 3) [16, 18–20]. Several genetic variants of the VDR were examined (Table 3). In two studies a significant association was found between the rs10735810 (FokI) C allele and PD, with a higher frequency of the C allele in PD compared to controls [19, 20]. In contrast, Suzuki et al. found that the VDR variant FokI CC was associated with milder forms of PD in a Japanese population [21].
Vitamin D supplementation in PD: Interventional studies
Suzuki et al. evaluated the effect of vitamin D supplementation on disease progression in PD [22]. In a double-blind, placebo-controlled trial among 104 patients with PD during 12 months, 56 received 1200 IU vitamin D per day and 58 received placebo. Primary outcome was the percentage worsening on the modified H&Y scale and the Unified Parkinson Disease Rating Scale (UPDRS). The authors found that worsening of the H&Y and the UPDRS part II was significantly less in the vitamin D supplementation group [H&Y: RR 2.37 (95% CI: 1.06–5.31) p = 0.028], [UPDRS part II: RR 1.68 (95% CI 1.68 (1.02–2.78) p = 0.036)]. Interaction analyses showed that the VDR genotype rs10735810 (FokI) modified the effect of vitamin D. Patients with the FokI TT allele had a significant and consistent response to vitamin D supplementation whereas individuals with VDR FokI CT had a moderate response. However, patients with FokI CC showed no significant effect of vitamin D supplementation compared to placebo. None of the other VDR variants [rs1544410 (BsmI), rs731236 (TaqI) rs7975232 (ApaI), rs11568820 (Cdx2)] influenced the effect of vitamin D.
Vitamin D supplementation in rodents
Five studies in animal models of PD investigated the effect of vitamin D therapy on substantia nigra dopamine neurons after 6-OHDA or MPTP lesion (Table 4) [23–27]. One study showed a significantly higher dopamine level and two a significantly higher concentration of the enzyme promoting conversion to dopamine, tyrosine hydroxylase (TH), in the substantia nigra of the vitamin D supplemented rodent group [25–27]. One of these studies also demonstrated that oxidative injury of the substantia nigra was significantly lower after vitamin D supplementation [25]; this result was in line with another study reporting higher neuronal survival in the substantia nigra when 1,25(OH)2D was injected [23]. However, very high concentrations of 1,25(OH)2D enhanced neurotoxity, as also found in Klotho-insufficient mice that resemble human aging and exhibit abnormal levels of serum 1,25-(OH)2D caused by abnormally high vitamin D metabolism in the kidney [23, 28]. On the other hand, Kaluef et al. showed significantly impaired motor function in mice that totally lacked the vitamin D receptor [29].
DISCUSSION
To our knowledge, this is the first systematic review to focus on the relationship between vitamin D and PD: 20 studies (14 observational, 1 interventional and 5 rodent studies) were examined. In almost all human studies, patients with PD had lower serum 25(OH)D levels than controls [8–13] and higher 25(OH)D levels were associated with better motor function [8–13, 16]. Furthermore, higher 25(OH)D levels were associated with a lower risk of PD in humans [8–13, 17] and with neuroprotection in rodent models of PD [23–27].
Our meta-analysis revealed that the average serum 25(OH)D level in patients with PD was 11.6 ug/ml (SD 12.2-10.9) lower than in healthy controls. The absence of a correlation between vitamin D status and PD in one study [14] may have been because the 25(OH)D levels of the control group were measured in winter whereas the patients were measured in summer. The high prevalence of vitamin D deficiency in PD may be a consequence of reduced outdoor activity and, therefore, less sun exposure due to limited mobility. The case-control study of Peterson et al. showed that higher serum 25(OH) D was related to better automatic postural responses in PD [16], whereas in a clinical trial vitamin D supplementation with 1200 IU vitamin D prevented worsening on the H&Y and UPDRS scales [22]. In addition to a symptomatic effect on motor function, vitamin D may have neurotrophic or neuroprotective effects in PD. All rodent studies showed a beneficial effect of vitamin D on the degree of toxin-induced loss of dopamine neurons, with the exception of excessively high vitamin D levels or an abnormally high activation of vitamin D in Klotho mice that enhanced neurotoxic effects [23, 28]. These latter findings are in line with results from human studies in which extremely high doses of vitamin D were shown to enhance fractures [30]. An important consideration is that the toxin-induced dopamine neuron loss in rodent models of PD is different from the much slower injury to the substantia nigra in PD.
