Organoleptic, nutritional and anti-carcinogenic characteristics of the fruit and rooting performance of cuttings of black mulberry (Morus nigra L.) genotypes
Abstract
BACKGROUND:
Black mulberry fruits have high antioxidant capacity, exhibiting health protective properties. In order to exploit their entire dynamic though, elite genotypes must be vegetatively propagated.
OBJECTIVE:
The purpose was to assess the physiological, organoleptic, phytochemical and anti-carcinogenic (only of the best two genotypes) properties of eleven new black mulberry genotypes, compared to the elite hybrid Fengchisang and the possibility of easy asexual propagation through semi-hardwood and hardwood cuttings.
METHODS:
Fruits from eleven genotypes were analyzed for their organoleptic and physiological characteristics as well as for their total phenol, o-diphenol, flavonoid, flavanol, anthocyanin content and their organic acid and sugar composition. The best two were also tested for anti-carcinogenic properties. Furthermore, the rooting potential of semi-hardwood and hardwood cuttings was also investigated.
RESULTS:
The genotypes differed regarding fruit characteristics and rooting potential. The principal component analysis lead to a grouping of genotypes, while one, ANS8, was grouped separately, exhibiting high antioxidant capacity and phenol content. ANS8 genotype reduced cancer cells viability more efficiently compared to Fengchisang.
CONCLUSIONS:
The ANS8 genotype exhibited the highest antioxidant capacity, higher than Fengchisang and better anti-carcinogenic properties, which along with the ease of rooting, may become a valuable source of health promoting phytochemicals.
1Introduction
The mulberry tree (Morus sp.) is a perennial, economically important fruit tree species easily adapted in various climates, such as tropical, subtropical and temperate climate [1– 3]. There are many species within Morus genus, with the most cultivated ones being the white mulberry (Morus alba L.), the black mulberry (Morus nigra L.) and the red mulberry (Morus rubra L.). The white mulberry is widely known, as its leaves have been used, since many centuries, as the exclusive feed for silkworms (Bombix mori L.). Black mulberry is cultivated in many countries around the world for its fruits, which are usually consumed fresh or frozen or are constituents of jams, syrup, ice-cream, liquors, while they are also used as color additives in food industry and for medicinal purposes in pharmaceutical industry [1, 4].
Recently a growing interest on black and white mulberries emerged concerning their potential benefits on human health, due to their biologically active substances conferring antioxidant, antimicrobial, antihyperglycemic, antiviral, hypolipidemic, neuroprotective, anti-inflammatory, antimutagenic and anti-carcinogenic properties [2, 5– 8]. Recent studies have revealed that black mulberry fruits have positive effects on human health due to their content in ascorbic acid, minerals, phenols, and flavonoids, some organic acids and anthocyanin content, all contributing to the high antioxidant properties of the fruit [5, 9].
Phytochemical content and antioxidant capacity of the fruits are influenced by the combined effect of genetic and pedoclimatic factors as well as cultivation techniques [3]. Therefore, in order to exploit mulberry fruit dynamic, orchards of elite clones should be planted. However, as sexual propagation is undesirable due to the enormous heterozygosis of F1 plants, mulberry cultivars must be asexually propagated. According to Zenginbal and Eşitken [10] propagation through cuttings is unsuccessful due to low rooting potential, which might be due to physiological and environmental factors [11]. In fact, there are great differences between black and white mulberry trees, as black mulberry is considered to be more difficult for vegetative propagation, while variable results have been reported in the literature [11].
As in any other fruit species, mulberry fruit trade is driven by both fresh market and processing industry requirements. Therefore, the introduction of new cultivars to the market should meet both producer needs (i.e. easy to propagate and grown, high yields, etc) as well as market demands for high-quality products (good sensory characteristics and high contents of biologically active compounds). Therefore, the aim of the present study was a) to investigate the rooting dynamic of eleven new black mulberry genotypes by the use of both hardwood and semi-hardwood leafy cuttings, b) to compare their fruit organoleptic, physiological and phytochemical characteristics against an elite international hybrid i.e. Fengchisang (Zhongsang 5801×5801×Huasang) [12] and c) to assess the anti-carcinogenic action of the best two genotypes, based on their phytochemical properties.
2Materials and methods
2.1Plant material – rooting experiments
Eleven black mulberry genotypes, derived as random crosses, were evaluated in the present study. All genotypes were chosen based on high yield production of black mulberry fruits. The genotypes were growing in Attika prefecture, Greece. Mother plants were severely pruned every year in order to induce the production of juvenile shoots. Semi-hardwood shoots were harvested during late August and 15 cm leafy cuttings were cut from the basal and middle part of the shoot. Only two leaves at the top of each cutting were kept and trimmed half in order to reduce transpiration area. Half of the cuttings produced served as control, by dipping their base into tap water for few seconds, while the bases of the other half cuttings were treated with auxin (2000 ppm of indole-3-butyric acid (IBA) powder, commercial name Rooton A, as this concentration has been found to be optimum for rooting of mulberry cuttings [11]). Cuttings were then placed into a mist system in a peat:perlite (1 : 3) substrate. The base of the cuttings were kept at a temperature of 24±2 C, while the mist system operated for 14 hours per day, providing mist every 15 min for 10 seconds. Cuttings’ rooting dynamic was evaluated after two months, when the rooting percentage, root number and length were recorded.
In early winter (early November) hardwood cuttings were collected from the same trees and the remaining senescent leaves were hand-removed. Shoots were cut into 20 cm cuttings, which were treated as described above (control and IBA treated cuttings). The cuttings were then planted in a bottom-heated cold frame, where the base temperature was kept at 21±0.5 C by the use of a proportional– integral– derivative (PID) controller. Perlite was used as substrate and it was kept moist during the entire period and the rooting dynamic of the cuttings was evaluated three and a half months later, in mid February. The rooting percentage and the root number and length were recorded.
During the vegetative period at least 10 mature, fully grown leaves were sampled from each genotype and the following phenotypical characteristics were assessed; the number of lobes, length of central lobe, leaf length, pedicel length and leaf width, in order to be used as morphological traits for genotype discrimination.
2.2Fruit sampling
Rooted cuttings from both methods (leafy semi-hardwood and leaf-less hardwood cuttings) were used as the starting material for the establishment of an orchard of the 11 genotypes to be evaluated.
Three years later, fruits from bearing trees of these genotypes were collected at fully mature stage. Harvest took place in three cycles (“hands”). Fruits sampled were immediately transferred to the laboratory under low temperature, in an insulated box filled with frozen gel packs. At the same time fruits from the elite hybrid Fengchisang grown at the same orchard, were harvested and served as the market control, since this cultivar has shown exceptionally good quality characteristics [12].
2.3Physiological parameters determination
Individual fruit weight, diameter and length was measured by the use of a calibrated balance (Kern 470, Kern and Sohn, GmbH, Germany) and digital caliper (Starrett, 727 Series, Athol, New England, USA). The fruits were then homogenized with a house-hold homogenizer and put in a freezer at – 24±2 C till analyses. The percent dry matter of fruits was also determined after drying them in an oven at 70 C till constant weight.
2.4Organoleptic parameters determination
A small fraction of the homogenous mixture was centrifuged at 4000 g for 6 min and the supernatant clear juice was analyzed for total soluble solids (TSS), pH and titratable acidity (TA). TSS was evaluated at 20 C with a Hanna HI96801 digital refractometer (Hanna Instruments, Woonsocket, Rhode Island, United States) and expressed as Brix. pH was measured after dilution of the juice at a ratio 1 : 20 with distilled water. TA was determined in that diluted juice solution by titrating to pH 8.2 using 0.1M NaOH.
