Sleep Dysfunction in Huntington’s Disease: Impacts of Current Medications and Prospects for Treatment
Abstract
Sleep dysfunction is highly prevalent in Huntington’s disease (HD). Increasing evidence suggests that such dysfunction not only impairs quality of life and exacerbates symptoms but may even accelerate the underlying disease process. Despite this, current HD treatment approaches neither consider the impact of commonly used medications on sleep, nor directly tackle sleep dysfunction. In this review, we discuss approaches to these two areas, evaluating not only literature from clinical studies in HD, but also that from parallel neurodegenerative conditions and preclinical models of HD. We conclude by summarizing a hierarchical framework of current medications with regard to their impact on sleep, and by outlining key emerging sleep therapies.
INTRODUCTION
Huntington’s disease (HD) is an autosomal-dominant hereditary neurodegenerative condition, characterized by a triad of motor, cognitive and psychiatric features, resulting from a polyglutamine expansion mutation in exon 1 of the huntingtin gene. As there is currently no treatment that slows or halts the progression of the disease, contemporary treatment approaches are symptomatic.
Sleep dysfunction represents one such highly prevalent symptom. On a clinical level, this manifests most commonly as onset and maintenance insomnia, as well as abnormal motor activity during sleep and nocturnal awakenings [1–5]. On a polysomnographic level, such dysfunction results in sleep structure fragmentation [6, 7] (Fig. 1) and loss of slow wave sleep (SWS) and rapid eye movement (REM) sleep. Notably, these sleep deficits have been identified during the premanifest stage far from disease onset [2, 7, 8], arguing against the idea that sleep dysfunction occurs predominantly as a result of medication or psychiatric symptoms of HD such as depression or anxiety.
Fig. 1
Polysomnography exhibiting insomnia and sleep architecture fragmentation in HD, sourced from ongoing research in our group (unpublished). Figures depict hypnograms from two control examples and two early manifest HD patient examples, between 22:00 and 07:00. U, unscored; W, wake; R, rapid eye movement (red); N1, stage 1 sleep; N2, stage 2 sleep; N3, slow wave sleep.
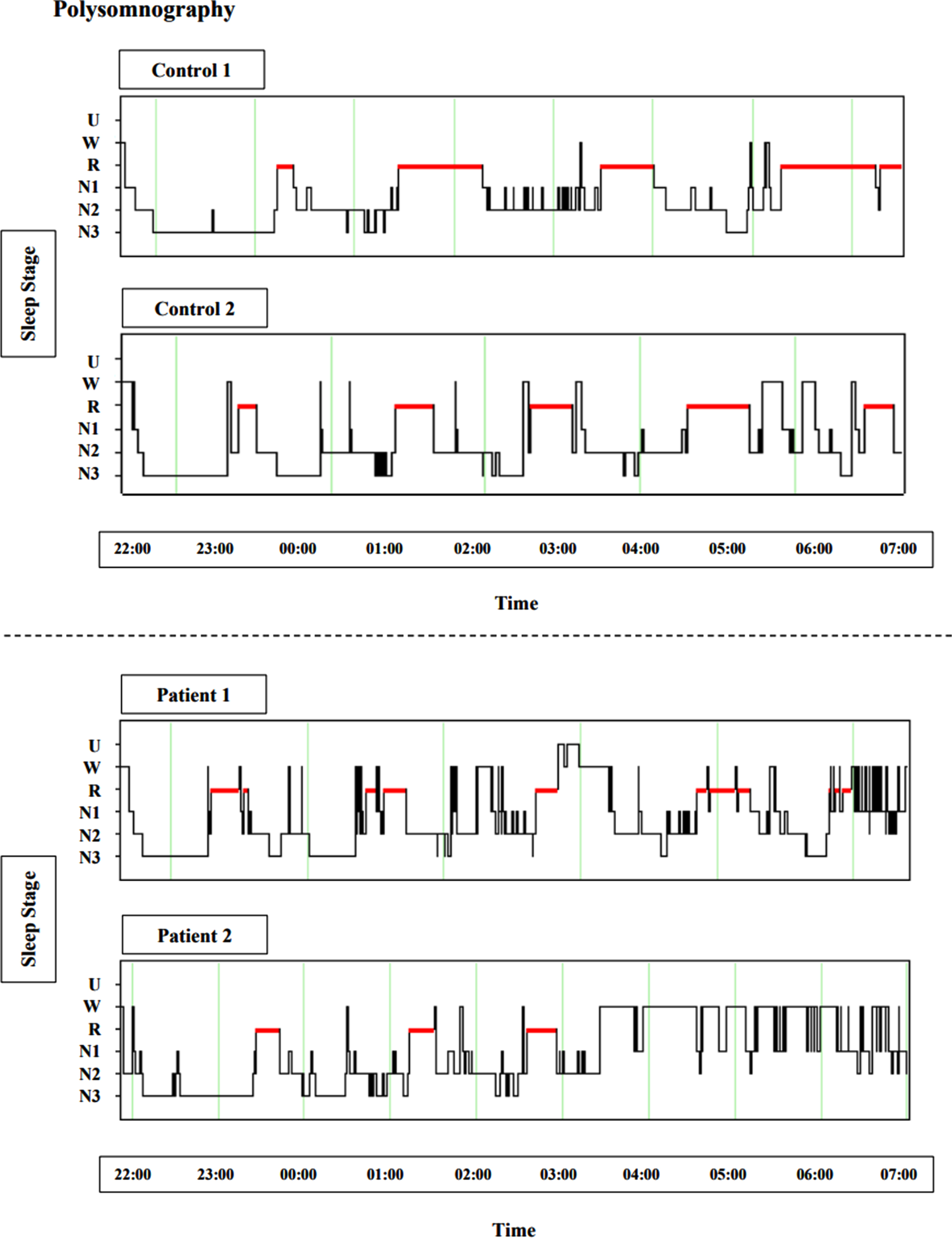
Importantly, growing evidence suggests a damaging feedforward cycle between such sleep dysfunction and neurodegeneration. Sleep dysfunction is recognized to exacerbate a range of cognitive symptoms, including deficits in executive function, memory consolidation, attention, and processing speed, as well as affective features such as impulsivity and emotional liability [9–13]. Sleep disruption may also directly affect neurodegenerative processes by inducing neuroinflammation [14] or impairing SWS-dependent glymphatic clearance of neurotoxic waste [15, 16]. Consequently, sleep deprivation is associated with an increase in neurotoxic proteins such as amyloid-β and tau; known contributors of neurodegeneration [16, 17]. This is also relevant to HD given the recent links to tau and disease progression [18].
Given this feedforward relationship, addressing sleep pathology in HD potentially offers a treatment approach that not only could alleviate motor, cognitive, and psychiatric symptoms but may also modify disease progression. Consistent with this, sleep interventions in preclinical models of HD have enhanced both behavioral and survival outcomes [19–24]. Thus, there exists a clear need for i) greater consideration of how commonly prescribed HD medications impact sleep in this condition, and ii) identification of the best approaches for treating such sleep dysfunction. This review aims to discuss these two areas (Table 1).