Genetic studies indicated a possible relation between PD and the VDR polymorphism FokI, The FokI ‘CC’ allele has been associated with milder forms of PD, possibly due to a higher and more efficient functionality of this VDR allele variant. This is in line with results of other studies investigating the FokI polymorphisms [31, 32]. Indeed, patients with a FokI ‘T’ allele and (presumably) less functionality, were more responsive to vitamin D supplementation [22]. However, the results remain contradictory because two studies showed a small but significantly higher frequency of the VDR variant ‘FokI C’ allele in PD and suggest the opposite, i.e. a possible higher risk for PD in the presence of a FokI C allele [19, 20]. More studies are needed to confirm and elucidate the relationship between FokI VDR polymorphisms and PD.
A strength of the present study is that it provides a broad view on what is known about the relationship between vitamin D and PD. Limitations include the possibility of publication bias, differences between the patient populations, and variations in the applied methodology (e.g. variations in study population, vitamin D supplementation, and outcome measures).
In conclusion, this systematic review indicates that PD is associated with lower serum vitamin D levels [8–13]. Secondly, higher vitamin D levels are associated with better balance, and vitamin D supplementation appears to have a positive effect on PD motor symptoms [16, 22]. Finally, results from rodent models suggest that vitamin D may also have a neuroprotective effect [23–27]. Additional studies are needed to further explore and elucidate the symptomatic and potential neuroprotective effects of vitamin D in Parkinson’s disease.
CONFLICTS OF INTEREST
The authors declare no conflicts of interest.
REFERENCES
[1] | Prufer K , Veenstra TD , Jirikowski GF , & Kumar R ((1999) ) Distribution of 1,25-dihydroxyvitamin D3 receptor immunoreactivity in the rat brain and spinal cord. J Chem Neuroanat, 16: , 135–145. |
[2] | Eyles DW , Smith S , Kinobe R , Hewison M , & McGrath JJ ((2005) ) Distribution of the vitamin D receptor and 1 alpha-hydroxylase in human brain. J Chem Neuroanat, 29: , 21–30. |
[3] | Lowe KE , Maiyar AC , & Norman AW ((1992) ) Vitamin D-mediated gene expression. Crit Rev Eukaryot Gene Expr, 2: , 65–109. |
[4] | Garcion E , Wion-Barbot N , Montero-Menei CN , Berger F , & Wion D ((2002) ) New clues about vitamin D functions in the nervous system. Trends Endocrinol Metab, 13: , 100–105. |
