Purple Queen® fruits of Punica granatum L.: Nutraceutical properties and unconventional growing substrates
Abstract
BACKGROUND:
Purple Queen® is an early ripening pomegranate cultivar growing well in soilless cultivation. Substrates have direct effects on plant development and, due to progressive peatland exhaustion, the request for new materials for plant growth is high.
OBJECTIVE:
The objective of this work was to verify the effects of a new potential substrate obtained from the remediation of marine port sediments on the nutraceutical profile of Purple Queen® fruits, using 50% and 100% of reclaimed sediment.
METHODS:
The study was carried out determining ellagitannins and polysaccharides obtained from peel after decoction, and anthocyanins in aril juice on fruits from two agronomic seasons.
RESULTS:
The presence of 100% of the sediment had a partial detrimental effect on fruits size and weight. Compared to a peat-based commercial substrate (control), remediated sediment preserved ellagitannin content and increased the anthocyanin amount (up to 40% higher) and polysaccharide content up to 34% on dry fruit. High molecular weight polysaccharides (>2000 kDa) were identified in all the fruits with glucose and galacturonic acid as the major sugars.
CONCLUSIONS:
Fruits from plants cultivated only on sediment or in a mixture with 50% of peat showed significant increases of bioactive compounds as stress response in two consecutive seasons.
1Introduction
Pomegranate (Punica granatum L.) is classified among the top seven fruits with the highest beneficial properties for human health [1]. The fruits, usually consumed fresh or in form of juice, are widely appreciated by consumers and known as good source of nutraceutical compounds [2, 3]. To date, it has been demonstrated that the health benefits from pomegranate are related to the intake of compounds present both in juice, mainly anthocyanins, and in peel (mesocarp + esocarp), mainly ellagitannins and polysaccharides. Pomegranate peel represents the 40–50% of the whole fresh fruit weight, and it is the main by-product obtained after juice extraction [4]. The main bioactive molecules of pomegranate are ellagitannins as α punicalagin and β punicalagin, together with other minor constituents as ellagic acid and their derivatives [5, 6]. The ellagitannins of pomegranate are water soluble, consequently easily extractable by polar media as hydro-alcoholic solutions or a simply decoction, being these molecules also thermally stable [6]. Furthermore, nowadays, polysaccharides of pomegranate peel, are gaining growing scientific interest thanks to the their antioxidant effects [7], antiglycation and tyrosinase inhibition properties [8], in vitro prebiotic properties [9], immunomodulatory and anti-inflammatory effects [10]. It has been observed that a simple water decoction from mesocarp of pomegranate guaranteed the extraction of both ellagitannins and polysaccharides [9], and that this extract showed the ability to counteract initial, intermediate and late stages of colon carcinogenesis in rats, suggesting a possible use of the decoction in primary and secondary prevention of human colon cancer [11].
Purple Queen® is greatly appreciated for its productivity and early ripening (second half of August in Alicante and Murcia, Spain). These peculiarities are notably functional for economic and marketing strategies since they broaden the availability of pomegranate fruits for both fresh consumption and processing. Registration has been requested for Purple Queen in various countries outside the European Union, especially in the southern hemisphere to respond to the demand for pomegranate fruit which has strongly increased over recent years.
Peat is one of the main components of substrates used in agriculture. However, due to the progressive exhaustion of peatland, the demand for alternative substrates with suitable physico-chemical properties is increasing. To meet sustainability criteria in the plant nursery industry, attention is currently focused on the reutilization of waste-derived substrates. Among these, dredged remediated sediments have already been proposed as soilless growing media for the cultivation of ornamental and food crops [12, 13].
Dredging, normally performed to guarantee navigability, involves the excavation of sediment from the bottom of water basins and its reallocation in another place [14]. This process generates a huge volume of dredged spoils which must be appropriately managed following national regulations. Unpolluted sediments are generally re-used for beach nourishment and embankments while contaminated sediments, being classified as waste, are placed in landfill. Phytoremediation has proven to be a sustainable technology for reclaiming highly-polluted sediments [15] and for converting sediment into a “techno-soil” able to support vegetation [16, 17]. Hence, the potential reallocation of remediated dredged materials in agriculture could provide an alternative solution to the disposal in landfills and, at the same time, reduce the intensive use of raw material as in the case of the requested peat. As reported by Mattei et al., [13], the cultivation of an ornamental crop in remediated sediments showed a significantly lower environmental impact with respect to the use of traditional peat. Recently, dredged sediments were used as growing media for soilless cultivation of Purple Queen® evaluating the plant productivity and the main nutritional characteristic of the juice were evaluated [18]. The authors observed that fruit yield was partially reduced due to the high sediment bulk density which limited overall plant development. At the same time, fruits cultivated on sediment showed a significant increase of soluble solids, fructose and glucose content in the arils, indicating that the presence of sediment promoted greater dry weight accumulation in this tissue.
The present study was focused on the characterization and quantification of the bioactive components of whole fruits (arils and peel) obtained from Purple Queen® trees cultivated on dredged remediated sediment as previously described by Melgarejo et al., [18]. An evaluation of the nutraceutical quality of the fruits from two agronomic seasons was carried out determining anthocyanins in juice as well as polysaccharides and ellagitannins in peel after extraction by decoction. To the best of our knowledge, this is the first study investigating the effect of innovative substrates on the main nutritional components of the arils and peel from pomegranate fruits obtained in soilless cultivation.
2Materials and methods
2.1Experimental design and fruit sampling
Purple Queen® plants were cultivated in a remediated dredged sediment as described by Melgarejo et al. [18]. Briefly, the sediments were dredged from the Livorno port (Italy) and were subjected to three years of phytoremediation as described by Masciandaro et al. [16]. Afterwards, the sediment underwent three months of landfarming, a bioremediation consisting in periodic aeration and irrigation of the sediment in order to homogenize the matrix. The remediated sediment was used to prepare two growing substrates: PQ-100 (PQ2017-100 and PQ2018-100 samples), the remediated sediment alone, and PQ-50 (PQ2017-50 and PQ2018-50 samples) derived from the remediated sediment mixed with a traditional peat-based commercial substrate (1 : 1, v/v). The same peat-based commercial substrate present in PQ-0 was used as control treatment (PQ2017-0 and PQ2018-0 samples). Moreover, samples of Purple Queen® fruits purchased in the local market were also analyzed (PQ-C) as further control samples. The main physico-chemical parameters of the substrates are presented in Table 1.
Table 1
Main chemical parameters of the commercial peat substrate (0), mixture sediments: peat v/v 1 : 1 (50), and remediated sediment used as pure (100). The values are the mean (n = 3) and standard deviation (in brackets)
Parameters | 0 | 50 | 100 |
pH | 6.2 (0.1) | 7.9 (0.2) | 8.21 (0.3) |
EC (μs/cm) | 1129 (7) | 596 (14) | 352 (16) |
NH3 (mg/L) | 1.03 (0.19) | 0.25 (0.03) | 0.08 (0.03) |
NO3– (mg/L) | 304 (1) | 190 (18) | 26 (0.1) |
TN (%) | 1.3 (0.2) | 0.29 (0.01) | 0.12 (0.02) |
PO4 (mg/L) | 16.8 (0.2) | 0.4 (0.0) | 0.4 (0.0) |
Chloride (mg/kg) | 20.5 (0.2) | 12.4 (2.1) | 11.4 (0.5) |
K (mg/kg) | 65 (5) | 19 (0.5) | 9 (0.1) |
Mg (mg/kg) | 22.1 (0.3) | 11.4 (0.2) | 8.4 (1.5) |
Ca (mg/kg) | 114 (1) | 67 (2) | 31 (4) |
Cu (mg/kg) | 14.1 (0.3) | 43.7 (0.9) | 55.4 (1.2) |
Zn (mg/kg) | 13 (1) | 170 (8) | 194 (7) |
Ni (mg/kg) | 0.2 (0.0) | 35 (2) | 39 (2) |
Pb (mg/kg) | 1.4 (0.0) | 34 (3) | 38 (6) |
The fruit samples used in this experiment were harvested at full ripening at the end of two growing seasons (2017 and 2018). All plants (PQ-0, PQ-50 and PQ-100) received a complete Hoagland nutrient solution, composed of KNO3, NH4NO3, K2SO4, HNO3, H3PO4, and a complex mix of microelements [18]. The arils and peel (mesocarp + exocarp) used for the analyses are presented in Table 1S.