2.5Total phenol content, total anthocyanin content and antioxidant capacity determination
Approximately 0.5 g of the frozen mulberry fruit mixture were extracted twice with 5 mL 75% (v/v) ethanol under periodical stirring at 45 C. After centrifugation the supernatant was assessed for total phenol and total o-diphenol content according to Roussos et al. [13] and the results expressed as mg equivalent gallic acid (GAE) and caffeic acid (CAE) per g fresh weight (FW), respectively. The total flavonoid content was determined according to Meyers et al. [14], while the total flavanol content was determined according to Arnous et al. [15] and the results expressed as mg equivalent caffeic acid and catechin (CtE) per g FW, respectively. For the total anthocyanin content, a portion of the frozen mixture was extracted twice with 5 mL each time of acidified 80% acetone with 1% (v/v) concentrated HCl for 1 h at 4 C under periodical stirring. The total anthocyanin content was assessed based on pH differentiation method. Results were expressed as mg cyanidine 3-glucoside equivalents (C-3-GE)/100 g fresh weight (MW = 449 and e = 26900 L mol–1 cm–1, based on Beer-Lambert law, i.e. Absorbance = e * C * L, where e is the extinction coefficient of the measured substance, C its concentration in Molarity and L the length of cuvette path)[12].
The antioxidant capacity of mulberry fruits was evaluated in the supernatant produced by the extraction of phenolic compounds using the DPPH and FRAP assays [13]. The antioxidant capacity was determined by using the chromogenic radical DPPH (2,2-diphenyl-1-picryl hydrazyl) [16]. To 0.1 mL of the diluted juice sample (dilution 1 : 5 with 75% (v/v) ethanol) 2 mL of 0.1 mM DPPH solution was added and the absorbance was measured, after 60 min in the dark at room temperature, at 517 nm. The scavenging capacity of the extracts was expressed in μmol Trolox equivalents (TE) per g fresh weight.
For the FRAP assay 1.5 mL of freshly prepared FRAP reagent was added to 50 μl of the extract and incubated at 37 C for 30 min before the absorbance being measured at 593 nm. Trolox standard dilutions were used to express the antioxidant capacity as μmoles TE per g fresh weight.
2.6Organic acid determination
For the organic acid determination a sample of approximately 0.5 g of frozen fruit mixture was extracted twice with 5 mL 3% (w/v) metaphosphoric acid in water at room temperature for 30 min with periodical stirring. At the end of every 30 min period the mixture was centrifuged and the two supernatants were combined and filtered through a 0.45 μm pore nylon syringe filter. The analysis of organic acids took place in an HPLC system (HP1050, Agilent, Santa Clara, California, United States) equipped with a UV detector (series 1050, Agilent Santa Clara, California, United States) operating at 200 nm and a Li-Chrospher RP18 column (250 mm x 4.6 mm, 5 μm, Merck Kenilworth, New Jersey, United States), which was eluted isocratically at a flow rate of 1 mL min–1 with a mobile phase of 0.02% (v/v) formic acid in water. The identification of organic acids was based on their retention times compared with those of authentic standards. Four major organic acids were determined, namely citric (CA), malic (MA), fumaric (FA) and ascorbic acid (ASA) while the total organic acid (TOA) concentration was also calculated.
2.7Soluble sugars determination
Soluble sugar extraction and determination was performed by the method described by Roussos et al. [13]. For the HPLC analysis, a Waters 510 isocratic pump (Milford, Massachusetts, United States) was used at a flow rate 0.6 mL min–1 of water, while the separation of the carbohydrates was achieved through a Hamilton HC-75 cation exchange column, calcium form (Ca 2 +)(305 mm x 7.8 mm, 9 μm) (Hamilton, Bonaduz, Switzerland), equilibrated at 80 C. Three soluble sugars were detected (HP1047A, refractive index detector, Agilent, Santa Clara, California, United States) in the studied mulberry genotypes, sucrose, glucose and fructose. Total sugar concentrations were estimated by summing the concentrations of the individual sugars detected by HPLC.
The sweetness index (SI) of the fruit, an estimate of the total sweetness perception, was calculated based on the relative amount and sweetness properties of each individual carbohydrate [13]. The contribution of each carbohydrate to sweetness perception was calculated based on the fact that sucrose is 1.35 times sweeter than glucose and fructose 2.3 times sweeter than glucose and hence the SI was calculated as [1.00 * (glucose concentration) +1.35 * (sucrose concentration) +2.3 * (fructose concentration)].
2.8Cell culture and viability assay
The epithelial human breast adenocarcinoma cell line -MCF-7 (ATCC® HTB-22trademark) (estrogen-receptor positive, progesterone receptor positive and HER2 negative), and the epithelial human cervical aggressive adenocarcinoma cell line- HeLa (ATCC® CRM-CCL-2trademark), were purchased by the American Tissue Culture Collection (ATCC, Manassas, Virginia, USA) and were grown as monolayers in adherent cultures in a humidified incubator at 37°C, 5% CO2. The culture medium consisted of 90% Dulbecco’s Modified Eagle Medium (Biochrom Gmbh, Berlin, Germany) supplemented with 10% Fetal Bovine Serum (Thermo Fisher Scientific, Waltham, MA, USA), 2 mM l-glutamine, 0.5 mM sodium pyruvate and 1% Penicillin-Streptomycin (Biowest, Nuaillé, France).
Cytotoxicity of the berry ethanolic extracts on cells treated for 24 and 48 hours was determined using the MTT (3-(4,5-dimethylthiazol-2-yl)-2,5-diphenyltetrazolium bromide) colorimetric assay to assess cell viability in accordance with a modified protocol of the method described by Mossman [17]. The cells were seeded in 96 cell culture well plates (SPL LIFE SCIENCES Co., Ltd., Pocheon-si, Korea) (8,000 and 6,000 cells per well for 24 and 48 h incubation respectively) and allowed to adhere overnight. The next day cells were treated with different concentrations (0.5, 1, 1.5, 2, 2.5 mg/mL) of lyophilized ethanolic berry extracts (genotypes ANS8 and Fengchisang) in standard culture medium with 1% FBS for 24 and 48 h (in a total volume of 200 μl per well). The final solvent concentration in the medium was 0.2% and each concentration was tested in five replicates. Then, 0.5 mg/mL MTT (Duchefa Biochemie, Haarlem, Netherlands) was added and cells were incubated with the dye for 3 hours. After incubation, the medium was removed and cells were solubilized with 200 μl dimethylsulfoxide (DMSO) per well. Absorbance was taken at a PowerWave240 plate reader (BioTek, Winooski, VT, USA) at 560 nm. The experiment was repeated independently three times for each treatment and the mean results expressed as % cell viability normalized to biological control.
2.9Trial design and statistical analysis
The rooting experiments followed the completely randomized design, with four replications of at least 15 cuttings per replication. Data were analyzed as a two way Anova, with the first factor being the genotype and the second the auxin concentration. Data on organoleptic, physiological and phytochemical analyses were analyzed as an one way Anova, with the factor being the genotype, with three replications. Statistically significant differences were determined using the Tukey HSD test at α= 0.05. Correlation analysis took place in order to evaluate the relationship between antioxidant capacity and phenolic compounds assayed.
Principal component analysis (PCA) based on fruit organoleptic, physiological and phytochemical characteristics was used to produce eigenvectors and principal component scores in order to evaluate the relative discriminative power of the axes (principal components) and to describe the genotypes and the hybrid by a limited number of parameters. Discriminant analysis was also performed in order to classify the genotypes and hybrid into groups using the same data as PCA.
In order to evaluate possible relationship among clones, a dendrogram was constructed using the group average method in cluster analysis. Data on leaf phenotypical characteristics i.e. the number of lobes, length of central lobe, leaf length, pedicel length, leaf width, along with the fruit organoleptic, physiological and phytochemical parameters and cuttings’ rooting performance were loaded in order to construct the dendrogram. For the figures standard errors (S.E.) were calculated from the residual variances of the two way Anova. Data on cell viability were subjected to Student’s T-test in order to assess significant differences. All test comparisons were made at probability level p < 0.05.