[5] | Naveilhan P , Neveu I , Wion D , & Brachet P ((1996) ) 1,25-Dihydroxyvitamin D3, an inducer of glial cell line-derived neurotrophic factor. Neuroreport, 7: , 2171–2175. |
[6] | Musiol IM , & Feldman D ((1997) ) 1,25-dihydroxyvitamin D3 induction of nerve growth factor in L929 mouse fibroblasts: Effect of vitamin D receptor regulation and potency of vitamin D3 analogs. Endocrinology, 138: , 12–18. |
[7] | Neveu I , Naveilhan P , Baudet C , Brachet P , & Metsis M ((1994) ) 1,25-dihydroxyvitamin D3 regulates NT-3, NT-4 but not BDNF mRNA in astrocytes. Neuroreport, 6: , 124–126. |
[8] | Topal K , Paker N , Bugdayci D , Ozer F , & Tekdos D ((2010) ) Bone mineral density and vitamin D status with idiopathic Parkinson’sDisease. Osteoporosis Int, 21: , S141–S142. |
[9] | Sato Y , Kikuyama M , & Oizumi K ((1997) ) High prevalence of vitamin D deficiency and reduced bone mass in Parkinson’s disease. Neurology, 49: , 1273–1278. |
[10] | Abou-Raya S , Helmii M , & Abou-Raya A ((2009) ) Bone and mineral metabolism in older adults with Parkinson’s disease. Age Ageing, 38: , 675–680. |
[11] | Evatt ML , DeLong MR , Kumari M , Auinger P , McDermott MP , & Tangpricha V ((2011) ) High prevalence of hypovitaminosis D status in patients with early Parkinson disease. Arch Neurol, 68: , 314–319. |
[12] | Senel K , Alp F , Baykal T , Melikoglu M , Erdal A , Ugur M. ((2011) ) Preliminary study: Is there a role of vitamin D in Parkinson disease? Osteoporosis Int, 22: , S167. |
[13] | Van den Bos F , Speelman AD , van NM , van der Schouw YT , Backx FJ , Bloem BR , Munneke M , & Verhaar HJ ((2013) ) Bone mineral density and vitamin D status in Parkinson’s disease patients. J Neurol, 260: , 754–760. |
[14] | Petersen MS , Bech S , Christiansen DH , Schmedes AV , & Halling J ((2014) ) The role of vitamin D levels and vitamin D receptor polymorphism on Parkinson’s disease in the Faroe Islands. Neurosci Lett, 561: , 74–79. |
[15] | Sato Y , Honda Y , Iwamoto J , Kanoko T , & Satoh K ((2005) ) Abnormal bone and calcium metabolism in immobilized Parkinson’s disease patients. Mov Disord, 20: , 1598–1603. |
[16] | Peterson AL , Mancini M , & Horak FB ((2013) ) The relationship between balance control and vitamin D in Parkinson’s disease-a pilot study. Mov Disord, 28: , 1133–1137. |
[17] | Miyake Y , Tanaka K , Fukushima W , Sasaki S , Kiyohara C , Tsuboi Y , Yamada T , Oeda T , Miki T , Kawamura N , Sakae N , Fukuyama H , Hirota Y , & Nagai M ((2011) ) Lack of association of dairy food, calcium, and vitamin D intake with the risk of Parkinson’s disease: A case-control study in Japan. Parkinsonism Relat Disord, 17: , 112–116. |
