The backscattering story: A personal view
1.Munich 1965–1970
On May 5th 1966 I passed my examination in Theoretical Physics to obtain the Diploma. The next day I started a 6 month trip to Nepal with 2 friends and an old VW bus. We came back from this marvellous adventure with the desire to work. My two friends joined industry as engineers. I looked for a thesis together with my friend Manfred Birr.
Fig. 1.
Manfred Birr celebrating his thesis on QNS in Glycerol (1969).
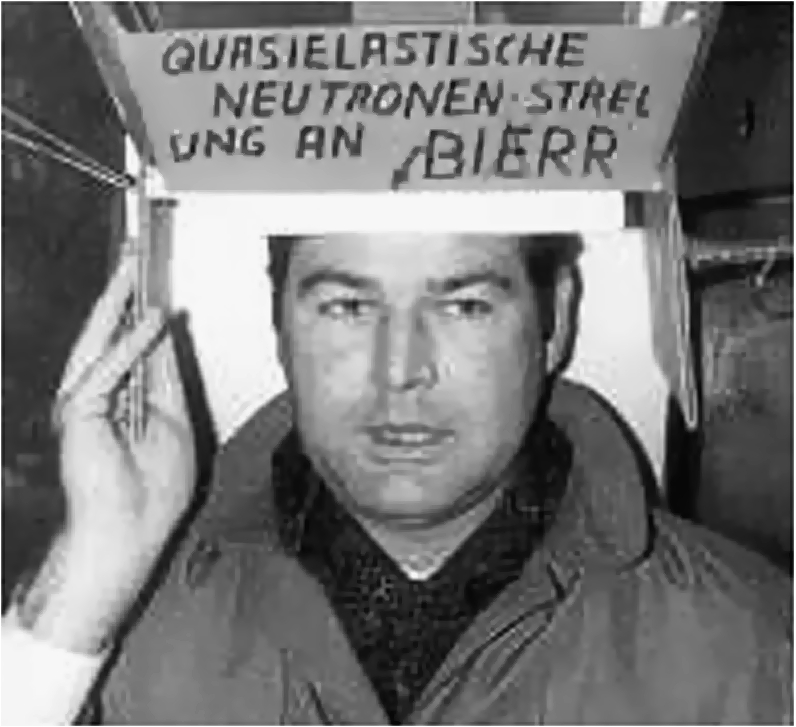
After some unsuccessful attempts, we visited the institute of Heinz Maier-Leibnitz at the FRM in Garching and were received by the big boss personally. His nickname at the institute was ML. He proposed two subjects for a thesis. One was about improvements of the existing gravity diffractometer and to measure coherent neutron scattering lengths with very high precision. The other was to develop from scratch a new technique in neutron spectroscopy. ML scribbled on his blackboard a setup consisting of a neutron beam out of the reactor, a crystal, a sample, a second crystal, and a neutron detector; like a TAS spectrometer with the difference that both crystals were oriented with a Bragg angle of 90°.
ML explained to us that in this way a very high-energy resolution of the order of
Fig. 2.
Heinz Maier-Leibnitz (1911–2000).
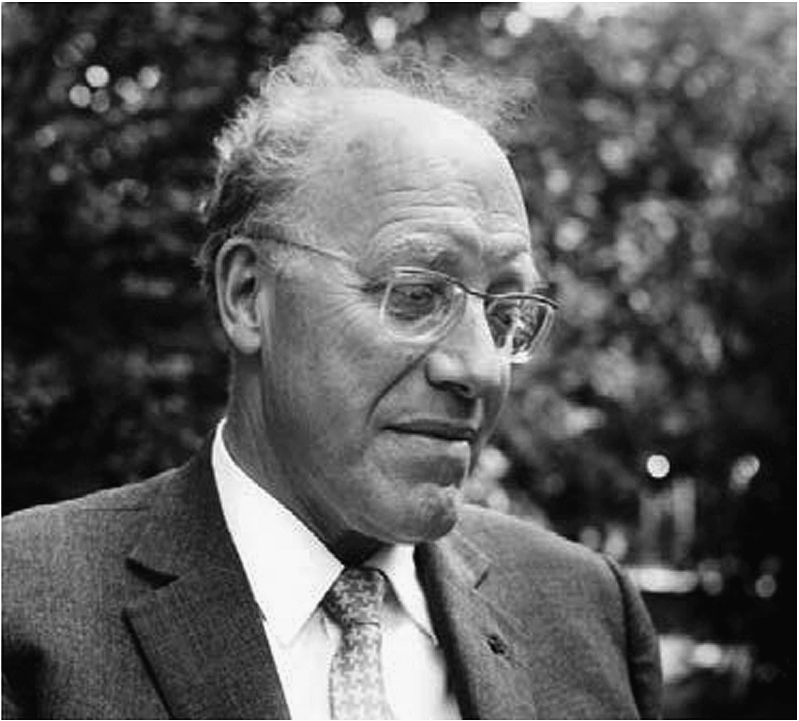
Bert Alefeld became our tutor. A few weeks after our start ML left the institute in Garching to manage the HFR project in Grenoble. So later we met ML only about once every two months, where we were invited to present our progress reports to him. This was quite good for us, leaving us a lot of freedom. We enjoyed very much to work with Alefeld, who was in the process of finishing his own thesis, which was too demanding a task, consisting of building a bundle of three neutron guides (the first ones at the FRM) and the BSS! When we arrived at the FRM in November 1966, the first neutron guide was just finished. We helped Alefeld to perform gold-foil activation measurements to determine the total neutron flux. Instead of developing the BSS, Alefeld installed at the end of this guide a Backscattering diffractometer (BSD) to perform high-precision measurements (
A year before Alefeld had installed a double-crystal setup in near BS geometry on a vertical beam tube in the FRM above the core, and did the first BS experiments with lead and silicon crystals proving that one can achieve μeV with the BS geometry. He showed also, for the first time, the effect of gravity on free-falling neutrons.