2.2Standards and reagents
All solvents were of analytical HPLC grade from Sigma Aldrich (St. Louis, Missouri, USA). Ultrapure water was obtained by the Milli-Q-system (Millipore SA, Molsheim, France). Ellagic acid (purity≥95%) and punicalagin (purity≥98%) were purchased from Sigma Chemical Co. (St. Louis, MO, USA). Oenin chloride (purity≥95%) was purchased from Extrasynthese (Genay, France). Dextrans at different molecular weights (2000, 1100, 410, 150, and 50 kDa) and sucrose (360 Da) used for SEC analyses were from Sigma-Aldrich, USA. The ellagitannins were quantified according to their maximum absorption at either 380 nm using a five-point calibration curve of a racemic mixture of α punicalagin and β punicalagin (purity≥99%, linearity range 2–5μg, R2 = 1.000) or 370 nm using a five-point calibration curve of ellagic acid (purity 95%) (linearity range 0–1.7μg, R2 = 1.000). The anthocyanins were quantified at 520 nm with a four-point calibration curve of oenin chloride (purity≥95%; linearity range 0–2.6μg, R2 = 0.999).
2.3Anthocyanins from arils
Arils from PQ2018 (0; 50; 100) and Purple Queen® commercial sample (PQ-C) were used for the preparation of juices using a domestic Hurom extractor which guarantees the preparation of juices through a rapid process at low temperatures. The arils derived from fruits collected from the different soils were divided into three aliquots to obtain a triplicate. Each juice was diluted (1 : 1, v/v) with ethanol (2% HCOOH) to better stabilize the solution before analysis. The samples were centrifuged at 14000 rpm for 5 min, the supernatant was recovered and analyzed by HPLC-DAD-MS.
2.4Ellagitannins and polysaccharides from peel
The peel and arils from fruits (n = 10) were manually separated, weighted and freeze-dried until constant weight was reached. The freeze-dried peel (5 g) was boiled in 200 mL of ultrapure water, for 1 h as for Khatib et al., [9]. The supernatant was recovered after cooling and centrifugation (4500 rpm, 8 min, 4°C) and taken to a final volume of 200 mL with ultrapure water. Ten mL were used for the analysis of ellagitannins. The remaining amount of supernatant was treated with 300 mL of ethanol and kept at 0° C to induce polysaccharides precipitation. After centrifugation (4500 rpm, 8 min, 4°C) the recovered polysaccharides were freeze-dried and weighed to calculate the yield.
2.5HPLC-DAD analysis of phenolic extracts
The ellagitannins in peel and juice and the anthocyanins in juice were analyzed using a HP 1200 L liquid chromatograph equipped with a DAD detector (Agilent Technologies, Palo Alto, CA, USA) after removing suspended solids by centrifugation at 14,000 rpm for 10 min. A Kinetex, 100, EC-C18 (30×3 mm, 2.6μm, Agilent, USA) column was used to determine the two phenolic subclasses by a single chromatographic run; solvent A was CH3CN and solvent B was H2O acidified by HCOOH (3%,v/v). The elution profile was as follow: 0–8 min, 5–25% A (v/v); 8–18 min, 25% A (v/v); 18–20 min, 25–95% A(v/v); 20–26 min, 95% A (v/v). Total time of analysis was 28 min, equilibration time 10 min, and flow rate 0.4 mL/min. Injection volume: 2μL for ellagitannins extracts (decoction) and 10μL for anthocyanins extracts (centrifuged juices). Chromatograms were recorded at 370 nm, 380 nm and 520 nm.
2.6MS analysis
The extracts from decoction and juices from arils were analyzed on a quadrupole ionic trap LTQ (Thermo Finnigan) coupled to an HPLC (Thermo Finnigan Surveyor, San Jose, CA, USA); the HPLC conditions were the same used for the HPLC-DAD analysis. The analyses were conducted with the following ESI parameters (electrospray ionization): Sheath Gas Flow Rate: 35; Aux Gas Flow Rate: 10; Sweep Gas Flow Rate: 7; Spray Voltage: 4.20 V; Capillary temperatures: 280°C; Capillary Voltage: –23 V; Tube Lens: –53. Acquisition for mass analysis was performed in negative and positive ions in full spectrum scan in the range of m/z from 100 to 1800. Further MS analyses were performed with a LC-MS system consisting of an UHPLC-MS system Agilent Infinity Lab LC/MSD. The HPLC conditions were the same used for the HPLC-DAD analysis. The analyses were conducted with the following ESI parameters (electrospray ionization): drying gas flow and temperature: 10.5 L/min, 350°C, respectively; nebulizer pressure 1811 Torr; capillary voltage 3500 V. Acquisition for mass analyses was performed in negative and positive ions in full spectrum scan in the range of m/z from 100 to 1800. Different fragmentor voltages were utilized at 70 V and 200 V for negative ion mode and at 200 V and 300 V for positive ion mode.
2.7Size exclusion chromatography (SEC) for polysaccharides
The samples containing the total polysaccharides were analyzed by SEC to determine the apparent molecular weight of the main constituents. Briefly, after freeze drying the samples were dissolved in distilled water at a final concentration close to 0.5 mg/mL. The samples were analyzed according to as Chamizo et al., [19] using a Pro Star HPLC Chromatograph (Varian USA) equipped with a refractive index detector (mod 355), using two columns, PolySep-GFC-P 6000 and PolySep-GFC-P 4000 from Phenomenex, USA, connected in series. The columns (700 mm length and 7.8 mm internal diameter) had separation ranges of 100 kDa to 15 MDa and 0.3 to 400 kDa. HPLC-grade water was used for the isocratic elution, with a flow of 0.6 mL/min, and total time of 70 min. Blue-dextrans at various molecular weights ranging from 50 kDa to 2000 kDa were used as internal standards to determine the hydrodynamic volume of the main polymers.
2.8Sugars analysis
The two samples (PQ-C and PQ2018-100) were dialysed (cut-off 12–14 kDa), freeze-dried and treated according to Nunes et al., [20] for the determination of neutral sugars after acid hydrolysis (H2SO4, 72%) and conversion to the corresponding alditol acetates. Gas chromatography was performed using a Hewlett-Packard 5890 with a split injector (split ratio 1 : 60) and FID detector. A 25 m column CP-Sil-43 CB (Chrompack, Holland) with 0.15 mm i.d. and 0.20μm film thickness was used. With the injector and detector operating at 220°C, the following temperature program was used: 180°C for 5 min and 200°C for 20 min, with a rate of 0.5°C/min; linear velocity of the carrier gas (H2) was set at 50 cm/s at 200°C. In addition, uronic acids were colorimetrically determined using m-phenylphenol as previously reported [20].
2.9Statistics
Results were expressed as mean±SD using EXCEL software (version 2013) in-house routines. One-way ANOVA and F-test (p < 0.05) by Microsoft Excel statistical software and Fisher’s LSD (DSAASTAT software v. 1.1, Onofri, Pisa, 2007) were used to point out significant differences. The mean value set of quantitative parameters from each cultivar was used to visualize in a heatmap the correlation distance among variables and average linkage among objects by adopting the CLUSTVIS on line tool (https://biit.cs.ut.ee/clustvis/).
3Results and discussion
3.1Morphological characteristics of Purple Queen® fruits
As reported in Table 1S, all the fruits showed a similar mean weight for both 2017 and 2018; control fruits (PQ-0), although the plants were cultivated in pots, showed a mean weight of 299 g which was comparable to that of the commercial fruits (PQ-C) at 303 g. The presence of sediments in both the tested percentages negatively affected fruit size. Whole fruits, arils and peel total weight followed a common trend with PQ-0 > PQ-50 > PQ-100 in both the years. These findings clearly indicate that the physical and chemical composition of the sediments (Table 1), different from peat, induced plant stress resulting in a lower plant productivity as previously highlighted [12, 18].