The statistical analyses were performed using the Statgraphics Centurion XVII statistical software (Statpoint Technologies Inc., Warrenton, USA).
3Results
3.1Rooting experiments
The genotype had a significant effect on the rooting performance of softwood cuttings as can be seen in Fig. 1. Auxin on the other hand significantly influenced rooting percentage and the number of roots, but not their mean length, while the same stood for the interaction between genotype and auxin. The genotypes ANS7, ANS8 and AUA20 exhibited the highest rooting percentages (reaching almost 60%), while ANS12 proved to be quite recalcitrant to root. In most genotypes treatment of cutting’ base with auxin did not improve rooting any further, while the highest rooting percentage was recorded with ANS8 reaching 63.5% rooted cuttings under no auxin treatment. The root number was highest in ANS6 genotype reaching 14.6 roots per rooted cutting, under the influence of IBA (Fig. 1B). It was clear that IBA treatment enhanced the production of roots, resulting in a rich root system compared to control. The genotypes H3, ANS6, ANS8 and ANS9 presented the longest roots (Fig. 1C) while there were not any significant differences between IBA treated cuttings and control ones regarding root length.
Fig.1
Effect of genotype and auxin treatment on the rooting performance of mulberry softwood leafy cuttings. The bars at the right side of each graph are the standard error of the multifactor Anova. Abbreviations: G, genotype, A, auxin, x, denotes the interaction, ns, not significant, **, p < 0.01, ***, p < 0.001.
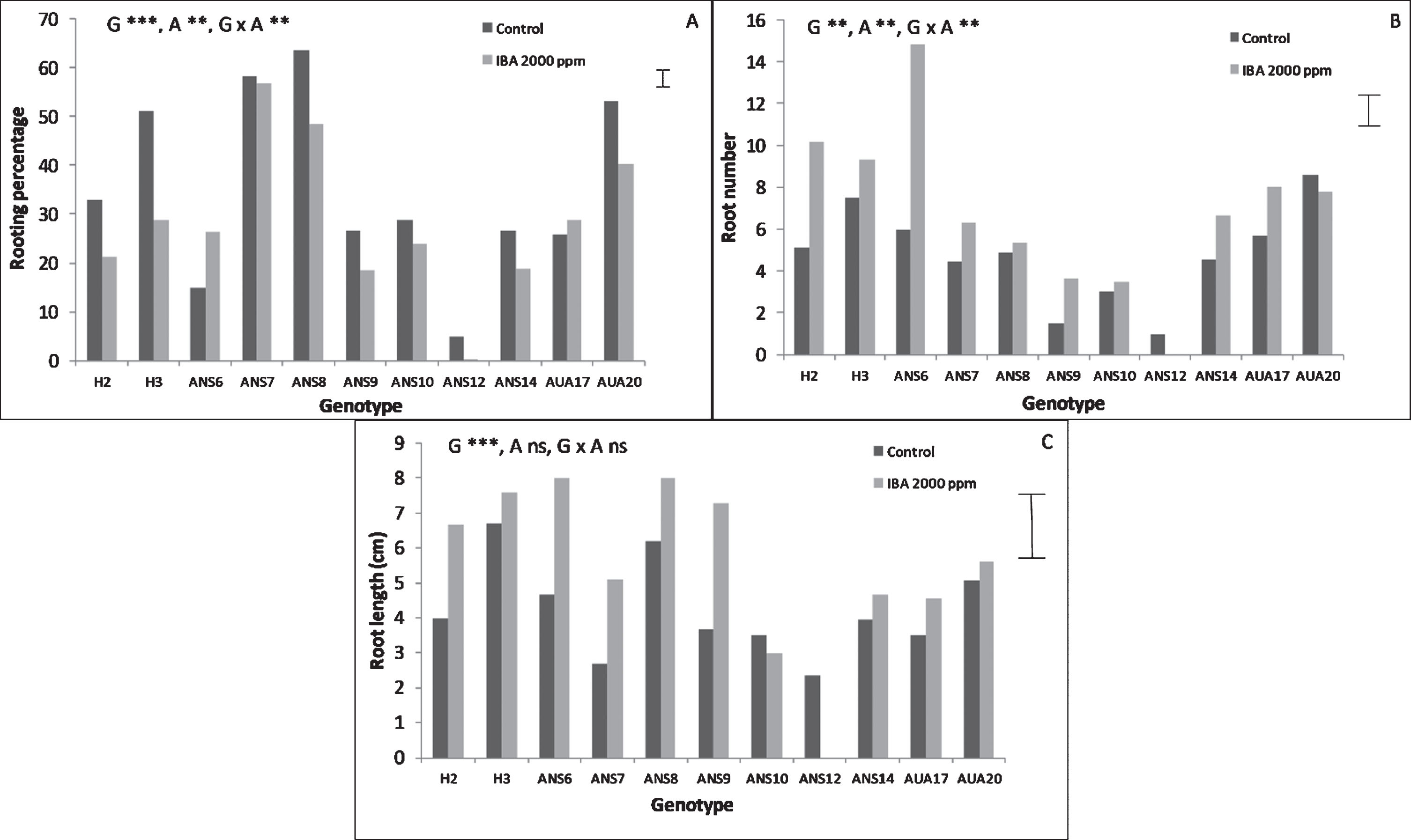
Genotype, auxin treatment as well as their interaction played a significant role in the rooting performance of the hardwood cuttings (Fig. 2). The genotypes H2, H3, ANS7, ANS8 and AUA17 presented the highest rooting percentages, reaching almost 85% (ANS7). Auxin treatment proved to be critical in inducing rooting of hardwood cuttings, since many clones failed to root in the absence of auxin (Fig. 2A). The number of roots was highest in ANS8 under the effect of IBA, reaching almost 14.8 roots per rooted cutting. Auxin treatment induced the formation of more roots than control (Fig. 2B). Root length was highest in H3 under control conditions, reaching almost 8 cm in length. In general though, auxin treatment induced longer roots than control (Fig. 2C).
Fig.2
Effect of genotype and auxin treatment on the rooting performance of mulberry hardwood cuttings. The bars at the right side of each graph are the standard error of the multifactor Anova. Abbreviations: G, genotype, A, auxin, x, denotes the interaction, **, p < 0.01, ***, p < 0.001.