Fig. 3.
(a) The first BS setup on a vertical tube at the FRM (1964). (b) Berthold Alefeld (2003).
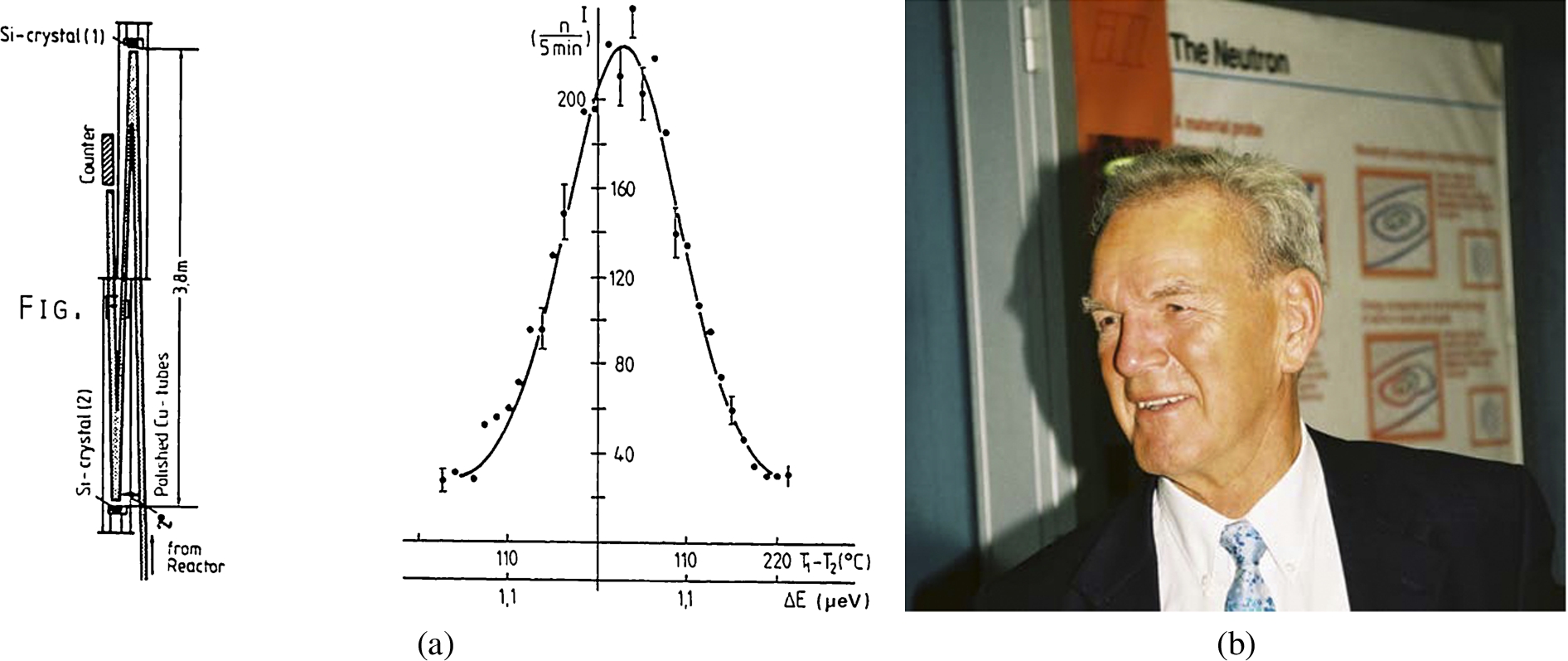
This experiment was published in the “Sitzungsberichte der Bayerischen Akademie der Wissenschaften”, a typical choice for ML, who liked to publish in not so well known journals!
So that was the start. Coming back to our thesis work, the first thing to do was to try different techniques to build small neutron guides. One idea was to use commercially available microwave guides made of brass. The problem was the surface quality. So after some unsuccessful polishing trials we abandoned this line. Fortunately Erich Steichele had just started to develop a 140 m long thermal neutron guide at the FRM, as a prototype for a project at the HFR in Grenoble. After many useful discussions with Steichele, we were able to build our own glass guides with the required dimensions (4 × 4 and 2 × 2 cm2 cross section respectively).
Fig. 4.
Erich Steichele: Technology transfer from neutron guides to living room tables (2006?).