3.2Anthocyanins in juice
In order to investigate on specific differences in the amount of the single compounds, the qualitative and quantitative evaluation of anthocyanins was carried out by HPLC-DAD-MS. The anthocyanin chromatographic profiles of fruits collected in 2017 showed similar patterns for the produced juices independently from the substrate (data not shown). In light of these preliminary findings, the fruits collected in 2018 were used also to carry out a quantitative evaluation. As expected, the anthocyanin fingerprints were similar to those found in Purple Queen® from 2017 and to profiles reported in the literature for other varieties [5] (Fig. 1SA).
Fig. 1
Anthocyanins in juices obtained from fruits PQ-0, PQ-50, PQ-100 and PQ-C collected in 2018: a) distribution of the main compounds, and b) total anthocyanins content. The data are expressed as mg/L as a mean of a triplicate. Error bar represent the standard deviation. Different letters mean statistically significant differences (p < 0.05).
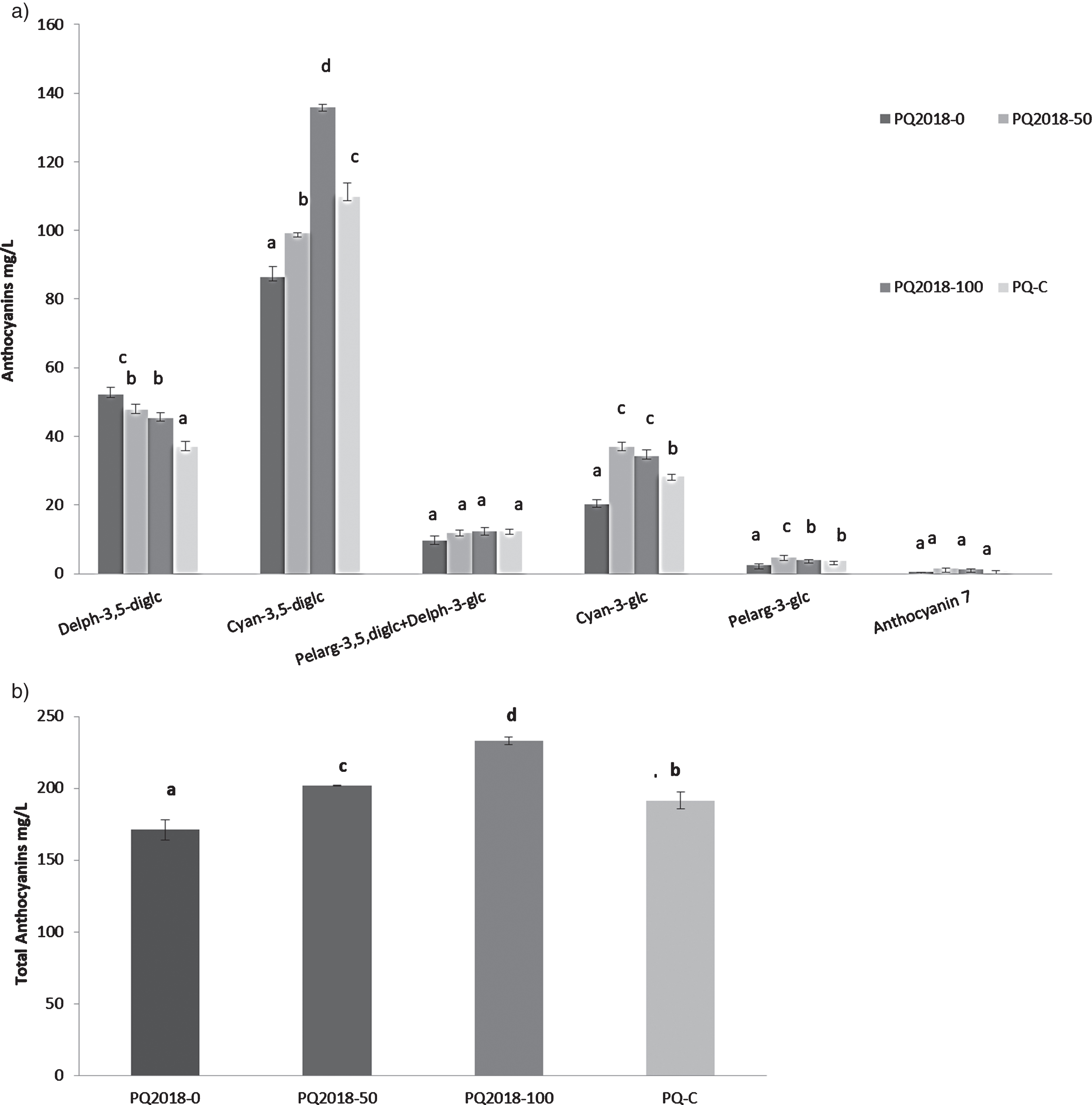
The absence of α and β punicalagins in our samples is linked to the method applied to obtain the juices from arils only, unlike most commercial pomegranate juices which are usually made by pressing half of the whole fruit, meaning a co-extraction of some ellagitannins from peel [5]. The anthocyanins identification (Table 2S) was performed by MS analyses in positive ionization mode at different fragmentation energies by identifying the molecular ion and the corresponding aglycone (Fig. 2S–6S). The resulted profiles highlighted the presence of the same anthocyanins already reported in the literature [5] in several very different varieties of pomegranate [21]. MS results highlighted that the dredged sediment did not modify the qualitative profile of the anthocyanins. The use of the sediments particularly influenced the concentration of cyanidin-3,5- diglucoside which showed the highest values (140 mg/L) in the PQ2018-100 sample; the control (PQ2018-0) showed the lowest value (90 mg/L). Moreover, Fig. 1A shows that also cyanidin-3-glucoside was significantly increased in 2018 fruits cultivated on sediment-based media (100% and 50%) with respect to the control (PQ2018-0) and commercial fruit (PQ-C). It should be pointed out that an environmental factor, such as the growing media, positively affected the content of cyanidin, an important molecule with beneficial qualities such as neuroprotective, antioxidant and anti diabetic properties [22]. An opposite trend was observed for delphinidin-3,5-diglucoside which resulted statistically higher in control fruit compared to the other treatments, although with slight differences (Fig. 1A). The total anthocyanins ranged from 171 to 233 mg/L in PQ2018-0 and PQ2018-100, respectively (Fig. 1B).
Fig. 2
Total ellagitannins in decoction of peel: samples PQ2017 and PQ2018 grown with different media (sediments % of 0; 50; 100) compared to Purple Queen® commercial sample (PQ-C). Data (mg/g DW) are mean of a triplicate. Different letters mean statistically significant differences (p < 0.05).
