Wireless multichannel electroencephalography in the newborn
Abstract
OBJECTIVES: First, to determine the feasibility of an ultra-compact wireless device (microEEG) to obtain multichannel electroencephalographic (EEG) recording in the Neonatal Intensive Care Unit (NICU). Second, to identify problem areas in order to improve wireless EEG performance.
STUDY DESIGN: 28 subjects (gestational age 24–30 weeks, postnatal age <30 days) were recruited at 2 sites as part of an ongoing study of neonatal apnea and wireless EEG. Infants underwent 8-9 hour EEG recordings every 2–4 weeks using an electrode cap (ANT-Neuro) connected to the wireless EEG device (Bio-Signal Group). A 23 electrode configuration was used incorporating the International 10–20 System. The device transmitted recordings wirelessly to a laptop computer for bedside assessment. The recordings were assessed by a pediatric neurophysiologist for interpretability.
RESULTS: A total of 84 EEGs were recorded from 28 neonates. 61 EEG studies were obtained in infants prior to 35 weeks corrected gestational age (CGA). NICU staff placed all electrode caps and initiated all recordings. Of these recordings 6 (10%) were uninterpretable due to artifacts and one study could not be accessed. The remaining 54 (89%) EEG recordings were acceptable for clinical review and interpretation by a pediatric neurophysiologist. Of the recordings obtained at 35 weeks corrected gestational age or later only 11 out of 23 (48%) were interpretable.
CONCLUSIONS: Wireless EEG devices can provide practical, continuous, multichannel EEG monitoring in preterm neonates. Their small size and ease of use could overcome obstacles associated with EEG recording and interpretation in the NICU.
Abbreviations
aEEG | Amplitude-Integrated EEG |
cEEG | Conventional EEG |
CFM | Cerebral Function Monitor |
NICU | Neonatal Intensive Care Unit |
1Introduction
Predicting and improving neurodevelopmental outcome is a primary concern in the care of newborn infants. A variety of techniques have been developed to examine the newborn brain, to assess pathology and, possibly, direct treatment. The role of electroencephalography (EEG) in assessing newborn infants is continuously evolving. In addition to its established role in detecting seizures, several studies have demonstrated the relationship between EEG changes and neurodevelopmental outcome [1–4]. Abnormalities on EEG have also been associated with intraventricular hemorrhage, periventricular leukomalacia and white matter injury [5–7].
Conventional EEG (cEEG) is the “gold standard” for measuring cerebral electrical activity and diagnosing seizure activity [8]. Obtaining cEEG recordings is technically challenging and requires specialized technicians to initiate and monitor recordings. cEEG can be expensive and difficult to initiate rapidly. Amplitude-integrated EEG (aEEG) obtained using a Cerebral Function Monitor (CFM) is a more practical alternative to cEEG. aEEG allows bedside staff to initiate a limited EEG recording rapidly without the assistance of specialized staff. aEEG utilizes only 2 to 4 electrodes, and thus may not detect localized abnormalities in cerebral electrical activity such as focal seizures [9–11]. Investigations using aEEG in preterm infants have found a high rate of artifacts [12, 13]. Despite these limitations, aEEG use has been widely adopted at tertiary care Neonatal Intensive Care Units (NICUs) [14, 15]. The increasing use of aEEG in clinical as well as research settings has raised concerns [16].
An ideal system for performing EEG in the NICU would combine the best qualities of both aEEG and cEEG. A new ultra-compact wireless device known as microEEG has the potential to provide practical multichannel EEG recording in the NICU. Previous studies have demonstrated the feasibility of this device in the adult emergency department setting [17, 18]. These studies demonstrated advantages over existing multichannel EEG systems such as easier application, enhanced portability and ease of use. We proposed that a wireless EEG device is feasible for use in the NICU as well. The first objective of this study was to determine the feasibility and advantage of serial wireless EEG recordings of preterm infants in the NICU. Our second objective was to identify problem areas in order to improve wireless EEG performance in the clinical setting.
2Methods
2.1Study design
This is an observational study and preterm newborn subjects were enrolled in a prospective study examining neonatal apnea, bradycardia and desaturation being conducted at two Level IIIB NICUs. Parental consent was obtained when preterm infants manifested apnea, bradycardia or desaturation. This study was approved by the Institutional Review Boards of SUNY Health Science Center at Brooklyn and Brookdale University Medical Center.
2.2Inclusion and exclusion criteria
Preterm neonates were eligible for this study if they fulfilled the following criteria: 1) gestational age between 24 0/7 to 32 6/7 weeks. 2) postnatal age ≤30 days and 3) had at least 2 episodes of apnea (cessation of breathing greater than 20 seconds), bradycardia (heart rate less than 100 beats per minute) or desaturation (oxygen saturation less than 80%) within a 12 hour period.