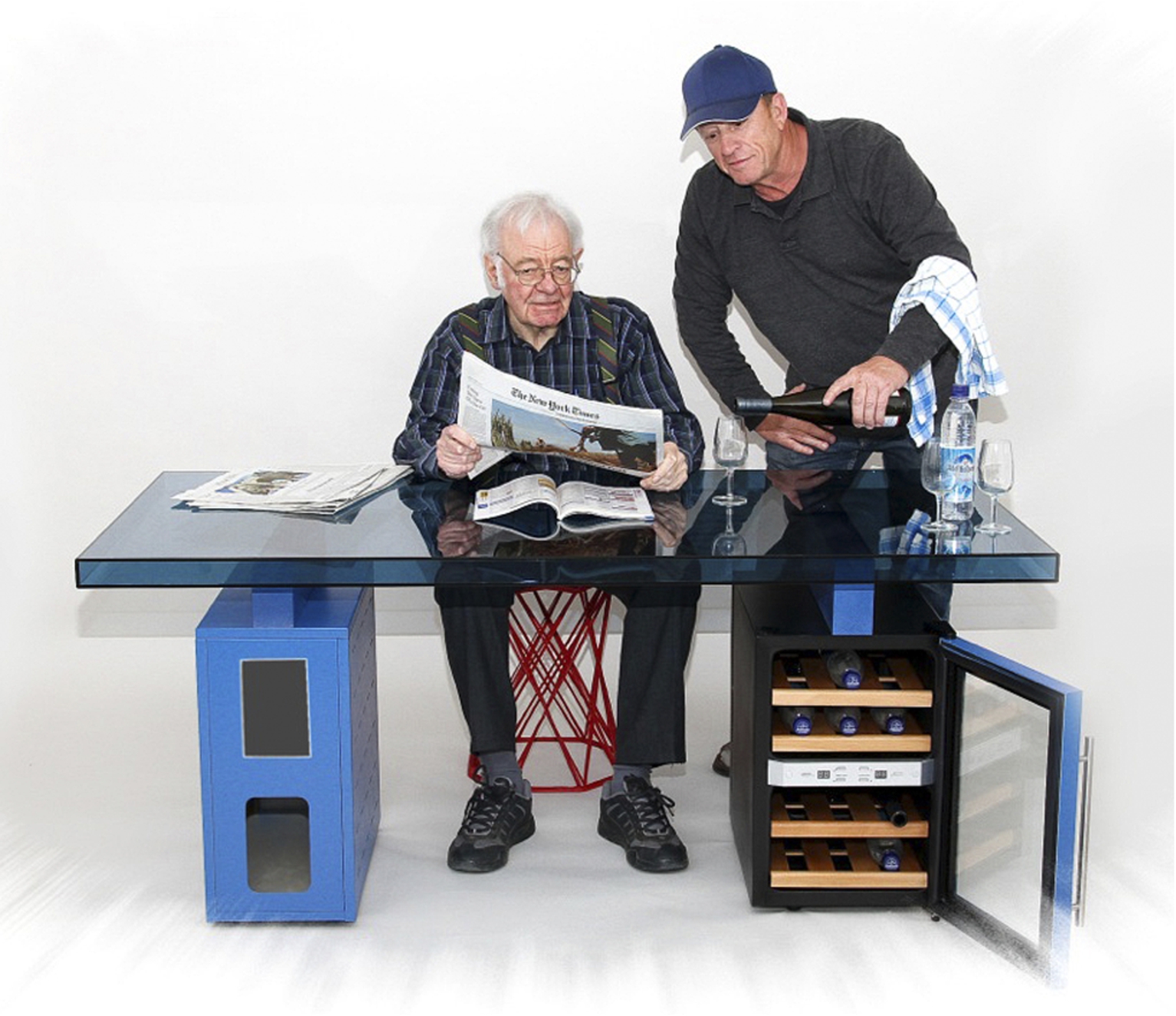
The spectrometer included also a neutron trumpet: a converging guide with a nickel coated inner surface focussing the beam onto the sample. The Doppler drive for the monochromator was the same as that of Alefeld, who had brought it from the Mössbauer institute next door. A disk chopper, used to separate the incident from the scattered neutrons, was developed by us, as well as all other mechanical parts. We subcontracted the fabrication to the mechanical workshop of the institute. It was very important to have excellent relations with the workshop. This was in part achieved with a gift of a ‘Bier-Tragl’ now and then.
The biggest problem was how to build the large solid-angle analyser: how to align about 2000 Si single crystals with a precision better than1/10° on a spherically curved surface? The problem was finally solved like the ‘Ei des Kolumbus’: A laboratory at Siemens was able to cut silicon single-crystal rods into thin slices with a precision of 0.10°. A small company produced 25 Al plates for us with a curved surface. We glued the 2000 Si single crystal slices by pressure with our thumbs and later (after health problems) with a pneumatic piston using a special contact glue. NB.: the crystals remained bent on the curved surface. In this way the optical quality of the mirror was improved, and the strain induced an increase in the reflectivity.
After about 1.5 years of hard work the spectrometer was finished and we started the tests. A vanadium spectrum was obtained in 10 days with a signal to noise ratio of about 1. The good news was: the energy resolution was 0.6 μeV FWHM for an incident energy of 2 meV.
Now, new physics could be tackled in the domain of μeV or GHz. Manfred Birr started to measure Plexiglas at different temperatures obtaining only unbroadened spectra. Much more successful was the Quasielastic Neutron Scattering (QNS) in glycerol. The QNS broadening was much narrower than the one determined previously by Larson with much coarser energy resolution with a time of flight spectrometer.