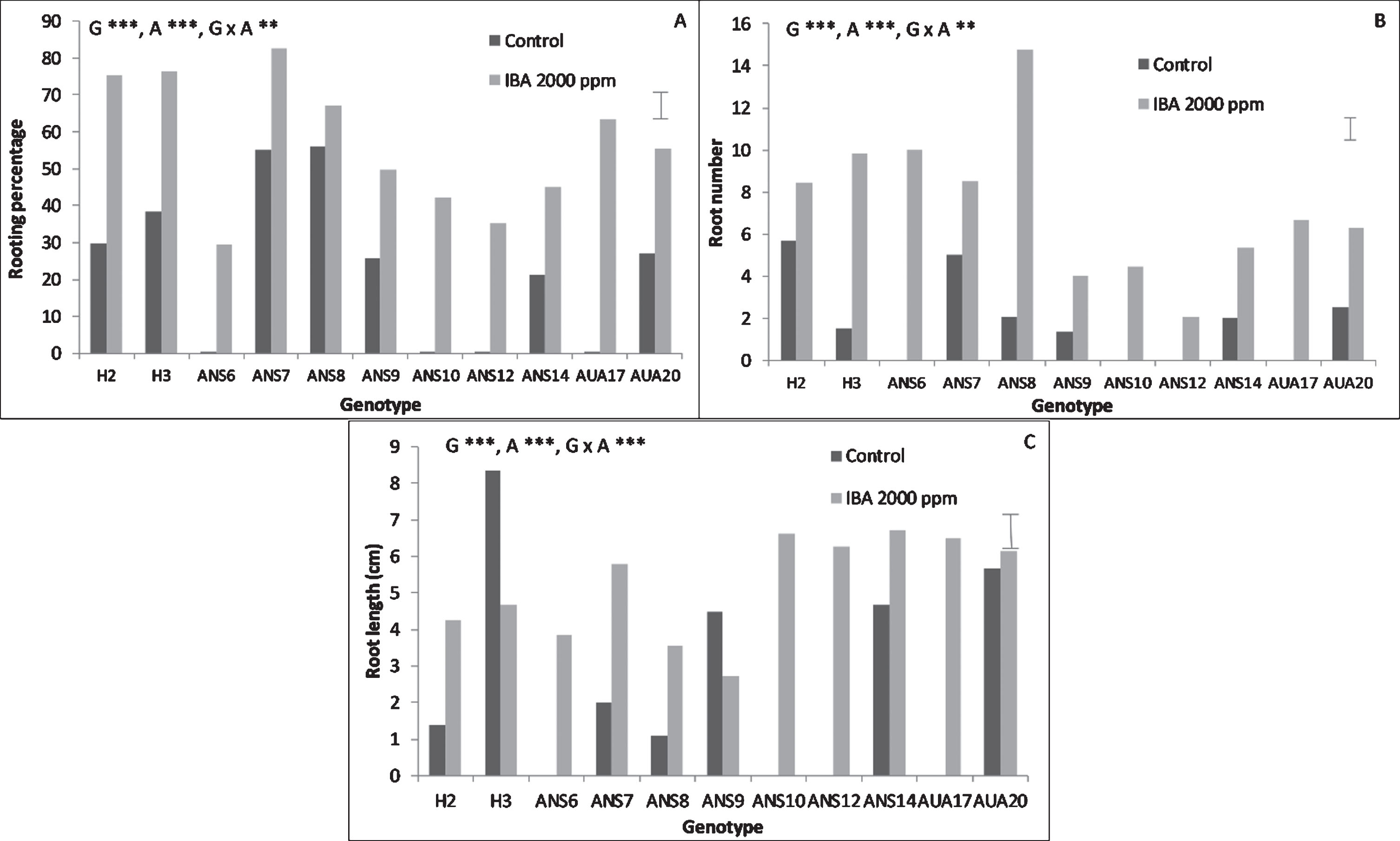
3.2Physiological, organoleptic and phytochemical characteristics of the fruits.
Most genotypes produced fruits with an average weight of approximately 2– 2.5 g (Table 1). The largest fruits though were harvested from genotype H2, which was initially selected for its large berries, since it also exhibited the highest diameter and length among clones. Similar length was measured also in Fengchisang. The highest diameter:length ratio was determined in the fruits of genotypes ANS12 and AUA20, which were more round than the other clones, while the most oblong fruits were produced by Fengchisang. The DW ranged from the low 11.61% in ANS8 to the highest 22.62% in ANS10, with significant differences between them (Table 1).
Table 1
Physiological characteristics of the studied mulberry genotypes
Genotypes | Weight (g) | Diameter (mm) | Length (mm) | Diameter/Length | DW (%) |
ANS10 | 2.18bc | 14.35bcd | 21.17c | 0.68ab | 22.62a |
ANS12 | 2.75bc | 16.15bc | 21.41bc | 0.75a | 17.75ab |
ANS14 | 3.29b | 16.21b | 25.71abc | 0.63bc | 17.77ab |
ANS6 | 2.18bc | 13.28d | 25.27abc | 0.53d | 14.51bc |
ANS7 | 2.06c | 13.56d | 22.04bc | 0.62bc | 14.83bc |
ANS8 | 2.34bc | 15.21bcd | 22.27bc | 0.68ab | 11.61c |
ANS9 | 2.86bc | 14.97bcd | 24.75abc | 0.61bc | 13.82bc |
AUA17 | 2.64bc | 14.04d | 26.68ab | 0.53d | 14.70bc |
AUA20 | 2.28bc | 15.15bcd | 20.88bc | 0.73a | 12.60bc |
Fengchisang | 3.09bc | 14.26cd | 28.11a | 0.51d | 13.10bc |
H2 | 4.73a | 18.17a | 29.88a | 0.61bcd | 17.64ab |
H3 | 2.88bc | 14.87bcd | 25.16abc | 0.59cd | 15.72bc |
Means within the same column followed by the same letter do not differ significantly based on Tukey HSD at α= 0.05. Abbreviations: DW, dry weight.
The highest total soluble solid content was determined in the fruits of ANS10 genotype, while the lowest one in ANS8 (Table 2). The latter exhibited the highest TA too, followed by ANS6, while the lowest was determined in fruits of ANS14 and H2 genotypes. The ratio TSS/TA, which represents in many fruits the maturation index, was highest in ANS10, reaching 102.43, while the lowest was determined in ANS8 with only 12.60. The pH of the juice ranged between 4.07 (ANS8) and 6.03 (ANS10).
Table 2
Organoleptic characteristics of the studied mulberry genotypes
Genotypes | TSS (Brix) | TA (% citric acid) | TSS/TA | pH |
ANS10 | 18.35a | 0.18d | 102.43a | 6.03a |
ANS12 | 14.20bc | 0.17d | 86.52ab | 5.45ab |
ANS14 | 16.20ab | 0.16d | 107.68a | 6.07a |
ANS6 | 11.13cd | 0.56a | 21.52d | 4.27d |
ANS7 | 12.53cd | 0.23cd | 57.35abcd | 5.20bc |
ANS8 | 7.33e | 0.59a | 12.60d | 4.07d |
ANS9 | 10.55de | 0.29bcd | 41.21bcd | 5.09bcd |
AUA17 | 11.73cd | 0.41abc | 29.71cd | 4.54cd |
AUA20 | 10.90de | 0.26bcd | 41.92bcd | 5.70ab |
Fengchisang | 11.10cd | 0.45ab | 25.39d | 4.29d |
H2 | 13.43bcd | 0.16d | 92.98ab | 5.61ab |
H3 | 12.60cd | 0.17d | 79.18abc | 5.50ab |
Means within the same column followed by the same letter do not differ significantly based on Tukey HSD at α= 0.05. Abbreviations: TSS, total soluble solids, TA, titratable acidity.
The highest total phenols, total o-diphenols and total flavonoids concentration was determined in the fruits of ANS8, while the lowest in H3 (total phenols and total flavonoids) and in H2 (total o-diphenols) (Table 3). ANS10 fruits presented the highest total flavanol content with the lowest being found in ANS7. The richest fruits in anthocyanins proved to be those of ANS8, followed by Fengchisang. The same was also determined concerning the antioxidant capacity of the fruits, with fruits of ANS8 exhibiting the highest antioxidant capacity, followed by those of Fengchisang.
