Determinants and Characterization of Locomotion in Adults with Late-Onset Pompe Disease: New Clinical Biomarkers
Abstract
Background:
The late-onset form of Pompe disease (LOPD) is characterized by muscle weakness, locomotor limitations and a risk of falls. The mechanisms responsible for altered locomotion in adults with LOPD are unknown. The identification of clinical biomarkers is essential for clinical follow-up and research.
Objectives:
To identify muscle determinants of impaired locomotor performance, gait stability and gait pattern, and biomechanical determinants of falls in adults with LOPD.
Methods:
In this cross-sectional, case-control study, LOPD and control participants underwent 3D gait analysis, locomotor performance tests and muscle strength measurements (isokinetic dynamometer). We explored the muscular determinants of locomotor performance (gait speed, 6-minute walk test distance and timed up and go test), gait stability (spatiotemporal gait variables) and the gait pattern. We also explored biomechanical gait determinants of falls. After intergroup comparisons, determinants were sought to use forward stepwise multiple regression.
Results:
Eighteen participants with LOPD and 20 control participants were included. Locomotor performance, gait stability, and the gait pattern were significantly altered in LOPD compared to control participants. Hip abductor strength was the main common determinant of locomotor performance, gait stability and pelvic instability. Hip flexor strength was the main determinant of abnormal gait kinematics at the hip and knee. Percentage duration of single support phase during the gait cycle was the main determinant of falls.
Conclusions:
Hip abductor strength and percentage duration of single support during gait were the major determinants of locomotor performance, gait stability, falls and the gait pattern in LOPD. These new clinical biomarkers should therefore be systematically assessed using instrumented tools to improve the follow-up of adults with LOPD. They should also be considered in future studies to accurately assess the effects of new therapies. Hip abductor strength and single support phase should also be priority targets for rehabilitation.
INTRODUCTION
Pompe disease is a rare and progressive neuromuscular disorder caused by a genetic deficiency of the enzyme acid α-glucosidase (caused in 90% of cases by c.-32-13T>G mutation), which induces an overload of lysosomal glycogen [1].
Late Onset Pompe Disease (LOPD) is characterized by weakness of axial and proximal lower limb muscles. According to the current literature, the muscles that are initially and most severely affected are, hierarchically, the hip adductors and abductors, the lumbar extensors, the hip extensors, the knee and hip flexors, and the knee extensors (the ankle muscles are less affected) [2–4]. However, the heterogeneity of studies on muscular impairments limits the precision of this classification. In almost 80% of adults with LOPD, these muscular deficiencies are associated with alterations in locomotor activities. [4, 5].
Locomotor performance is defined as the result of forward progression the body during a walking task, corresponding to the walking speed or distance. For example, walking speed or distance are considered performance parameters. Locomotor performance can also be evaluated by assessing biomechanical gait variables, including spatiotemporal gait parameters, the gait pattern and gait stability. Gait stability is defined as the ability to limit body sway during gait and refers to gait adaptations to achieve the required task without falling [6].
Alterations in walking performance (in straight line or complex tasks as Timed up and go) and alterations in walking stability are common in adults with LOPD [7–10] and are often associated with recurrent falls [8, 9]. Associated with these alterations in stability, gait pattern adaptations are reported in adults with LOPD, with a gait often described as waddling [4, 5, 7, 10].
However, the mechanisms underlying the reduced locomotor performance and stability and altered gait pattern remain little explored in LOPD. Identifying the determinants of these locomotor alterations is crucial to increase our knowledge of gait disorders in LOPD. Knowledge of the relationship between muscle weakness and locomotor alterations in adults with LOPD would provide a better understanding of the mechanisms underlying these impairments and therefore would guide their treatment. To our knowledge, only two studies have explored these relationships [11, 12].
The first study showed that the strength of the hip abductors and hip flexors, assessed with a manual dynamometer, was related to impaired walking ability, measured on a four-category scale [11]. However, locomotor assessments should not be reduced to a measure of capacity, and the small number of muscles assessed highlights the need for further investigations. The second study assessed walking with inertial sensors and muscular strength with manual muscle testing (MMT) [12]. It found moderate correlations between adductor and hip flexor strength and cadence, as well as between an overall hip muscle score and the percentage duration of the single support phase during the gait cycle (% SSP). These two studies used MMT or a manual dynamometer, however these tools lack reliability and sensitivity (they are therapist-dependent and cover a wide range of absolute strength values) [13, 14]. Use of the recommended gold standard strength assessment isokinetic dynamometer would provide more robust results [13, 15, 16].
The relationship between % SSP and proximal lower limb strength in LOPD found by Schneider et al. is interesting since this parameter is often used to characterize dynamic stability during gait [12, 17]. % SSP is a spatiotemporal gait parameter that provides information on how the person generates their walking speed.
Identification of the determinants of locomotor alterations requires the exploration of biomechanical gait variables (not only the ability or timed performances). The only three studies that explored spatiotemporal gait parameters in adults with LOPD found a decrease in walking speed, cadence and % SSP compared to controls [7, 10, 12]. One study also analyzed the gait pattern and found an increase in pelvic tilt and hip adduction range of motion compared to controls [7]. However, these studies did not explore the mechanisms behind the impairment of locomotor performance and the gait pattern. Gait stability has not been studied, neither have the determinants of falls and their relationship with gait variables or muscle weakness.
For these reasons, it is essential to identify the determinants of impaired locomotor performance, gait stability and the gait pattern in adults with LOPD. Data relating to the criteria highlighted could guide assessments and rehabilitation and could be the focus of further longitudinal studies aiming to find sensitive-to-change clinical biomarkers. This cross-sectional study aimed to identify the muscular and biomechanical gait determinants of altered locomotor performance, gait stability, and the gait pattern in adults with LOPD. To achieve this aim, we first identified the locomotor alterations relating to performance, stability, and the gait pattern in adults with LOPD compared to healthy controls. Given the major impairments of hip muscle strength in LOPD, we hypothesized that these muscles would be mainly involved in the locomotor alterations identified.
MATERIALS AND METHODS
Protocol and registration
This case-control, cross-sectional study was conducted between November 2019 and March 2022 at the Raymond Poincaré University Hospital, neuromuscular disease reference center. This study is ancillary to the “POMPE” protocol (ClinicalTrials: NCT03564561), a longitudinal follow-up of adults with LOPD aiming to identify sensitive biomarkers for future therapy trials.
Standard protocol approvals, registrations, and patient consents
The study was conducted in accordance with the ethical codes of the World Medical Association (Helsinki 1975). It was approved by the regional ethics committee (CPP X Ile-de-France, Protocol n° 43-2018). Written informed consent was obtained from all participants in the study. This study is presented according to the STROBE 2007 guidelines (presented in the Supplementary material).
Data availability
Data were collected in our motion analysis laboratory and the neurology department. Anonymized data not published within this article will be made available by request from any qualified investigator.
Participants
Participants with LOPD were recruited from neuromuscular consultations if they met the eligibility criteria. Healthy participants were recruited from the general population (via advertisements) and matched to the LOPD group after screening for confounders (age, sex, weight and height).
Eligibility criteria
Inclusion criteria
To be included, all individuals had to be aged between 18 and 80 years. Adults with LOPD had to have Pompe disease (c.-32-13T>G mutation confirmed by a genetic test) and be able to walk 10 meters without technical or human assistance.
Non-inclusion criteria
Adults with LOPD were not included if they were dependent on invasive mechanical ventilation, could not walk independently without a technical device, had comorbidities or had an estimated survival of less than 12 months. Healthy subjects were not included if they had neurological, orthopedic, medical or other conditions that could interfere with gait.