Exclusion criteria included, major congenital anomalies, exposed dermis due to immature skin and scalp lesions.
2.3EEG recordings
Enrolled infants underwent EEG recordings at 2–4 week intervals. All EEGs were initiated at the bedside by a neonatologist who underwent a brief training in device operation. The neonatologists were trained to apply the cap quickly and with no skin preparation. This was done to simulate the manner in which the device would be applied in an everyday clinical (i.e. non-research) setting. Both NICUs were of an open bay design and no recordings took place in a private room or isolation room. No specialized electronic shielding was employed during recording. EEG recordings were scheduled for 8-9 hours, except when there were overriding clinical and diagnostic procedures (such as Magnetic Resonance Imaging) requiring that the EEG recording be shortened. The duration of the recording was limited by the battery life of the wireless device which was approximately 10 hours.
2.4Placement of EEG recording cap
EEG was obtained in all patients using an electrode cap (Waveguard, ANT-Neuro, Enchede, The Netherlands) connected to the wireless EEG device (microEEG® Bio-Signal Group, Brooklyn, NY). The electrode caps are specifically designed for neonatal use and contain tin electrodes held in place by a soft, flexible cloth covering. The cap utilizes a 23 electrode configuration incorporating the International 10–20 System, similar to conventional EEG. The size of the cap was matched to the individual subject’s head circumference with sizes ranging from 25 cms to 35 cms. Conducting gel was applied to the electrodes prior to placement of the cap. The cap was then placed directly onto the scalp. For infants with nasal prongs the straps for the prongs were secured over the cap. The skin was not abraded prior to cap application. These procedures were designed to minimize disturbance of the infant during placement and minimize the time required for placement. The procedures used in our study differ from previously described protocols in which the electrode gel is applied after the cap is in place. Placement of the cap took approximately 1 minute. The average time required for the neonatologist to place the electrode cap and initiate recording was 13 min (SD 9 min). In addition to scalp electrodes a simultaneous electrocardiogram was recorded along with the EEG recording and was available to the interpreting neurophysiologist. At this time the device does not fully incorporate video into the EEG recording.
2.5Wireless EEG device
The microEEG® (Fig. 1) is a miniature (9.4× 4.4×3.8 cms, 88 grams), wireless device that digitizes EEG signals for wireless transmission. The device is FDA approved for use in neonates. The device digitizes analog EEG electrical signals at a point close to the electrode. This allows for wireless digital transmission of EEG recordings via Bluetooth® protocol. The technical aspects of microEEG recordings have been reported elsewhere [18]. Its accuracy has been verified in studies of adult patients presenting to the emergency department and shown to be non-inferior to recordings obtained with standard devices [17].
The wireless EEG device was connected to the electrode cap via a specialized adaptor (Biosignal Corp). The EEG device and all connecting wires remained inside of the patient isolette or bassinette throughout the recording, thus optimizing thermoregulation and minimizing disturbance to the infant (Fig. 2). All equipment was cleaned and disinfected prior to each patient use. The device transmitted the EEG recording to a standard “PC” laptop computer running specialized software (Fig. 3). This software displayed the EEG recordings in real time. The EEG recordings were stored in the non-proprietary European Data Format (.edf) and could be read by commonly available EEG reading software. A backup of the recording was stored on the device in the event the Bluetooth connection was interrupted. After recording the EEG data was uploaded to a central server. EEGs were then read remotely from the central server by a pediatric neurophysiologist who sent back the result to the neonatal unit.
2.6Clinical care
No changes were made to the patient care regimen during recording and all activities of routine and critical neonatal care continued unabated. This included orogastric and oral feeding, invasive and non-invasive mechanical ventilation, as well as intravenous fluid and medication administration.
2.7EEG analysis
EEG recordings were interpreted by a pediatric neurophysiologist (GC) who was blinded to all clinical information except for the gestational age and the chronological age. The EEG recordings were reviewed using the Persyst Insight software (Persyst Development Corporation, San Diego, CA) (Fig. 4). A more limited EEG montage utilizing a typical neonatal montage was used for analysis. EEGs were determined to be acceptable when the cerebral activity was identified for at least 90–120 minutes during the recordings. Recordings were deemed uninterpretable if no cortical activity could be identified due to the presence of artifacts. Examples of artifacts included continuous 60 Hz interference in all channels (that could not be corrected by use of the notch filter), multiple electrode artifacts and disconnection of the electrode cap due to technical issues.