Table 1
Sleep-Related Effects of Treatments Relevant to HD
Treatment | Populations Trialed | Mechanism of Action | Reported Sleep Benefits | Reported Adverse Events |
Pharmacological | ||||
Tetrabenazine | HD, hyperkinetic movement disorders | VMAT2 inhibitor | Unknown | Insomnia, depression, daytime somnolence |
Deutetrabenazine | HD, hyperkinetic movement disorders | VMAT2 inhibitor | Unknown | Daytime somnolence (lower rates than with tetrabenazine) |
Atypical Antipsychotics Olanzapine Risperidone Quetiapine | HD, depression, schizophrenia, healthy | Serotonin-dopamine (D2) antagonist | ↑ TST ↑ SE ↑ SWS proportion ↓ Insomnia | Cognitive impairment, hip fracture, CVA, death |
SSRIs/SNRIs | Depression | Selective serotonin/norepinephrine reuptake inhibitor | Unknown | Insomnia, ↓ sleep continuity, ↓ REM sleep, ↑ REM latency |
Sedating Antidepressants Mirtazapine Trazodone Doxepin | Primary insomnia, depression, healthy | Antagonism at 5HT2 receptor | ↑ TST ↑ SE ↑ SWS proportion | Daytime somnolence, cognitive impairment |
Benzodiazepines/Z-drugs | HD (preclinical), AD, healthy | GABA agonist | ↓ sleep latency ↑ sleep continuity | ↓ REM, ↓ SWS, tolerance, dependance, withdrawal, cognitive impairment |
Lamotrigine | Epilepsy | Glutamate-release inhibitor | Inconclusive (potential ↑ REM) | Inconclusive (potential insomnia and ↓ SWS) |
Amantadine | HD, PD | Likely NMDA receptors | Unknown | Insomnia |
Riluzole | HD | Glutamate antagonist | ↓ insomnia (limited evidence) | Insignificant |
Exogenous Melatonin | AD, PD, MCI | Circadian rhythm | Inconclusive | None |
Sodium Oxybate | PD, healthy | GABA receptor agonist | ↑ SWS duration | None |
Orexin Antagonists Suvorexant Lemborexant | AD, healthy | Orexin inhibitors | ↑ TST ↓ WASO | None |
Nonpharmacological | ||||
CBT-I | HD (preclinical and premanifest), mixed dementia, MCI, AD, PD, healthy | Behavioral measures | ↑ SE ↑ SWS duration | None |
Bright Light Therapy | HD (preclinical), dementia | Circadian rhythm | Inconclusive | None |
Acoustic Stimulation | MCI, healthy | Nonlemniscal acoustic pathway and thalamocortical projections | ↑ SWS | Long-term effects unknown |
TST, total sleep time; SE, sleep efficiency; SWS, slow wave sleep; WASO, waketime after sleep onset; ↑, increase; ↓, decrease; HD, Huntington’s disease; PD, Parkinson’s disease; AD, Alzheimer’s disease; MCI, mild cognitive impairment; CVA, cerebrovascular accident.
CURRENT MEDICATIONS: IMPACT ON SLEEP
Tetrabenazine/deutetrabenazine
Tetrabenazine and deutetrabenazine are the most commonly used antidopaminergic agents to treat chorea associated with HD. Both are vesicular monoamine transporter 2 (VMAT2) inhibitors that block the loading of dopamine into presynaptic vesicles, thereby reducing dopamine release and, consequently, lessening chorea. Currently, tetrabenazine and deutetrabenazine are the only agents approved by the US Food and Drug Administration for the pharmacological treatment of the chorea in HD.
Multiple studies of tetrabenazine (TBZ) in hyperkinetic movement disorders demonstrate that it elicits insomnia in 5– 33% of patients [25–30]. Moreover, studies have shown that TBZ also carries a risk of causing depression in 8– 37% of patients [26–29, 31]. Given the potential for depression to provoke or exacerbate insomnia [32], this further adds to the risk of this agent mediating sleep disruption in HD. There is also evidence that TBZ may influence daytime alertness: multiple studies have noted drowsiness or somnolence as occurring in 25– 39% of patients treated with tetrabenazine [25, 26, 28, 30], and in a 12-week randomized controlled trial conducted by the Huntington Study Group, the treatment group receiving tetrabenazine experienced a significant dose dependent increase in drowsiness and insomnia compared to the control group [33].
In contrast, studies of deutetrabenazine in hyperkinetic movement disorders have either shown no, rare, or insignificant levels of insomnia as an adverse event [34], as well as low rates of depression [34–36]. A randomized clinical trial of deutetrabenazine conducted by the Huntington Study Group cited somnolence as reported by 11% of patients in the treatment group [34], but there was no significant difference in Epworth Sleepiness Scale (ESS) scores. Other studies also report somnolence as the most common adverse event in patients treated with deutetrabenazine, but at lower rates (5– 15%) than those observed for tetrabenazine [35–37]. Moreover, a direct head-to-head study found a significantly lower risk of adverse events, including depression, drowsiness/somnolence, and insomnia, associated with deutetrabenazine compared to tetrabenazine [38].
Antipsychotics
Dopamine receptor antagonists, such as typical and atypical antipsychotics, are used to treat both psychiatric symptoms and chorea in HD patients.
Unlike VMAT2 inhibitors, antipsychotics exhibit a relatively favorable sleep profile. Olanzapine has been shown to improve behavioral symptoms and chorea in HD patients with no significant adverse effects related to sleep disturbance [39–41]. Polysomnographic (PSG) studies in healthy individuals, and in those with depression or schizophrenia, have shown increases in total sleep time, sleep efficiency and proportion of slow wave sleep (SWS) in response to atypical antipsychotic therapy [42–47]. These studies all investigated olanzapine, however acute administration with other atypical antipsychotics, such as risperidone [48, 49] and quetiapine [50, 51], are also associated with increases in total sleep time, percentage of SWS and improved sleep efficiency in healthy populations as well as in subjects with depression. Such an effect on SWS is particularly notable, given the specific loss of SWS in HD, and the role of SWS in glymphatic clearance of neurotoxic waste and memory consolidation [15, 52–54]. Though there are no PSG studies on the effects of antipsychotics specifically in HD, several studies using clinical outcome measures have shown improvements in insomnia [55, 56]. Typical antipsychotics, such as haloperidol, have been associated with sedation and somnolence side effects, while other studies have demonstrated that atypical antipsychotics tend to have less of a sedative effect without compromising efficacy [57].
Thus, atypical antipsychotics represent a comparatively sleep-benign option in the treatment of chorea in HD. Nonetheless, this benefit needs to be offset against data from the broader dementia literature that suggest antipsychotics carry an increased risk of hip fracture, cognitive decline, cerebrovascular accident, and death [58–61], which may also be the case for HD [62].
Antidepressants
Due to the bidirectional relationship between sleep loss and depression [63], pharmacological treatment of depression bears potential to improve sleep quality. Indeed, sleep and depression have been notably tightly bound in HD, with sleep disturbances occurring more prevalently in HD patients with coexisting depression [64, 65].
However, on a pharmacological level, both SSRIs and SNRIs have been found to carry a risk of eliciting insomnia, with clinical trials showing treatment-emergent insomnia in 17% of patients treated with SSRIs compared to 9% in the placebo group and in 13% of patients treated with SNRIs compared to 7% on placebo [66]. Moreover, regarding sleep architecture, both SSRIs and SNRIs have been found to reduce sleep continuity and REM sleep as well as increase REM latency [67]. Such adverse events are greatest during the first weeks of treatment but may persist.
Given this sleep-disrupting effect observed with SSRIs and SNRIs, sedating antidepressants, such as mirtazapine, amitriptyline, trazodone, and doxepin, are a logical consideration. Mirtazapine, in particular, has been shown to improve sleep continuity and SWS via 5HT2 receptor antagonism in both healthy individuals and those with depression [68–73]. Increases in total sleep time and SWS have similarly been observed in studies of trazodone as a treatment for primary insomnia and co-occurring depression and insomnia as well as in healthy individuals [74, 75]. Doxepin has also been shown to increase total sleep time and overall sleep efficiency in young, middle-aged, and elderly primary insomnia patients [76–78].
Nonetheless, a randomized controlled trial of mirtazapine in Alzheimer’s disease found no significant benefit to sleep outcomes [79], and the majority of sedating antidepressants have been found to carry a risk of significant daytime somnolence and cognitive impairment, with consequent adverse outcomes [58, 75, 80]. The average prevalence of somnolence from the US Food and Drug Administration study register was 54% in patients treated with mirtazapine compared to 18% in placebo-treated patients and 46% in patients treated with trazodone compared to 19% in placebo. This is compared to reports of somnolence in only 16% of SSRI-treated patients and 8% in the placebo arm and 10% of SNRI-treated patients with 5% in the placebo group [66].
Benzodiazepines and Z-drugs
Benzodiazepines and Z-drugs (e.g., zopiclone, zolpidem, and zaleplon) both act as GABA agonists. Such agents are commonly prescribed to address sleep symptoms in HD patients and the general population, due to their known ability to reduce sleep latency and improve sleep continuity.
Studies in preclinical models of HD have shown cognitive benefits through the pharmacological normalization of sleep-wake cycles with a benzodiazepine [19]. However, this is unlikely to translate to clinical populations: both benzodiazepines and Z-drugs carry risks of tolerance, dependence, and withdrawal [81] as well as impairment of attention, psychomotor speed, and memory consolidation [82–87]. Additionally, reductions of REM and SWS have been observed in both benzodiazepine and Z-drug studies of healthy young, adult, and elderly populations [88–91]. Studies in healthy elderly populations and in Alzheimer’s disease have shown worse outcomes with benzodiazepine use [92–94]. Given these outcomes and the risk of side effects, benzodiazepines and Z-drugs are not recommended as pharmacological agents in the treatment of sleep dysfunction in HD.