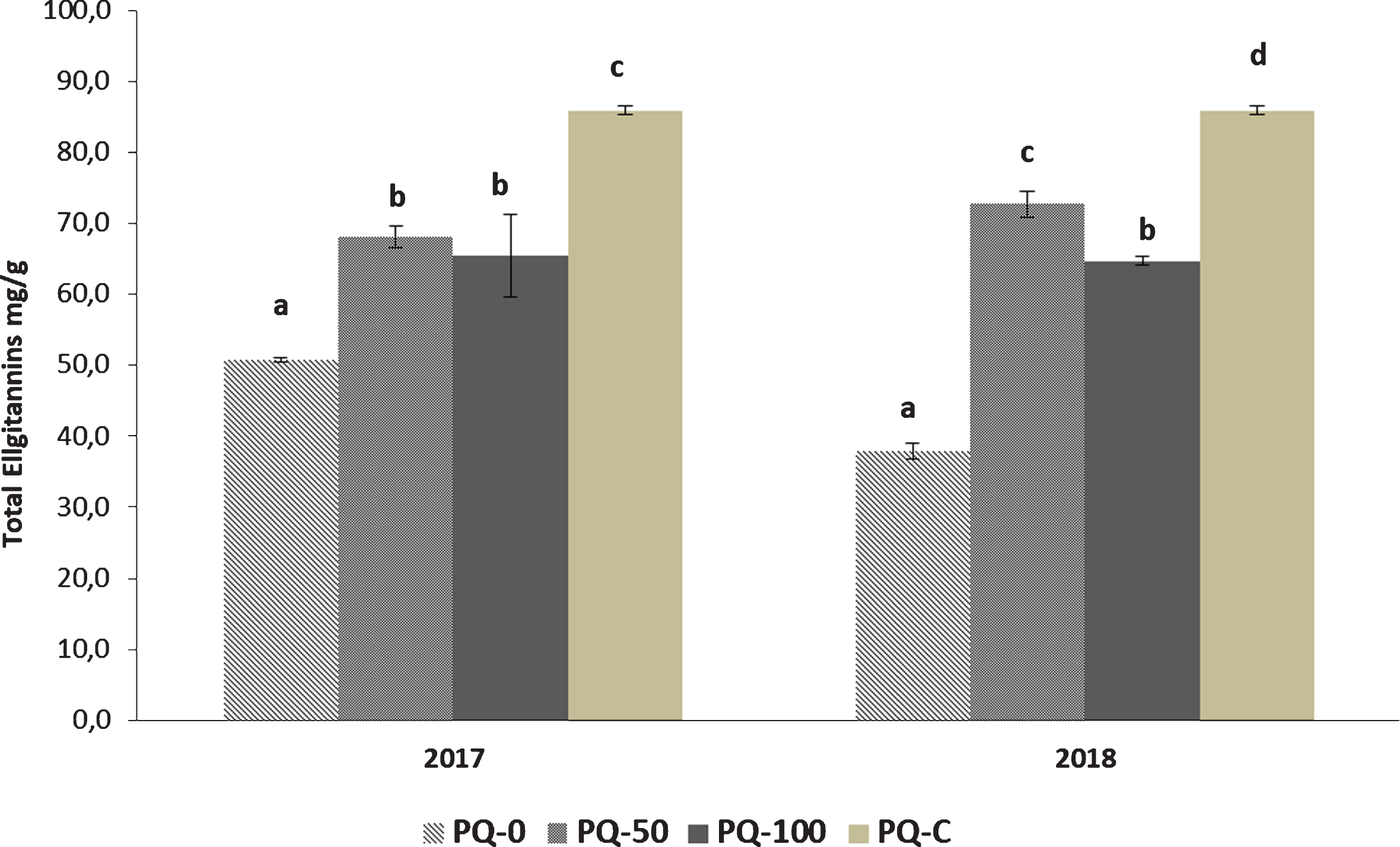
It is noteworthy that both PQ-100 and PQ-50 from 2018 samples were richer in anthocyanins than the control and commercial fruit (PQ2018-0 and PQ-C, respectively). Based on these results, plants PQ2018-100 and PQ2018-50 synthetized higher anthocyanins content in response to a greater stress, presumably caused by the sediment. Previous data regarding anthocyanins content in Purple Queen grown under the same reclaimed sediment did not evidence significant variations, probably due to a different extraction procedure and the application of analytical methods based on the spectrophotometric determination of the total anthocyanins [18].
Findings from fruits of fifteen Iranian varieties of pomegranate indicated 252 mg/L as the highest anthocyanin content determined by HPLC-DAD [23]. Comparing these results with the amount measured in Purple Queen®, it turns out that this variety is a good source of anthocyanins, with values comparable to those of the richest Iranian varieties even when grown on pure remediated sediments (PQ2018-100).
Anthocyanins are well-known to have a strong positive impact on human health [4] and, although there are no fixed values for anthocyanins intake, in 2013 the Chinese Nutrition Society, suggested providing a daily intake of at least 50 mg.
The importance of finding new substrates for cultivation suitable to maintain or even better to increase the phenolic expression of the anthocyanins in arils, is certainly of great interest, since pomegranate juice is the only part of the fruit usually consumed so far.
3.3Ellagitannins from peel
The peel is known to be as the richest tissue of pomegranate fruit in terms of ellagitannins and polysaccharides, two classes of bioactive compounds that are well soluble and chemically stable in hot water [9]. Due to these properties, in this work, peel extraction was carried out by applying a decoction because this procedure resulted suitable to efficiently recover both ellagitannins and polysaccharides with high yields. The possibility of using only water as extractive solvent can be strategic to facilitate the valorization of this by-product obtained in large quantities during the juice production [9]. Applying this process, it is also possible to propose the use of the dry decoction, almost representing the 70% of the dry weight of the peel, as a new functional ingredient to enrich different foods with ellagitannins and pomegranate polysaccharides.
Analogously to what observed for the anthocyanins, the phenolic components detected in Purple Queen® decoction (mass spectra in (Fig. 7S–10S), were the same as those previously found in other varieties [5] (Table 2S), with galloyl-hexoside (2), α punicalagin (3), β punicalagin (4), ellagic acid pentoside (7) and ellagic acid (8) resulting as the principal ellagitannins (Table 2). All the chromatographic profiles related to the ellagitannins found in peel grown in 2017 and 2018 in the different substrates resulted very similar and almost completely super imposable (Fig. 1SB). It can be said that the presence of remediated dredged sediments did not induce changes in the biosynthetic pathways of these phenolic compounds. The ellagitannins content in the fruits harvested in the two seasons showed a similar trend (Fig. 2): the presence of sediment mixed with peat (i.e. PQ-50) determined the maximum increase in both years, with a more pronounced effect on the fruits of 2018, while the highest concentration of ellagitannins was found in the commercial sample (PQ-C). The amount of α and β punicalagins in Purple Queen®, cited as major ellagitannins responsible for the majority of the biological properties of pomegranate, ranged from 54% to 60% of total phenols for all the fruits grown in pots, while the percentage increased in the commercial sample (PQ-C) with approximately 70% of the total content (Table 2).
Table 2
Ellagitannins in the decoction of peel of Purple Queen® (PQ) samples collected in 2017 and 2018 grown in pots in different conditions (PQ-0;PQ-50;PQ-100); PQ-C was a commercial sample from 2018. Data (mg/g DW) are a mean a triplicate with SD values in brackets
PQ-0 | PQ-50 | PQ-100 | PQ-C | ||||
Compounds | 2017 | 2018 | 2017 | 2018 | 2017 | 2018 | 2018 |
Ellagitannin derivative (1) | 1.8 (0.04) | 0.8 (0.02) | 2.1 (0.03) | 2.6 (0.02) | 2.6 (0.05) | 2.2 (0.05) | 1.9 (0.05) |
galloyl-hexoside (2) | 12.5 (0.05) | 7.1 (0.02) | 18.6 (0.05) | 22.5 (0.05) | 19.1 (0.04) | 22.5 (0.05) | 19.3 (0.01) |
α punicalagin (3) | 8.0 (0.02) | 7.2 (0.05) | 11.6 (0.01) | 11.7 (0.03) | 8.8 (0.04) | 9.0 (0.03) | 17.5 (0.03) |
β punicalagin (4) | 21.9 (0.04) | 14.7 (0.04) | 27.9 (0.01) | 27.5 (0.02) | 24.5 (0.05) | 23.9 (0.02) | 39.2 (0.02) |
Ellagitannin derivative (5) | 1.2 (0.03) | 0.7 (0.02) | 0.7 (0.02) | 1.3 (0.04) | 0.6 (0.01) | 0.5 (0.04) | 1.9 (0.04) |
Ellagic acid derivative (6) | 1.5 (0.01) | 0.9 (0.05) | 0.9 (0.08) | 1.5 (0.03) | 0.7 (0.01) | 0.8 (0.01) | 2.0 (0.04) |
Ellagic acid pentoside (7) | 1.1 (0.03) | 2.3 (0.03) | 1.4 (0.01) | 1.7 (0.02) | 1.4 (0.03) | 1.4 (0.02) | 1.3 (0.03) |
Ellagic acid (8) | 1.8 (0.07) | 3.9 (0.01) | 4.7 (0.01) | 3.3 (0.10) | 3.8 (0.04) | 3.9 (0.01) | 2.2 (0.04) |
Ellagic acid derivative (9) | 0.1 (0.01) | 0.7 (0.05) | 0.1 (0.03) | 0.2 (0.02) | 0.1 (0.01) | 0.1 (0.01) | 0.2 (0.01) |
Ellagic acid derivative (10) | 0.1 (0.01) | 0.1 (0.01) | 0.1 (0.01) | 0.2 (0.01) | 0.1 (0.01) | 0.1 (0.01) | 0.3 (0.02) |
The values reported in the literature for total ellagitannins in pomegranate peel range from 67 mg/g to 262 mg/g DW depending on the different varieties, geographical and environmental factors [5, 9, 24]. Findings from a previous work on Wonderful and Laffan varieties showed that the total ellagitannins extracted with a hydroalcoholic medium (ethanol 70%) and hot water gave very similar amounts [6].