2.8Statistical methods
Interpretable and uninterpretable EEGs were compared in regards to four patient characteristics at the time of recording (corrected gestational age, weight, chronological age, and head circumference). For each characteristic, a mixed linear model was fitted. This model was used to account for the non-independence of the observations. The fixed factor was whether the EEG was uninterpretable. Child ID number was introduced as a random factor. Satterthwaite corrections were made to denominator degrees of freedom; model residuals were inspected for outliers and for skew. Model-generated adjusted means with standard errors and confidence intervals are reported.
3Results
3.1Study subjects
Twenty-eight infants were enrolled from January 2013 until December 2014. All subjects were African American consistent with our patient demography. The subjects underwent a total of 84 recordings. One recording could not be accessed after completion due to an undetermined technical cause. Two patients (a set of twins) withdrew from the study based on parental request after two recordings. One infant was transferred for patent ductus arteriosus ligation after two recordings and died at the referral hospital. All recordings were included in the final analysis. The baseline characteristics of the infants are shown in Table 1. Respiratory and feeding interventions ongoing during recording are listed in Table 2.
3.2Recordings
No infant had difficulties that prevented a recording from taking place on the assigned day. The average recording lasted 7.9 hrs (SD 1.6 hrs). After completion, EEG recordings were successfully uploaded to a central server in 49 (58%) cases. When the recording file could not be uploaded it was transferred manually to a desktop computer via USB storage device. The primary reason for upload failure was due to infrastructure problems, including network and server related issues.
As shown in Table 2, uninterpretable recordings generally occurred when the infants were older, more mature, heavier, and had a larger head circumference. 89% of recordings performed prior to 35 weeks CGA were interpretable. The primary reason for uninterpretable recording was that EEG waveforms could not be identified secondary to disconnection of the cap from the scalp. There were no adverse events attributable to cap placement or EEG recording. All infants had a temporary skin indentation at the site of the electrodes. This indentation resolved quickly after removal of the cap.
4Discussion
This study demonstrated that wireless EEG devices can provide full spectrum EEG in the NICU setting and has many advantages compared to established devices. This is the first time the microEEG device has been used in the newborn. First, we were able to initiate recording quickly, with no skin preparation, and without assistance from specialized staff. The effect on clinical care was negligible, even in critically ill infants. Second, unlike aEEG, our recordings fulfilled many of the technical specifications for continuous EEG monitoring in neonates outlined by the American Clinical Neurophysiology Society (8). These specifications include multichannel recording using the International 10–20 System, simultaneous electrocardiogram (EKG) recording and prolonged recording over one hour. Third, an ultra-compact device is smaller and more portable than commonly used aEEG and cEEG equipment. This allows for easy storage and use in areas where space is limited. Fourth, the interpretation standards used in our study were similar to multichannel conventional EEG recording. All recordings were read and interpreted by an experienced pediatric neurophysiologist.
Wireless EEG recording as used in our study has limitations. First, continuous recording was limited to up to 9–10 hours due in part to limitations in battery life. In future studies this could be overcome by using a backup battery system. Additional studies are needed to determine if a 24 hour recording with video is feasible. This is the minimum recording time recommended for the detection of electrographic seizures [8]. With the advent of inexpensive, miniature video cameras, incorporating this technology into future wireless EEG systems should not be difficult. Second, the smallest electrode cap we used required a head circumference of 25 cms. This limited our ability to record from the smallest infants. Development of smaller sized of caps will correct this limitation. Third, during the study, the device integrates EKG data but does not fully integrate respiratory and pulse oximetry monitoring. This limits the device’s ability to discover associations between clinical events and EEG findings. Fourth, we had difficulty uploading recordings to a central server. In order to consistently obtain rapid, remote EEG interpretation, all of these issues are being addressed. Notwithstanding, our study shows the feasibility and advantages of the wireless EEG in the newborn.
We had greater success in recording smaller and less mature infants. It is important to note that most uninterpretable recordings occurred when subjects were no longer critically ill. Thus, these recordings were more prone to motion artifacts and difficulties in maintaining cap placement. The EEG recording caps used were chosen because they are available in head sizes appropriate for premature infants [19]. There are a wide variety of EEG electrode cap technologies available for larger infants. These technologies (and future cap technologies) might be less prone to artifacts.
5Conclusion
Our results demonstrate the feasibility and potential advantages of ultra-compact, wireless EEG devices to expand access to EEG recording in the NICU. Further technological advances, along with improvements in recording technique, could lead to wireless EEG devices that combine the diagnostic capability of cEEG with the ease and practicality of aEEG.
Conflict of interest
Dr. Samah Abdel Baki is an employee of the Biosignal Group and is co-inventor on patents for the microEEG device. The remaining authors have nothing to disclose.
Funding source
All phases of the study were supported by NIH#R41HD072881 (STTR program).