[18] | Lin CH , Chen KH , Chen ML , Lin HI , & Wu RM ((2014) ) Vitamin D receptor genetic variants and Parkinson’s disease in a Taiwanese population. Neurobiol Aging, 35: , 1212–1213. |
[19] | Han X , Xue L , Li Y , Chen B , & Xie A ((2012) ) Vitamin D receptor gene polymorphism and its association with Parkinson’s disease in Chinese Han population. Neurosci Lett, 525: , 29–33. |
[20] | Torok R , Torok N , Szalardy L , Plangar I , Szolnoki Z , Somogyvari F , Vecsei L , & Klivenyi P ((2013) ) Association of vitamin D receptor gene polymorphisms and Parkinson’s disease in Hungarians. Neurosci Lett, 551: , 70–74. |
[21] | Suzuki M , Yoshioka M , Hashimoto M , Murakami M , Kawasaki K , Noya M , Takahashi D , & Urashima M ((2012) ) 25-hydroxyvitamin D, vitamin D receptor gene polymorphisms, and severity of Parkinson’s disease. Mov Disord, 27: , 264–271. |
[22] | Suzuki M , Yoshioka M , Hashimoto M , Murakami M , Noya M , Takahashi D , & Urashima M ((2013) ) Randomized, double-blind, placebo-controlled trial of vitamin D supplementation in Parkinson disease. Am J Clin Nutr, 97: , 1004–1013. |
[23] | Shinpo K , Kikuchi S , Sasaki H , Moriwaka F , & Tashiro K ((2000) ) Effect of 1,25-dihydroxyvitamin D(3) on cultured mesencephalic dopaminergic neurons to the combined toxicity caused by L-buthionine sulfoximine and 1-methyl-4-phenylpyridine. J Neurosci Res, 62: , 374–382. |
[24] | Sanchez B , Relova JL , Gallego R , Ben-Batalla I , & Perez-Fernandez R ((2009) ) 1,25-Dihydroxyvitamin D3 administration to 6-hydroxydopamine-lesioned rats increases glial cell line-derived neurotrophic factor and partially restores tyrosine hydroxylase expression in substantia nigra and striatum. J Neurosci Res, 87: , 723–732. |
[25] | Lin AM , Fan SF , Yang DM , Hsu LL , & Yang CH ((2003) ) Zinc-induced apoptosis in substantia nigra of rat brain: Neuroprotection by vitamin D3. Free Radic Biol Med, 34: , 1416–1425. |
[26] | Dean ED , Mexas LM , Capiro NL , McKeon JE , DeLong MR , Pennell KD , Doorn JA , Tangpricha V , Miller GW , & Evatt ML ((2012) ) 25-Hydroxyvitamin D depletion does not exacerbate MPTP-induced dopamine neuron damage in mice. PLoS One, 7: , e39227. |
[27] | Wang JY , Wu JN , Cherng TL , Hoffer BJ , Chen HH , Borlongan CV , Wang Y ((2001) ) Vitamin D(3) attenuates 6-hydroxydopamine-induced neurotoxicity in rats. Brain Res, 904: , 67–75. |