Other agents
Lamotrigine
Lamotrigine, a mood stabilizer that acts by reducing the release of glutamate, may be used to address issues of mood lability and motor symptoms in HD patients [95, 96]. There has as yet, been no direct study of the influence of lamotrigine on sleep in HD. Symptomatic studies in epilepsy have suggested lamotrigine may cause insomnia in 6– 19% of patients [97, 98]. PSG studies investigating lamotrigine in the treatment of epilepsy have been inconsistent, with some reporting loss of SWS [99] while others report a significant gain in REM sleep [100].
Amantadine
Amantadine has been used to treat chorea and balance impairment in HD. Though its mechanism of action is not fully known, amantadine is thought to work at the level of the striatum on dopamine via NMDA receptors. In a blinded, randomized controlled trial of 24 HD patients, 25% experienced subjective insomnia during the treatment phase, compared to 12.5% when on placebo [101]. However, rates of insomnia and somnolence were insignificant or nonexistent in other randomized controlled trials of amantadine in both Huntington’s and Parkinson’s disease patients [102, 103].
Riluzole
Riluzole, a benzothiazole that reduces glutamate release, has been reported to improve chorea associated with HD without significant sleep-related adverse events in one study [104]. A three-year randomized controlled study of riluzole in HD found lower insomnia frequency in the treatment group compared to placebo [105].
PROSPECTS FOR TREATMENT
To date, there has been no large-scale sleep intervention study in a clinical HD population. However, positive results from preclinical studies, as well as clinical studies in other neurodegenerative conditions, merit consideration in guiding prospects for treatment.
Treating abnormal motor activity in sleep
Several studies report finding an increased prevalence of a periodic limb movement disorder (PLMD) in HD [1, 2]. Where suspected, rationalizing any tricyclic or SSRI antidepressant use may therefore be warranted, alongside consideration of gabapentin or clonazepam, taking into account the caveats discussed above. However, there is considerable controversy as to the nature of abnormal nocturnal motor activity in HD, with several studies instead identifying nocturnal motor activity as chorea, repetitive ballistic movements and atypical high amplitude repositioning movements during arousals to wakefulness [3–5]. As such, where a patient or their bed partner reports abnormal nocturnal motor activity which does not respond to olanzapine, referral for dedicated video polysomnography may be worthwhile.
Cognitive behavioral therapy for insomnia
Cognitive behavioral therapy for insomnia (CBT-I) employs a combination of behavioral measures to improve sleep quality, including stimulus control, sleep restriction, sleep hygiene, cognitive therapy, and relaxation techniques. As an example, sleep hygiene involves adherence to regular sleep/wake times and optimization of bedroom temperature, among other factors.
Strong evidence exists for the efficacy of CBT-I and sleep hygiene behavioral measures in improving sleep quality in healthy populations [106]. A randomized controlled trial in older adults with chronic primary insomnia found greater improvements in sleep efficiency and duration of SWS associated with CBT-I compared to zopiclone [107]. Supportive evidence also exists for improved sleep efficiency in mixed dementia populations and in patients with mild cognitive impairment, Alzheimer’s disease, and Parkinson’s disease [108–115].
Of note, CBT-I-like behavioral interventions in preclinical models of HD have been beneficial, demonstrating cognitive improvements [51]. Moreover, though limited, a pilot study of CBT-I in premanifest HD patients along with exercise (aerobic and resistance training) and social measures (social events and group intervention sessions) showed gains in REM sleep [116].
However, several elements of CBT-I are clearly impractical in advanced dementia, and attrition rates have been reported to be as high as 40% in community studies [117]. Thus, the efficacy and feasibility of long-term CBT-I to treat sleep dysfunction in HD remains ambiguous and is likely to be of most use in addressing sleep dysfunction present in the premanifest or prodromal phase of the condition.
Bright light therapy
Bright light therapy is known to modulate both circadian rhythm and sleep quality through its influence on melatonin secretion.
Notably, preclinical models of HD have suggested a possible benefit of bright light therapy to motor performance and survival outcomes [21, 22], raising hopes of translation to clinical HD populations. Some clinical studies have found evidence of benefits in dementia cohorts, reporting increased total sleep time and reduced wake time after sleep onset [118–120]. However, many of these studies are marred by a likely placebo effect [121, 122]. Overall, meta-analyses have not suggested high efficacy in dementia populations [123–126]. As with CBT-I, there are also clear practical considerations limiting use of bright light therapy in dementia and other psychiatric populations, as non-adherence to the treatment protocol is typically high [127], as was also evident in our own pilot study of bright light therapy in HD (A. Lazar and R. A. Barker, 2023, personal communication).
Melatonin
Allied to bright light therapy, direct exogenous melatonin has been widely studied as an alternative pharmacological treatment for sleep dysfunction. It is of particular relevance to Huntington’s disease, since a diminished melatonin secretion profile has been recognized as part of the condition [128, 129].
However, the evidence for the beneficial effect of melatonin on sleep dysfunction in neurodegenerative populations remains inconclusive. Multiple studies in both Alzheimer’s and Parkinson’s disease have failed to consistently show that melatonin has a beneficial effect on sleep quality. Evidence from a randomized controlled trial in Alzheimer’s disease patients [130], and a blinded cross-over study in individuals with mild cognitive impairment [131] suggested improvement in sleep quality with exogenous melatonin. However, in contrast, three other randomized controlled trials in Alzheimer’s disease have shown no benefit [132–134]. Similarly, no clinically significant benefits have been reported from placebo-controlled trials in Parkinson’s disease [135, 136].
Though no studies to date have directly investigated the use of melatonin as a pharmacological intervention to treat sleep abnormalities in Huntington’s disease, an ongoing pilot blinded clinical trial is examining the effects of melatonin as a dietary supplement in improving sleep quality in Huntington’s disease patients (https://clinicaltrials.gov/ct2/show/NCT04421339).
Sodium oxybate
Typically used to treat narcolepsy, sodium oxybate is thought to act through GABA receptor activity to reduce cataplexy and daytime somnolence. A number of studies have demonstrated parallel beneficial effects on slow wave sleep, and an ability to counter the cognitive impact of sleep loss in healthy adults [137]. Two studies in Parkinson’s disease have supported the efficacy of sodium oxybate in improving both subjective sleep quality and objective slow wave sleep duration [138, 139]. Moreover, animal models of neurodegeneration investigating sodium oxybate observed a protective action against toxic proteinopathies [140]. Such promising results position sodium oxybate as an important potential pharmacological treatment for sleep dysfunction in neurodegenerative disorders. However, sodium oxybate has not yet been directly trialed as a pharmacological agent in the treatment of Alzheimer’s disease or Huntington’s disease, and its use is not straightforward due to its classification as a controlled substance.
Acoustic stimulation
There is robust evidence supporting the ability of pulsed acoustic stimulation during sleep to augment slow wave sleep and enhance memory consolidation [141, 142]. While it may seem improbable that pulses of sound alone could have such an effect, this has been demonstrated in numerous blinded studies and is thought to be mediated by the unique interaction between the nonlemniscal acoustic pathway and diffuse thalamocortical projections during sleep, wherein acoustic stimuli evoke widespread synchronous slow oscillations that underpin slow wave sleep.
Such benefits of acoustic stimulation to slow wave sleep and cognition have been demonstrated in healthy populations including elderly cohorts [143–149]. To date, there has only been one study in a neurodegenerative cohort, that of mild cognitive impairment, in which an increase in slow wave activity was observed and associated with improving memory performance [150].
Acoustic stimulation, for example delivered by headset devices such as the Philips Deep Sleep Headband, therefore represents a further novel therapy for HD, with its non-pharmacological and non-invasive method of delivery, practicality in the home, and low cost all making it an attractive option. However, the long-term effects of acoustic stimulation currently remain unknown, and no clinical trial has yet been undertaken in an HD cohort.
Orexin antagonists
Orexin antagonists, such as suvorexant and lemborexant, represent a further emerging area of sleep therapies. They are designed to treat insomnia through their inhibition of orexin, a neuropeptide that acts as a key alerting agent in the regulation of the sleep-wake cycle.