Acknowledgments
The authors acknowledge the outstanding contributions of the late Rudolph Parris DrPH, who assisted in study planning, coordination and data collection. The authors acknowledge Dr. Andre Fenton and Dr. Ahmet Omurtag of the Biosignal Group for their assistance in the early phases of the study. We acknowledge Dr. Rita Marshall and Dr. Akosua Agyepong for their assistance with data collection. We acknowledge Ernest A. Cuni of Biomedical Communications at SUNY Downstate for studio photography. We acknowledge the nursing and respiratory therapy staff at SUNY Downstate Medical Center and Brookdale Hospital for their assistance with the EEG recordings. We would like to express our gratitude to Dr. Gloria Valencia for her continuing support of these endeavors.
References
[1] | Holmes GL , Lombroso CT . Prognostic value of background patterns in the neonatal EEG. J Clin Neurophysiol (1993) ;10: (3):323–52. |
[2] | Vesoulis ZA , Inder TE , Woodward LJ , Buse B , Vavasseur C , Mathur AM . Early electrographic seizures, brain injury, and neurodevelopmental risk in the very preterm infant. Pediatr Res (2014) ;75: (4):564–9. |
[3] | Murray DM , Boylan GB , Ryan CA , Connolly S . Early EEG findings in hypoxic-ischemic encephalopathy predict outcomes at 2 years. Pediatrics (2009) ;124: (3):e459–67. |
[4] | El-Dib M , Massaro AN , Glass P , et al. Early amplitude integrated electroencephalography and outcome of very low birth weight infants. Pediatr Int (2011) ;53: (3):315–21. |
[5] | Clancy RR , Tharp BR , Enzman D . EEG in premature infants with intraventricular hemorrhage. Neurology (1984) ;34: (5):583–90. |
[6] | Olischar M , Klebermass K , Waldhoer T , Pollak A , Weninger M . Background patterns and sleep-wake cycles on amplitude-integrated electroencephalography in preterms younger than 30 weeks gestational age with peri-/intraventricular haemorrhage. Acta Paediatr (2007) ;96: (12):1743–50. |
[7] | Inder TE , Buckland L , Williams CE , et al. Lowered electroencephalographic spectral edge frequency predicts the presence of cerebral white matter injury in premature infants. Pediatrics (2003) ;111: (1):27–33. |
[8] | Shellhaas RA , Chang T , Tsuchida T , et al. The American clinical neurophysiology society’s guideline on continuous electroencephalography monitoring in neonates. J Clin Neurophysiol (2011) ;28: (6):611–7. |
[9] | Bourez-Swart MD , van Rooij L , Rizzo C , et al. Detection of subclinical electroencephalographic seizure patterns with multichannel amplitude-integrated EEG in full-term neonates. Clin Neurophysiol (2009) ;120: (11):1916–22. |
[10] | Wusthoff CJ , Shellhaas RA , Clancy RR . Limitations of single-channel EEG on the forehead for neonatal seizure detection. J Perinatol (2009) ;29: (3):237–42. |
[11] | Toet MC , van der Meij W , de Vries LS , Uiterwaal CS , van Huffelen KC . Comparison between simultaneously recorded amplitude integrated electroencephalogram (cerebral function monitor) and standard electroencephalogram in neonates. Pediatrics (2002) ;109: (5):772–9. |
[12] | Suk D , Krauss AN , Engel M , Perlman JM . Amplitude-integrated electroencephalography in the NICU: Frequent artifacts in premature infants may limit its utility as a monitoring device. Pediatrics (2009) ;123: (2):e328–32. |
[13] | Hagmann CF , Robertson NJ , Azzopardi D . Artifacts on electroencephalograms may influence the amplitude-integrated EEG classification: A qualitative analysis in neonatal encephalopathy. Pediatrics (2006) ;118: (6):2552–4. |
[14] | Boylan G , Burgoyne L , Moore C , O’Flaherty B , Rennie J . An international survey of EEG use in the neonatal intensive care unit. Acta Paediatr (2010) ;99: (8):1150–5. |
[15] | Ponnusamy V , Nath P , Bissett L , Willis K , Clarke P . Current availability of cerebral function monitoring and hypothermia therapy in UK neonatal units. Arch Dis Childhood Fetal Neonatal Ed (2010) ;95: (5):F383–4. |
[16] | Boylan GB . EEG monitoring in the neonatal intensive care unit: A critical juncture. Clinical Neurophysiol (2011) ;122: (10):1905–7. |
[17] | Grant AC , Abdel-Baki SG , Omurtag A , et al. Diagnostic accuracy of microEEG: A miniature, wireless EEG device. Epilepsy Behav (2014) ;34: :81–5. |
[18] | Omurtag A , Baki SG , Chari G , et al. Technical and clinical analysis of microEEG: A miniature wireless EEG device designed to record high-quality EEG in the emergency department. Int J Emerg Med (2012) ;5: (1):35. |
[19] | Vanhatalo S , Metsaranta M , Andersson S . High-fidelity recording of brain activity in the extremely preterm babies: Feasibility study in the incubator. Clin Neurophysiol (2008) ;119: (2):439–45. |
Figures and Tables
Fig.1
The microEEG EEG device.