[28] | Kosakai A , Ito D , Nihei Y , Yamashita S , Okada Y , Takahashi K , & Suzuki N ((2011) ) Degeneration of mesencephalic dopaminergic neurons in Klotho mouse related to vitamin D exposure. Brain Res, 1382: , 109–117. |
[29] | Kalueff AV , Lou YR , Laaksi I , & T uohimaa P ((2004) ) Impaired motor performance in mice lacking neurosteroid vitamin D receptors. Brain Res Bull, 64: , 25–29. |
[30] | Sanders KM , Nicholson GC , & Ebeling PR ((2013) ) Is high dose vitamin D harmful? Calcif Tissue Int, 92: , 191–206. |
[31] | Orton SM , Morris AP , Herrera BM , Ramagopalan SV , Lincoln MR , Chao MJ , Vieth R , Sadovnick AD , & Ebers GC ((2008) ) Evidence for genetic regulation of vitamin D status in twins with multiple sclerosis. Am J Clin Nutr, 88: , 441–447. |
[32] | Gezen-Ak D , Dursun E , Bilgic B , Hanagasi H , Ertan T , Gurvit H , Emre M , Eker E , Ulutin T , Uysal O , & Yilmazer S ((2012) ) Vitamin D receptor gene haplotype is associated with late-onset Alzheimer’s disease. Tohoku J Exp Med, 228: , 189–196. |
Figures and Tables
Fig.1
Flow diagram of the included studies.
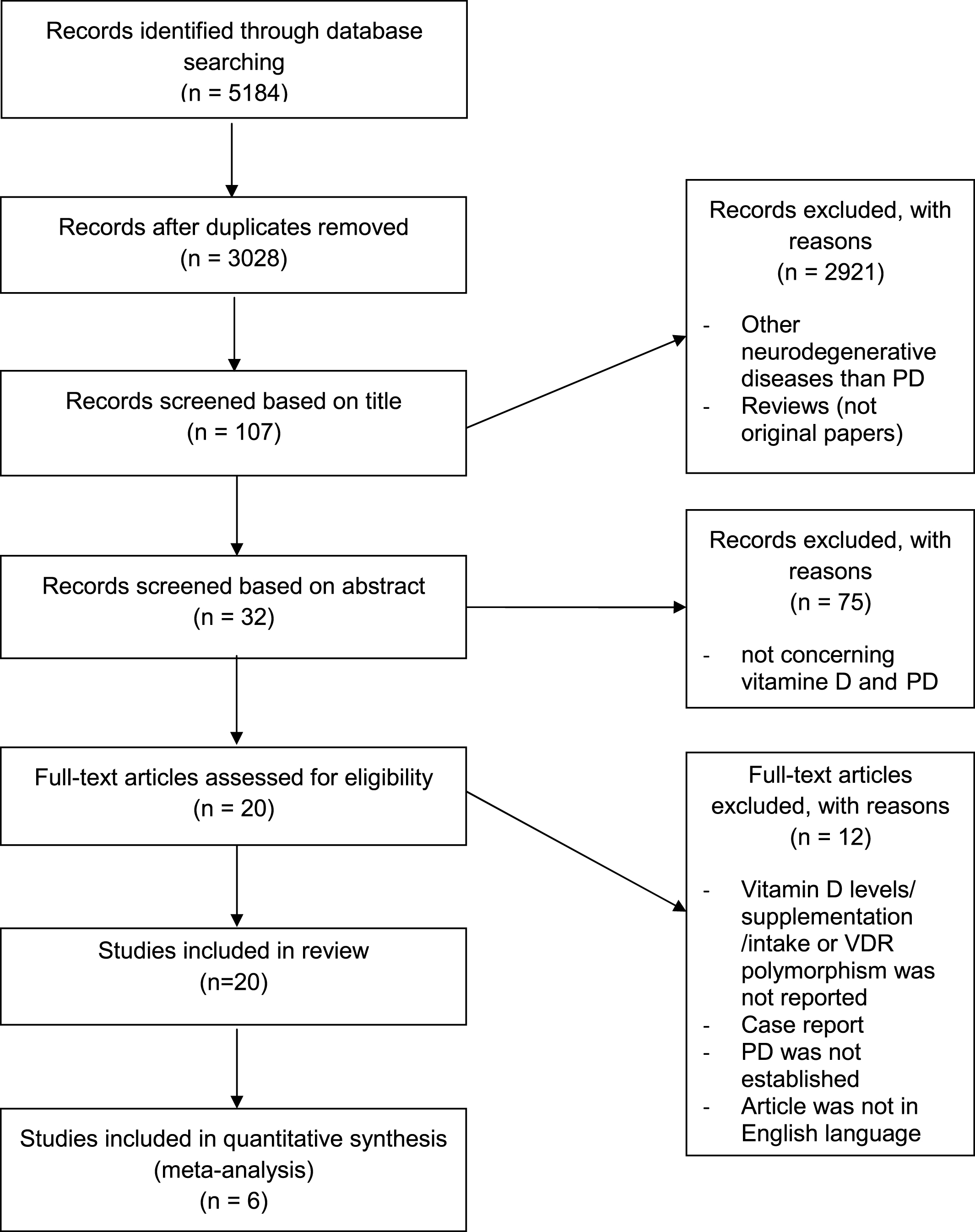
Table 1
Levels of serum 25(OH)D in patients with Parkinson’s disease and in healthy controls
Author | Patients | H&Y | Serum level 25(OH) D | p-value PD vs controls |
Sato et al. 1997 [9] | 20 PD H&Y 1-2 | 1-2,3–5 | PD H&Y 1-2 : 21.7 (8.5) ng/ml | H&Y 3–5 P < 0.001 |
51 PD H&Y 3–5 | PD H&Y 3–5 : 8.9(3.2) ng/ml | H&Y 1-2 P > 0.05 | ||
33 controls | controls: 21.6 (3.1) ng/ml | |||
Sato et al. 2005 [15] | 142 PD | 3.3 (1.1) | PD: 11.0 (3.2) ng/ml | P < 0.001 |
99 controls | controls: 33.3 (3.1) ng/ml | |||
Abou- Raya et al. 2009 [10] | 82 PD | 3.0 (0.5) | PD 12.9 (9.9) ng/ml | p = 0.001 |
68 controls | controls: 21.6 (4.8) ng/ml | |||
Topal et al. 2010 [8] | 48 PD patients and 50 controls | Not reported | PD 27.5 ng/ml | P < 0.01 |
controls: 33.8 ng/ ml | ||||
Evatt et al. 2011 [11] | 97 PD, | 1.7 (0.5) [1-2.5] | PD: 31.9 (13.6) ng/mL, | P = 0.03 |
(97 AD patients) | controls: 37.0 (14.5) ng/ml | |||
99 controls | ||||
Senel et al. 2011 [12] | 19 PD patients | Not reported | PD:12.63 ng/ml | P < 0.01 |
20 controls | controls: 20.42 ng/ml | |||
Van den Bos et al. 2013 [13] | 186 PD | <2.5 | pd: 19.3 (8.1) ng/ml | P < 0.000 |
802 controls | controls: 22.7 (9.2) ng/ml | |||
Petersen et al. 2014 [14] | 80 PD | 2.6 (1.3) | PD 15.7 (9.6) ng/ml | P = 0.49 |
154 controls | controls: 16.4 (9.9) ng/ml |
PD = Parkinson’s disease; H&Y = Hoehn and Yahr rating scale.