The science part of my thesis was to try to measure hyperfine splittings in solids. Two options were considered: quadrupole or magnetic interactions. The first one was tackled with an As2O3 sample at room temperature. From NQR a splitting of 0.5 μeV (about 120 MHz) was known to exist. After a very long run of 450 hours not even the elastic peak could be seen! (Later, I repeated the experiment several times at the ILL without success. In a discussion with Otto Schärpf he tried to explain to me that the experiment cannot work in principle! Until now, I could not prove experimentally that Schärpf is wrong.)
Fig. 5.
Tasso Springer in the ‘Garchinger Heide’ meditating on the FRM project (1956).
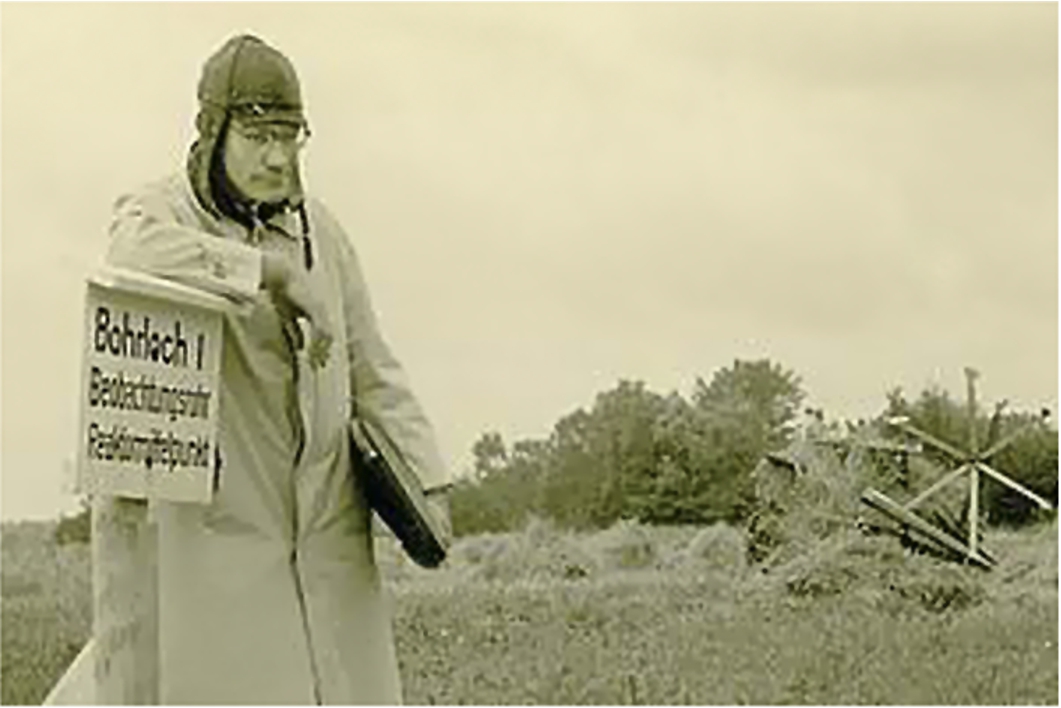
My second trial was V2O3 below its antiferromagnetic phase transition. For this I needed a liquid-nitrogen cryostat, which we had to develop by ourselves. After a very long run of 505 hours a spectrum was obtained at 105K with still bad statistics but which showed a shoulder on each side of the elastic peak: Inelastic Spin Flip Scattering on Zeeman hyperfine split nuclear levels of Vanadium was born! Or at least there was some indication of it. Fitting this spectrum with a 3 peak model gave a HF splitting of 175 ± 15 KOe (0.81 μeV) at the vanadium nucleus. The value was confirmed in later experiments. This was a nice result. The Glycerol and the V2O3 results were published and our twin theses were finished at the end of 1969. We had given the proof to ML that a twin team of thesis students could work.
We both started to look for jobs. Manfred ended up in industry. After a 2 month trial in a laboratory at Siemens in Munich I felt strongly that industry was not my place of choice, and I was very lucky that I could get a postdoc position at the FRM in the institute I had left only a few months before! The first thing in 1970 was to dismantle the BSS because of the very low data acquisition rate, as I knew that a new project was in the pipeline in Jülich. I had a number of students working on several other BS development projects: Measurements of lattice parameters near phase transitions, very high resolution tests of Si at higher orders, monochromator tests in BS conditions, tests of BS with thermal neutrons, bunching TOF tests and preparation of experiments to be performed at the coming Pi-spectrometer at the KFA Jülich. There was also Mathias Hetzelt, a thesis student, who continued the work left by Knut Berndorfer on polarised neutrons with polarisation analysis in collaboration with our theoretician Kurt Binder, who later became the Monte Carlo simulation specialist.
2.Jülich 1970–1972
Bert Alefeld left Garching in 1969 to take the job proposed to him by Tasso Springer at the KFA Jülich to build a new BS spectrometer (the Pi-spectrometer) on the cold-neutron source of the Dido reactor.
The flux expected was about a factor 100 higher than in Munich! A new transparent Doppler drive was developed. A pyrolytic graphite crystal deflector was inserted in the primary spectrometer. Crystal quality tests were performed at the FRM. Several Q values could be measured simultaneously with a multi-arm analyser. When the spectrometer was ready for tests, Bert Alefeld invited a few young scientists to help him to commission the instrument. Tanja Peterlin, Claude Zeyen and I were among the happy few. Tanja was in the process of finishing her thesis on QNS in polymers. Claude worked on Ferroelectrics. I continued to work on hyperfine interactions. After my arrival in Jülich with my samples and a specially developed 4He cryostat with a narrow tail it turned out that the cryostat formed a leak during the travel in my VW Käfer! So a technician at KFA helped me to get rid of the leak. When we were ready to go down to He temperatures a wrong manipulation made the 4He vessel implode! But again, with the help of the cryogenics group, the vessel was rebuilt in record time and finally the V2O3 sample could be measured. Before that the resolution function had been measured under different conditions. A FWHM of about 0.3 μeV was obtained with polished Si crystals, an excellent result! The intensity was as good as expected.