Several randomized clinical trials offer support for the use of orexin antagonists in both healthy older adults and in Alzheimer’s disease patients to treat insomnia, demonstrating increases in total sleep time as well as a reduction in wake time after sleep onset [151–154]. One study comparing an orexin antagonist and zolpidem in young healthy adults found less cognitive and executive impairments under the orexin antagonist [155]. Moreover, orexin antagonists do not appear to have any detrimental effect on sleep architecture and seem, overall, to be well tolerated [151, 153, 156]. Though no clinical trials have yet been conducted in HD, importantly, preclinical data has supported the potential benefit of orexin antagonists in addressing sleep dysfunction [24].
CONCLUSION
Addressing sleep dysfunction in HD bears potential to improve clinical outcomes and overall quality of life for patients and their families. There is a pressing need for greater consideration of the impact on sleep when managing patients with HD, including the possible detrimental effects of drugs used to treat other aspects of HD (e.g., chorea) on sleep.
Of commonly used medications, current evidence suggests that atypical antipsychotics and sedating antidepressants carry the most favorable profile with respect to supporting improved and healthy sleep. However, in both cases this needs to be offset against their risk of eliciting daytime somnolence and consequent cognitive impairment, falls, and other adverse events. In terms of VMAT inhibitors, deutetrabenazine appears to carry a more sleep-benign profile when compared against tetrabenazine. Limited evidence exists with respect to the effect of lamotrigine, amantadine and riluzole on sleep profiles, but so far the data suggest that only riluzole may have benefits for sleep. Benzodiazepines and Z-drugs are not recommended, both due to their detrimental effect on sleep architecture and associated adverse outcomes.
With respect to direct sleep therapies, clinical trials in parallel neurodegenerative conditions have, to date, largely failed to demonstrate benefit from bright light therapy or exogenous melatonin. Nevertheless, the results of a pilot trial of melatonin in an HD cohort are awaited. Supportive evidence exists for the benefit of CBT-I, both from preclinical models of HD and clinical studies in other neurodegenerative conditions. Nonetheless, practical considerations are clearly paramount in such an intervention in an HD cohort, such that it is likely that CBT-I would be most efficacious in premanifest or prodromal HD patients. By contrast, sodium oxybate, orexin antagonists and slow wave sleep augmentation by acoustic stimulation are all emerging as promisingly efficacious and practical new sleep therapies, and clinical trials of these agents in HD should be undertaken.
ACKNOWLEDGMENTS
Zanna Voysey is funded by an Association of British Neurologists/Guarantors of Brain Clinical Research Fellowship. This research was supported by the NIHR Cambridge Biomedical Research Centre (NIHR203312). The views expressed are those of the authors and not necessarily those of the NIHR or the Department of Health and Social Care.
CONFLICT OF INTEREST
The authors have no conflict of interest to report.
REFERENCES
[1] | Piano C , Losurdo A , Della Marca G , Solito M , Calandra-Buonaura G , Provini F , et al. Polysomnographic findings and clinical correlates in Huntington disease: A cross-sectional cohort study. Sleep. (2015) ;38: (9):1489–95. |
[2] | Arnulf I , Nielsen J , Lohmann E , Schieffer J , Wild E , Jennum P , et al. Rapid eye movement sleep disturbances in Huntington disease. Archives of Neurology. (2008) ;65: (4):482–8. |
[3] | Neutel D , Tchikviladzé M , Charles P , Leu-Semenescu S , Roze E , Durr A , et al. Nocturnal agitation in Huntington disease is caused by arousal-related abnormal movements rather than by rapid eye movement sleep behavior disorder. Sleep Med. (2015) ;16: (6):754–9. |
[4] | Ranjan S , Kohler S , Harrison MB , Quigg M Nocturnal post-arousal chorea and repetitive ballistic movement in Huntington’s disease. Mov Disord Clin Pract. (2016) ;3: (2):200. |
[5] | Fish DR , Sawyers D , Allen PJ , Blackie JD , Lees AJ , Marsden CD The effect of sleep on the dyskinetic movements of Parkinson’s disease, Gilles de la Tourette syndrome, Huntington’s disease, and torsion dystonia. Arch Neurol. (1991) ;48: (2):210–4. |
[6] | Zhang Y , Ren R , Yang L , Zhou J , Li Y , Shi J , et al. Sleep in Huntington’s disease: A systematic review and meta-analysis of polysomnographic findings. Sleep. (2019) ;42: (10):zsz154. |
[7] | Lazar AS , Panin F , Goodman AO , Lazic SE , Lazar ZI , Mason SL , et al. Sleep deficits but no metabolic deficits in premanifest Huntington’s disease. Ann Neurol. (2015) ;78: (4):630–48. |
[8] | Goodman AO , Rogers L , Pilsworth S , McAllister CJ , Shneerson JM , Morton AJ , et al. Asymptomatic sleep abnormalities are a common early feature in patients with Huntington’s disease. Curr Neurol Neurosci Rep. (2011) ;11: :211–7. |
[9] | Alhola P , Polo-Kantola P Sleep deprivation: Impact on cognitive performance. Neuropsychiatr Dis Treat. (2007) ;3: (5):553–67. |
[10] | Aidman E , Jackson SA , Kleitman S Effects of sleep deprivation on executive functioning, cognitive abilities, metacognitive confidence, and decision making. Appl Cogn Psychol. (2019) ;33: (2):188–200. |
[11] | Chua EC , Yeo SC , Lee IT , Tan LC , Lau P , Cai S , et al. Sustained attention performance during sleep deprivation associates with instability in behavior and physiologic measures at baseline. Sleep. (2014) ;37: (1):27–39. |
[12] | Beattie L , Kyle SD , Espie CA , Biello SM Social interactions, emotion and sleep: A systematic review and research agenda. Sleep Med Rev. (2015) ;24: :83–100. |
[13] | Groeger JA , Lo J , Santhi N , Lazar AS , Dijk DJ Contrasting effects of sleep restriction, total sleep deprivation, and sleep timing on positive and negative affect. Front Behav Neurosci. (2022) :268. |
[14] | Manchanda S , Singh H , Kaur T , Kaur G Low-grade neuroinflammation due to chronic sleep deprivation results in anxiety and learning and memory impairments. Mol Cell Biochem. (2018) ;449: :63–72. |
[15] | Xie L , Kang H , Xu Q , Chen MJ , Liao Y , Thiyagarajan M , et al. Sleep drives metabolite clearance from the adult brain. Science. (2013) ;342: (6156):373–7. |
[16] | Holth JK , Fritschi SK , Wang C , Pedersen NP , Cirrito JR , Mahan TE , et al. The sleep-wake cycle regulates brain interstitial fluid tau in mice and CSF tau in humans. Science. (2019) ;363: (6429):880–4. |
[17] | Rothman SM , Herdener N , Frankola KA , Mughal MR , Mattson MP Chronic mild sleep restriction accentuates contextual memory impairments, and accumulations of cortical Aβ and pTau in a mouse model of Alzheimer’s disease. Brain Res. (2013) ;15298: :200–8. |
[18] | Vuono R , Winder-Rhodes S , De Silva R , Cisbani G , Drouin-Ouellet J , Spillantini MG , et al. The role of tau in the pathological process and clinical expression of Huntington’s disease. Brain. (2015) ;138: (7):1907–18. |