VARIABLES AND MEASUREMENTS
Locomotor assessments
Locomotor performances
Locomotor performance was explored using 3 tasks:
– Walking distance during the 6-minute walking test (6MWT);
– Spontaneous gait speed measured using 3D gait analysis (averaged speed of 8 trials on 10m walkway);
– Performance time of a complex locomotor task evaluated using the Timed Up and Go (TUG) test performed as quickly as possible
For these tests, no walking aids were used.
Material and procedures of the gait analysis
The 3D gait analysis was performed using an optoelectronic system OptiTrack (NaturalPoint, Corvallis, OR, USA) comprising 15 optoelectronic cameras. The trajectories of 58 reflective markers positioned on anatomical landmarks according to the Conventional Gait Model were recorded [18]. Participants performed 8 barefoot gait trials at a comfortable pace over a 10 m long walkway. QTM 2020.2 and MOKKA Biomechanical ToolKit 0.6.2 were used to respectively label markers and define gait cycle phases. All spatiotemporal and joint kinematic gait parameters were post-processed with PyCGM 2.5, an open-source Python implementation of the Conventional Gait Model [18]. The same experimenters performed all the analyses to ensure reliability.
Gait stability parameters
The spatiotemporal gait parameters are commonly used to assess dynamic gait stability [19, 20]. Spatial parameters include step length and step width, and temporal parameters include cadence and the phases of the gait cycle (speed is a spatiotemporal parameter). The gait cycle is divided into swing and support phases (including single and double support phases). Among the gait cycle phases, the single support phase (SSP) is the most unstable phase of gait (small base of support on one leg) and is closely related to the risk of falls [19, 20]. This parameter is considered to be the one that best characterizes gait stability, and the mean value and variability of % SSP are often used in studies of individuals with neurological diseases [17, 19, 21–24]. We also analyzed the percentage duration of the double support phase, the cadence, the step length and the step width [22, 25].
As recommended, the mean value and variability (standard deviation) of the spatiotemporal gait parameters were analyzed in both groups, since these dispersion measures are relevant for the evaluation of gait stability [19, 20, 26, 27].
Finally, knowing that the major consequence of locomotor stability alterations in neuromuscular diseases is the falls, the number of complete falls reported by the LOPD participants during the last 3 months was collected.
Gait pattern
We calculated the following kinematic joint variables from the 3D gait analysis to analyze the gait patterns:
• Pelvis: mean tilt (sagittal), tilt ROM, contralateral drop (frontal) and rotation ROM,
• Hip: maximum peaks and ROM in the 3 planes,
• Knee: maximum peaks and ROM in flexion and extension and mean position at foot strike.
• Ankle: maximum peak dorsiflexion and plantar flexion during the support phase and maximum peak dorsiflexion during the swing phase.
Propulsion, breaking and vertical ground reaction forces were recorded with 2 AMTI platforms (BP400600, Advanced Mechanical Technology Inc.) during the gait analysis.
Muscle strength assessments
Maximal voluntary torque (MVT) was measured using an isokinetic dynamometer (Biodex Medical System 4, Shirley, NY) to assess muscle strength. The hip flexors and extensors were assessed in supine (hip at 30°). The hip adductors and abductors were assessed in side lying (hip at 15°). The knee flexors and extensors were assessed in sitting (knee at 90°), and the ankle flexors and extensors were assessed with the knee and ankle in neutral. The trunk and the thighs were strapped to the device, and the participants placed their hands on their chests to avoid compensatory movements. Given the symmetry of the muscle impairment in LOPD, and to limit fatigue during the assessments, only the dominant side was assessed. All measurements were performed by the same therapist to avoid inter-rater variability [28]. After familiarization with the dynamometer, a warm-up was performed with 3 submaximal contractions (weak, medium and strong). Three trials of the maximal voluntary contraction were then recorded for each muscle group. Participants were verbally encouraged to perform maximal contractions [29]. A 1-minute rest was imposed between each contraction. The highest torque value of the 3 trials was selected.
STATISTICAL METHODS
Data were analyzed using JASP® version 0.16.4 with an alpha risk of 5%. Means, standard deviations and medians were calculated. The standard deviations of the gait parameters were calculated across each gait cycle for each participant and then the average variability for each group was calculated.
Before identifying the determinants of locomotor alterations, we compared gait and muscle strength variables between LOPD and control participants using the nonparametric Mann-Whitney U. test (p <0.05) and calculated the α rank biserial correlation to indicate the effect size.
For our primary objective, determinants were identified using forward stepwise multiple regression models. We submitted to the model all muscle strength parameters (MVT) and altered spatiotemporal gait parameters (highlighted by significant intergroup comparisons).
All variables below the 0.05 significance level were retained by the model as input variables. After their entry into the regression model, variables that did not contribute to the increase in variance were excluded at a significance level of 0.1. Box-Cox’s transformation method was used for non-normally distributed data before inclusion in the regression model.
To identify the muscular determinants of locomotor performance, 3 forward stepwise multiple regression models were used for the gait speed, 6MWT distance and TUG performance time.
A forward stepwise multiple regression was conducted to identify the biomechanical gait determinants of falls and then a second stepwise regression was conducted to identify the muscular determinants of gait stability variables.
The forward stepwise multiple regression models were also used to identify the muscular determinants of major kinematic characteristics in the adults with LOPD (compared to the controls).
RESULTS
Thirty-eight individuals were included: 18 adults with LOPD (c.-32-13T>G mutation confirmed by a genetic analysis), mean (SD) age 58.0 (12.8) years, and 20 healthy controls aged 58.1 (9.1) years. The mean time since diagnosis of LOPD was 15.0 (9.2) years. Participant characteristics are shown in Table 1. Adults with LOPD did not have a special diet and 16/18 took part in physiotherapy in the community at least once per week. There were no between-group differences for sex ratio, age, height or weight. Ninety percent of the participants were on treatment (two refused treatments and one stopped treatment prematurely due to an allergic reaction).
Table 1
Participant characteristics
LOPD group | Control group | p-value | |
(n = 18) | (n = 20) | ||
Sex ratio, M/F, n | 10/8 | 10/10 | 0.85 |
Age years, mean (SD) | 58.0 (12.8) | 58.1 (9.1) | 0.96 |
Height, cm, mean (SD) | 168.2 (8.1) | 169.8 (9.6) | 0.84 |
Weight, kg, mean (SD) | 70.1 (15.0) | 72.1 (12.5) | 0.78 |
Modified Gardner-Medwin and Walton scale | 2.9 (0.6) | – | – |
Use of walking aids for complex daily life activities (n) | 5 | – | – |
Time since diagnosis, years, means (SD) | 15.0 (9.2) | – | – |
Treatment by ERT, n | 15/18 | – | – |
Time since ERT start, years, means (SD) | 7.3 (5.2) | – | – |
Use of non-invasive ventilation n | 5/20 | – | – |
Physiotherapy (n) | 16/18 | – | – |
Number of fallers in the last 3 months | 10 | – | – |
Average number of falls per faller | 1.4 | – | – |
% falls during locomotor activities | 89.3% | – | – |
SD: Standard deviation.
The results for muscle strength are presented in Table 2 and in Fig. 1. The MVT values for the hip, knee and ankle were all significantly lower in the LOPD group than in the control group. Furthermore, the deficits were homogeneous with proximal-distal involvement of muscle groups. The correlation matrix for the impaired muscle groups is provided in the supplementary document.
