Safety Monitoring of Gene Therapy for Spinal Muscular Atrophy with Onasemnogene Abeparvovec –A Single Centre Experience
Abstract
Background:
Recently gene therapy with onasemnogene abeparvovec has been approved for the treatment of spinal muscular atrophy (SMA). As the experience from clinical trials is limited, there are still uncertainties for which patient population the treatment can be considered safe and effective.
Methods:
We report our experience with eight consecutive patients with SMA who were treated with the standard dose of onasemnogene abeparvovec (1.1×1014 vg/kg) at the University Hospital Bonn, Germany. All patients received prophylactic immunosuppression with 1 mg/kg/d prednisolone for four weeks starting on the day before gene therapy.
Results:
We treated eight patients (4 male, 4 female, age range 10–37 months) with a body weight between 7.1 and 11.9 kg. All patients had 2 or 3 copies of the SMN2-gene and were previously treated with nusinersen. Following treatment with onasemnogene abeparvovec all patients showed a temporary increase of the body temperature and an increase of transaminase levels. In all but one patient it was necessary to increase or prolong the standard steroid dose to control the immune response. In one severe case, liver damage was associated with impaired liver function. This patient received a steroid pulse therapy for five days. Blood counts revealed asymptomatic thrombocytopenia (<150×109/L) in 6/8 patients and a significant increase of monocytes following gene therapy. Liver values and blood counts returned to almost normal levels during the post-treatment observation period. Troponin I increased above normal limit in 4/8 patients but was not associated with any abnormalities on cardiac evaluation.
Conclusions:
In a broader spectrum of patients, treatment with onasemnogene abeparvovec was associated with a higher rate of adverse events. In our cases it was possible to control the immune response by close monitoring and adaptation of the immunosuppressive regimen. Further research is needed to better understand the immune response following gene therapy and ideally to identify patients at risk for a more severe reaction.
INTRODUCTION
Spinal muscular atrophy (SMA) is a neurodegenerative disease, which primarily affects spinal motor neurons and leads to progressive muscle weakness. The spectrum of severity ranges from severe cases with onset during the first six months of (SMA type 1) to later onset during childhood or adolescence (SMA types 2–4) [1, 2]. Without treatment SMA type 1 is associated with death or the need for permanent ventilation within the first two years of life. SMA is caused by biallelic mutations of the survival motor neuron (SMN1) gene [3]. In humans there is a second, almost homologous gene called SMN2, which differs from SMN1 only by a few nucleotides. One of these nucleotide exchanges leads to aberrant splicing, so that SMN2 produces only about 10%of SMN protein compared to SMN1. The number of SMN2 copies varies between individuals and is therefore the main predictor for disease severity. Higher numbers of SMN2 copies are associated with milder phenotypes [4].
Over the past years, the availability of a growing number of drug treatments has significantly changed the course of the disease. Nusinersen (Spinraza®) and risdiplam (Evrysdi®) increase the SMN protein production through modifying the splicing of SMN2 [5]. Onasemnogene abeparvovec (Zolgensma®) is an adeno-associated viral (AAV9) vector-based gene therapy, which introduces a functional copy of the SMN1 gene into motor neurons by means of a single intravenous injection. Due to the broad clinical spectrum and the rarity of SMA, pivotal trials for these drugs often cover only a limited spectrum of age and disease severity.
Clinical trials for onasemnogene abeparvovec include only SMA type 1 patients up to the age of 8 months [6]. Nevertheless, the Food and Drug Administration (FDA) approved the treatment for all types of SMA up to the age of two years. The European Medicine Agency extended the label to all patients either showing a phenotype of SMA type 1 or having up to three SMN2 copies. This broad label has caused discussions about the safety and efficacy of onasemnogene abeparvovec in older and heavier patients [7]. One concern is related to the fact that the dose of onasemnogene abeparvovec is proportional to the body weight and that higher numbers of viral vectors might lead to more pronounced off-target effects. So far, main adverse events that have been observed in preclinical and clinical trials concern the liver, the heart, and the hematopoietic system.