[19] | Pallier PN , Maywood ES , Zheng Z , Chesham JE , Inyushkin AN , Dyball R , et al. Pharmacological imposition of sleep slows cognitive decline and reverses dysregulation of circadian gene expression in a transgenic mouse model of Huntington’s disease. J Neurosci. (2007) ;27: (29):7869–78. |
[20] | Pallier PN , Morton AJ Management of sleep/wake cycles improves cognitive function in a transgenic mouse model of Huntington’s disease. Brain Res. (2009) ;1279: :90–8. |
[21] | Wang HB , Whittaker DS , Truong D , Mulji AK , Ghiani CA , Loh DH , et al. Blue light therapy improves circadian dysfunction as well as motor symptoms in two mouse models of Huntington’s disease. Neurobiol Sleep Circadian Rhythms. (2017) ;2: :39–52. |
[22] | Ouk K , Aungier J , Morton AJ Prolonged day length exposure improves circadian deficits and survival in a transgenic mouse model of Huntington’s disease. Neurobiol Sleep Circadian Rhythms. (2017) ;2: :27–38. |
[23] | Whittaker DS , Loh DH , Wang HB , Tahara Y , Kuljis D , Cutler T , et al. Circadian-based treatment strategy effective in the BACHD mouse model of Huntington’s disease. J Biol Rhythms. (2018) ;33: (5):535–54. |
[24] | Cabanas M , Pistono C , Puygrenier L , Rakesh D , Jeantet Y , Garret M , et al. Neurophysiological and behavioral effects of anti-orexinergic treatments in a mouse model of Huntington’s disease. Neurotherapeutics. (2019) ;16: (3):784–96. |
[25] | Jankovic J , Beach J Long-term effects of tetrabenazine in hyperkinetic movement disorders. Neurology. (1997) ;48: (2):358–62. |
[26] | Shen V , Clarence-Smith K , Hunter C , Jankovic J Safety and efficacy of tetrabenazine and use of concomitant medications during long-term, open-label treatment of chorea associated with Huntington’s and other diseases. Tremor Other Hyperkinet Mov (N Y). (2013) ;3. |
[27] | Frank S Tetrabenazine as anti-chorea therapy in Huntington disease: An open-label continuation study. Huntington Study Group/TETRA-HD Investigators. BMC Neurol. (2009) ;9: (1):62. |
[28] | Kenney C , Jankovic J Tetrabenazine in the treatment of hyperkinetic movement disorders. Expert Rev Neurothera. (2006) ;6: (1):7–17. |
[29] | Scott LJ Tetrabenazine: For chorea associated with Huntington’s disease. CNS Drugs. (2011) ;25: :1073–85. |
[30] | Fasano A , Cadeddu F , Guidubaldi A , Piano C , Soleti F , Zinzi P , et al. The long-term effect of tetrabenazine in the management of Huntington disease. Clin Neuropharmacol. (2008) ;31: (6):313–8. |
[31] | Jankovic J , Orman J Tetrabenazine therapy of dystonia, chorea, tics, and other dyskinesias. Neurology. (1988) ;38: (3):391–4. |
[32] | Franzen PL , Buysse DJ Sleep disturbances and depression: Risk relationships for subsequent depression and therapeutic implications. Dialogues Clin Neurosci. (2008) ;10: (4):473–81. |
[33] | Huntington Study Group. Tetrabenazine as antichorea therapy in Huntington disease: A randomized controlled trial. Neurology. (2006) ;66: (3):366–72. |
[34] | Frank S , Testa CM , Stamler D , Kayson E , Davis C , Edmondson MC , et al. Effect of deutetrabenazine on chorea among patients with Huntington disease: A randomized clinical trial. JAMA. (2016) ;316: (1):40–50. |
[35] | Coffey B , Jankovic J , Claassen DO , Jimenez-Shahed J , Gertz BJ , Garofalo EA , et al. Efficacy and safety of fixed-dose deutetrabenazine in children and adolescents for tics associated with Tourette syndrome: A randomized clinical trial. JAMA Netw Open. (2021) ;4: (10):e2129397. |
[36] | Jankovic J , Coffey B , Claassen DO , Jimenez-Shahed J , Gertz BJ , Garofalo EA , et al. Safety and efficacy of flexible-dose deutetrabenazine in children and adolescents with Tourette syndrome: A randomized clinical trial. JAMA Netw Open. (2021) ;4: (10):e2128204. |
[37] | Fernandez HH , Stamler D , Davis MD , Factor SA , Hauser RA , Jimenez-Shahed J , et al. Long-term safety and efficacy of deutetrabenazine for the treatment of tardive dyskinesia. J Neurol Neurosurg Psychiatry.. (2019) ;90: (12):1317–23. |
[38] | Claassen DO , Carroll B , De Boer LM , Wu E , Ayyagari R , Gandhi S , et al. Indirect tolerability comparison of Deutetrabenazine and Tetrabenazine for Huntington disease. J Clin Mov Disord. (2017) ;4: :3. |
[39] | Bonelli RM , Mahnert FA , Niederwieser G Olanzapine for Huntington’s disease: An open label study. Clin Neuropharmacol. (2002) ;25: (5):263–5. |
[40] | Paleacu D , Anca M , Giladi N Olanzapine in Huntington’s disease. Acta Neurol Scand. (2002) ;105: (6):441–4. |
[41] | Grove VE Jr , Quintanilla J , DeVaney GT Improvement of Huntington’s disease with olanzapine and valproate. N Engl J Med. (2000) ;343: (13):973–4. |
[42] | Lindberg N , Virkkunen M , Tani P , Appelberg B , Virkkala J , Rimon R , et al. Effect of a single-dose of olanzapine on sleep in healthy females and males. Int Clin Psychopharmacol. (2002) ;17: (4):177–84. |
[43] | Sharpley AL , Vassallo CM , Cowen PJ Olanzapine increases slow-wave sleep: Evidence for blockade of central 5-HT2C receptors in vivo. Biol Psychiatry. (2000) ;47: (5), 468–70. |
[44] | Sharpley AL , Attenburrow ME , Hafizi S , Cowen PJ Olanzapine increases slow wave sleep and sleep continuity in SSRI-resistant depressed patients. J Clin Psychiatry. (2005) ;66: (4):450–4. |
[45] | Lazowski LK , Townsend B , Hawken ER , Jokic R , du Toit R , Milev R Sleep architecture and cognitive changes in olanzapine-treated patients with depression: A double blind randomized placebo controlled trial. BMC Psychiatry. (2014) ;14: :202. |
[46] | Salin-Pascual RJ , Herrera-Estrella M , Galicia-Polo L , Laurrabaquio MR Olanzapine acute administration in schizophrenic patients increases delta sleep and sleep efficiency. Biol Psychiatry. (1999) ;46: (1):141–3. |
[47] | Katshu MZ , Sarkar S , Nizamie SH Effect of olanzapine on clinical and polysomnography profiles in patients with schizophrenia. Schizophr Res Treatment. (2018) ;2018: :3968015. |
[48] | Yoshimura A , Matsuo M , Imai M , Yamada N , Okawa M Low-dose oral risperidone lengthened sleep duration in healthy participants. Sleep Biol Rhythms. (2007) ;5: :277–83. |
[49] | Matsumoto T , Mizuno S , Tsukada J , Inami Y , Horiguchi J Effect of risperidone on polysomnography in healthy subjects. Sleep Biol Rhythms. (2007) ;5: (2):146–8. |
[50] | Cohrs S , Rodenbeck A , Guan Z , Pohlmann K , Jordan W , Meier A , et al. Sleep-promoting properties of quetiapine in healthy subjects. Psychopharmacology. (2004) ;174: :421–9. |
[51] | Gedge L , Lazowski L , Murray D , Jokic R , Milev R Effects of quetiapine on sleep architecture in patients with unipolar or bipolar depression. Neuropsychiatr Dis Treat. (2010) :501–8. |
[52] | Fultz NE , Bonmassar G , Setsompop K , Stickgold RA , Rosen BR , Polimeni JR , et al. Coupled electrophysiological, hemodynamic, and cerebrospinal fluid oscillations in human sleeScience. (2019) ;366(6465):628–31. |
[53] | Rasch B , Born J About sleep’s role in memory. Physiol Rev. (2013) ;93: (2):681–766. |
[54] | Todorova R , Zugaro M Isolated cortical computations during delta waves support memory consolidation. Science. (2019) . 366: (6463):377–81. |
[55] | Alpay M , Koroshetz WJ Quetiapine in the treatment of behavioral disturbances in patients with Huntington’s disease. Psychosomatics. (2006) ;47: (1):70–2. |