The V2O3 sample was put into the cryostat in an evening in early 1971 and the run started at 5K. I went to bed and had bad dreams. Remember that the statistics of the spectra obtained at the FRM were not very good. Next morning Bert and I went to the instrument. Everything seemed to be OK. The instrument was working the sample temperature was still at 5K. We stopped the run and looked at the screen: There was a nice elastic peak on it, and no inelastic lines. I felt close to a heart attack. A disaster! Maybe my whole thesis was untenable.
But a closer look at the spectrum revealed an indication of some weak inelastic feature at the bottom of the resolution peak. Optimistic conclusion: Maybe the sample was not good anymore. In fact V2O3 is a member of the Magneli family
The Pi spectrometer was a great success. Thesis students of Bert Alefeld used it to open new research fields in the μeV resolution domain of QNS. Ait Salem studied self diffusion in a Na single crystal. Dieter Richter and Johannes Töpler investigated H motions in N doped Nb and NH4 reorientations in NH4Cl. Bert Alefeld measured the tunnelling spectrum of CH3 groups in Di-methyl-di-acetylene for the first time. Many other successful experiments followed and some of them were improved and completed later at the IN10 in Grenoble.
3.Grenoble and elsewhere 1971–2014
ML left the position of the ILL director in 1970 and Rudolf Mössbauer took over. This was the time when discussions took place about the second generation of ILL instruments. Tasso Springer pushed for a BSS at the ILL. ML discussed the option of a so-called bunching TOF spectrometer, where one uses time focussing of neutrons from an accelerated mica monochromator. Tanja Peterlin was engaged in this project. Feri Mezei came up with his ingenious idea of neutron spin echo (NSE).
So Mössbauer had to make a decision. He was very enthusiastic about NSE and thought that a BSS was less interesting. But under the pressure of the lobby in Munich and Jülich he decided to have both the NSE and the BSS built. But he posed some conditions for the BS project. It should be cheap (an attitude that came from his former thesis adviser – ML): the Springer institute in Jülich should provide the Doppler drive and the PDP11 computer. Munich should provide a postdoc for the time of construction. The postdoc was me. I was happy to leave Munich for about 2 years. I arrived in Grenoble in my VW Käfer, after a wonderful trip through the Alps passing through Chamonix and admiring the view of the Mont Blanc in autumn 1972.
Fig. 6.
Mike Lomer and Rudolf Mössbauer, ILL Directors (1974).
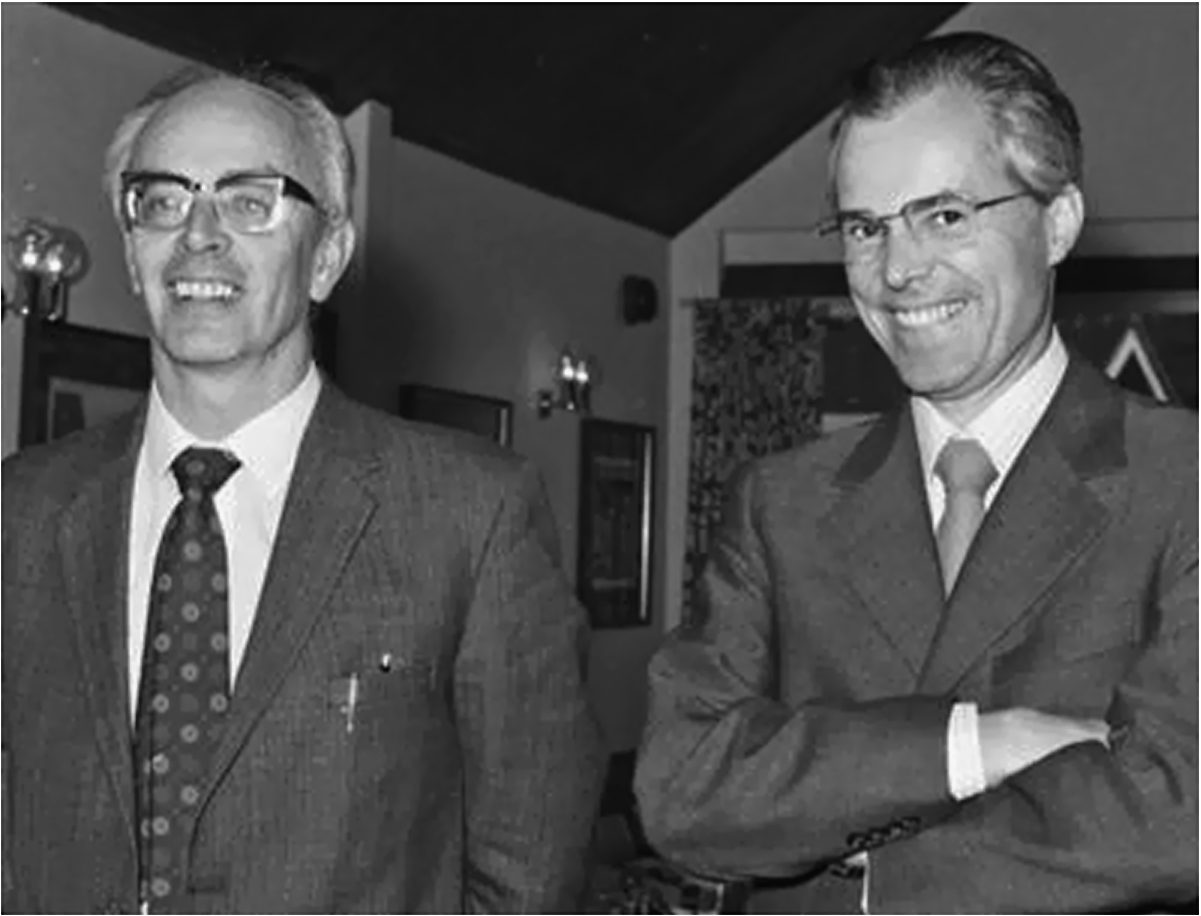