In this case series we report preliminary safety findings from a series of eight consecutive patients treated at the University Hospital Bonn, Germany. All of them were older than eight months, thus outside the population that has been studied in clinical trials.
METHODS
This is a retrospective analysis of routine data collected from all patients treated with onasemnogene abeparvovec at the Department of Neuropediatrics, University Hospital Bonn, Germany, between December 2019 and September 2020. The data collection and analysis were approved by the institutional Ethics Committee (reference 405/20). In addition, all parents provided consent to participate in the SMArtCARE longitudinal data collection [8]. Investigations before and after gene therapy were performed as recommended by a recent German consensus paper [9]. After the infusion patients stayed in hospital for at least 48 hours. Follow-up visits were scheduled every week for at least four weeks, followed by biweekly intervals for two months, and monthly intervals for another three months. Additional visits were scheduled if needed based on clinical condition or laboratory abnormalities. All patients received the standard dose of 1.1×1014 vg/kg onasemnogene abeparvovec. Prednisolone treatment was started on the day before administration of gene therapy at a standard dose of 1 mg/kg body weight per day and continued for at least four weeks. Steroid dose was adapted according to clinical needs.
Laboratory tests and investigations
Complete blood count, kidney and liver function, creatine kinase and troponin I and T levels were measured at the timepoints listed above. Cardiac evaluation with echocardiography and electrocardiogram were performed before and every three months after treatment.
Functional assessments
For functional assessments, we followed the SMArtCARE recommendations (www.smartcare.de). Trained physiotherapists performed CHOP-INTEND, Hammersmith Functional Motor Scale Expanded, and Bayley-III tests as appropriate for age and functional status. Motor milestones were documented according to WHO definitions.
RESULTS
Patients
Eight patients (4 males, 4 females) with SMA due to homozygous deletions of SMN1 were treated with onasemnogene abeparvovec. At the time of treatment, the age ranged between 10 and 37 months, and the body weight between 7.1 and 11.9 kg. Before treatment AAV9 antibody titres were below 1:50 in all patients. All patients had previously been treated with nusinersen and switched to gene therapy with the hope to see additional benefit and to avoid repetitive lumbar punctures. Two patients started treatment with nusinersen in a pre-symptomatic stage. Additional four patients were able to sit before initiation of any disease modifying treatment (SMA type 2). None of them was able to walk before treatment with nusinersen (SMA type 3). Only one patient received nocturnal non-invasive ventilation to support chest development. SMN2 copy numbers and clinical characteristics of individual patients at the time of gene therapy are shown in Table 1. The observation period after treatment with gene therapy ranged from 2 to 11 months.
Table 1
Patient characteristics at time of gene therapy. To reduce the potential risk of re-identification of individual patients, we provide ranges for age and body weight and do not disclose the sex of individual patients in this table. *Values refer to time of gene therapy
Patient No. | Weight range* (kilogram) | Age range* (months) | Functional status* | Respiratory support and tube feeding* | Age at disease onset (months) | Previous drug treatment (age at start in months) | Best motor function before treatment with nusinersen | SMN2 copy number |
#1 | 7–7.9 | 19–21 | Sitting without support | None | 4–6 | Nusinersen (13–15) | Sitting without support | 3 |
#2 | 8–8.9 | 10–12 | Sitting without support | None | 4–6 | Nusinersen (7–9) | Head control | 2 |
#3 | 9–9.9 | 16–18 | Sitting without support | None | 7–9 | Nusinersen (13–15) | Sitting without support | 3 |
#4 | 9–9.9 | 10–12 | No head control | Nocturnal non-invasive ventilation, tube for additional fluids | 1–3 | Nusinersen (1–3) | No head control | 2 |
#5 | 10–10.9 | 16–18 | Sitting without support | None | 1–3 | Nusinersen (7–9) | Head control | 3 |
#6 | 10–10.9 | 37–39 | Sitting without support | None | 10–12 | Nusinersen (28–30) | Sitting without support | 3 |
#7 | 11–11.9 | 19–21 | Walking | None | Newborn-screening | Nusinersen (1–3) | n.a. | 2 |
#8 | 10–10.9 | 10–12 | Sitting without support | None | Newborn-screening | Nusinersen (1–3) | n.a. | 3 |
Clinical adverse events
All patients showed a temporary increase of the body temperature above 38.5° C, which occurred between days 2 and 5 and did not last more than 2 days. We observed occasional vomiting following gene therapy in 3 patients. Three parents reported reduced appetite over a period of several weeks.