In our samples, the mean values for total ellagitannins extracted by decoction ranged from 38 to 85 mg/g dry peel (Fig. 2) confirming that Purple Queen® is a variety with a medium content of ellagitannins. To the best of our knowledge, this is the first study to evaluate ellagitannins in peel of the Purple Queen® variety and compare the content obtained from pomegranate plants grown in pots on different substrates grounds containing peat and remediated dredged sediments.
3.4Polysaccharides from peel
Pomegranate peel has been recognized to be a good source of polysaccharides, representing approximately 10–12% of the fruit dry weight and being mainly present as pectin [7, 9, 10, 25]. Several biological properties concerning the polysaccharides of pomegranate have been recently highlighted in the literature: immunomodulatory activity [10], scavenging properties, and ability to reduce the growth of tumors in mice in combination with doxorubicin [8], and in vitro prebiotic activity [9] contributing to maintain the health of human microbiota.
In light of these studies, although polysaccharides of pomegranate can be considered a part of the bioactive molecules of the fruit, scarce data are available on their structure and no information is available on Purple Queen® till now. In this study, initially, the yields in total polysaccharides were gravimetrically evaluated after precipitation induced by ethanol from decoction. As shown in Fig. 3, similar values were obtained for the 2017 and 2018 samples: the increased content of polysaccharides resulted proportional to the percentage of remediated sediment in the substrate. The amount of polysaccharides found in fruits of plants cultivated in remediated sediment (PQ-100) showed a strong increment in both years with respect to the values measured for the commercial sample (PQ-C). The total percentage of polysaccharides expressed in the dry weight of peel ranged from approximately 12% in PQ2017-0 to a maximum of 32% in PQ2017-100. These amounts are clearly greater with respect to other pomegranate varieties [9]. In general, the increase of polysaccharides production in plants has been associated to abiotic stresses, like water deficit, nevertheless no data are available regarding the effect of reclaimed sediment on these compounds. A greater accumulation of total soluble solids has been observed also in strawberries and pomegranates fruits from plants grown on the same reclaimed sediment [18, 26], associated to the stress induced by the sediment with unsuitable physical characteristics, such as high bulk density and low porosity. Therefore, the increase of polysaccharides contributes to the enhanced accumulation of dry weight within the fruit peel, in agreement with previous observation [18].
Fig. 3
Total polysaccharides in Purple Queen® fruit samples collected in 2017 and 2018 from the three treatments compared with a commercial sample (PQ-C). Data (mg/g DW) are the mean of a triplicate. Different letters mean statistically significant differences (p < 0.05).
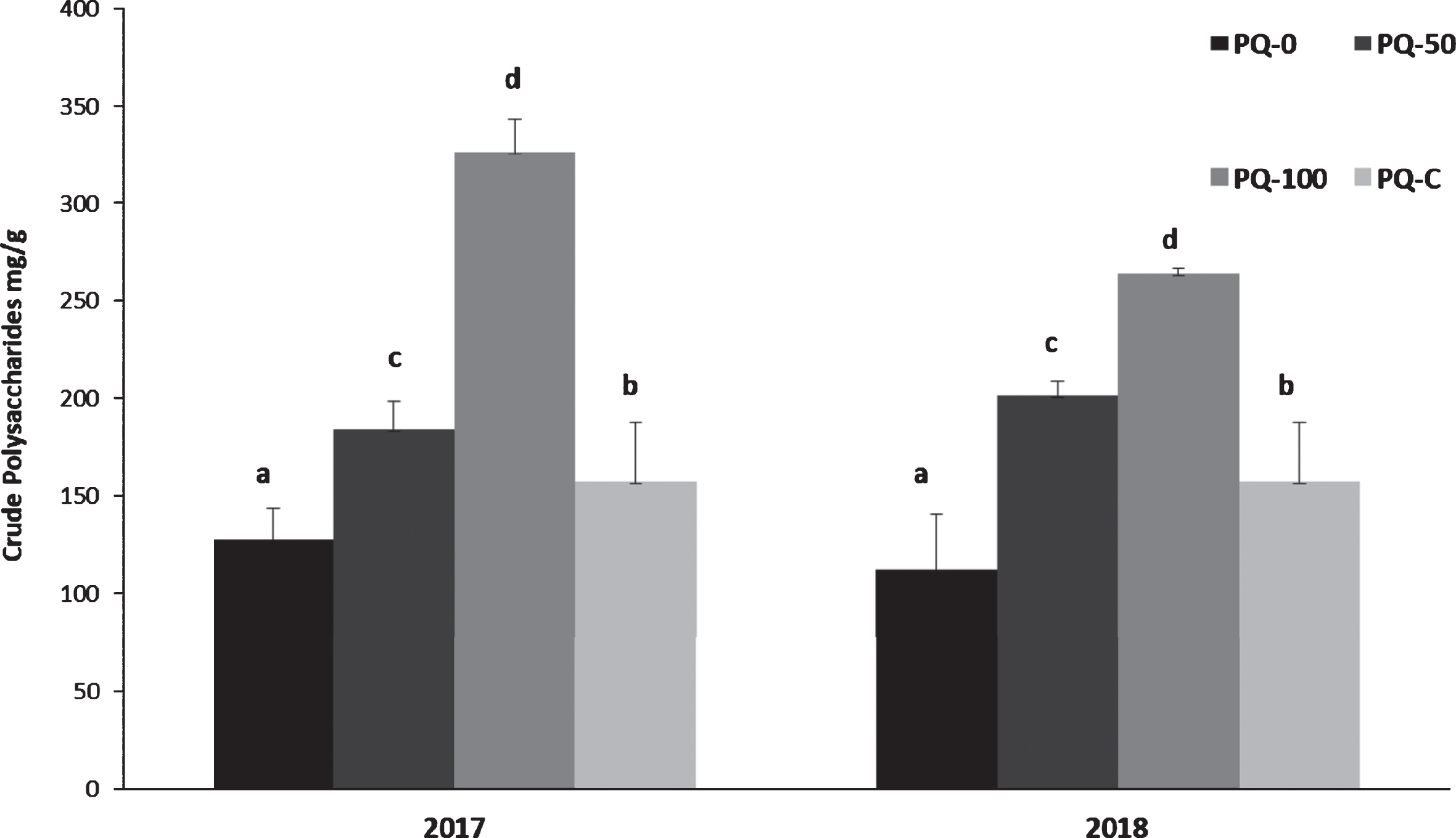
To evaluate possible changes in the apparent molecular weight (hydrodynamic volume) of the main polysaccharides, the samples were analyzed by SEC, using a pool of dextrans to determine the hydrodynamic volume of the main polysaccharides recovered after decoction from the peel of Purple Queen® samples. Figure 4, in reference to the two samples of 2017 and 2018 without the presence of sediment (PQ-0), shows very similar profiles with about 50% constituted by oligosaccharides (white column, <0.36 kDa) and approximately the other 50% by polysaccharides at high molecular weight (black column) with values >2000 kDa. On the other hand, the samples grown on 100% remediated dredged sediment show different profiles, particularly for the fruits of 2017. On the opposite, samples collected in 2018 have a similar polysaccharides distribution for all pot-grown plants with an almost superimposable profile of PQ2018-50 compared to the commercial sample (PQ-C). Therefore, concerning the biosynthesis of polysaccharides, the major changes in terms of molecular weight distribution were observed for the younger plants from 2017, while the older plants were less susceptible to the effect of the sediment.