The choice of the instrument site was a free position on the cold neutron guide H15 between D7 in construction and D11 already operational. The instrument got the name IN10 because IN9 existed as a project for a TOF instrument with polarisation analysis using a polarised proton target (Peter Seyfert and Graham Jenkin): a project later abandoned. The technical services of the ILL (G. Gobert, I. Lefevre, P. Ledept and others) did a remarkable job to build the IN10 spectrometer in record time of about 2 years with a very tight budget, orders of magnitude smaller than what is spent today for a similar instrument! Naturally, everything was modest, but it worked. The use of the PDP11 computer was, by the way, a nightmare! Beginning 1974 we started to commission the instrument. The intensity was as expected about 10 times higher than in Jülich, the energy resolution very similar. After some vanadium runs I put a sheet of cobalt metal into the beam position of IN10. After one night the result was fantastic: a spectrum consisting of 3 completely separated peaks of nearly equal height with a hyperfine splitting of about 1 μeV ready to be published in a text book.
I printed the spectrum with the teletype, went up to the office of Mössbauer, and slipped the paper under the locked door (it was a Sunday morning). On the following day Mössbauer called me up and told me that he was very impressed by the result.
Fig. 7.
Hyperfine splitting in cobalt at room temperature. The spectrum obtained on the BSS IN10 was published in Z. Physik B20, 385–389 (1975).
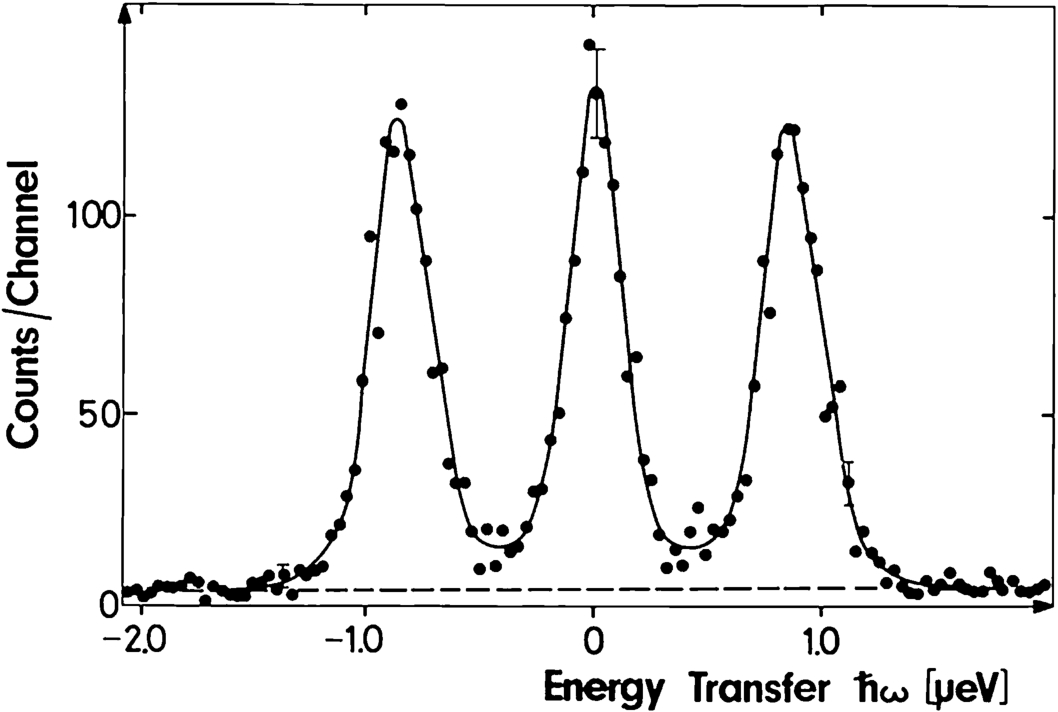
The first user experiment to be published was that of Julia Higgins et al. on QNS in a polymer. This was the beginning of the success story of IN10, which became the workhorse in BS spectroscopy and stayed that way for a long time. I remember with pleasure the excellent collaboration with Spencer Howells, who developed the first data evaluation program for IN10. My scientific activity was at that time concentrated on the study of hyperfine interactions with a challenge to measure nuclear spin waves (NSW) with neutrons. A thesis student, Bob Word, of Stephen Lovesey did a lot of calculations of INS cross-sections. With Dieter Richter we tried hard but without success to do a suitable experiment. I finally stopped working in the nuclear spin excitation domain and joined the tunnelling experts! Only rather recently the NSW domain has been revived by Tapan Chatterji, who investigated successfully Nd, Co and Ho intermetallics. In a recent paper by him he claims that he has detected an indication of NSW’s: to be confirmed!