During the period of severe liver damage, patient #7 showed vomiting, reduced activity and appetite, and an increase of bilateral hand tremor, which was only mildly present before. Due to the young age mental status was difficult to assess but hepatic encephalopathy was not evident during this period. Temporary arterial hypertension was noted on two days during the steroid pulse therapy but did not require specific treatment.
Laboratory abnormalities
Table 2 summarizes the frequency and characteristics of the most relevant laboratory abnormalities observed in our cohort after the administration of gene therapy. Figure 1 illustrates the development of individual laboratory findings for each patient over time.
Table 2
Frequency and characteristics of laboratory abnormalities after gene therapy with onasemnogene abeparvovec in our cohort
Value | Patients with abnormal values (total n = 8) | Observed abnormalities/comments |
Transaminases | 8/8 | Peak between days 3 and 7 in 6 patients, highest value below 1000 U/l in 7 patients, above 1000 U/l in patient #7 (associated with impaired liver function, see results for details on patient #7), secondary increase after reduction of prednisolone |
C-reactive protein | 6/8 | Temporary mild increase up to 18 mg/L (ULN 3 mg/L) |
Troponin I | 4/8 | Normal before treatment in all cases, increase above ULN (17.5 ng/L) in 4 patients, highest values between 27 and 66 ng/L in week 3 and 4 after treatment, no abnormalities on electrocardiogram and echocardiography |
Troponin T | Already above ULN (14 ng/L) before treatment in 6 patients, fluctuation between measurements, no clear pattern | |
Thrombocytes | 6/8 | Thrombocytopenia (<150 x 109/L) in all 6 affected patients between days 6 and 8, lowest count 43×109/L, no clinical signs or symptoms |
Monocytes | 7/8 | Increase of absolute monocyte count above the age appropriate ULN (1.45×109/L), peak around day 7 |
ULN: Upper limit of normal.
Fig. 1
Development of laboratory values over time. On the horizontal axis d 0 refers to the day of treatment with onasemnogene abeparvovec. The daily dose of prednisolone in mg/kg is shown below the days. ALT: alanine aminotransferase, AST: aspartate aminotransferase, CRP: C-reactive protein, TA: transaminases.