Fig. 4
Distribution of the apparent molecular weight determined by SEC for the whole polysaccharide fractions recovered after decoction by ethanol addition; data are expressed as peak area % on total areas; H, high molecular weight; L, low molecular weight.
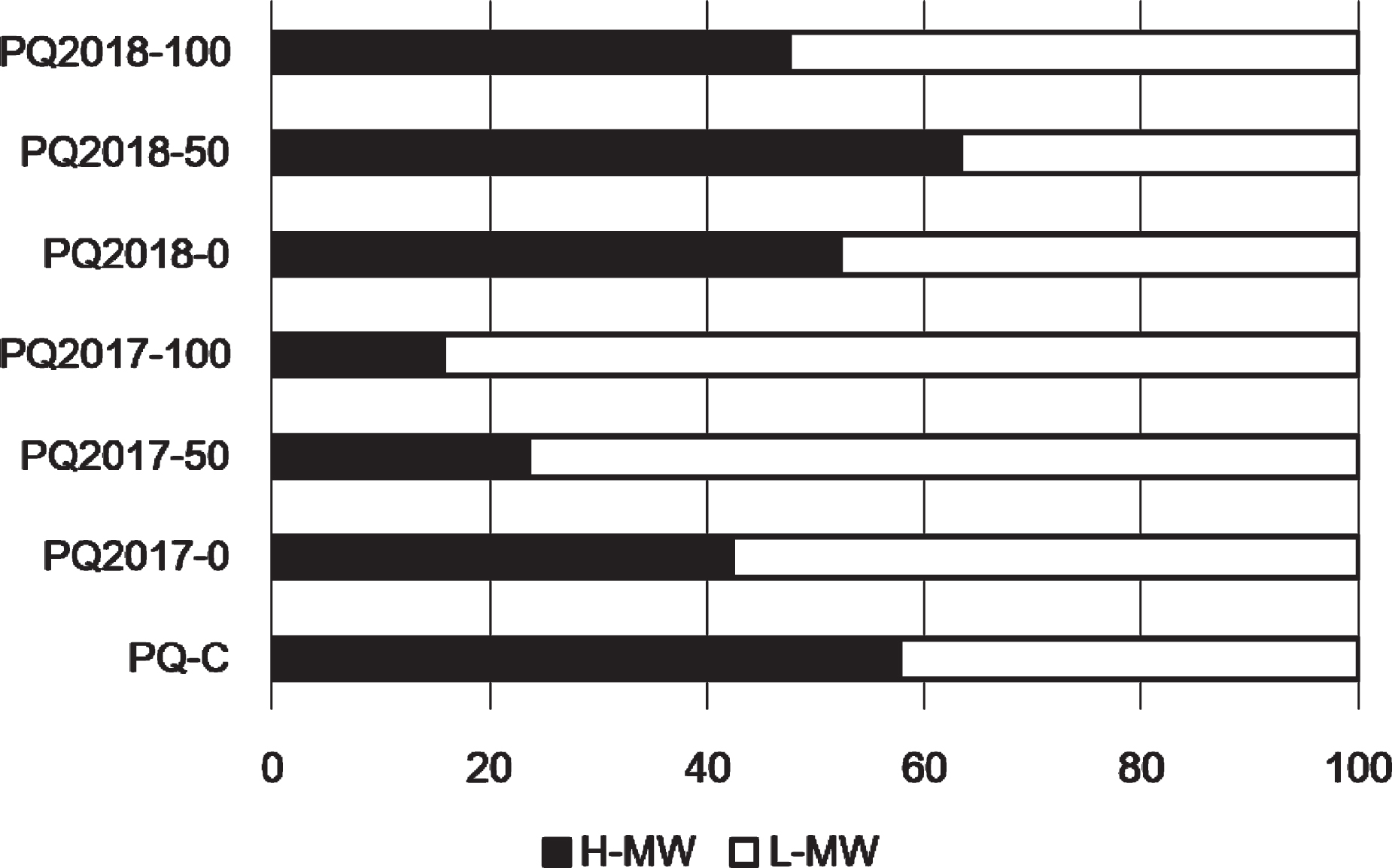
To further investigate the sugar composition of the total polysaccharide fractions, samples PQ-C and PQ2018-100 were dialysed and subjected to acidic hydrolysis. The aim was to verify if the similarity in their profiles after SEC could also be confirmed in terms of sugars content. Our findings highlighted the presence of glucose as major neutral sugar: 45% and 52% in PQ-C and PQ2018-100, respectively (Fig. 5). This result was predictable because of the presence of cellulose, which is reported as close to 20% of the total dietary fiber in pomegranate [27]. According to previous data on the absence or a very low content of starch in pomegranate fruit [28], the presence of glucose cannot be ascribable to the presence of starch. Galacturonic acid was 35% and 31% in PQ-C and PQ2018-100, respectively: galacturonic acid reported as the main polysaccharides in pomegranate [24, 29, 30] is linked to pectin structure.
Fig. 5
Sugars composition of Purple Queen® commercial (PQ-C) and Purple Queen® 2018 with 100% of sediment (PQ2018-100). The results are expressed in μmol% as a mean of a duplicate.
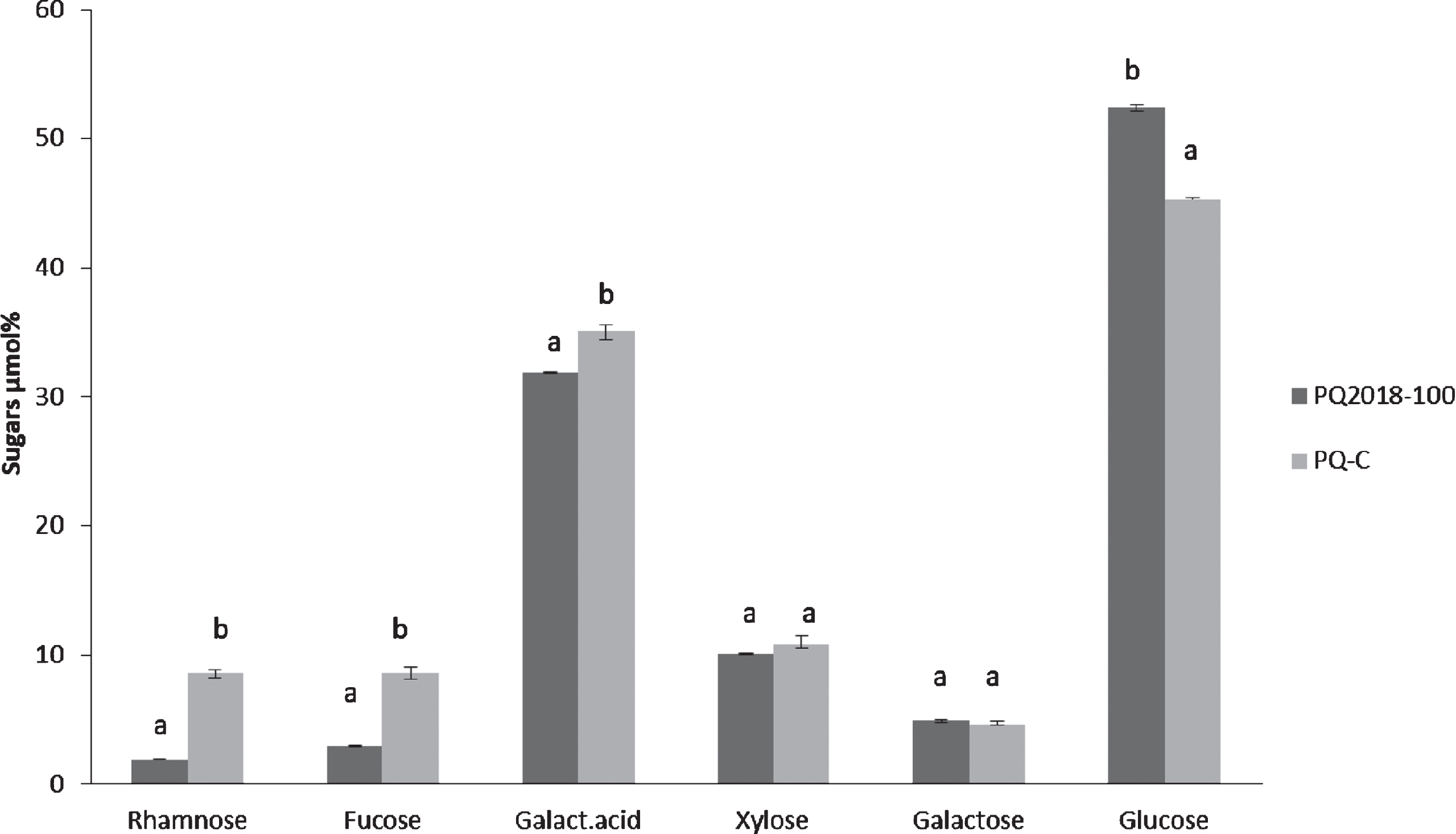
In Purple Queen, the percentage of galacturonic acid was lower than previous values obtained for other pomegranate varieties [9] and it could be related to the fact that the analysis was carried out on the total crude polysaccharides and not on a purified extract containing only pectin. Other sugars, such as rhamnose, fucose, xylose, arabinose and galactose, are present in smaller quantities, less than 10% in both the samples.