Table 2
Muscle strength measurement results
LOPD group (n = 18) | Control group (n = 20) | p-value | |
mean (SD) | mean (SD) | ||
Isokinetic dynamometer | |||
Maximum strength of hip flexors (Nm) | 61.5 (47.5) | 122.2 (51.5) | <.001* |
Maximum strength of hip extensors (Nm) | 47.6 (40.6) | 119.7 (54.8) | <.001* |
Maximum strength of hip abductors (Nm) | 45.3 (40.6) | 99.6 (29.8) | <.001* |
Maximum strength of hip adductors (Nm) | 37.3 (27.5) | 77.5 (32.9) | <.001* |
Maximum strength of knee flexors (Nm) | 36.3 (27.6) | 64.2 (27.7) | 0.001* |
Maximum strength of knee extensors (Nm) | 87.7 (62.7) | 172.5 (78.3) | <.001* |
Maximum strength of plantar flexors (Nm) | 104.6 (42.9) | 146.3 (50.4) | 0.009* |
Maximum strength of dorsi flexors (Nm) | 27.1 (12.0) | 37.9 (13.0) | 0.015* |
SD: Standard deviation. Maximum strength evaluated by isokinetic dynamometer. *Significant difference between LOPD and control groups, p <0.05 with Mann-Whitney U test.
Fig. 1
Percentage of the remaining strength of the LOPD group compared to the strength of the control group (color figure for the online PDF).
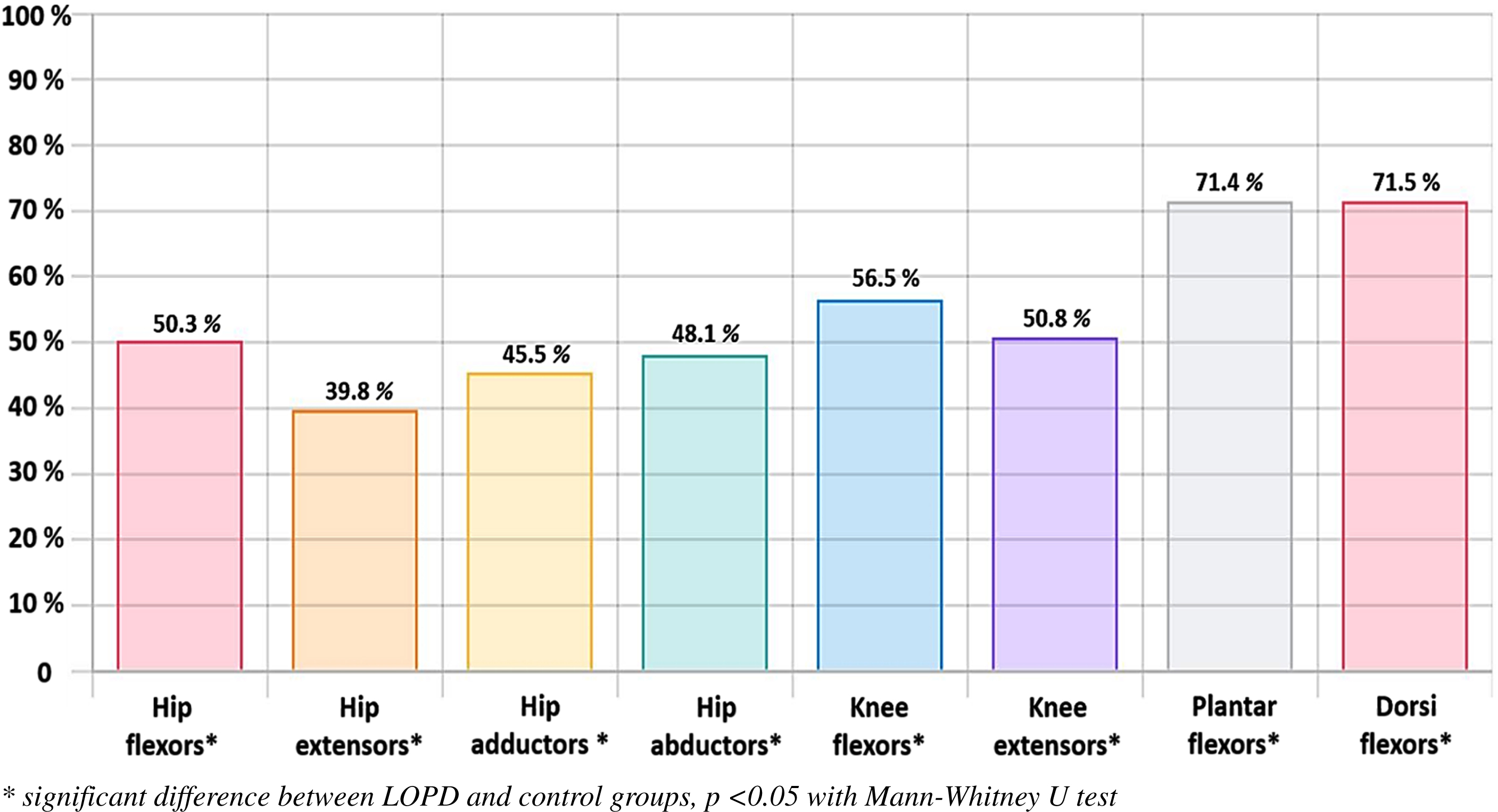
Locomotor performance in adults with LOPD
Distance walked on the 6MWT was significantly shorter (380.3 vs. 544.1 m, p <0.001, α=0.72) gait speed on the 10 m walk test was significantly slower (0.87 vs. 1.24 m/s, p=0.002, α=0.61) and TUG performance time was significantly longer (10.0 vs. 5.4 s, p <0.001, α=0.78) in the LOPD than the control group (Table 3 and Fig. 2).
Table 3
Clinical locomotor variables and spatiotemporal gait variables
Intergroup mean difference | Intergroup SD difference | |||||
LOPD (n=18) | Control (n=20) | p-value | α | p-value SD | α SD | |
mean (SD) | mean (SD) | |||||
TUG (s) | 10.0 (3.5) | 5.4 (0.6) | <.001* | 0.78 | – | – |
6MWT (m) | 380.3 (116.6) | 544.1 (112.9) | <.001* | 0.72 | – | – |
Spontaneous walking | 0.87 (0.4) | 1.24 (0.13) | 0.002* | 0.61 | 0.552 | 0.12 |
speed over10-m (m/s) | ||||||
Cadence (step/min) | 96.4 (20.1) | 113.8 (8.7) | 0.001* | 0.62 | 0.004* | 0.57 |
Step length (m) | 0.53 (0.2) | 0.65 (0.07) | 0.017* | 0.46 | 0.002* | 0.61 |
Stride width (m) | 0.21 (0.04) | 0.16 (0.03) | 0.70 | 0.08 | 0.074 | 0.35 |
% SSP | 35.1 (7.5) | 40.6 (1.5) | 0.003* | 0.63 | <.001* | 0.63 |
% DSP | 29.5 (7.5) | 18.1 (1.5) | 0.002* | 0.56 | 0.011* | 0.49 |
*Significant difference between LOPD and control groups, p <0.05% SSP: Percentage of Single Support Phase during the gait cycle. % DSP: Percentage of Double Support Phase during the gait cycle. Owing to the small number of trials performed for the TUG (3 tests) and 6MWT (1 test), we did not analyze the variability of these results.
Fig. 2
Percentage of locomotor variable values of the LOPD group compared to the control group.