[56] | Ciammola A , Sassone J , Colciago C , Mencacci NE , Poletti B , Ciarmiello A , et al. Aripiprazole in the treatment of Huntington’s disease: A case series. Neuropsychiatr Dis Treat. (2009) ;5: :1–4. |
[57] | Miller DD Atypical antipsychotics: Sleep, sedation, and efficacy. Prim Care Companion J Clin Psychiatry. (2004) ;6: (Suppl 2), 3–7. |
[58] | Oderda LH , Young JR , Asche CV , Pepper GA Psychotropic-related hip fractures: Meta-analysis of first-generation and second-generation antidepressant and antipsychotic drugs. Ann Pharmacother. (2012) ;46: (7-8), 917–28. |
[59] | Mittal V , Kurup L , Williamson D , Muralee S , Tampi RR Risk of cerebrovascular adverse events and death in elderly patients with dementia when treated with antipsychotic medications: A literature review of evidence. Am J Alzheimers Dis Other Demen.. (2011) ;26: (1):10–28. |
[60] | Ballard C , Hanney ML , Theodoulou M , Douglas S , McShane R , Kossakowski K , et al. The dementia antipsychotic withdrawal trial (DART-AD): Long-term follow-up of a randomised placebo-controlled trial. Lancet Neurol. (2009) ;8: (2):151–7. |
[61] | Chiu Y , Bero L , Hessol NA , Lexchin J , Harrington C A literature review of clinical outcomes associated with antipsychotic medication use in North American nursing home residents. Health Policy. (2015) ;119: (6):802–13. |
[62] | Harris KL , Kuan WL , Mason SL , Barker RA Antidopaminergic treatment is associated with reduced chorea and irritability but impaired cognition in Huntington’s disease (Enroll-HD). J Neurol Neurosurg Psychiatry. (2020) ;91: (6):622–30. |
[63] | Fang H , Tu S , Sheng J , Shao A Depression in sleep disturbance: A review on a bidirectional relationship, mechanisms and treatment. J Cell Mol Med. (2019) ;23: (4):2324–32. |
[64] | Videnovic A , Leurgans S , Fan W , Jaglin J , Shannon KM Daytime somnolence and nocturnal sleep disturbances in Huntington disease. Parkinsonism Relat Disord. (2009) ;15: (6):471–4. |
[65] | Aziz NA , Anguelova GV , Marinus J , Lammers GJ , Roos RA Sleep and circadian rhythm alterations correlate with depression and cognitive impairment in Huntington’s disease. Parkinsonism Relat Disord. (2010) ;16: (5):345–50. |
[66] | Wichniak A , Wierzbicka A , Walȩcka M , Jernajczyk W Effects of antidepressants on sleep. Curr Psychiatry Rep. (2017) ;19: :1–7. |
[67] | Wilson S , Argyropoulos S Antidepressants and sleep: A qualitative review of the literature. Drugs. (2005) ;65: (7):927–47. |
[68] | Ruigt GS , Kemp B , Groenhout CM , Kamphuisen HA Effect of the antidepressant Org on human sleep. Eur J Clin Pharmacol. (1990) ;38: :551–4. |
[69] | Winokur A , Sateia MJ , Hayes JB , Bayles-Dazet W , MacDonald MM , Gary KA Acute effects of mirtazapine on sleep continuity and sleep architecture in depressed patients: A pilot study. Biol Psychiatry. (2000) ;48: (1):75–8. |
[70] | Winokur A , DeMartinis NA , McNally DP , Gary EM , Cormier JL , Gary KA Comparative effects of mirtazapine and fluoxetine on sleep physiology measures in patients with major depression and insomnia. J Clin Psychiatry. (2003) ;64: (10):1224–9. |
[71] | Aslan S , Isik E , Cosar B The effects of mirtazapine on sleep: A placebo controlled, double-blind study in young healthy volunteers. Sleep. (2002) ;25: (6):666–8. |
[72] | Schittecatte M , Dumont F , Machowski R , Cornil C , Lavergne F , Wilmotte J Effects of mirtazapine on sleep polygraphic variables in major depression. Neuropsychobiology. (2002) ;46: (4):197–201. |
[73] | Schmid DA , Wichniak A , Uhr M , Ising M , Brunner H , Held K , et al. Changes of sleep architecture, spectral composition of sleep EEG, the nocturnal secretion of cortisol, ACTH, GH, prolactin, melatonin, ghrelin, and leptin, and the DEX-CRH test in depressed patients during treatment with mirtazapine. Neuropsychopharmacology. (2006) ;31: (4):832–44. |
[74] | Yamadera H , Nakamura S , Suzuki H , ENDO S Effects of trazodone hydrochloride and imipramine on polysomnography in healthy subjects. Psychiatry Clin Neurosci. (1998) ;52: (4):439–43. |
[75] | Kaynak H , Kaynak D , Gözükırmızı E , Guilleminault C The effects of trazodone on sleep in patients treated with stimulant antidepressants. Sleep Med.. (2004) ;5: (1):15–20. |
[76] | Hajak G , Rodenbeck A , Voderholzer U , Riemann D , Cohrs S , Hohagen F , et al. Doxepin in the treatment of primary insomnia: A placebo-controlled, double-blind, polysomnographic study. J Clin Psychiatry. (2001) ;62: (6):453–63. |
[77] | Roth T , Rogowski R , Hull S , Schwartz H , Koshorek G , Corser B , et al. Efficacy and safety of doxepin 1mg, 3mg, and 6mg in adults with primary insomnia. Sleep. (2007) ;30: (11):1555–61. |
[78] | Scharf M , Rogowski R , Hull S , Cohn M , Mayleben D , Feldman N , et al. Efficacy and safety of doxepin 1mg, 3mg, and 6mg in elderly patients with primary insomnia: A randomized, double-blind, placebo-controlled crossover study. J Clin Psychiatry. (2008) ;69: (10):1557. |
[79] | Scoralick FM , Louzada LL , Quintas JL , Naves JO , Camargos EF , Nóbrega OT Mirtazapine does not improve sleep disorders in Alzheimer’s disease: Results from a double-blind, placebo-controlled pilot study. Psychogeriatrics. (2017) ;17: (2):89–96. |
[80] | Coupland C , Dhiman P , Morriss R , Arthur A , Barton G , Hippisley-Cox J Antidepressant use and risk of adverse outcomes in older people: Population based cohort study. BMJ. (2011) :343. |
[81] | George CF Pyrazolopyrimidines. Lancet. (2001) ;358: (9293):1623–6. |
[82] | Curran HV , Birch B Differentiating the sedative, psychomotor and amnesic effects of benzodiazepines: A study with midazolam and the benzodiazepine antagonist, flumazenil. Psychopharmacology. (1991) ;103: :519–23. |
[83] | Leufkens TRM , Lund JS , Vermeeren A Highway driving performance and cognitive functioning the morning after bedtime and middle-of-the-night use of gaboxadol, zopiclone and zolpidem. J Sleep Res. (2009) ;18: :387–96. |
[84] | Barker MJ , Greenwood KM , Jackson M , Crowe SF Cognitive effects of long-term benzodiazepine use: A meta-analysis. CNS Drugs. (2004) ;18: :37–48. |
[85] | Otmani S , Demazières A , Staner C , Jacob N , Nir T , Zisapel N , et al. Effects of prolonged release melatonin, zolpidem, and their combination on psychomotor functions, memory recall, and driving skills in healthy middle aged and elderly volunteers. Hum Psychopharmacol. (2008) ;23: :693–705. |
[86] | Hall-Porter JM , Schweitzer PK , Eisenstein RD , Ahmed HAH , Walsh JK . The effect of two benzodiazepine receptor agonist hypnotics on sleep-dependent memory consolidation. J Clin Sleep Med. (2014) ;10: :27–34. |
[87] | Aeschbach D , Dijk DJ , Trachsel L , Brunner DP , Borbély AA . Dynamics of slow-wave activity and spindle frequency activity in the human sleep EEG: Effect of midazolam and zopiclone. Neuropsychopharmacology. (1994) ;11: (4):237–44. |
[88] | Vermeeren A , Coenen AML . Effects of the use of hypnotics on cognition. Prog Brain Res.. (2011) ;190: :89–103. |
[89] | Arbon EL , Knurowska M , Dijk DJ . Randomised clinical trial of the effects of prolonged-release melatonin, temazepam and zolpidem on slow-wave activity during sleep in healthy people. J Psychopharmacol. (2015) ;29: :764–76. |
[90] | Brunner DP , Dijk DJ , Münch M , Borbély AA . Effect of zolpidem on sleep and sleep EEG spectra in healthy young men. Psychopharmacology. (1991) ;104: :1–5. |