3.5Multivariate analysis
The heatmap in Fig. 6 illustrates the couples of highest similarity among the studied fruit samples obtained from the trees grown on different substrates. Namely, PQ2018-0, the control sample, resulted very similar to the commercial fruits for the morphological traits and anthocyanin and polysaccharide concentration, while the fruit content of ellagitannins was quite different. On the other hand, the fruits of the trees grown on the remediated substrate (pure or mixed with commercial peat-based substrate), are clustered because of the lower weight of fruit organs, as well as for their higher content of anthocyanins and polysaccharides. Considering the studied parameters, all the morphological traits resulted highly correlated among them; a similar situation was observed for anthocyanin and polysaccharide concentration, while the content of ellagitannins resulted less associated to the previous compounds.
Fig. 6
Heatmap based on the average values of the quantitative morphological and biochemical parameters.
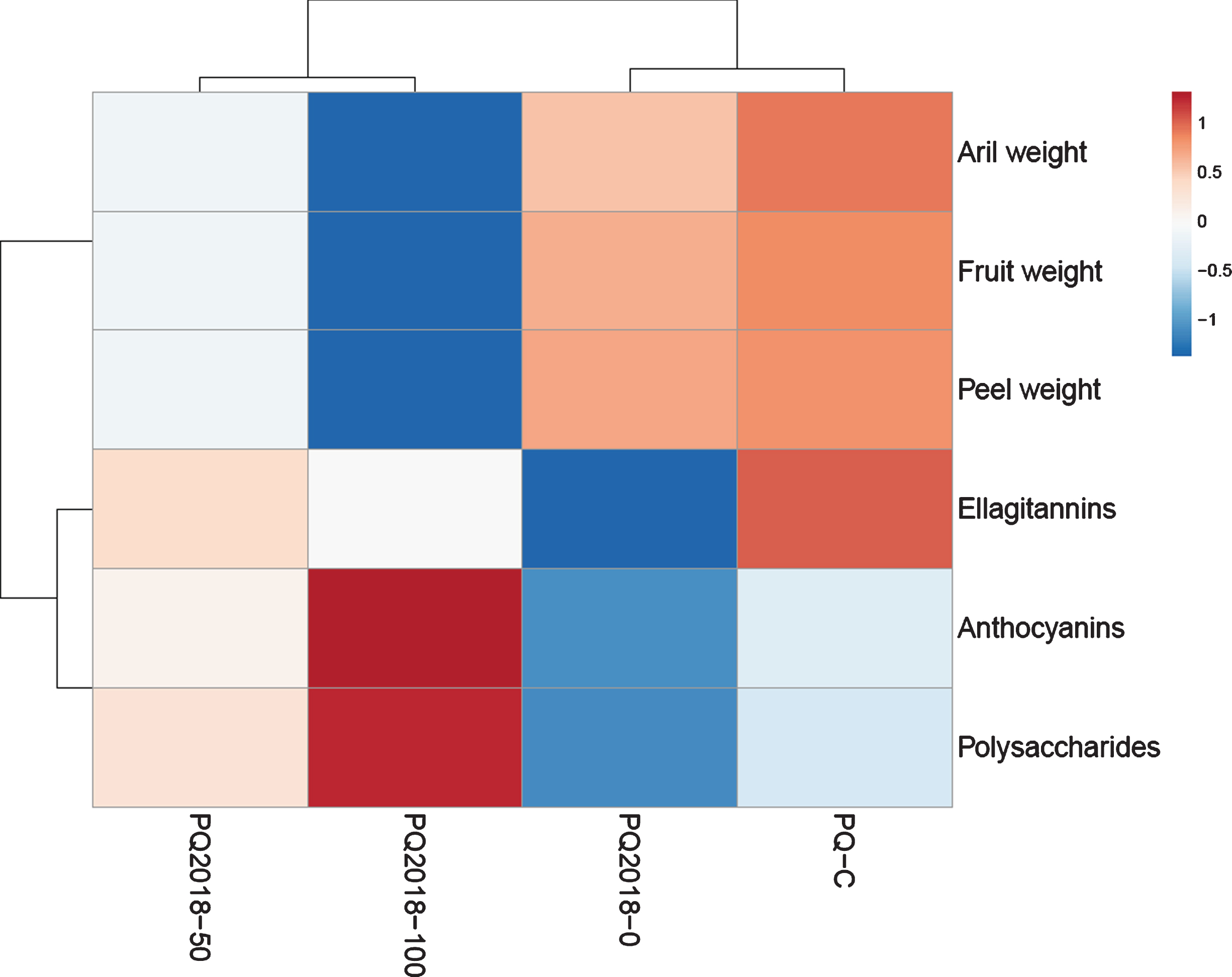
4Conclusions
In this work, the nutraceutical profile of pomegranate fruits from trees cultivated on reclaimed dredged sediments was studied for the first time. The presence of the sediment was confirmed having a detrimental effect on fruit size and weight due to its unsuitable physical-chemical characteristics. However, this negative effect has been reduced by limiting the percentage of sediments added to the soil of older plants already adapted to growth on this new mixture of peat and sediment. The investigation by mass spectrometry allowed to confirm that the qualitative phenolic profiles of anthocyanins and ellagitannins was not modified by the presence of the dredged sediment. Conversely, fruits from plants cultivated only on sediment or in a mixture with peat showed significant increases of bioactive compounds both in arils and in peel in the two consecutive seasons studied. The juice and peel showed significant higher concentrations of anthocyanins and ellagitannins, respectively. A similar trend was also found for the polysaccharide fraction, which was notably increased proportionally to the percentage of the remediated sediment with glucose and galacturonic acid as main sugars.
Although our findings pointed out a positive impact on the nutraceutical profile of the fruits, further studies are needed to better elucidate the physiological mechanisms behind the biosynthesis of the bioactive compounds in pomegranate in relation to the use of sediment in cultivation.
Conflict of interest statement
The authors have no conflict of interest to report.
Authors’ contributions
DB and FT carried out the experiments and drafted the manuscript. MK, AA, PM and GM participated in the data analysis. MI supervised the analytical aspects of this research. EG and NM designed and coordinated this research. The authors read and approved the final manuscript.
Supplementary material
The supplementary material is available in the electronic version of this article: https://dx.doi.org/10.3233/JBR-200546.
Acknowledgments and Funding
The study was supported by LIFE14 ENV/IT/000113 “HORTISED” and by Foundation Ente Cassa di Risparmio di Firenze (project code 2016.0959).