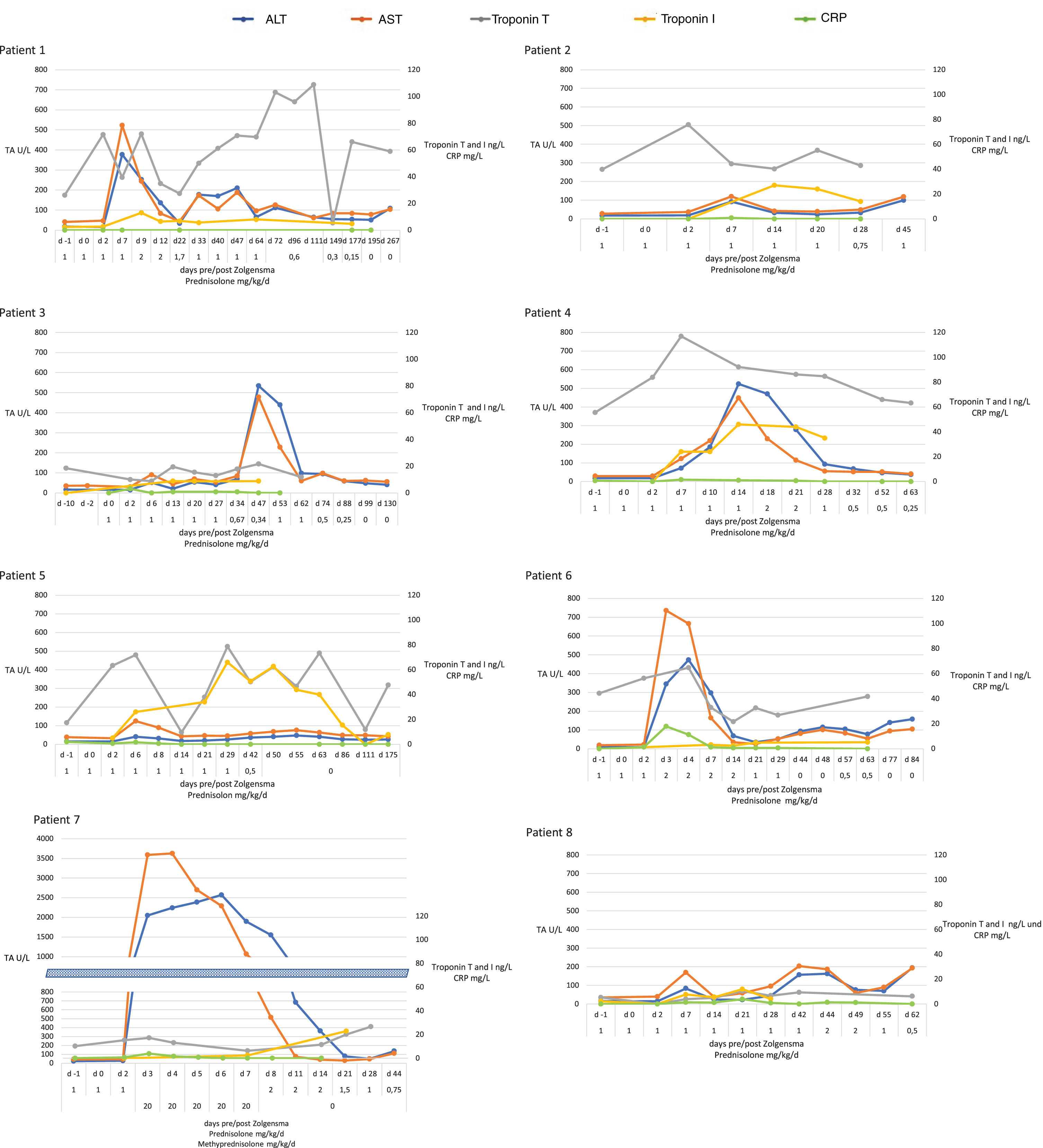
All patients showed increase of transaminases above normal ranges. In most patients (6 of 8) the maximum value was reached between day 3 and day 7, in one patient the peak occurred only after reduction of prednisolone (week 7). In all but one patient elevations of transaminases remained below 1,000 U/L. Patient #7 showed elevation of aspartate aminotransferase (AST) up to 4,512 U/L and alanine aminotransferase (ALT) up to 2,595 U/L, which was also associated with an impaired liver function with international normalized ratio (INR) of 1.6 (normal range 0.9–1.1). Serum cholinesterase and bilirubin remained within normal range. In patient #7, INR returned to normal within three days and transaminases to almost normal levels within four weeks after treatment. Increased transaminases typically responded to steroid treatment.
Creatine kinase levels were normal or mildly elevated but did not increase significantly following treatment.
Troponin I values were normal in all patients before treatment and increased above ULN (17.5 ng/L) in 4/8 patients after administration of gene therapy. The highest values for troponin I were reached during the third and fourth week after treatment. Troponin T values were less consistent and already above normal (ULN 14 ng/L) in 6/8 patients before treatment. During follow-up, values fluctuated between normal and up to 117 ng/L. Details are shown in Fig. 1.