Table 2
Meta-analysis of serum 25(OH)D levels in patients with Parkinson’s disease and in healthy controls
PD | Control | Mean Difference | Mean Difference | |||||||
Study or Subgroup | Mean | SD | Total | Mean | SD | Total | Weight | IV,Fixed,95% CI | Year | IV,Fixed,95% CI |
Sato 1997 H&Y 3–5 [9] | 8.9 | 3.2 | 51 | 21.6 | 3.1 | 33 | 24.8% | –12.70 [–14.07, –11.33] | 1997 | ![]() |
Sato 2005 [15] | 11.9 | 6.5 | 142 | 33.3 | 3.1 | 99 | 30.9% | –21.40 [–22.63, –20.17] | 2005 | |
Abou-Raya 2009 [10] | 12.9 | 9.9 | 82 | 21.6 | 4.8 | 68 | 7.9% | –8.70 [–11.13, –6.27] | 2009 | |
Evatt 2011 [11] | 31.9 | 13.6 | 97 | 37 | 14.5 | 99 | 3.0% | –5.10 [–9.03, –1.17] | 2011 | |
Van des Bos 2013 [13] | 19.3 | 8.1 | 186 | 22.7 | 9.2 | 802 | 26.6% | –3.40 [–4.73, –2.07] | 2012 | |
Peterson 2014 [14] | 15.7 | 9.6 | 80 | 16.4 | 9.9 | 154 | 6.8% | –0.70 [–3.32, 1.92] | 2014 | |
Total(95% CI) | 638 | 1255 | 100.0% | –11.55 [–12.23, –10.86] | ||||||
Hetergeneity: Chi2 = 474.94, df = 5(P < 0.00001); I2 = 99% | ||||||||||
Test for overall effect: Z = 33.09(P < 0.00001) |
H&Y = Hoehn and Yahr.
Table 3
Vitamin D receptor polymorphisms in patients with Parkinson’s disease (PD) and a healthy control group
Authors | Ethnicity | Study population | Mean age (years) | H&Y | UPDRS | Genetic variants VDR | Odds ratio (95% CI) | P-value |
Han et al. 2012 [21] | Chinese Han | PD 260 | 70.9 ±6.1 | 2(1–4) | UPDRS part III: 15 (3–36) | rs10735810 (FokI) | 1.340 (1.044–1.720) for common C allel | 0.023* |
controls 282 | 69.4 ±9.7 | rs1544410 (BsmI) | 1.151 (0.734–1.806) | 0.567 | ||||
Török et al. 2013 [20] | Hungarian | PD 100 | 66.4±9.3 | N.A | N.A | rs10735810 (FokI) | 1.615 (1.087–2.399) for common C allel | 0.017* |
controls 109 | 64.0±8.2 | rs1544410 (BsmI) | 0.997 (0.665–1.434) | 0.905 | ||||
rs731236 (TaqI) | 0.802 (0.540–1.190) | 0.273 | ||||||
rs7975232 (ApaI) | 1.177 (0.793–1.748) | 0.417 | ||||||
Lin et al. 2014 [18] | ||||||||
Taiwanese | PD 700 | 68.7±11.2 | N.A | N.A | rs2853559 | 0.85 (0.68–1.06) | 0.15 | |
controls 792 | N.A | rs4334098 | 1.06 (0.86–1.31) | 0.57 | ||||
rs7299460 | 1.12 (0.89–1.42) | 0.33 | ||||||
rs7968585 | 1.02 (0.83–1.25) | 0.86 | ||||||
rs7976091 | 0.92 (0.73–1.16) | 0.49 | ||||||
rs10083198 | 1.07 (0.86–1.33) | 0.57 | ||||||
Peterson et al. 2014 [14] | Farao Islands | PD 121 | 74.5±9.9 | 2.6 (1.3) | N.A | rs7975232 (ApaI) | 1.14 (0.83–1.56) | 0.41 |
Conrols 235 | 75.0±9.9 | rs1544410 (BsmI) | 1.04 (0.76–1.43) | 0.81 | ||||
rs731236 (TaqI) | 1.05 (0.76–1.44) | 0.77 |
*P < 0.05. H&Y = Hoehn and Yahr rating scale; UPDRS = unified Parkinson’s disease rating scale. N.A. = not applicable; VDR = vitamin D receptor.