Table 3
Total phenol, o-diphenol, flavonoid and flavanol concentration, and antioxidant capacity (DPPH and FRAP) of the studied mulberry genotypes
Genotypes | Phenols | o-diphenols | Flavonoids | Flavanols | Anthocyanins | DPPH | FRAP |
(mg GAE g–1 FW) | (mg CAE g–1 FW) | (mg CAE g–1 FW) | (mg CtE g–1 FW) | (mg C-3-GE 100 g–1 FW) | (μmol TE g–1 FW) | ||
ANS10 | 4.49bcd | 2.11bcd | 0.87bc | 0.32a | 8.79e | 5.80b | 16.01d |
ANS12 | 6.75ab | 2.98bc | 0.91bc | 0.22bcd | 128.39bc | 14.69a | 19.97d |
ANS14 | 3.24cd | 1.75def | 0.57cd | 0.31ab | 9.15e | 5.80b | 14.90d |
ANS6 | 6.29b | 2.57bcd | 0.88bc | 0.18cd | 131.18bc | 14.45a | 44.28bc |
ANS7 | 2.73cd | 1.03f | 0.39d | 0.13d | 7.76e | 3.98b | 10.85d |
ANS8 | 8.98a | 4.34a | 1.33a | 0.19cd | 403.44a | 17.61a | 82.45a |
ANS9 | 3.36cd | 1.69def | 0.88bc | 0.23abc | 36.53cde | 4.59b | 25.91cd |
AUA17 | 6.60ab | 3.1b | 1.09ab | 0.22bc | 125.74bcd | 12.96a | 48.78b |
AUA20 | 5.27bc | 1.97cdef | 0.53cd | 0.17cd | 11.38de | 5.35b | 19.02d |
Fengchisang | 6.79ab | 2.07cde | 1.10ab | 0.16cd | 190.43b | 15.41a | 57.23b |
H2 | 2.80cd | 0.99f | 0.41d | 0.17cd | 12.98de | 4.51b | 11.55d |
H3 | 2.57d | 1.07ef | 0.40d | 0.15cd | 17.07cde | 4.58b | 11.54d |
Means within the same column followed by the same letter do not differ significantly based on Tukey HSD at α= 0.05. Abbreviations: GAE, gallic acid equivalents, CAE, caffeic acid equivalents, CtE, catechin equivalents, C-3-GE,cyanidine 3-glucoside equivalents, TE, trolox equivalents.
The major organic acid in most genotypes was found to be citric acid (Table 4), followed by malic acid. ANS14 exhibited the highest malic acid concentration with the lowest being detected in ANS8. AUA20 presented the highest ascorbic acid concentration and ANS14 the lowest, while citric acid was found at the highest concentration in ANS8. Fumaric acid was detected in very low concentration as ascorbic acid, while the total organic acid concentration was highest in ANS6, with ANS12 exhibiting the lowest one.
Table 4
Organic acid concentration of the fruit of the studied mulberry genotypes
Genotypes | Malic acid | Ascorbic acid | Citric acid | Fumaric acid | Total Organic acids |
(mg 100 g–1 FW) | |||||
ANS10 | 217.8ab | 7.0abc | 301.5bcd | 17.8ab | 544.1bc |
ANS12 | 112.2cd | 4.4bcd | 271.0cd | 7.3cd | 394.9c |
ANS14 | 296.9a | 1.9d | 127.9d | 17.4ab | 444.2bc |
ANS6 | 92.4cd | 6.0abc | 779.6a | 5.5cd | 883.5a |
ANS7 | 91.8cd | 6.0a-d | 325.4bcd | 6.1cd | 429.4bc |
ANS8 | 40.5d | 6.4a-d | 820.3a | 2.8d | 870.0a |
ANS9 | 91.8cd | 8.5ab | 446.6bc | 7.6cd | 554.5bc |
AUA17 | 152.2bc | 3.1cd | 513.5b | 4.8d | 673.5ab |
AUA20 | 64.7cd | 9.9a | 325.2bcd | 7.6cd | 407.4bc |
Fengchisang | 77.5cd | 4.4bcd | 532.8b | 6.0cd | 620.7abc |
H2 | 209.6b | 5.8bcd | 314.7bcd | 11.0bc | 541.1bc |
H3 | 127.2bc | 4.7bcd | 277.2cd | 8.0cd | 417.1bc |
Means within the same column followed by the same letter do not differ significantly based on Tukey HSD at α= 0.05.
The main sugars detected in mulberry fruits were fructose and glucose while only small amounts of sucrose was found (Table 5). ANS10 had the highest concentration of both fructose and glucose as well as the highest sweetness index, while ANS8 exhibited the lowest values.
Table 5
Carbohydrate concentration and sweetness index (SI) of the studied mulberry genotypes
Genotypes | Sucrose | Glucose | Fructose | Total sugars | SI |
(mg g–1 FW) | |||||
ANS10 | 0.32c | 58.54a | 62.49a | 121.36a | 223.09a |
ANS12 | 0.53c | 48.33ab | 53.41abc | 102.27ab | 188.62ab |
ANS14 | 0.68c | 51.99ab | 56.04ab | 108.72ab | 199.76ab |
ANS6 | 0.45c | 34.25cd | 33.58def | 68.28cd | 123.93cd |
ANS7 | 0.45c | 40.75bcd | 41.91cdef | 83.11bcd | 151.86bcd |
ANS8 | 0.67c | 18.27e | 18.79g | 37.74e | 68.56e |
ANS9 | 0.87bc | 35.11cd | 35.55def | 71.53cd | 130.03cd |
AUA17 | 0.47c | 39.21bcd | 43.26bcde | 82.95bcd | 152.92bcd |
AUA20 | 0.73bc | 26.59de | 27.31fg | 54.62de | 99.43de |
Fengchisang | 0.63c | 27.86de | 33.36ef | 61.85de | 144.97de |
H2 | 1.63a | 48.28ab | 49.82abc | 99.73ab | 181.40ab |
H3 | 1.45ab | 44.41bc | 47.30bcd | 93.17bc | 170.21bc |
Means within the same column followed by the same letter do not differ significantly based on Tukey HSD at α= 0.05. Abbreviations: SI, Sweetness index.
From the correlation analysis that took place it was obvious that the antioxidant capacity of genotypes’ fruit is greatly dependent on the concentration of total phenols, total o-diphenols, total flavonoids and total anthocyanins (Table 6) but not on total flavanols concentration.
Table 6
Correlation coefficient of the phenolic compounds assayed and antioxidant capacity of the fruit of the studied mulberry genotypes
Parameters | Phenols | o-diphenols | Flavanols | Flavonoids | Anthocyanins | FRAP | DPPH |
Phenols | 1 | ||||||
o-diphenols | 0.92*** | 1 | |||||
Flavanols | ns | ns | 1 | ||||
Flavonoids | 0.84*** | 0.86*** | ns | 1 | |||
Anthocyanins | 0.85*** | 0.85*** | ns | 0.82*** | 1 | ||
FRAP | 0.84*** | 0.79*** | ns | 0.82*** | 0.91*** | 1 | |
DPPH | 0.89*** | 0.81*** | ns | 0.81*** | 0.84*** | 0.80*** | 1 |
Abbreviations: ***, p < 0.001. ns, not significant.
3.3Principal component, discriminant analysis and possible relationship among genotypes.
The principal component analysis revealed five components with eigenvalue above 1.0 with the first two explaining together the 66.7% of the variability of the original data (Fig. 3). It was clear that based on the physiological, organoleptic and phytochemical characteristics of the fruits, eight distinct groups could be formed, with ANS8 belonging to the far positive side of component 1 axis, characterized by high levels of total flavonoids, o-diphenols, total phenols, anthocyanins, citric acid and titratable acidity and high antioxidant capacity. Fengchisang was grouped also at the positive side of component 1, along with ANS6 and AUA17, exhibiting similar characteristics as ANS8.
Fig.3
Biplot presentation of the scores of the first two components of the principal component analysis of the physiological, organoleptic and phytochemical characteristics of the fruits. Ellipses include points depicting the groups of genotypes with similar characteristics, which are depicted by the vectors. Abbreviations: TSS, total soluble solids, TA, titratable acidity, FA, fumaric acid, DW, dry weight, MA, malic acid, FLANOLS, total flavanols, D, fruit diameter, L, fruit length, W, fruit weight, ASA, ascorbic acid, FLOIDS, total flavonoids, TPh, total phenols, ODs, total o-diphenols, ANTH, total anthocyanins, CA, citric acid, TOA, total organic acids, Fen, Fengchisang.