References
[1] | Pereira PHF , Oliveira TÍS , Rosa MF , Cavalcante FL , Moates GK , Wellner N , Waldron KW , Azeredo HMC . Pectin extraction from pomegranate peels with citric acid. Int. J. Biol. Macromol. (2016) ;88: :373–9. |
[2] | Hussein L , EL Mostafa G . Pomegranate: Cultivation, Pomological properties, Processing, Global Market and Health benefits. Food Sci. Technol. 2018. |
[3] | Tom FA , Selma V . Urolithins, the rescue of “old” metabolites to understand a “new” concept:Metabotypes as a nexus among phenolic metabolism, microbiota dysbiosis, and host. Mol. Nutr. Food Res. 2017;61. |
[4] | Singh B , Pal J , Kaur A , Singh N . Phenolic compounds as beneficial phytochemicals in pomegranate (Punica granatum L.) peel: A review. Food Chem. (2018) ;261: :75–86. |
[5] | Fischer UA , Carle R , Kammerer DR . Identification and quantification of phenolic compounds from pomegranate (Punica granatum L.) peel, mesocarp, aril and differently produced juices by HPLC-DAD–ESI/MSn. Food Chem. (2011) ;127: :807–21. |
[6] | Khatib M , Innocenti M , Giuliani C , Tamimi AA- , Romani A , Mulinacci N . Mesocarp and Exocarp of Laffan and Wonderful Pomegranate Varieties: By-Products as a Source of Ellagitannin. Int. J. Food Nutr. Sci. (2017) ;4: :1–7. |
[7] | Joseph MM , Aravind SR , George SK , Varghese S , Sreelekha TT . A galactomannan polysaccharide from Punica granatum imparts in vitro and in vivo anticancer activity. Carbohy. Polym. (2013) ;98: :1466–75. |
[8] | Rout S , Banerjee R . Free radical scavenging, anti-glycation and tyrosinase inhibition properties of a polysaccharide fraction isolated from the rind from Punica granatum . Bioresour. Technol. (2007) ;98: :3159–63. |
[9] | Khatib M , Giuliani C , Rossi F , Adessi A , Al-Tamimi A , Mazzola G , Gioia D Di , Innocenti M , Mulinacci N . Polysaccharides from by-products of the Wonderful and Laffan pomegranate varieties: New insight into extraction and characterization. Food Chem. (2017) ;235: :58–66. |
[10] | Ahmadi Gavlighi H , Tabarsa M , You SG , Surayot U , Ghaderi-Ghahfarokhi M . Extraction, characterization and immunomodulatory property of pectic polysaccharide from pomegranate peels: Enzymatic vs conventional approach. Int. J. Biol. Macromol. (2018) ;116: :698–706. |
[11] | Tortora K , Femia A Pietro , Romagnoli A , Sineo I , Khatib M , Mulinacci N , Giovannelli L , Caderni G . Pomegranate By-Products in Colorectal Cancer Chemoprevention: Effects in Apc-Mutated Pirc Rats and Mechanistic Studies In Vitro and Ex Vivo. Mol. Nutr. Food Res. (2018) ;62: :1–10. |
[12] | Tozzi F , Pecchioli S , Renella G , Melgarejo P , Legua P , Doni S , Masciandaro G , Giordani E , Lenzi A . Remediated marine sediment as growing medium for lettuce production: assessment of agronomic performance and food safety in a pilot experiment. J. Sci. Food Agric. (2019) ;99: :5624–30. |
[13] | Mattei P , Gnesini A , Gonnelli C , Marraccini C , Masciandaro G , Macci C , Doni S , Iannelli R , Lucchetti S , Nicese FP , Renella G . Phytoremediated marine sediments as suitable peat-free growing media for production of red robin photinia (Photinia x fraseri). Chemosphere. (2018) ;201: :595–602. |
[14] | DelValls TA , Andres A , Belzunce MJ , Buceta JL , Casado-Martinez MC , Castro R , Riba I , Viguri JR , Blasco J . Chemical and ecotoxicological guidelines for managing disposal of dredged material. Trends Anal. Chem. (2004) ;23: :819–28. |
[15] | Bert V , Seuntjens P , Dejonghe W , Vandecasteele B . Phytoremediation as a management option for contaminated sediments in tidal marshes, flood control areas and dredged sediment landfill sites. Environ. Sci. Pollut. Res. (2009) ;16: :745–64. |
[16] | Masciandaro G , Biase A Di , Macci C , Peruzzi E , Iannelli R , Doni S . Phytoremediation of dredged marine sediment: Monitoring of chemical and biochemical processes contributing to sediment reclamation. J. Environ. Manage. t. (2014) ;134: :166–74. |
[17] | Doni S , Macci C , Peruzzi E , Iannelli R , Masciandaro G . Heavy metal distribution in a sediment phytoremediation system at pilot scale. Ecol. Eng. (2015) ;81: :146–57. |
[18] | Melgarejo P , Legua P , Pérez-Sarmiento F , Martínez-Font R , Martínez-Nicolás JJ , Giordani E , Tozzi F , Hernández F . Effect of a new remediated substrate on bioactive compounds and antioxidant characteristics of pomegranate (Punica granatum L.) cultivar ‘Purple Queen’. Arch. Agron. Soil Sci. (2019) ;65: :1565–74. |
[19] | Chamizo S , Adessi A , Mugnai G , Simiani A , Philippis R De . Soil Type and Cyanobacteria Species Influence the Macromolecular and Chemical Characteristics of the Polysaccharidic Matrix in Induced Biocrusts. Microb. Ecol. (2018) ;78: :482–93. |
[20] | Nunes FM , Coimbra MA . Chemical Characterization of the High Molecular Weight Material Extracted with Hot Water from Green and Roasted Arabica Coffee. J. Agric. Food Chem. (2001) ;49: :1773–82. |
[21] | Balli D , Cecchi L , Khatib M , Bellumori M , Cairone F , Carradori S , Zengin G , Cesa S , Innocenti M , Mulinacci N . Characterization of Arils Juice and Peel Decoction of Fifteen Varieties of Punica granatum L. : A Focus on Anthocyanins, Ellagitannins and Polysaccharides. Antioxidants. (2020) ;9: (3)pii:E238. doi: 10.3390/antiox9030238 |
[22] | Cásedas G , Les F , González-burgos E , Gómez-serranillos MP , Smith C , López V . Cyanidin-3-O-glucoside inhibits different enzymes involved in central nervous system pathologies and type-2 diabetes. S. Afr. J. Bot. (2019) ;120: :241–46. |
[23] | Alighourchi H , Barzegar M . Anthocyanins characterization of 15 Iranian pomegranate (Punica granatum L.) varieties and their variation after cold storage and pasteurization. Eur. Food Res. Technol. (2008) ;3: :881–7. |
[24] | Singh B , Singh JP , Kaur A , Singh N . Phenolic compounds as beneficial phytochemicals in pomegranate (Punica granatum L.) peel: A review. Food Chem. (2018) ;261: :75–86. |
[25] | Shakhmatov EG , Makarova EN , Belyy VA . Structural studies of biologically active pectin-containing polysaccharides of pomegranate Punica granatum. Int. J. Biol. Macromol. (2019) ;122: :29–36. |
[26] | Tozzi F , Del Bubba M , Petrucci WA , Pecchioli S , Macci C , Hernández García F , Martínez Nicolás JJ , Giordani E . Use of a remediated dredged marine sediment as a substrate for food crop cultivation: Sediment characterization and assessment of fruit safety and quality using strawberry (Fragaria x ananassa Duch.) as model species of contamination transfer. Chemosphere. (2020) ;238: :124651. |
[27] | Hasnaoui N , Wathelet B , Jiménez-araujo A . Valorization of pomegranate peel from 12 cultivars: Dietary fibre composition, antioxidant capacity and functional properties. Food Chem. (2014) ;160: :196–03. |
[28] | Gupta U , Solanki H . Quantification of ash and selected primary metabolites from parts of several fruits. International Int. J. Pharm. Pharm. Sci. (2015) ;7: :288–90. |
[29] | Abid M , Cheikhrouhou S , Renard CMGC , Bureau S , Cuvelier G , Attia H , Ayadi MA . Characterization of pectins extracted from pomegranate peel and their gelling properties. Food Chem. (2017) ;215: :318–25. |
[30] | Zhai X , Zhu C , Zhang Y , Sun J , Alim A , Yang X . Chemical characteristics, antioxidant capacities and hepatoprotection of polysaccharides from pomegranate peel. Carbohy. Polym. (2018) ;202: :461–69. |