Blood counts revealed thrombocytopenia (<150×109/L) in 6/8 patients with lowest values in all six patients between days 6 and 8. None of the patients showed cutaneous, mucosal or other bleeding signs. Thrombocytes normalized in all patients in the weeks following treatment.
In addition, we observed an increase of absolute monocyte count above the age appropriate ULN (1.45×109/L) in 7/8 patients. Increase of monocytes counts correlated with transaminases and also peaked around day 7. For development of blood counts over time see Supplemental Figure 1.
Steroid treatment
In 7/8 patients it was necessary to adapt the standard dose (1 mg/kg/d prednisolone for four weeks with consecutive tapering). In 5/8 we increased the daily dose above 1 mg/kg due to increased transaminases and/or thrombocytopenia. In most of these cases the dose was increased to 2 mg/kg. In patient #7 with massive increase of transaminases and impaired liver function, we administered a pulse therapy with a daily dose of 20 mg/kg methylprednisolone for five consecutive days starting on day 3 post gene therapy. Prednisolone treatment was continued beyond the four weeks of standard treatment in 7/8. Four of these patients stopped receiving steroids after 2–6 months, in three patients, treatment is ongoing, but the observation period is relatively short. Details of steroid dose over time for individual patients are shown below the horizontal axis for each patient in Fig. 1 and Supplemental Figure 1.
Cardiac evaluation
Regular cardiac assessment with echocardiography and electrocardiogram did not detect any abnormalities in cardiac function or deviation from baseline despite increased values for troponin in serum.
Functional outcomes
Functional assessment with standardized outcome measures are challenging in this age group due to limited and changing cooperation during the evaluations. Most parents reported improvements during the weeks after treatment, but longer observation periods are needed to measure and objectify these findings. Interestingly, two families reported an increase of pre-existing tremor after gene therapy.
DISCUSSION
In this case series we describe safety findings of eight consecutive patients with SMA who received treatment with onasemnogene abeparvovec. Interestingly, despite prophylactic steroid treatment all patients showed an increase of transaminase levels, which was associated with impaired liver function in one patient. In addition, we observed increased body temperature for one or two days during the first week after treatment in all patients. Asymptomatic thrombocytopenia was observed in 6 out of 8 patients. These findings most likely reflect an acute immune response to the treatment with onasemnogene abeparvovec. Many patients also showed a secondary increase of transaminase values after reduction of the prednisolone dose, but in most cases, this was less pronounced than during the first week after treatment. The rate of clinical and laboratory findings in our case series is much higher than reported in the pivotal clinical trial. Mendell et al. reported elevated aminotransferase levels only in 3 of the 12 patients who received the dose now used in clinical practice and in our patients [6].
Adeno-associated virus (AAV) vectors are increasingly used as gene delivery tools, as they can drive long-term transgene expression through transduction of terminally differentiated tissues. However, immune responses in humans undergoing gene transfer with AAV vectors have been an important obstacle to the advancement in the field [10, 11]. As AAV vectors lack any coding viral sequence, the main antigen sources for immune responses are the viral vector and the transgene product. Reactions can involve both, the innate and adaptive immune system. Experience from clinical trials suggests that AAV vector immunogenicity is dose-dependent to some extent [12].
The high rate of clinical and laboratory signs of immune response in our series compared to the pivotal trial might thus be due to the difference in age and body weight between the two cohorts. While in the original study all patients were less than 8 months of age with body weights between 3.6 and of 8.4 kg, all of our patients were older and had body weights between 7.1 and 11.9 kg. As the dose is proportional to body weight, the total viral vector load was therefore significantly higher in our cohort. This observation was also corroborated by a recent publication of Waldrop et al. In a series of 21 children between 1 and 23 months of age, they also observed a more pronounced elevation of transaminases in older children [13].