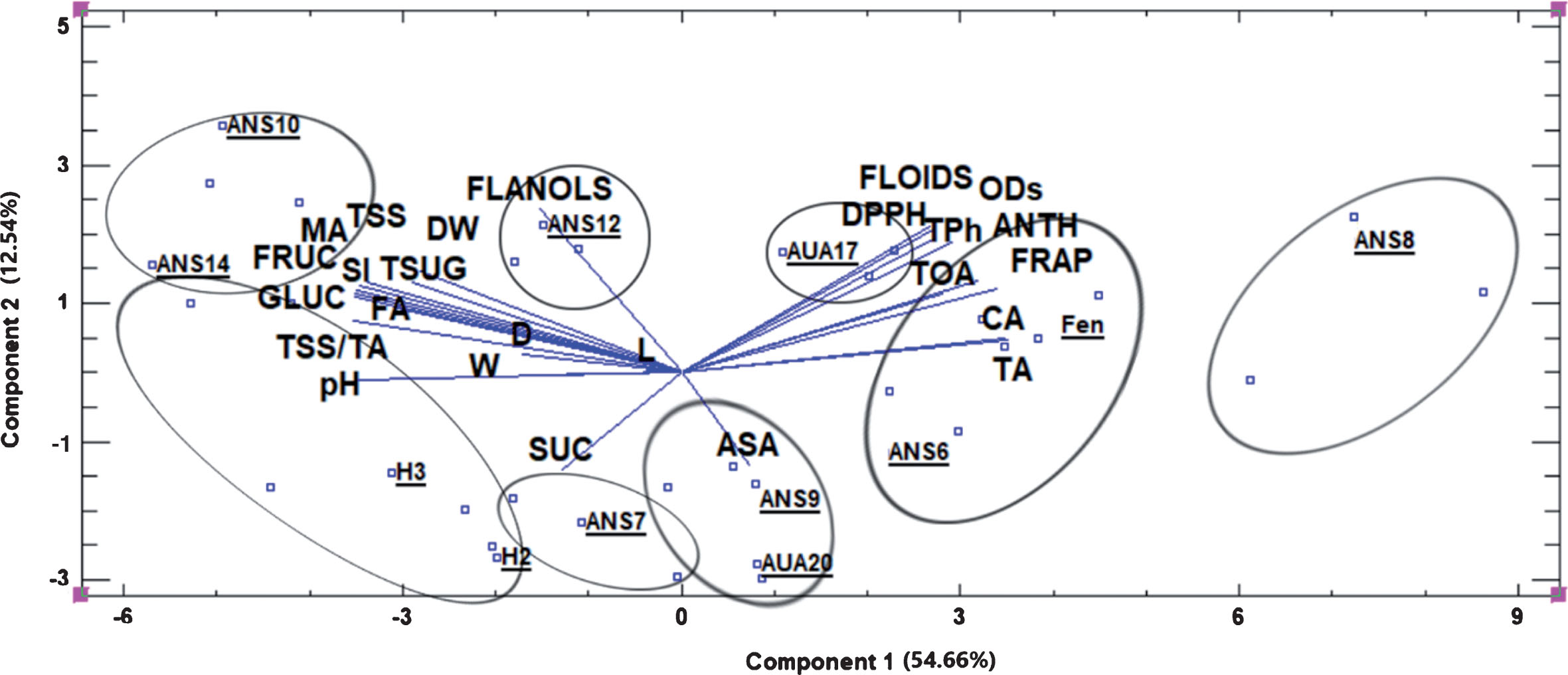
The discriminant analysis resulted in the functions presented in Fig. 4. It was clear that the genotypes can be easily discriminated from each other, with Fenchisang being closer to ANS8, ANS6 and ANS12, while ANS14, AUA17, ANS10 and H2 being far apart from the other genotypes.
Fig.4
Plot of discriminant factors presenting the grouping of the studied genotypes based on data of the physiological, organoleptic and phytochemical characteristics of the fruits. Abbreviations: Fen, Fengchisang.
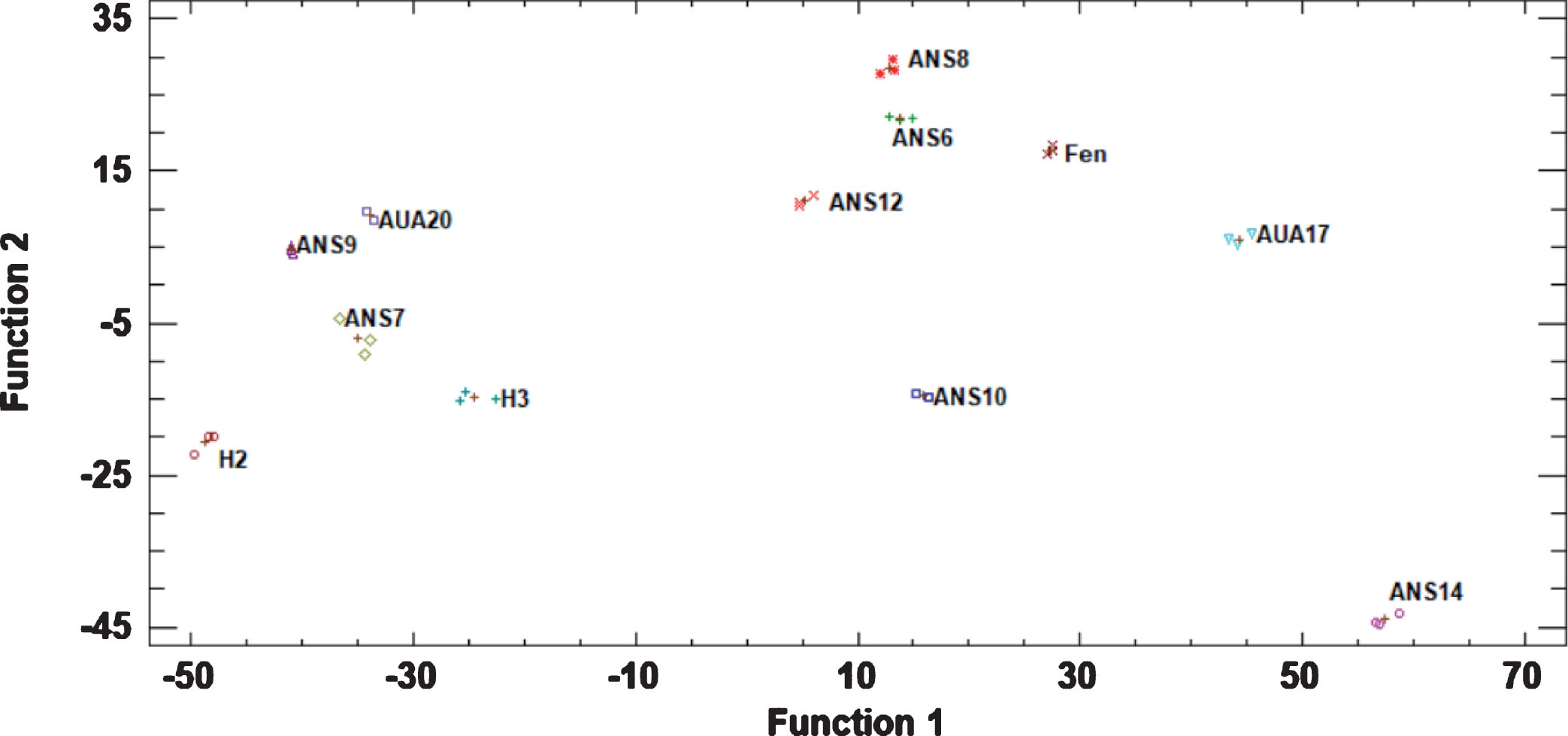
The dendrogram produced by the cluster analysis, using all the parameters assayed regarding fruit quality characteristics, along with leaf phenotypic characteristics and rooting performance of genotype cuttings revealed five clusters (Fig. 5). ANS8 was categorized in a separate cluster from the others, while the genotypes showing a weak relationship were H3 and AUA20. The other groups were constructed based on weaker relationships, according to the distance of the cluster.