Key experiments were among others the tunnelling motions of CD4 and CD3 groups. It was a great pleasure to work with Werner Press, Alfred Hüller, Jim A. Morrison, Michael Prager during these, and other, related experiments. Another grateful thought comes up remembering many exciting experiments done on different ILL spectrometers with the NMR specialist Stan Clough. We were complementary: NMR combined with INS!
I cannot forget one event that happened on IN10. Alan Leadbetter and Francis Temme did an experiment on QNS on a liquid crystal in a magnetic field. They used a water-cooled electromagnet. One early morning during this experiment I checked the state of the instrument. To my surprise water was dripping out under the sample area. I stopped the experiment, opened the instrument sample area and found under the sample magnet a pool of water! A leak of the cooling system of the magnet had filled the sample area of IN10 with a couple of litres of water! I hope that Alan Leadbetter, who became later was one of the ILL directors, will forgive me for publishing this story here!
IN10 retired in 2013 after 39 years of loyal service! During this long period the instrument evolved. In 1991 a temperature-scanning monochromator with a number of different crystals became available, allowing energy scans up to 1 meV compared to 15 μeV with the Doppler drive. Jeremy Cook and Jean-François Barthélémy were much involved in the development of the cryo-furnace. With this mode the temperature dependence of the roton minimum of He4 could be investigated with μeV resolution.
During this time the competition between BSS and NSE continued. But it rapidly turned out that the techniques are complementary to each other. A paper comparing the 2 techniques was published in 1984. BS is very good for incoherent scattering from hydrogen etc, especially true for inelastic features in the μeV domain. NSE is the tool for coherent QNS at small momentum transfers in the ns to ms time domain.
IN10 was built for cold neutrons. But from start a second set of analysers with Si(311) crystals was assembled allowing experiments with momentum transfers up to 3.8 A−1. This setup was used by Ruep Lechner to measure successfully the Elastic Incoherent Structure Factor (EISF) as a function of momentum transfer. This experiment triggered the construction of a new BSS for thermal neutrons. The instrument IN13 was installed in an intermediate position on the thermal neutron guide H24 at the ILL, and became operational in 1981. Momentum transfers up to 5.5 A−1 with an energy resolution of 5 μeV at an incident energy of 16 meV could be attained. The beam position on a thermal guide is not ideal. Due to the small beam divergence of the nickel-coated guide, the resulting small intensity limits quite severely the domain of applications. Since a number of years IN13 is used as a CRG instrument for biophysical applications. The preferred scan method is that of the so-called fixed window scan at zero-energy transfer by scanning the sample temperature. The instrument is still in operation in 2014 and was developed in collaboration with Jean-Louis Buevoz, Ian S. Anderson and Winfried Petry.
Returning to IN10 a proposal was made in 1990 to shift the instrument to a better guide position that became available on the second cold source during the 2ème soufflé. Finally it was decided to leave IN10 where it was, and to build a new BSS IN10B in the new guide hall ILL22. Andreas Magerl was the project leader, succeeded by Bernhard Frick. IN10B had a higher flux, about a factor of 2 compared to IN10, the energy resolution being the same. The new instrument was easier to use due to its multicounter and more comfortable mechanics.
The ILL has always been a very dynamic institute that constantly developed new ideas and improved existing instruments. The Millenium Project is a clear example of this activity. Bernhard Frick proposed a new cold BSS profiting from a excellent new cold guide using super-mirror focussing techniques, and applying the phase-space transformation from white to wide invented in 1984 by Alefeld and Schelten. This technique was for the first time used at NIST for their BSS in 2000. Later it was also realised at the new FRMII reactor near Munich in 2003 (Spheres). This technique allows an intensity gain factor of about a factor 5.
So the ILL was obliged to invest in this project in order to remain competitive. The new instrument IN16B started operation in 2013. It is a very sophisticated machine that can be run in several modes of operation. It has vacuum in the primary and secondary flight path to optimise intensity and to get a very low background. The new Doppler drive uses the principle of a linear motor, developed by Michael Prager in collaboration with industry. It provides a larger energy transfer range up to ±30 μeV. The intensity is about 5 times higher than that of the preceding instrument, which was dismantled and parts of it are in use on the new instrument. Compared to the first BSS at the FRM the new IN16B has a flux at the sample position more than 4 orders of magnitude higher! This enormous gain in flux is explained by a better reactor (100), with a cold source (10), a ballistic neutron guide using focussing super-mirror technology (10), and a phase-space transformer (PST) (5). Evidently an important amount of research and development has been invested in the IN16B project using modern Monte Carlo simulations for the neutron optics, finite-element calculations for the PST, and know-how of the industry for the Doppler drive. All this explains also the budget needed to build IN16B and similar instruments like Spheres at the FRMII and the one at NIST. It can be expected that the use of IN16B with its high flux will allow tackling more difficult experiments on samples with low scattering, high absorption, small size etc.