Of clinical concern in our case series was patient #7 who developed a more than 20fold increase of transaminase levels starting on day 3 post treatment, which was also associated with impaired liver function. We immediately started a steroid pulse therapy, which was associated with normalisation of liver function within a couple of days. Liver enzymes returned to normal levels during the following weeks without any signs of long-term liver damage.
Recently, Feldmann and all published two similar cases with subacute liver failure following gene therapy with onasemnogene abeparvovec. The two children were 6 and 20 months old, respectively, and had previously been treated with nusinersen [14]. From the available experience it is evident that in addition to vector dependent factors like design and dose, there are also host dependent factors that might trigger and influence the extent of immune response. This could include pre-existing immunity, concurrent infections, age or genetic background. As the impact of these factors is not fully understood, it is currently not possible to predict the likelihood of a severe immune response in an individual patient. Recently, three fatalities associated with liver failure following an AAV8 vector-based gene therapy for x-linked myotubular myopathy were reported. Even though these cases illustrate the potential risks associated with gene therapy, there are important differences that preclude direct comparison with gene therapy for SMA. First, the two products use different vectors with different properties concerning immunogenicity and tissue tropism, and second, all three patients who died demonstrated evidence of pre-existing hepatobiliary disease [15, 16].
Preclinical studies have shown dorsal root ganglia damage following intrathecal administration of onasemnogene abeparvovec and high-dose intravenous treatment with an AAV9 variant (AAVhu68) carrying a human SMN transgene [17]. The clinical relevance of these finding is not clear and it remains to be seen, if intrathecal dosing might be associated with a better risk-benefit ratio in older patients [7]. In our cohort we did not observe any sensory signs or symptoms, but these are difficult to assess in this young age group. Reliable neurophysiological tests are also challenging and were not part of our routine clinical care.
Cardiac monitoring revealed a post-treatment increase of troponin I above ULN in half of the patients, but this was not associated with any clinical symptoms or abnormalities on electrocardiogram and echocardiography. In contrast to troponin I, troponin T measurements were less consistent and often elevated above ULN even before treatment. There are several pathophysiological and analytical differences between troponin I and T [18], but little is known about normal troponin values in healthy children [19, 20] and in children with SMA [21, 22]. Our data suggests that troponin I is more appropriate to monitor cardiotoxicity than troponin T. Whether troponin I values above a certain cut-off are indicative of cardiac tissue injury and should induce additional examinations or an escalation of immunosuppression remains unclear.
Blood counts revealed asymptomatic thrombocytopenia in 6/8 patients, which consistently peaked around day 7. In addition, we found high monocyte counts in 7/8 patients as confirmed by manual cell count. Monocytes originate from the common myeloid progenitor cell and take part in innate as well as adaptive immunity. They circulate in the bloodstream as monocytes and migrate into tissues, where they can differentiate into tissue resident macrophages or dendritic cells [23]. As dendritic cells they take part in antigen sensing, as macrophages they drive inflammation in early phases of infections. High monocyte counts are common in paediatric infections with Epstein-Barr virus, adenovirus or respiratory syncytial virus [24]. AAV9 has been shown to stimulate dendritic cells via TLR9 with activation of adaptive immunity [25]. To better understand the immune activation following gene therapy with AAV9 vectors a more detailed characterisation regarding subpopulations of blood cells, cytokines and the time course of those events would be necessary, but was not part of the routine clinical care in this case series. Elucidation of the involved pathways would also be fundamental to select appropriate second line drugs for those cases where steroids alone are not sufficient to control the immune reaction after administration of onasemnogene abeparvovec.