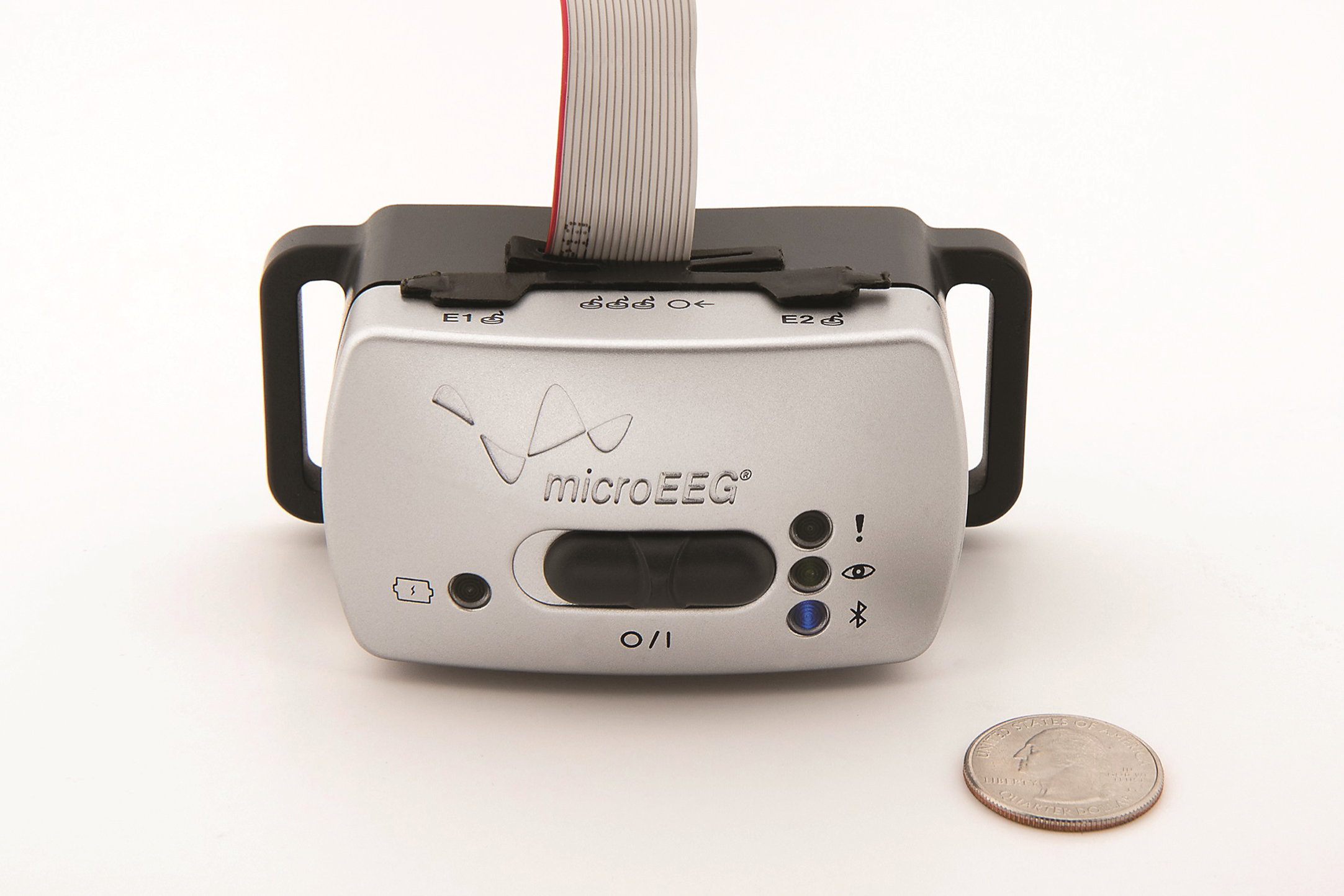
Fig.2
Preterm infant undergoing wireless EEG recording. The recording equipment is contained entirely within the isolette. The device transmits EEG recordings wirelessly to a bedside computer in real time. 1) Wireless EEG recording device 2) Connector cable 3) Electrode cap.