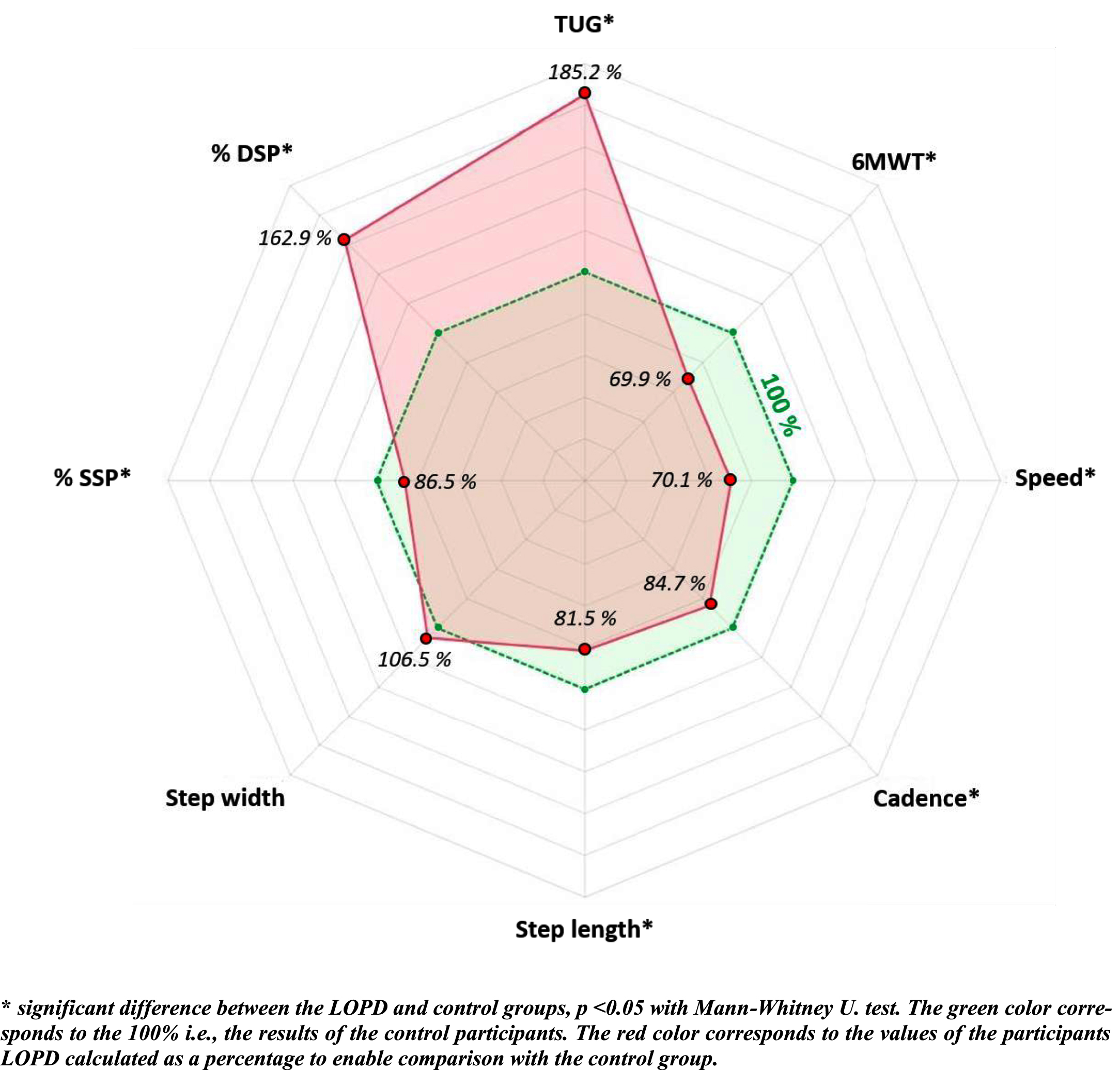
Muscular determinants
Table 4 shows the input variables and results for locomotor performance for the forward stepwise multiple regression.
Table 4
Muscular determinants of locomotor performance. The forward stepwise multiple regression results
Performance variable | Assessment | Input variables* | Regression results |
Muscular determinants of gait speed over 10 m | Isokinetic dynamometer | - Hip flexors (ρ=0.61, p=0.01)- Hip abductors (ρ=0.81, p <0.001)- Knee flexors (ρ=0.57, p=0.019)- Plantar flexors (ρ=0.65, p=0.006)- Dorsal flexors (ρ=0.66, p=0.005 | Strength assessed by dynamometer1. Hip abductors (p <0.001; adjusted R2=0.66),2. Hip abductors + hip flexors + ankle plantar flexors (p <0.001; adjusted R2=0.89) |
Muscular determinants of % predicted distance on 6MWT | Isokinetic dynamometer | - Hip abductors (ρ=0.54, p=0.02)- Plantar flexors (ρ=0.51, p=0.03) | Strength assessed by dynamometer1. Hip abductors (p <0.001; adjusted R2=0.41),2. Hip abductors + ankle plantar flexors (p <0.003; adjusted R2=0.53) |
Muscular determinants Timed Up and Go performance time | Isokinetic dynamometer | - Hip flexors (ρ=-0.53, p=0.03)- Hip abductors (ρ=-0.72, p=0.003)- Knee flexors (ρ=-0.56, p=0.02)- Knee extensors (ρ=-0.57, p=0.02)- Plantar flexors (ρ=-0.66, p=0.001)- Dorsal flexors (ρ=-0.51, p=0.04) | Strength assessed by dynamometer1. Hip abductors (p <0.001; adjusted R2=0.56)2. Hip abductors + ankle plantar flexors (p <0.001; adjusted R2=0.81) |
% : percentage. * Threshold of probability of entry defined at p≥0.05.
Hip abductor MVT explained 66% of gait speed variance (p <0.001); the variance explained increased to 89% (p <0.001; adjusted R2=0.89) with the addition of hip flexor and ankle plantar flexor MVT.
Hip abductor MVT explained 41% of walking distance variance (p <0.001); the variance explained increased to 53% (p <0.001) with the addition of ankle plantar flexor MVT.
Hip abductor strength MVT explained 56% of TUG performance time variance (p <0.001); the variance explained increased to 81% (p <0.001) with the addition of ankle plantar flexor MVT.
Falls and Gait Stability in Adults With LOPD
In total, 10/18 of the LOPD group reported falls in the last 3 months, with an average of 1.4 falls per faller. More than 89% of falls occurred during a locomotor task (Table 1). Most spatiotemporal gait parameters differed significantly between the LOPD and control groups, except for step width (Table 3 and Fig. 2). The variability of the spatiotemporal gait parameters variability was significantly greater in the LOPD than the control group, particularly the % SSP, except for variability in speed and step width, which was not different between groups (Table 3).
Biomechanical and muscular determinants
Table 5 shows the forward stepwise multiple regression results for gait stability determinants and the input variables.
Table 5
Muscular and gait variable determinants of stability. The forward stepwise multiple regression results
Stability variable | Assessment | Input variables* | Regression results |
Spatio-temporal gait parameters (mean, variability) determinants of falls | 3D gait analysis | - Speed (ρ=-0.78, p <0.01)- Cadence (ρ=-0.50, p =0.005)- % SSPmean (ρ=-0.73, p=0.005)- % DSP (ρ=0.65, p=0.006)- % SSPSD (ρ=-0.67, p=0.005) | 1. Speed (p <0.001; adjusted R2=0.61),2. Speed + % SSPmean (p <0.001; adjusted R2=0.72)3. Speed + % SSPmean + % SSPSD (p <0.001; adjusted R2=0.78) |
Muscle determinants of % SSPmean | Isokinetic dynamometer | - Hip abductors (ρ=-0.72, p=0.003)- Plantar flexors (ρ=-0.66, p=0.001) | Strength (dynamometer)1. Hip abductors (p <0.001; adjusted R2=0.54) |
Muscle determinants of % SSPSD | Isokinetic dynamometer | - Hip abductors (ρ=0.70, p=0.003)- Knee extensors (ρ=0.52, p=0.001) | Strength (dynamometer)1. Hip abductors (p <0.006; adjusted R2=0.37) |
% SSP: percentage of single support phase of gait. % DSP: percentage of double support phase during gait cycle. * Threshold of probability of entry defined at p≥0.05.
Gait speed explained 61% of the number of falls variance (p <0.001); the variance explained increased to 72% (p <0.001) with the addition of the mean value of % SSP and to 78% (p <0.001) with the addition of the % SSP variability.
The hip abductor MVT was the only muscular determinant of the mean value of % SSP and % SSP variability and explained respectively 54% and 37% of their variance (p <0.001).