In conclusion, our experience with eight patients older than eight months adds important findings to the increasing body of evidence that treatment of SMA with onasemnogene abeparvovec is often associated with an immune response against the AAV vector. This immune response mainly effects the liver and the hematopoietic system, and can be severe in some cases. In all our patients it was possible to control the response by pro-active monitoring and adaptation of the steroid dose, and we did not detect any long-term side effects due to the immune response. However, it is premature to judge whether severe organ damage with long term sequelae can always be avoided. Further research and data collection are needed to reveal the underlying mechanisms and to identify patients who are at risk for more severe side effects. Total viral vector dose and thus body weight is most likely one aspect, but there might be other host-dependent factors that are more difficult to identify.
CONFLICT OF INTEREST STATEMENT
The authors did not receive financial or material support for this research. JK received support from Avexis/Novartis Gene Therapy, Biogen, Roche, Scholar Rock for educational activities, consultancy and/or clinical research projects. The other authors have no conflict of interest to report.
SUPPLEMENTARY MATERIAL
[1] The supplementary material is available in the electronic version of this article: https://dx.doi.org/10.3233/JND-200593.
REFERENCES
[1] | Finkel RS , Mercuri E , Meyer OH , Simonds AK , Schroth MK , Graham RJ , et al. Diagnosis and management of spinal muscular atrophy: Part 2: Pulmonary and acute care; medications, supplements and immunizations; other organ systems; and ethics. Neuromuscul Disord. (2018) ;28: (3):197–207. |
[2] | Mercuri E , Finkel RS , Muntoni F , Wirth B , Montes J , Main M , et al. Diagnosis and management of spinal muscular atrophy: Part 1 Recommendations for diagnosis, rehabilitation, orthopedic and nutritional care. Neuromuscul Disord. (2018) ;28: (2):103–15. |
[3] | Lefebvre S , Burglen L , Reboullet S , Clermont O , Burlet P , Viollet L , et al. Identification and characterization of a spinal muscular atrophy-determining gene. Cell. (1995) ;80: (1):155–65. |
[4] | Calucho M , Bernal S , Alias L , March F , Vencesla A , Rodriguez-Alvarez FJ , et al. Correlation between SMA type and SMN2 copy number revisited: An analysis of 625 unrelated Spanish patients and a compilation of 2834 reported cases. Neuromuscul Disord. (2018) ;28: (3):208–15. |
[5] | Schorling DC , Pechmann A , Kirschner J . Advances in Treatment of Spinal Muscular Atrophy - New Phenotypes, New Challenges, New Implications for Care. J Neuromuscul Dis. (2020) ;7: (1):1–13. |
[6] | Mendell JR , Al-Zaidy S , Shell R , Arnold WD , Rodino-Klapac LR , Prior TW , et al. Single-Dose Gene-Replacement Therapy for Spinal Muscular Atrophy. N Engl J Med. (2017) ;377: (18):1713–22. |
[7] | Kirschner J , Butoianu N , Goemans N , Haberlova J , Kostera-Pruszczyk A , Mercuri E , et al. European ad-hoc consensus statement on gene replacement therapy for spinal muscular atrophy. Eur J Paediatr Neurol. (2020) ;28: :38–43. |
[8] | Pechmann A , Konig K , Bernert G , Schachtrup K , Schara U , Schorling D , et al. SMArtCARE - A platform to collect real-life outcome data of patients with spinal muscular atrophy. Orphanet J Rare Dis. (2019) ;14: (1):18. |