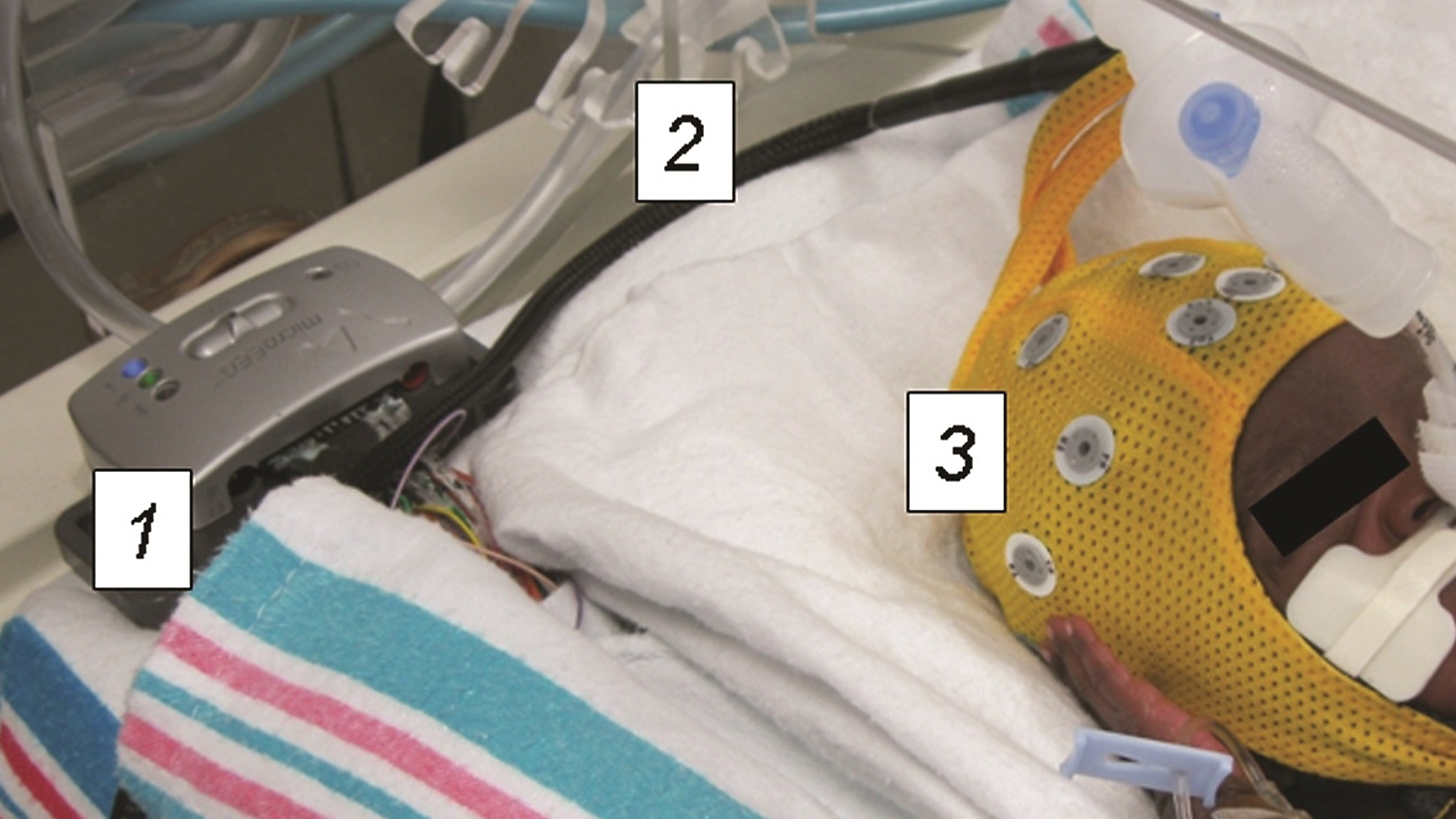
Fig.3
Flow diagram of the EEG recording process.