Gait pattern in adults with LOPD
Table 6 shows the significant differences in the joint kinematic variables between the adults with LOPD and controls. In summary:
Table 6
Kinematic joint variables and ground reaction force during gait
LOPD Group (N=18) | Control Group (N=20) | p-value | α | |
mean (SD) | mean (SD) | |||
PELVIS | ||||
Mean pelvic tilt (°) | 4.0 (6.8) | 9.5 (5.4) | 0.03* | 0.41 |
Pelvic tilt ROM (°) | 4.8 (1.8) | 2.9 (0.9) | 0.001* | 0.63 |
Pelvic contralateral drop (°) | 17.1 (5.3) | 11.1 (3.9) | 0.001* | 0.69 |
Pelvic rotation ROM | 11.4 (5.8) | 9.9 (3.5) | 0.5 | 0.13 |
HIP | ||||
Max hip extension (°) | 10.4 (9.7) | 11.7 (7.3) | 0.68 | 0.18 |
Max hip flexion (°) | 31.5 (5.5) | 33.7 (5.2) | 0.21 | 0.25 |
Hip sagittal ROM (°) | 45.3 (5.6) | 44.2 (4.5) | 0.71 | 0.79 |
Max hip adduction (support) (°) | 13.8 (4.2) | 11.7 (4.2) | 0.19 | 0.26 |
Max hip abduction (swing) (°) | 18.7 (5.3) | 12.6 (4.0) | <.001* | 0.66 |
Hip frontal ROM (°) | 32.5 (8.1) | 24.3 (7.1) | 0.001* | 0.61 |
Max medial rotation (°) | -1.1 (9.2) | -2.1 (6.8) | 0.79 | 0.07 |
Max lateral rotation (°) | 22.2 (11.1) | 17.8 (5.5) | 0.14 | 0.29 |
Hip rotation ROM (°) | 21.1 (7.2) | 15.7 (5.2) | 0.009* | 0.5 |
KNEE | ||||
Max extension (°) | -2.7 (8.2) | 0.11 (4.5) | 0.09 | 0.33 |
Max flexion (°) | 65.8 (4.4) | 61.9 (3.5) | 0.002* | 0.57 |
Ini. contact mean position (°) | 10.9 (7.7) | 0.39 (2.9) | <.001* | 0.82 |
Knee sagittal ROM (°) | 63.2 (7.7) | 66.6 (3.2) | 0.039* | 0.40 |
ANKLE | ||||
Max dorsiflexion (support) (°) | 13.8 (3.4) | 12.5 (2.3) | 0.09 | 0.37 |
Max dorsiflexion (swing) (°) | 11.6 (5.3) | 10.2 (2.4) | 0.12 | 0.30 |
Max plantar flexion (°) | 13.2 (7.2) | 16.7 (4.4) | 0.09 | 0.032 |
GROUND REACTION FORCES | ||||
Vertical GRF (N/BW) | 1.0 (0.1) | 1.2 (0.1) | 0.07 | 0.34 |
Breaking GRF (N/BW) | 0.1 (0.1) | 0.2 (0.1) | 0.006* | 0.52 |
Propulsion GRF (N/BW) | 0.1 (0.1) | 0.2 (0.1) | 0.003* | 0.55 |
*Significant difference (p <0.05) for intergroup comparison using Mann-Whitney U. test. Max: Maximum; Ini: initial; ROM: Range Of Motion; BW: Body Weight; GRF: ground reaction force. Negative values indicate an amplitude deficit in the given plane.
• pelvis: posterior tilt, sagittal pelvic ROM and contralateral pelvic drop were significantly greater in the LOPD than the control group.
• Hip: peak abduction during swing, and frontal and rotation ROM were significantly greater in the LOPD group.
• Knee: peak flexion during swing was higher, sagittal ROM was lower and knee flexion at foot strike was greater in the LOPD group.
• Propulsion and breaking forces were lower in the LOPD group.
The forward stepwise multiple regression results for the joint kinematics and the input variables are presented in Supplementary Table 1.
Muscular determinants of pelvic kinematic variables
The mean pelvic tilt position and the pelvic sagittal ROM were not correlated with the strength of any muscle group. Hip abductor MVT explained 75% of contralateral pelvic drop ROM variance (p <0.001); the variance explained increased to 85% (p <0.001) with the addition of ankle plantar flexor MVT.
Muscular determinants of Hip kinematic variables
Hip flexor MVT explained 51% of peak abduction during swing variance (p <0.001). Hip flexor MVT explained 46% of hip frontal ROM variance (p=0.003). The hip rotation ROM was not correlated with any muscle group.
Muscular determinants of Knee kinematic variables
The maximum peak flexion and the knee sagittal ROM were not correlated with any muscle group. Hip flexor MVT explained 38% of mean knee position at foot strike variance (p=0.007); the variance explained increased to 50% (p=0.003) with the addition of hip abductor MVT.
DISCUSSION
Our study revealed that hip abductor strength was the main muscular determinant of performance for all locomotor performances evaluated (10 m walking speed at comfortable pace, 6MWT distance and TUG time). Hip abductor strength also had a major impact on gait stability (mean value and % SSP variability). Besides muscle determinants, % SSP was a key determinant of the number of falls in adults with LOPD. Therefore, these variables can be considered as new key clinical biomarkers of locomotor performance and gait stability in LOPD. Furthermore, our study presents the locomotor performance, gait stability and gait pattern perturbations in adults with LOPD. Figure 3 presents these results and their clinical implications.
Fig. 3
Alterations, main determinants and recommendations for management in adults with LOPD.
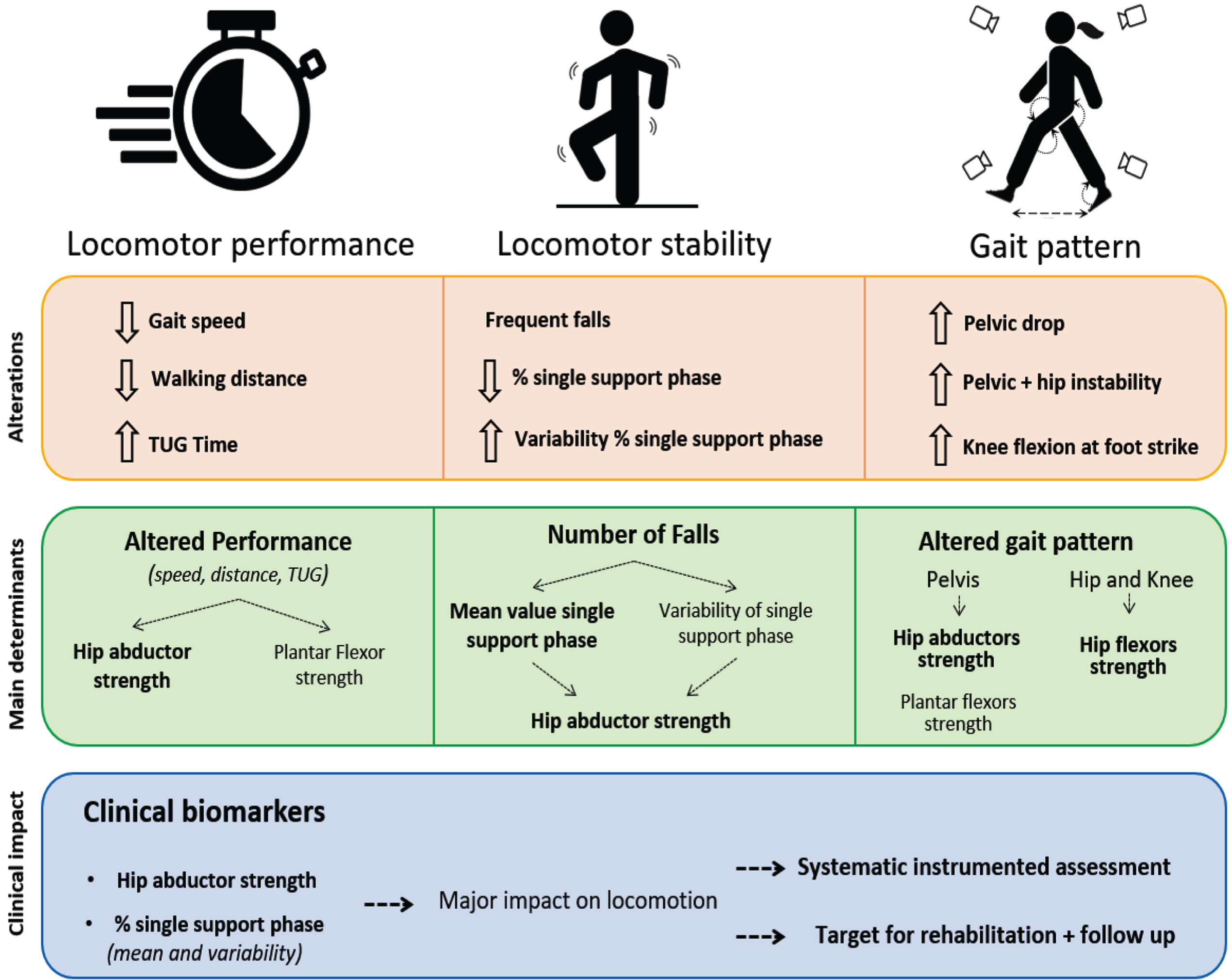
Muscular biomarkers of locomotor performance and gait stability
Our results confirmed that locomotor performance was impaired in adults with LOPD compared to controls, with a significant reduction in comfortable gait speed (-28%) and 6MWT distance (-31%) and an increase in TUG performance time (+85%) [4, 5, 9]. The TUG was the most affected task. This may be explained by the greater complexity of the sub-tasks of this test (half-turns and sit-to-stand) that require more strength and medio-lateral stabilization than straight-line walking [24].