Fig.5
Dendrogram of the selected genotypes showing their genetic relationship through Euclidean distance, based on phenotypic characteristics, rooting performance of their cuttings, organoleptic and physiological parameters and phytochemical content of their fruits.
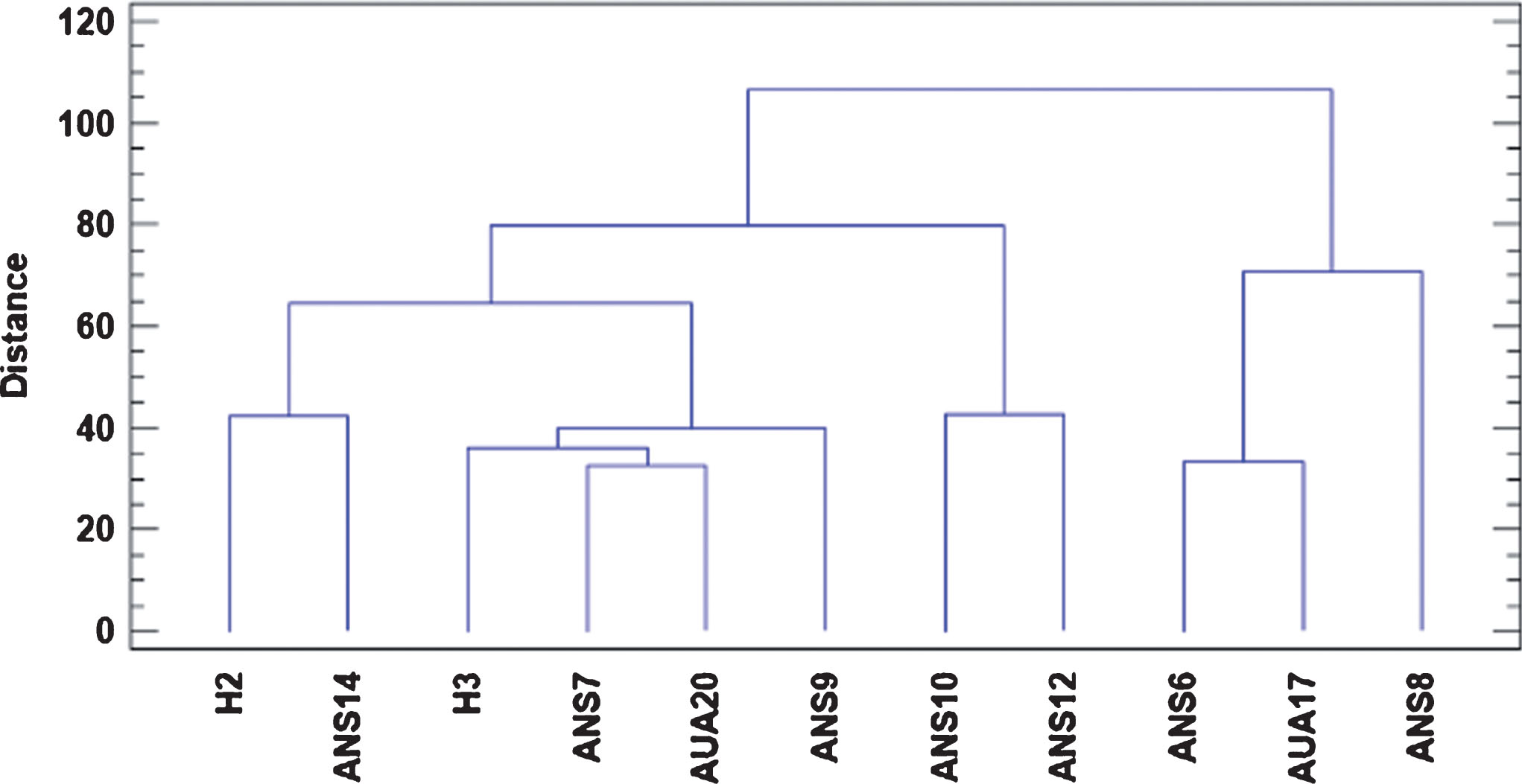
3.4Assessment of cell viability by the MTT assay
During this evaluation stage, experiments with two different berry genotypes were carried out, i.e. Fengchisang, the elite genotype and ANS8 the genotype with the highest phenol concentration and antioxidant capacity. The following Figure (Fig. 6) depicts the mean % viability of HeLa (A, B) and MCF-7 (C, D) cells treated with five lyophilized berry extract concentrations (0.5, 1, 1.5, 2, 2.5 mg/mL) of each genotype for 24 (A, C) and 48 h (B, D), respectively. In all measurements, the notation (0) in the graphs indicates the control solution, which is the nutrient medium with 0.2% ethanol. Figures 6A and B show the mean % viability of the human neuroblastoma HeLa cell line for different concentrations of both genotypes after 24 and 48 h incubation, whereas Figs. 6C and D depict the viability for MCF-7 cells.
Fig.6
Viability of HeLa (A, B) and MCF-7 (C, D) cells after 24 (A, C) and 48 h (B, D) incubation with different concentrations of Fengchisang and ANS8 ethanolic extracts in comparison with the control (% viability) (n = 5). Abbreviations: asterisks indicate significant differences from the control (*p < 0.05, **p < 0.01,***p < 0.001). Hashtags indicate significant differences from the respective treatment at 24 h (#p < 0.05, # #p < 0.01, # #<0.001). Dollar signs indicate significant differences between genotypes for the same extract concentration ($ <0.05, $$ <0.01, $$$ <0.001). Statistically significant differences were determined using the Student’s T-test at probability level p < 0.05.