[91] | Cummings SR , Nevitt MC , Browner WS , Stone K , Fox KM , Ensrud KE , et al. Risk factors for hip fracture in white women. N Engl J Med.. (1995) ;332: (12):767–73. |
[92] | Closser MH . Benzodiazepines and the elderly a review of potential problems. J Subst Abuse Treat. (1991) ;8: (1-2), 35–41. |
[93] | Paterniti S , Dufouil C , Alpérovitch A . Long-term benzodiazepine use and cognitive decline in the elderly: The Epidemiology of Vascular Aging Study. J Clin Psychopharmacol. (2002) ;22: (3):285–93. |
[94] | Ellul J , Archer N , Foy CM , Poppe M , Boothby H , Nicholas H , Brown RG , Lovestone S . The effects of commonly prescribed drugs in patients with Alzheimer’s disease on the rate of deterioration. J Neurol Neurosurg Psychiatry. (2007) ;78: (3):233–9. |
[95] | Shen YC . Lamotrigine in motor and mood symptoms of Huntington’s disease. World J Biol Psychiatry. (2008) ;9: (2):147–9. |
[96] | Kremer B , Clark CM , Almqvist EW , Raymond LA , Graf P , Jacova C , et al. Influence of lamotrigine on progression of early Huntington disease: A randomized clinical trial. Neurology. (1999) ;53: (5):1000–11. |
[97] | Sadler M . Lamotrigine associated with insomnia. Epilepsia. (1999) ;40: (3):322–5. |
[98] | Shen Y , Zhang M , Wang Y , Wang L , Xu X , Xiao G , et al. Subjective sleep disturbance in Chinese adults with epilepsy: Associations with affective symptoms. Epilepsy Res. (2017) ;135: :150–7. |
[99] | Foldvary N , Perry M , Lee J , Dinner D , Morris HH . The effects of lamotrigine on sleep in patients with epilepsy. Epilepsia. (2001) ;42: (12):1569–73. |
[100] | Placidi F , Marciani MG , Diomedi M , Scalise A , Giacomini P , Gigli GL . Effects of lamotrigine on nocturnal sleep, daytime somnolence and cognitive functions in focal epilepsy. Acta Neurol Scand. (2000) ;102: (2):81–6. |
[101] | O’Suilleabhain P , Dewey RB Jr. . A randomized trial of amantadine in Huntington disease. Arch Neurol. (2003) ;60: (7):996–8. |
[102] | Metman LV , Morris MJ , Farmer C , Gillespie M , Mosby K , Wuu J , et al. Huntington’s disease: A randomized, controlled trial using the NMDA-antagonist amantadine. Neurology. (2002) ;59: (5):694–9. |
[103] | Metman LV , Del Dotto P , Van Den Munckhof P , Fang J , Mouradian MM , Chase TN . Amantadine as treatment for dyskinesias and motor fluctuations in Parkinson’s disease. Neurology. (1998) ;50: (5):1323–6. |
[104] | Huntington Study Group. Dosage effects of riluzole in Huntington’s disease: A multicenter placebo-controlled study. Neurology. (2003) ;61: (11):1551–6. |
[105] | Landwehrmeyer GB , Dubois B , de Yébenes JG , Kremer B , Gaus W , Kraus PH , et al. Riluzole in Huntington’s disease: A 3-year, randomized controlled study. Ann Neurol. (2007) ;62: (3):262–72. |
[106] | Van Straten A , van der Zweerde T , Kleiboer A , Cuijpers P , Morin CM , Lancee J . Cognitive and behavioral therapies in the treatment of insomnia: A meta-analysis. Sleep Med Rev. (2018) ;38: :3–16. |
[107] | Sivertsen B , Omvik S , Pallesen S , Bjorvatn B , Havik OE , Kvale G , et al. Cognitive behavioral therapy vs zopiclone for treatment of chronic primary insomnia in older adults: A randomized controlled trial. JAMA. (2006) ;295: (24):2851–58. |
[108] | Richards KC , Beck C , O’Sullivan PS , Shue VM . Effect of individualized social activity on sleep in nursing home residents with dementia. J Am Geriatr Soc. (2005) ;53: (9):1510–7. |
[109] | Connell BR , Sanford JA , Lewis D . Therapeutic effects of an outdoor activity program on nursing home residents with dementia. J Hous Elderly. (2007) ;21: (3-4), 194–209. |
[110] | Naylor E , Penev PD , Orbeta L , Janssen I , Ortiz R , Colecchia EF , et al. Daily social and physical activity increases slow-wave sleep and daytime neuropsychological performance in the elderly. Sleep. (2000) ;23: (1):1–9. |
[111] | Richards KC , Lambert C , Beck CK , Bliwise DL , Evans WJ , Kalra GK , et al. Strength training, walking, and social activity improve sleep in nursing home and assisted living residents: Randomized controlled trial. J Am Geriatr Soc. (2011) ;59: (2):214–23. |
[112] | McCurry SM , LaFazia DM , Pike KC , Logsdon RG , Teri L . Development and evaluation of a sleep education program for older adults with dementia living in adult family homes. Am J Geriatr Psychiatry. (2012) ;20: (6):494–504. |
[113] | Cassidy-Eagle E , Siebern A , Unti L , Glassman J , O’Hara R . Neuropsychological functioning in older adults with mild cognitive impairment and insomnia randomized to CBT-I or control group. Clin Gerontol. (2018) ;41: (2), 136–44. |
[114] | Coe S , Franssen M , Collett J , Boyle D , Meaney A , Chantry R , et al. Physical activity, fatigue, and sleep in people with Parkinson’s disease: A secondary per protocol analysis from an intervention trial. Parkinsons Dis. (2018) ;2018: :1517807. |
[115] | Amara AW , Wood KH , Joop A , Memon RA , Pilkington J , Tuggle SC , et al. Randomized, controlled trial of exercise on objective and subjective sleep in Parkinson’s disease. Mov Disord. (2020) ;35: (6):947–58. |
[116] | Gibson RH , Gander PH , Dowell AC , Jones LM . Non-pharmacological interventions for managing dementia-related sleep problems within community dwelling pairs: A mixed-method approach. Dementia. (2017) ;16: (8):967–84. |
[117] | Bartlett DM , Lazar AS , Kordsachia CC , Rankin TJ , Lo J , Govus AD , et al. Multidisciplinary rehabilitation reduces hypothalamic grey matter volume loss in individuals with preclinical Huntington’s disease: A nine-month pilot study. J Neurol Sci. (2020) ;408: :116522. |
[118] | Figueiro MG , Plitnick BA , Lok A , Jones GE , Higgins P , Hornick TR , et al. Tailored lighting intervention improves measures of sleep, depression, and agitation in persons with Alzheimer’s disease and related dementia living in long-term care facilities. Clin Interv Aging. (2014) :1527–37. |
[119] | Fetveit A , Skjerve A , Bjorvatn B . Bright light treatment improves sleep in institutionalised elderly— an open trial. Int J Geriatr Psychiatry. (2003) ;18: (6):520–6. |
[120] | Dowling GA , Mastick J , Hubbard EM , Luxenberg JS , Burr RL . Effect of timed bright light treatment for rest-activity disruption in institutionalized patients with Alzheimer’s disease. Int J Geriatr Psychiatry. (2005) ;20: :738–43. |
[121] | Paus S , Schmitz-Hübsch T , Wüllner U , Vogel A , Klockgether T , Abele M . Bright light therapy in Parkinson’s disease: A pilot study. Mov Disord.. (2007) ;22: (10):1495–8. |
[122] | Videnovic A , Klerman EB , Wang W , Marconi A , Kuhta T , Zee PC . Timed light therapy for sleep and daytime sleepiness associated with Parkinson disease: A randomized clinical trial. JAMA Neurol. (2017) ;74: (4):411–8. |
[123] | Mitolo M , Tonon C , La Morgia C , Testa C , Carelli V , Lodi R . Effects of light treatment on sleep, cognition, mood, and behavior in Alzheimer’s disease: A systematic review. Dement Geriatr Cogn Disord. (2018) ;46: (5-6), 371–84. |
[124] | Forbes D , Blake CM , Thiessen EJ , Peacock S , Hawranik P . Light therapy for improving cognition, activities of daily living, sleep, challenging behaviour, and psychiatric disturbances in dementia. Cochrane Database Syst Rev. (2014) ;2:CD003946. |