Some studies have suggested a relationship between locomotor performance and muscle weakness in adults with LOPD [5, 7, 8, 11]; however, this had not been objectively tested. We found that hip abductor strength assessed with the isokinetic dynamometer was the main determinant of gait speed, walking distance and TUG time. This finding was surprising since hip flexor, knee extensor, and ankle plantar flexor strength have been shown to determine gait performance in other pathologies with muscle weakness [30–32]. Only Favejee et al. previously studied the muscular determinants of walking ability in adults with LOPD. They found that hip abductor and flexor strength were related to a walking ability score. They also showed that the combination of hip abductor and knee extensor weakness, sex, age and ventilation use was the best predictor of walking ability on that scale [11]. The hip abductors and flexors are among the weakest muscles in adults with LOPD (respectively -55% and -50% compared to controls). Our results extend those of Favejee et al., showing that hip abductor strength is a stronger determinant of locomotor task performance (and not only walking ability) than hip flexor strength. Interestingly, although the TUG test involves different sub-tasks compared to the other locomotor tests, the same muscle group, the hip abductors, was the primary determinant. Ankle plantar flexor strength was complementary to hip abductor strength for determining locomotor performance. Since the hip abductors are known to be the most affected and ankle plantar flexors the lease affected muscles, these results suggest a proximal-distal compensatory strategy, as found in individuals after stroke [33]. Therefore, it is essential to also assess the strength of these distal muscles because the ability to compensate is essential for gait in this degenerative disease.
Previous studies of the muscular determinants of locomotor performance in other pathologies highlighted the major role of the knee extensors, ankle plantar flexors and knee flexors, which differs from our findings in LOPD. However, the role of the hip muscles was insufficiently explored in those studies.
Our study also highlighted alterations in gait stability in the participants with LOPD. Indeed, the shorter time spent in the single support phase (mean % SSP -24%) in the LOPD group suggests the adoption of a cautious gait often observed in other faller groups [20, 27]. Additionally, the greater within-participant variability of the single support phase duration (+31% for SD of % SSP) suggests that participants with LOPD had difficulty controlling their stability during gait [22, 23]. Hip abductor weakness emerged as the primary determinant of % SSP, which is representative of gait stability.
Therefore, hip abductor weakness appears to be the main determinant of impaired gait stability and impaired locomotor performance in LOPD. These 2 results could be related since the key function of the hip abductors is to control the pelvis and the displacements of the center of mass in the frontal plane, especially during the single support phase [34]. We could hypothesize that to minimize instability mainly caused by hip abductor weakness, adults with LOPD reduce the time spent in the single support phase and increase the time in double support phase (as highlighted by our results), consequently reducing their locomotor performance (gait speed).
Abductor strength should therefore be considered as a clinical biomarker of both locomotor performance and gait stability in adults with LOPD and should be routinely assessed. A strengthening program for the hip abductors would be relevant to improve locomotor performance and reduce gait instability and falls in adults with LOPD; studies have shown positive effects of strengthening in LOPD [35–37].
Concerning assessment, an accurate and reliable assessment of the hip muscle groups (particularly the abductors, followed by the flexors) in adults with LOPD is essential. Ideally, this should be performed using an isokinetic dynamometer, the recommended gold standard, but hand-held dynamometers or strain gauges, which are more accessible, can be used as long as the method is standardized using rigid planes or straps [38, 39].
Biomechanical gait biomarkers of gait stability
Impaired stability was characterized by a high prevalence of falls: more than 50% of participants had fallen during the 3 months preceding the study. Eighty-nine percent of these falls were related to locomotor tasks which, along with the changes in the single support phase of the gait cycle, confirms the gait instability in LOPD. A decreased gait speed and a shorter and more variable duration of single support phase were the major determinants of falls.
The mean % SSP value was a stronger determinant of the number of falls than % SSP variability. These results contrast with those of some studies on falls in the elderly, which have shown that variability of spatiotemporal gait parameters is more closely related to fall risk than mean values [26, 40, 41]. Given these new results in adults with LOPD, the mean value and the variability of the % SSP should be systematically evaluated in longitudinal studies. However, to date, over 84% of studies of locomotion in adults with LOPD only used the 6MWT [4]. This is the case for the 2 large recent clinical trials evaluating new medical treatments for LOPD: the COMET [42] and PROPEL [43] trials. Performance on the 6MWT is influenced by cardiorespiratory capacity and therefore could lack sensitivity to change; this could explain the lack of change found by interventional studies [44].
With regards to assessment, we recommend assessing not only performance but also spatiotemporal gait parameters in adults with LOPD, especially the single support phase. The use of instrumented tools such as 3D gait analysis, walkways (such as the Gaitrite or Zeno) or inertial measurement units (with reliable measures) would allow an accurate evaluation of these variables for further studies on promising new therapies [10, 45].
Specific gait kinematics
Our spatiotemporal gait parameters confirm previous findings, including decreased speed, cadence, step length and percentage of single support in adults with LOPD compared to healthy controls [7, 10, 12]. Nevertheless, the non-significant difference in step width between adults with LOPD and healthy controls contrasts with the results of the only study that statistically compared this outcome between these groups [7]. Their sample was younger than ours, and age is known to influence step width [46], which could explain the differences between our results.
The gait pattern in LOPD is classically described as waddling (with a positive Trendelenburg sign) [4, 5, 7], which is related to pelvic instability. However, the only study that evaluated pelvic kinematics found no pelvic drop compared to control participants [7]. The authors explained this result by the great heterogeneity between participants. Our results showed a greater contralateral pelvic drop in the LOPD than the control group.
The magnitude of the pelvic drop was primarily determined by the strength of the hip abductors. The abductors play a major role in controlling the contralateral pelvic drop [47–50]. This lack of control is known as Trendelenburg gait and symbolizes the typical waddling gait in LOPD.