I did not mention the numerous experiments that failed, or were not published for diverse reasons during the past years. This is how research works: You cannot know the result before you start – otherwise you would not do the experiment!
I will not talk about the inverted TOF BS spectrometers like those at ISIS at Rutherford, BASIS at Oak Ridge and MARS at PSI. This subject merits another history story! Another story would be that of X-ray BS spectroscopy pioneered at DESY by Bruno Dorner and then further improved and brought to maturation at the ESRF by Francesco Sette and colleagues.
4.Conclusion
The neutron backscattering technique opened a new domain in neutron spectroscopy passing from meV (THz) to μeV (GHz). It is complementary to NSE. I think that the large number of publications of new results in various fields of science obtained from the use of the high-resolution neutron BS spectrometers shows that the following statement which is attributed to ML is true:
‘The development of a new method, whenever its precision, sensitivity, or resolution is much better than everything that existed before leads to new science’.
Acknowledgement
I would like to emphasise that this contribution represents a personal view of the history of BS without any claim of completeness. I apologise for all the omissions, errors and other mistakes. The idea was to put onto paper historical facts that cannot be found in the normal scientific publications, but are still important.
I would like to take the opportunity to thank here all my colleagues with whom I had the pleasure to work over many years.
Fig. 8.
The BS clan: from left to right: Anton Heidemann, Michel Prager, Bert Alefeld, and Bernard Frick, at the ILL in (2003).
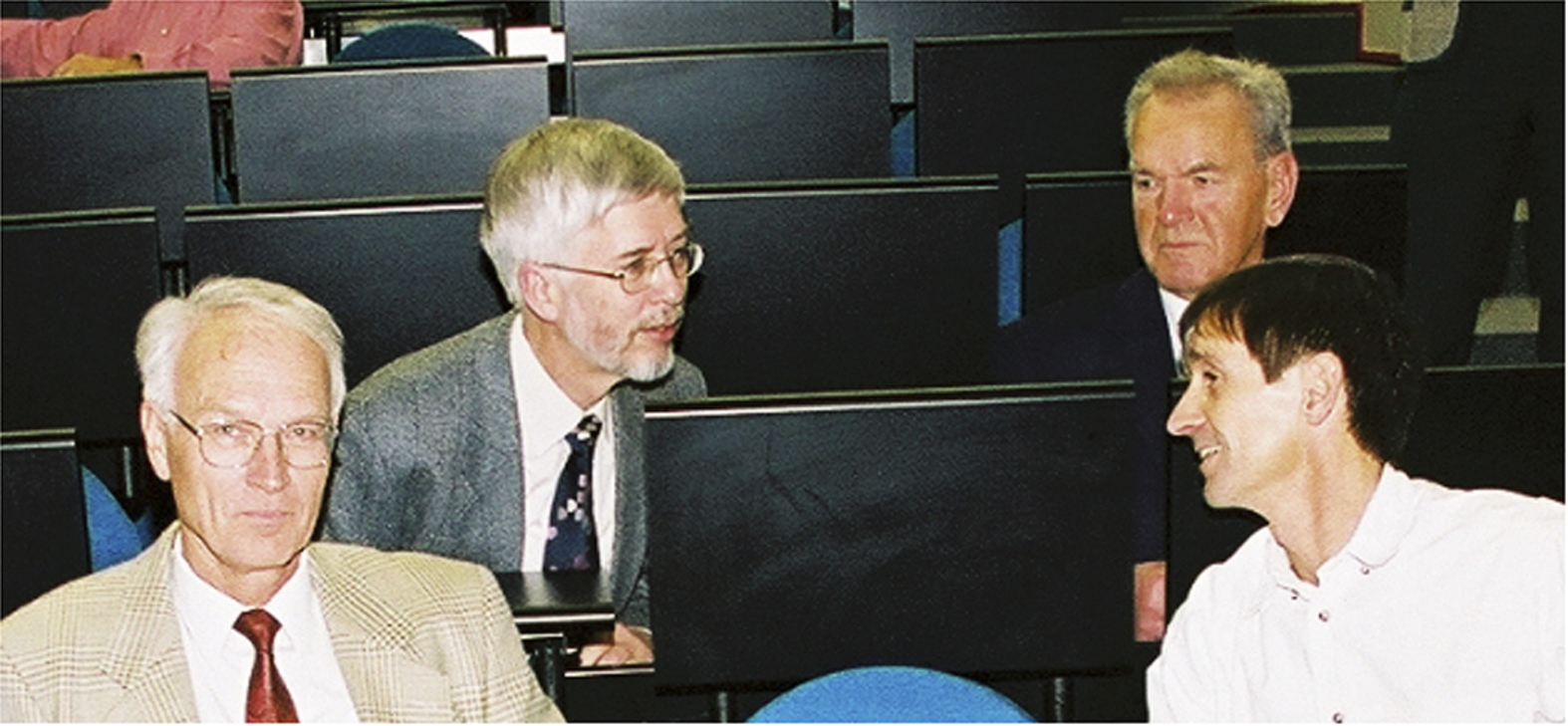
Many of them are not mentioned in this paper, but they are not forgotten. My special thanks are dedicated to my friend Manfred Birr for the wonderful time we have spent together during the thesis as twins. It is very sad that Bert Alefeld has left us too soon (Nov 2012). It is he, after all, who is the real father of backscattering of neutrons.
Grenoble, August 2014