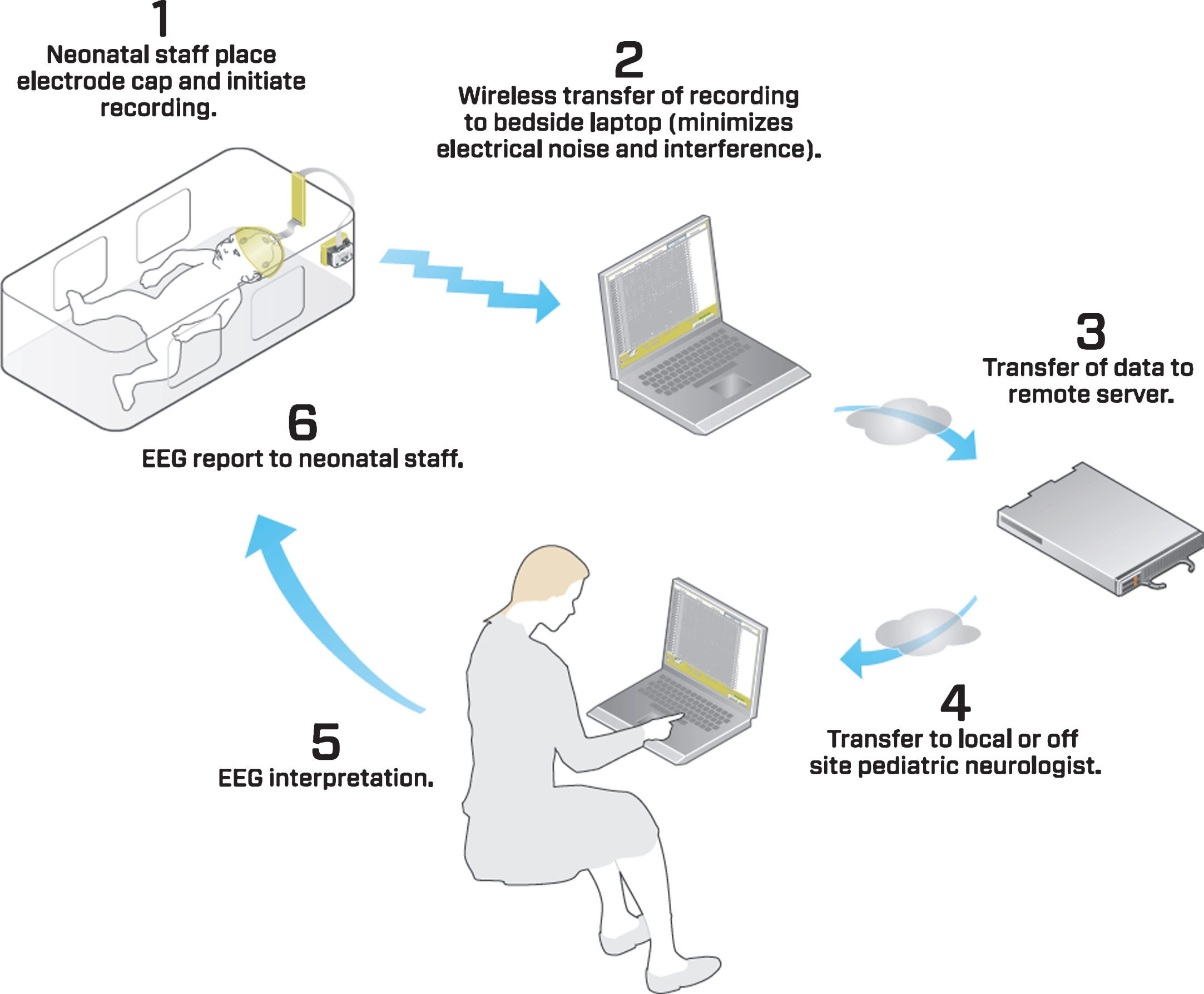
Fig.4
Wireless EEG recording in a 30 week corrected gestational age preterm infant displayed using Persyst® software.
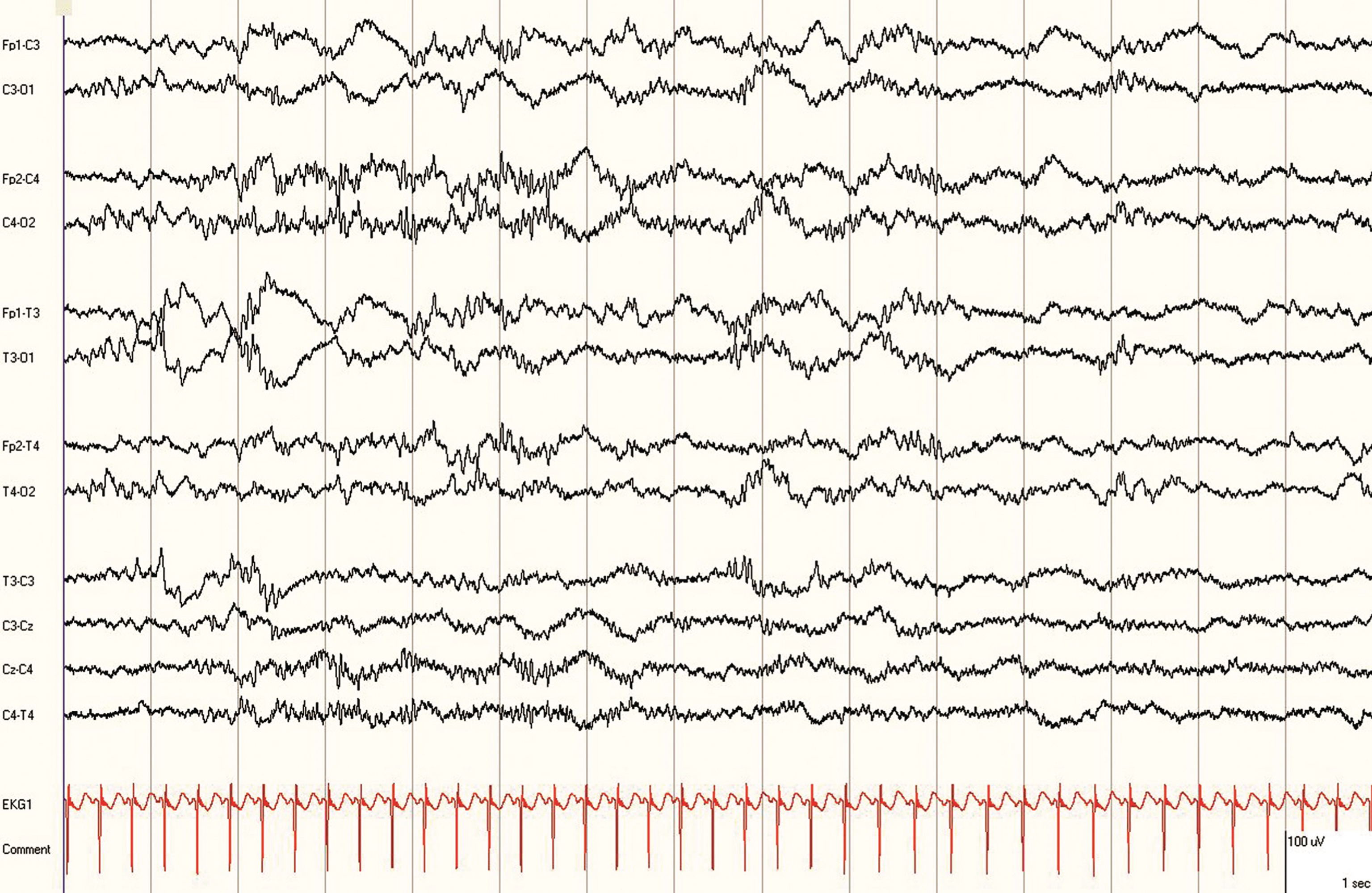
Table 1
Subject characteristics
Characteristic | Mean (SD) or n (%) |
Maternal age yr | 29.22 (6.4)a |
Gestational age wk | 27.4 (1.5)a |
Birth weight g | 988 (222)a |
Male | 14 (50)b |
Multiple gestation | 4 (14)b |
Cesarean delivery | 25 (89)b |
Antenatal steroids | 24 (85)b |
5-min Apgar score <5 | 1 (4)b |
aMean (SD); bn (%).
Table 2
Comparison of interpretable vs. uninterpretable recordings
Characteristics at time of recording | All | Interpretable | Uninterpretable | |
n (%) | 83a (100) | 65 (78) | 18 (22) | |
Corrected gestational age wk, mean (95% CI) | 33.2 (32.6–33.9) | 32.6 (31.9–33.3) | 35.5 (34.2–36.8) | P < 0.001b |