Adults with LOPD are often described as hyperlordotic; however, our results showed significantly more posterior pelvic tilt during gait in the LOPD than the control group, which was not related to muscle weakness. Besides, sagittal pelvic ROM was greater in the LOPD group, likely because of trunk extensor weakness. Weakness, compensatory strategies and pain could explain the increased posterior pelvic tilt [5].
These findings were complemented by an increase in peak abduction in the swing phase and in hip ROM in the sagittal and transverse planes in the LOPD group. More surprisingly, the knee was more flexed (+10 °) at foot strike in the LOPD than the control group. This finding was related to hip flexor and abductor weakness. It could also be explained by the posterior pelvic tilt and insufficient propulsion without hamstring shortening. There was also an increase in knee flexion in the swing phase, which could be a compensation for the contralateral pelvic drop. These kinematic hip and knee alterations have not been previously demonstrated in LOPD. Proximal weakness with distal compensation could cause fatigue during gait and restrict locomotor activities in the community.
Hip flexor strength was the major determinant of kinematic changes at the hip and knee. Although they are minor determinants of locomotor performance and gait stability, the hip flexors play a major role in the altered gait pattern in LOPD. This highlights the importance of targeting not only the abductors but also the hip flexors in the assessment and rehabilitation of adults with LOPD.
Limitations and perspectives
The small sample size of this study, owing to the low prevalence of adults with LOPD, could appear as a limitation. However, 9.1% of the French adult population with LOPD were included in our study, and the large effect sizes of the different variables measured ensuring confidence in our results. The sample could be considered heterogeneous since 3 of the 18 participants were not on ERT treatment. However, analyses performed without these 3 subjects (data not shown) did not alter the results, and the duration of the treatment was not correlated with locomotor parameters or the number of falls.
Future longitudinal follow-up studies and studies on the new therapies should investigate changes in locomotor performance, as well as gait stability and hip abductor strength, which have a major impact on these impairments. Given the lack of comparisons with other neuromuscular diseases, it is difficult to determine if these muscle determinants are specific to LOPD. This should be determined in further studies.
CONCLUSION
This study is the first to identify determinants of locomotor performance, gait stability and the gait pattern in LOPD using gold standard assessment tools (3D analysis for the gait and isokinetic dynamometer for muscle strength). Furthermore, we confirmed the alterations in locomotor performance and the gait pattern and revealed alterations in gait stability in adults with LOPD as compared with healthy controls. Ours study has also enabled us to identify new clinical biomarkers of locomotion in adults with LOPD. Hip abductor weakness was the main determinant of locomotor performance, gait stability and pelvic instability, and hip flexor weakness was the main determinant of abnormal hip and knee kinematics. The percentage duration of the single support phase of gait (the mean value and the variability) was the main determinant of gait stability.
The identification of these essential new clinical parameters leads us to recommend the systematic assessment of hip abductor strength and single support phase duration during gait in adults with LOPD using instrumented tools. These results also encourage the implementation of appropriate rehabilitation strategies, such as hip abductor strengthening and dynamic single-leg balance training in adults with LOPD. Self-rehabilitation could be a useful adjunct to the therapy sessions. Future longitudinal studies should determine how the locomotor alterations reported in the present study change over time (especially the percentage of single support during the gait cycle). Studies should also determine sensitivity to change in hip abductor strength, which have a significant impact on locomotor alterations in adults with LOPD.
ACRONYM LIST
• LOPD: Late Onset Pompe Disease
• MMT: Manual Muscle Testing
• % SSP: Percentage duration of the single support phase during the gait cycle
• 3D: Three-dimensional
• ROM: Range of motion
• 6MWT: Six-minute walk test
• TUG test: Timed Up and Go test
• SD: Standard Deviation
• MVT: Maximal Voluntary Torque
ACKNOWLEDGMENTS
We would like to thank all the participants included in this study and the ARS Ile de France: call for projects “support towards the emergence of teacher-researchers” 2018, Fonds d’Intervention Regional N°C 2018DOSRHS001. We thank the French National Research Agency (ANR) for supporting our project, which was led by Helge Amthor, and Nawal Derridj for coordinating with the Direction of Clinical Research, Innovation and Relations with Universities and Research Organizations (DRCI). We also thank Johanna Robertson for copyediting and constructive criticism.
FUNDING
This study was supported by 1) ARS Ile-de-France: call for projects “support for the emergence of lecturer-researchers” 2018, Regional Intervention Fund N°C 2018DOSRHS001 and 2) French National Research Agency (ANR) Grant N° PRTS15009. These supports partly financed a PhD.
CONFLICT OF INTEREST
The authors have no conflict of interest to report.
SUPPLEMENTARY MATERIAL
[1] The supplementary material is available in the electronic version of this article: https://dx.doi.org/10.3233/JND-230060.
REFERENCES
[1] | Van Der Ploeg , Reuser AJJ . Pompe’s disease. Lancet. (2008) ;372: :1342–53. |
[2] | Figueroa-Bonaparte S , Segovia S , Llauger J , et al. Muscle MRI Findings in Childhood/Adult Onset Pompe Disease Correlate with Muscle Function. PLoS ONE. (2016) ;11: :e0163493. |
[3] | Van der Beek , de Vries JM , Hagemans MLC , et al. Clinical features and predictors for disease natural progression in adults with Pompe disease: A nationwide prospective observational study. Orphanet J Rare Dis. (2012) ;7: :88. |
[4] | Maulet T , Bonnyaud C , Weill C , et al. Motor Function Characteristics of Adults With Late-Onset Pompe Disease: A Systematic Scoping Review. Neurology. (2023) ;100: :e72–e83. |
[5] | Chan J , Desai AK , Kazi ZB , et al. The emerging phenotype of late-onset Pompe disease: A systematic literature review. Mol Genet Metab. (2017) ;120: :163–72. |
[6] | Van Emmerik REA , Ducharme SW , Amado AC , et al. Comparing dynamical systems concepts and techniques for biomechanical analysis. Journal of Sport and Health Science. (2016) ;5: :3–13. |
[7] | Starbuck C , Reay J , Silk E , et al. Are there common walking gait characteristics in patients diagnosed with late-onset Pompe disease? Human Movement Science. (2021) ;77: :102777. |
[8] | Hagemans MLC , Winkel LPF , Van Doorn PA , et al. Clinical manifestation and natural course of late-onset Pompe’s disease in 54 Dutch patients. Brain. (2005) ;128: :671–7. |
[9] | Laforêt P , Laloui K , Granger B , et al. The French Pompe registry. Baseline characteristics of a cohort of 126 patients with adult Pompe disease. Rev Neurol (Paris). (2013) ;169: :595–602. |
[10] | McIntosh PT , Case LE , Chan JM , et al. Characterization of gait in late onset Pompe disease. Molecular Genetics and Metabolism. (2015) ;116: :152–6. |
[11] | Favejee MM , van der Meijden JC , Kruijshaar ME , et al. Association of Muscle Strength and Walking Performance in Adult Patients With Pompe Disease. Phys Ther. (2018) ;98: :925–31. |
[12] | Schneider I , Zierz S , Schulze S , et al. Characterization of Gait and Postural Regulation in Late-Onset Pompe Disease. Applied Sciences. (2020) ;10: :7001. |