[125] | Van Maanen A , Meijer AM , van der Heijden KB , Oort FJ . The effects of light therapy on sleep problems: A systematic review and meta-analysis. Sleep Med Rev. (2016) ;29: :52–62. |
[126] | O’Caoimh R , Mannion H , Sezgin D , O’Donovan MR , Liew A , Molloy DW . Non-pharmacological treatments for sleep disturbance in mild cognitive impairment and dementia: A systematic review and meta-analysis. Maturitas. (2019) ;127: :82–94. |
[127] | Rubiño JA , Gamundí A , Akaarir M , Canellas F , Rial R , Nicolau MC . Bright light therapy and circadian cycles in institutionalized elders. Front Neurosci. (2020) ;14: :359. |
[128] | Kalliolia E , Silajdžić E , Nambron R , Hill NR , Doshi A , Frost C , et al. Plasma melatonin is reduced in Huntington’s disease. Mov Disord. (2014) ;29: (12):1511–5. |
[129] | Videnovic A , Noble C , Reid KJ , Peng J , Turek FW , Marconi A , et al. Circadian melatonin rhythm and excessive daytime sleepiness in Parkinson disease. JAMA Neurol. (2014) ;71: (4):463–9. |
[130] | Asayama K , Yamadera H , Ito T , Suzuki H , Kudo Y , Endo S . Double blind study of melatonin effects on the sleep-wake rhythm, cognitive and non-cognitive functions in Alzheimer type dementia. J Nippon Med School. (2003) ;70: (4):334–41. |
[131] | Jean-Louis G , von Gizycki H , Zizi F . Melatonin effects on sleep, mood, and cognition in elderly with mild cognitive impairment. J Pineal Res. (1998) ;25: (3):177–83. |
[132] | Serfaty M , Kennell-Webb S , Warner J , Blizard R , Raven P . Double blind randomised placebo controlled trial of low dose melatonin for sleep disorders in dementia. Int J Geriatr Psychiatry. (2002) ;17: (12):1120–7. |
[133] | Gehrman PR , Connor DJ , Martin JL , Shochat T , Corey-Bloom J , Ancoli-Israel S . Melatonin fails to improve sleep or agitation in double-blind randomized placebo-controlled trial of institutionalized patients with Alzheimer disease. Am J Geriatr Psychiatry. (2009) ;17: (2):166–9. |
[134] | Singer C , Tractenberg RE , Kaye J , Schafer K , Gamst A , Grundman M , et al. A multicenter, placebo-controlled trial of melatonin for sleep disturbance in Alzheimer’s disease. Sleep. (2003) ;26: (7):893–901. |
[135] | Dowling GA , Mastick J , Colling E , Carter JH , Singer CM , Aminoff MJ . Melatonin for sleep disturbances in Parkinson’s disease. Sleep Med. (2005) ;6: (5):459–66. |
[136] | Medeiros CA , Carvalhedo de Bruin PF , Lopes LA , Magalhães MC , de Lourdes Seabra M , Sales de Bruin VM . Effect of exogenous melatonin on sleep and motor dysfunction in Parkinson’s disease: A randomized, double blind, placebo-controlled study. J Neurol. (2007) ;254: :459–64. |
[137] | Walsh JK , Hall-Porter JM , Griffin KS , Dodson ER , Forst EH , Curry DT , et al. Enhancing slow wave sleep with sodium oxybate reduces the behavioral and physiological impact of sleep loss. Sleep. (2010) ;33: (9):1217–25. |
[138] | Ondo WG , Perkins T , Swick T , Hull KL , Jimenez JE , Garris TS , et al. Sodium oxybate for excessive daytime sleepiness in Parkinson disease: An open-label polysomnographic study. Arch Neurol. (2008) ;65: (10):1337–40. |
[139] | Büchele F , Hackius M , Schreglmann SR , Omlor W , Werth E , Maric A , et al. Sodium oxybate for excessive daytime sleepiness and sleep disturbance in Parkinson disease: A randomized clinical trial. JAMA Neurol. (2018) ;75: (1):114–8. |
[140] | Maitre M , Klein C , Mensah-Nyagan AG . A proposed preventive role for Gamma-hydroxybutyrate (XyremR) in Alzheimer’s disease. Alzheimers Res Ther. (2016) ;8: (1):37. |
[141] | Grimaldi D , Papalambros NA , Zee PC , Malkani RG . Neurostimulation techniques to enhance sleep and improve cognition in aging. Neurobiol Dis. (2020) ;141: :104865. |
[142] | Wunderlin M , Züst MA , Hertenstein E , Fehér KD , Schneider CL , Klöppel S , et al. Modulating overnight memory consolidation by acoustic stimulation during slow-wave sleep: A systematic review and meta-analysis. Sleep. (2021) ;44: (7):zsaa296. |
[143] | Ong JL , Lo JC , Chee NI , Santostasi G , Paller KA , Zee PC , et al. Effects of phase-locked acoustic stimulation during a nap on EEG spectra and declarative memory consolidation. Sleep Med. (2016) ;20: :88–97. |
[144] | Ngo HV , Claussen JC , Born J , Mölle M . Induction of slow oscillations by rhythmic acoustic stimulation. J Sleep Res. (2013) ;22: (1):22–31. |
[145] | Ngo HV , Miedema A , Faude I , Martinetz T , Mölle M , Born J . Driving sleep slow oscillations by auditory closed-loop stimulation—a self-limiting process. J Neurosci. (2015) ;35: (17):6630–8. |
[146] | Diep C , Ftouni S , Manousakis JE , Nicholas CL , Drummond SP , Anderson C . Acoustic slow wave sleep enhancement via a novel, automated device improves executive function in middle-aged men. Sleep. (2020) ;43: (1):zsz197. |
[147] | Papalambros NA , Santostasi G , Malkani RG , Braun R , Weintraub S , Paller KA , et al. Acoustic enhancement of sleep slow oscillations and concomitant memory improvement in older adults. Front Hum Neurosci. (2017) ;11: :109. |
[148] | Leminen MM , Virkkala J , Saure E , Paajanen T , Zee PC , Santostasi G , et al. Enhanced memory consolidation via automatic sound stimulation during non-REM slee. Sleep. (2017) ;40: (3), zsx003. |
[149] | Stanyer EC , Baniqued PD , Awais M , Kouara L , Davies AG , Killan EC , et al. The impact of acoustic stimulation during sleep on memory and sleep architecture: A meta-analysis. J Sleep Res. (2022) ;31: (3):e13385. |
[150] | Papalambros NA , Weintraub S , Chen T , Grimaldi D , Santostasi G , Paller KA , et al. Acoustic enhancement of sleep slow oscillations in mild cognitive impairment. Ann Clin Transl Neurol. (2019) ;6: (7):1191–201. |
[151] | Herring WJ , Connor KM , Snyder E , Snavely DB , Zhang Y , Hutzelmann J , et al. Suvorexant in patients with insomnia: Pooled analyses of three-month data from phase-3 randomized controlled clinical trials. J Clin Sleep Med. (2016) ;12: (9):1215–25. |
[152] | Michelson D , Snyder E , Paradis E , Chengan-Liu M , Snavely DB , Hutzelmann J , et al. Safety and efficacy of suvorexant during 1-year treatment of insomnia with subsequent abrupt treatment discontinuation: A phase 3 randomised, double-blind, placebo-controlled trial. Lancet Neurol. (2014) ;13: (5):461–71. |
[153] | Herring WJ , Connor KM , Snyder E , Snavely DB , Zhang Y , Hutzelmann J , et al. Suvorexant in elderly patients with insomnia: Pooled analyses of data from phase III randomized controlled clinical trials. Am J Geriatr Psychiatry. (2017) ;25: (7):791–802. |
[154] | Herring WJ , Ceesay P , Snyder E , Bliwise D , Budd K , Hutzelmann J , et al. Polysomnographic assessment of suvorexant in patients with probable Alzheimer’s disease dementia and insomnia: A randomized trial. Alzheimers Dement. (2020) ;16: (3):541–51. |
[155] | Neylan TC , Richards A , Metzler TJ , Ruoff LM , Varbel J , O’Donovan A , et al. Acute cognitive effects of the hypocretin receptor antagonist almorexant relative to zolpidem and placebo: A randomized clinical trial. Sleep. (2020) ;43: (10):zsaa080. |
[156] | Black J , Pillar G , Hedner J , Polo O , Berkani O , Mangialaio S , et al. Efficacy and safety of almorexant in adult chronic insomnia: A randomized placebo-controlled trial with an active reference. Sleep Med. (2017) ;36: :86–94. |