[9] | Ziegler A , Wilichowski E , Schara U , Hahn A , Muller-Felber W , Johannsen J , et al. [Recommendations for gene therapy of spinal muscular atrophy with onasemnogene abeparvovec-AVXS-101: Consensus paper of the German representatives of the Society for Pediatric Neurology (GNP) and the German treatment centers with collaboration of the medical scientific advisory board of the German Society for Muscular Diseases (DGM)]. Nervenarzt. (2020) ;91: (6):518–29. |
[10] | Verdera HC , Kuranda K , Mingozzi F . AAV Vector Immunogenicity in Humans: A Long Journey to Successful Gene Transfer. Mol Ther. (2020) ;28: (3):723–46. |
[11] | Shirley JL , de Jong YP , Terhorst C , Herzog RW . Immune Responses to Viral Gene Therapy Vectors. Mol Ther. (2020) ;28: (3):709–22. |
[12] | Mingozzi F , Meulenberg JJ , Hui DJ , Basner-Tschakarjan E , Hasbrouck NC , Edmonson SA , et al. AAV-1-mediated gene transfer to skeletal muscle in humans results in dose-dependent activation of capsid-specific T cells. Blood. (2009) ;114: (10):2077–86. |
[13] | Waldrop MA , Karingada C , Storey MA , Powers B , Iammarino MA , Miller NF , et al. Gene Therapy for Spinal Muscular Atrophy: Safety and Early Outcomes. Pediatrics. (2020) ;146: (3). |
[14] | Feldman AG , Parsons JA , Dutmer CM , Veerapandiyan A , Hafberg E , Maloney N , et al. Subacute Liver Failure Following Gene Replacement Therapy for Spinal Muscular Atrophy Type I. J Pediatr. 2020. |
[15] | Shieh PB , Bonnemann CG , Muller-Felber W , Blaschek A , Dowling JJ , Kuntz NL , et al. Re: “Moving Forward After Two Deaths in a Gene Therapy Trial of Myotubular Myopathy” by Wilson and Flotte. Hum Gene Ther. (2020) ;31: (15-16):787. |
[16] | Wilson JM , Flotte TR . Moving Forward After Two Deaths in a Gene Therapy Trial of Myotubular Myopathy. Hum Gene Ther. (2020) ;31: (13-14):695–6. |
[17] | Hinderer C , Katz N , Buza EL , Dyer C , Goode T , Bell P , et al. Severe Toxicity in Nonhuman Primates and Piglets Following High-Dose Intravenous Administration of an Adeno-Associated Virus Vector Expressing Human SMN. Hum Gene Ther. (2018) ;29: (3):285–98. |
[18] | van der Linden N , Wildi K , Twerenbold R , Pickering JW , Than M , Cullen L , et al. Combining High-Sensitivity Cardiac Troponin I and Cardiac Troponin T in the Early Diagnosis of Acute Myocardial Infarction. Circulation. (2018) ;138: (10):989–99. |
[19] | Karlen J , Karlsson M , Eliasson H , Bonamy AE , Halvorsen CP . Cardiac Troponin T in Healthy Full-Term Infants. Pediatr Cardiol. (2019) ;40: (8):1645–54. |
[20] | Yoldas T , Orun UA . What is the Significance of Elevated Troponin I in Children and Adolescents? A Diagnostic Approach. Pediatr Cardiol. (2019) ;40: (8):1638–44. |
[21] | Djordjevic SA , Milic-Rasic V , Brankovic V , Kosac A , Vukomanovic G , Topalovic M , et al. Cardiac findings in pediatric patients with spinal muscular atrophy types 2 and 3. Muscle Nerve. 2020. |
[22] | Rittoo D , Jones A , Lecky B , Neithercut D . Elevation of cardiac troponin T, but not cardiac troponin I, in patients with neuromuscular diseases: Implications for the diagnosis of myocardial infarction. J Am Coll Cardiol. (2014) ;63: (22):2411–20. |
[23] | Ziegler-Heitbrock L , Ancuta P , Crowe S , Dalod M , Grau V , Hart DN , et al. Nomenclature of monocytes and dendritic cells in blood. Blood. (2010) ;116: (16):e74–80. |
[24] | Choi E , Ha KS , Song DJ , Lee JH , Lee KC . Clinical and laboratory profiles of hospitalized children with acute respiratory virus infection. Korean J Pediatr. (2018) ;61: (6):180–6. |
[25] | Zhu J , Huang X , Yang Y . The TLR9-MyD88 pathway is critical for adaptive immune responses to adeno-associated virus gene therapy vectors in mice. J Clin Invest. (2009) ;119: (8):2388–98. |