[13] | Tiffreau V , Ledoux I , Eymard B , et al. Isokinetic muscle testing for weak patients suffering from neuromuscular disorders: A reliability study. Neuromuscul Disord. (2007) ;17: :524–31. |
[14] | Van der Beek , Hagemans MLC , Reuser AJJ , et al. Rate of disease progression during long-term follow-up of patients with late-onset Pompe disease. Neuromuscul Disord. (2009) ;19: :113–7. |
[15] | Andreassen , Schlütter JM , Vissing J , et al. Effect of enzyme replacement therapy on isokinetic strength for all major muscle groups in four patients with Pompe disease-a long-term follow-up. Mol Genet Metab. (2014) ;112: :40–3. |
[16] | Chamorro C , Armijo-Olivo S , De la Fuente C , et al. Absolute Reliability and Concurrent Validity of Hand Held Dynamometry and Isokinetic Dynamometry in the Hip, Knee and Ankle Joint: Systematic Review and Meta-analysis. Open Med (Wars). (2017) ;12: :359–75. |
[17] | Duclos C , Desjardins P , Nadeau S , et al. Destabilizing and stabilizing forces to assess equilibrium during everyday activities. Journal of Biomechanics. (2009) ;42: :379–82. |
[18] | Leboeuf F , Baker R , Barré A , et al. The conventional gait model, an open-source implementation that reproduces the past but prepares for the future. Gait Posture. (2019) ;69: :235–41. |
[19] | Hamacher D , Singh NB , Van Dieën JH , et al. Kinematic measures for assessing gait stability in elderly individuals: A systematic review. J R Soc Interface. (2011) ;8: :1682–98. |
[20] | Sudarsky L , Ronthal M . Gait disorders in the elderly: Assessing the risk for falls. In: Falls, balance and gait disorders in the elderly. (1992) , pp. 117–27. |
[21] | Lemay J-F , Duclos C , Nadeau S , et al. Postural and dynamic balance while walking in adults with incomplete spinal cord injury. J Electromyogr Kinesiol. (2014) ;24: :739–46. |
[22] | Balasubramanian CK , Neptune RR , Kautz SA . Variability in spatiotemporal step characteristics and its relationship to walking performance post-stroke. Gait Posture. (2009) ;29: :408–14. |
[23] | Brach JS , Studenski SA , Perera S , et al. Gait variability and the risk of incident mobility disability in community-dwelling older adults. J Gerontol A Biol Sci Med Sci. (2007) ;62: :983–8. |
[24] | Bonnyaud C , Pradon D , Bensmail D , et al. Dynamic Stability and Risk of Tripping during the Timed Up and Go Test in Hemiparetic and Healthy Subjects. PLoS One. (2015) ;10: :e0140317. |
[25] | Bruijn SM , Meijer OG , Beek PJ , et al. Assessing the stability of human locomotion: A review of current measures. Journal of The Royal Society Interface. (2013) ;10: :20120999. |
[26] | Hausdorff JM , Rios DA , Edelberg HK . Gait variability and fall risk in community-living older adults: A 1-year prospective study. Arch Phys Med Rehabil. (2001) ;82: :1050–6. |
[27] | Woollacott MH , Tang PF . Balance control during walking in the older adult: Research and its implications. Phys Ther. (1997) ;77: :646–60. |
[28] | Van der Woude DR , Ruyten T , Bartels B . Reliability of Muscle Strength and Muscle Power Assessments Using Isokinetic Dynamometry in Neuromuscular Diseases: A Systematic Review. Phys Ther. (2022) ;102: :pzac099. |
[29] | Silva SB , de Abreu LC , Valenti VE , et al. Verbal and visual stimulation effects on rectus femoris and biceps femoris muscles during isometric and concentric. Int Arch Med. (2013) ;6: :38. |
[30] | Suzuki K , Imada G , Iwaya T , et al. Determinants and predictors of the maximum walking speed during computer-assisted gait training in hemiparetic stroke patients. Archives of Physical Medicine and Rehabilitation. (1999) ;80: :179–82. |
[31] | Patterson SL , Forrester LW , Rodgers MM , et al. Determinants of walking function after stroke: Differences by deficit severity. Arch Phys Med Rehabil. (2007) ;88: :115–9. |
[32] | Van der Krogt MM , Delp SL , Schwartz MH . How robust is human gait to muscle weakness? Gait Posture. (2012) ;36: :113–9. |
[33] | Roche N , Bonnyaud C , Geiger M , et al. Relationship between hip flexion and ankle dorsiflexion during swing phase in chronic stroke patients. Clinical Biomechanics. (2015) ;30: :219–25. |
[34] | Neptune RR , McGowan CP . Muscle Contributions to Frontal Plane Angular Momentum during Walking. J Biomech. (2016) ;49: :2975–81. |
[35] | Bhatnagar C , Shah J , Ramani B , et al. Safety and effectiveness of resistance training in patients with late onset Pompe disease – a pilot study. Neuromuscul Disord. (2022) ;32: :284–94. |
[36] | Angelini C . Exercise, nutrition and enzyme replacement therapy are efficacious in adult Pompe patients: Report from EPOC Consortium. Eur J Transl Myol. (2021) ;31: :9798. |
[37] | Van Den Berg , Favejee MM , Wens SCA , et al. Safety and efficacy of exercise training in adults with Pompe disease: Evalution of endurance, muscle strength and core stability before and after a 12 week training program. Orphanet J Rare Dis; 10. Epub ahead of print 2015. DOI: 10.1186/s13023-015-0303-0 |
[38] | Morin M , Hébert LJ , Perron M , et al. Psychometric properties of a standardized protocol of muscle strength assessment by hand-held dynamometry in healthy adults: A reliability study. BMC Musculoskelet Disord. (2023) ;24: :294. |
[39] | Hogrel J-Y , Payan CA , Ollivier G , et al. Development of a French Isometric Strength Normative Database for Adults Using Quantitative Muscle Testing. Archives of Physical Medicine and Rehabilitation. (2007) ;88: :1289–97. |
[40] | Hausdorff JM , Nelson ME , Kaliton D , et al. Etiology and modification of gait instability in older adults: A randomized controlled trial of exercise. J Appl Physiol. (2001) ;90: :2117–29. |
[41] | Maki BE . Gait changes in older adults: Predictors of falls or indicators of fear. J Am Geriatr Soc. (1997) ;45: :313–20. |
[42] | Diaz-Manera J , Kishnani PS , Kushlaf H , et al. Safety and efficacy of avalglucosidase alfa versus alglucosidase alfa in patients with late-onset Pompe disease (COMET): A phase 3, randomised, multicentre trial. The Lancet Neurology. (2021) ;20: :1012–26. |
[43] | Schoser , Roberts M , Byrne BJ , et al. Safety and efficacy of cipaglucosidase alfa plus miglustat versus alglucosidase alfa plus placebo in late-onset Pompe disease (PROPEL): An international, randomised, double-blind, parallel-group, phase 3 trial. The Lancet Neurology. (2021) ;20: :1027–37. |
[44] | Tard C , Bayot M , Davion J-B , et al. Factors impacting performance on the 6-minute walk test by people with late-onset Pompe disease. Muscle & Nerve. (2022) ;65: :693–7. |
[45] | Bachasson , Moraux A , Ollivier G , et al. Relationship between muscle impairments, postural stability, and gait parameters assessed with lower-trunk accelerometry in myotonic dystrophy type 1. Neuromuscul Disord. (2016) ;26: :428–35. |
[46] | Laurentius T , Quandel J , Bollheimer LC , et al. Spatiotemporal gait parameters in young individuals wearing an age simulation suit compared to healthy older individuals. Eur Rev Aging Phys Act. (2022) ;19: :29. |
[47] | Lee M , Youm C , Noh B , et al. Gait Characteristics under Imposed Challenge Speed Conditions in Patients with Parkinson’s Disease During Overground Walking. Sensors (Basel). (2020) ;20: :2132. |
[48] | Mikolajczyk T , Mikołajewska E , Al-Shuka HFN , et al. Recent Advances in Bipedal Walking Robots: Review of Gait, Drive, Sensors and Control Systems. Sensors (Basel). (2022) ;22: :4440. |
[49] | Nott CR , Neptune RR , Kautz SA . Relationships between frontal-plane angular momentum and clinical balance measures during post-stroke hemiparetic walking. Gait Posture. (2014) ;39: :129–34. |
[50] | Plotnik M , Bartsch RP , Zeev A , et al. Effects of walking speed on asymmetry and bilateral coordination of gait. Gait & Posture. (2013) ;38: :864–9. |