Weight g, mean (95% CI) | 1725 (1602–1847) | 1588 (1444–1732) | 2204 (1952–2455) | p < 0.001b |
Head circumference cm, mean (95% CI) | 28.4 (27.8–28.9) | 27.8 (27.1–28.5) | 30.2 (29.0–31.4) | p < 0.001b |
Chronological age d, mean (95% CI) | 42.4 (37.9–46.9) | 37.1 (32.6–41.7) | 61.4 (52.9–70.0) | p < 0.001b |
Mode of ventilation, n (%) | ||||
Room air | 31 (37) | 20 (31) | 11 (61) | |
Oxyhood | 1 (1) | 1 (2) | 0 (0) | |
Nasal cannula | 24 (29) | 21 (32) | 3 (17) | |
Continuous positive airway pressure | 11 (13) | 10 (15) | 1 (6) | |
Nasal intermittent mandatory ventilation | 6 (7) | 6 (9) | 0 (0) | |
Intubated | 10 (12) | 7 (11) | 3 (17) | |
Method of feeding, n (%) | ||||
No feeding | 9 (10) | 9 (13) | 0 (0) | |
Orogastric or nasogastric tube | 45 (54) | 38 (58) | 7 (39) | |
Oral | 29 (35) | 18 (28) | 11 (61) |
aExcludes one recording that could not be accessed. bCalculated using mixed linear model.