Table 4
Data on vitamin D supplementation in rodents
Authors | Rodent group | vitamin D supplementation | SNc intoxication | Primary measure | Results |
Shinpo et al. 2000 [23] | Not available | group 1 : 0 | BSO/ MPP+ | neuronal survival | group 1 : 42(2) % |
group 2 : 1 nmol 1.25 (OH)2D3 | group 2 : 62 (3) % * | ||||
group 3 : 10 nmol1.25 (OH)2D3 | group 3 : 63 (10) % * | ||||
group 4 : 100 nmol 1,25-(OH)2D3 | group 4 : 65 (7) % * | ||||
group 5 : 250 nmol 1.25 (OH)2D3 | group 5 : 43 (2) % | ||||
group 6 : 500 nmol 1.25 (OH)2D3 | group 6 : 20 (1) % * | ||||
Wang et al. 2001 [27] | 16 vitamin D supplemented | 8 days 1.25 (OH)2D3 1 ug/ml/kg intraperitoneal | 6-OHD | % loss of dopamine | vitamin D supplemented rats: 65 (10) % |
16 controls | – | control: 87 (18) % ** | |||
Neuronal density | vitamin D supplemented rats: 14.2 (0.8) cells/mm2 | ||||
control: 10 (0.8) cells/mm2 ** | |||||
Lin et al. 2003 [25] | 6 vitamin D supplemented | 13 days 1.25 (OH)2D3 1 ug/ml/kg intraperitoneal | 20 nmol zinc | Dopamine level | vitamin D supplemented rats: 50 pmol/mg |
6 controls | – | control: 40 pmol/mg ** | |||
Sanchez et al. 2009 [24] | 1.25 (OH)2D3 1 ug/ml/kg intraperitoneal | GNDF | |||
TH | |||||
40 group 1 | group 1: - | group 1: - | group 1: GNDF 0.78 (0.01) TH 0.60 (0.15) | ||
40 group 2 | group 2: - | group 2 : 6-OHDA | group 2: GNDF 0.60 (0.12) TH 0.46 (0.10) | ||
40 group 3 | group 3 : 7 days 1.25 (OH)2D3 | group 3 : 6-OHDA | group 3: GNDF 0.77 (0.15) TH 0.53 (0.10)** | ||
40 group 4 | group 4 : 21 days 1.25 (OH)2D3 (after intoxication) | group 4 : 6-OHDA | group 4: GNDF 0.88 (0.08) TH 0.60 (0.10) | ||
Dean et al. 2012 [26] | 4 vitamin D depleted | MPTP | Dopamine level | Vitamin D depleted rats: | |
4 controls | before intoxication: 32.735 (1.4) pmol/mg | ||||
after intoxication: 13.396 (5.0) pmol/mg)** | |||||
control: | |||||
before intoxication: 25.546 (3.6) pmol/mg | |||||
after intoxication: 10.523 (5.0)pmol/mg) |
*P < 0.01; ** P < 0.05 SN = substabtia nigra GNDF = glial cell line-derived neurotrophic factor TH = tyrosine hydroxylase MPTP: 1-methyl-4-phenyl-1,2,3,6-tetrahydropyridine BSO: DL-buthionine-S,R-sulfoximine MPP+: 1-methyl-4-phenylpyridinium 6-OHD: 6-hydroxydopamine.