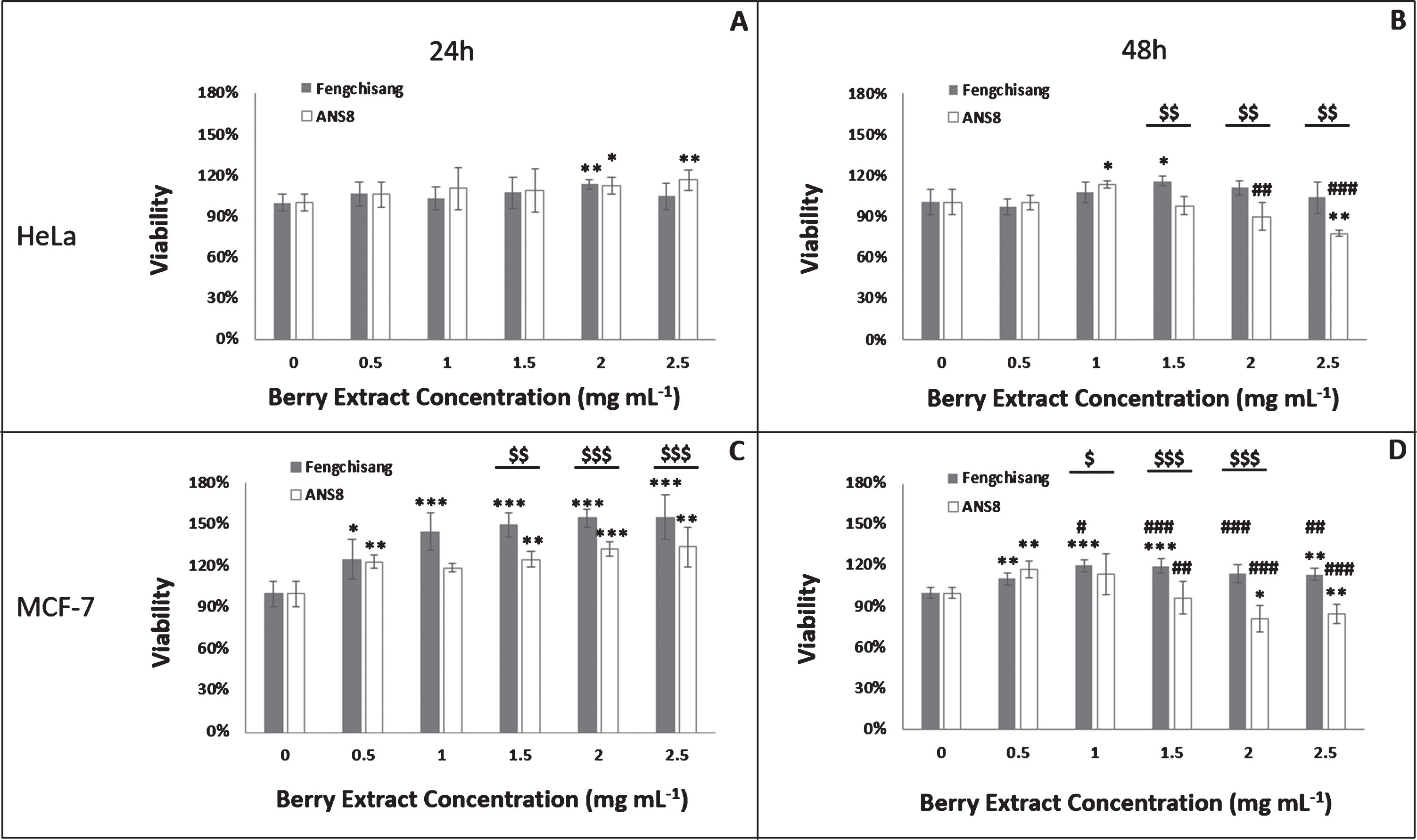
The results showed that berry extracts slightly increased cellular proliferation of HeLa cell line at 24 h with both genotypes (13% for Fengchisang and 16% for ANS8). At 48 h Fengchisang genotype once again elevated cell viability, especially with the concentration 1.5 mg/mL (16%), whereas ANS8 presented significant cytotoxic effects between the concentrations 1.5– 2.5 mg/mL.
Significant increase in cellular proliferation was also observed in MCF-7 cells after treatment with both genotypes at 24 h. Especially Fengchisang significantly induces an MTT uptake proportionally to the concentration (55% at 2.5 mg/mL). This effect downgraded at 48 h with Fengchisang genotype. On the other hand, ANS8 presented significant cytotoxic effects at concentrations between 1.5 – 2.5 mg/mL leading to a 15% reduction in cell viability.
4Discussion
The genotypes assayed exhibited different behaviour during the rooting process of their cuttings. There were genotypes whose cuttings rooted profoundly, both as softwood leafy and as hardwood cuttings (H3, ANS7 and ANS8), while there were also others which were found to be quite (ANS6, ANS10) or extremely (ANS12) recalcitrant to root. Similar results have been reported by other researchers too, regarding mulberry genotypes’ rooting behaviour [11, 18, 19], while the same has been encountered in many other species too [20, 21]. Koyuncu and Senel [19] attributed the low percentages observed mainly to the genetic material employed and secondly to the environmental factors during the rooting period. As the ease of propagation is consindered an important trait of a genotype from the nursery point of view, it becomes evident that the three genotypes, namely, H3, ANS7 and ANS8, are easy to propagate and could become a valuable plant material for the nursery industry.
Nonetheless, in order for a genotype to be successful, it must exhibit, apart from good rooting performance, high adaptation to different pedoclimatic conditions, high yields and great quality characteristics [22]. Significant differences were revealed among genotypes, concerning the organoleptic, physiological and phytochemical characteristics of their fruit. H2 genotype produced the largest fruits among the genotypes assessed, while ANS7 the smallest one, almost half of that of H2. In general, mulberry fruit weight ranges between 2 and 8 g [1– 3]. Some fruits were more round (ANS12 and ANS20) than others (Fengchisang), revealing the phenotypic differences between genotypes. Similarly, dry weight differed a lot among cultivars, as has been also reported by Koyuncu et al. [23] and Ercisli and Orhan [2].
Total soluble solids ranged from 7.33 Brix in ANS8 to 18.35 Brix in ANS10. The total soluble solids were significantly correlated with DW (0.86, p < 0.001), indicating that fruits accumulate great ammounts of soluble solids, which result in increasing their dry weight. The low TSS found in ANS8 and the consequent low ratio of TSS/TA indicate that the specific genotype is probably not a very sweet one, as indicated also by the low sweetness index, having though a relative balance between sweetness and acidity. On the other hand ANS10 exhibited the highest TSS/TA ratio, indicative of a sweet mulberry fruit, according to the sweetness index too. Nonetheless, the higher TSS and probably the higher sweetness of a genotype does not always correlate with the taste, as the latter depends on the relative balance of many factors, such as TSS and TA. Generally, the TSS found in the present genotypes are relatively lower than that stated in the literature [2, 7, 9, 25], probably due to both genotypic differences and pedoclimatic conditions. This is further supported by the similar TSS values reported by Makavelou et al. [12] concerning Fengchisang hybrid, as these trees were grown in vicinity to the trees used in the present experiment. Titratable acidity on the other hand was found to be lower than the values reported in the literature [5, 18, 23], balancing thus the low TSS content. All these traits, TSS, TA and pH have been found to be greatly influenced by environmental factors as well as by genetic material in other species too [22, 26– 28].
All mulberry genotypes assessed in the present experiment were quite rich in phenolic compounds. Phenolic compounds were closely related with antioxidant capacity of the fruits, as has been reported in the literature [5, 7, 22], with ANS8 presenting the highest total phenol content and the highest antioxidant capacity among genotypes. ANS8 exhibited the highest o-diphenols concentration, an important property, since polyphenolic compounds containing an o-dihydroxy moiety in their chemical structure are more vulnerable to oxidation, confering thus greater antioxidant capacity to the fruit [29]. In general, there were significant differences among genotypes, indicative of the role the genetic material plays in phytochemical constituents of the fruit. Similar differences among genotypes concerning their phenolic content have been reported by other researchers too [24, 29]. Genotype and its genetic backrground are the main factors defining the capacity of a fruit to accumulate bioactive constituents and demonstrate antioxidant functions [22].
The genotypes differed also in their organic acid concentration, according to the literature [9, 30]. Interestingly enough, citric acid was the major organic acid detected in most of the genotypes assayed. There are many studies reporting that malic acid is the major organic acid of mulberry fruits [9, 31– 33], but there are also many others reporting that citric acid is the major organic acid found in the fruits [5, 7]. According to Etienne et al. [33] agro-environmental factors affect the acidity of fleshy fruit by acting on various cellular mechanisms, which till now are not fully elucidated. The biochemical processes involved in the metabolic pathway of malic and citric acid in fruit cells are under genetic, environmental and agronomical practices control. Cultural practices such as irrigation, mineral fertilization, thinning and environmental factors such as temperature have a great effect on fruit acidity, but how they affect malic and citric acid accumulation in the cell remains unclear [33]. Thus the predominance of citric acid in the genotypes studied here could be the result of all the pre-mentioned factors, strenthening thus the belief that comparisons of genotypes should be executed under the same pedoclimatic and agronomical conditions [22].
Two were the main sugars in mulberry fruits, i.e. fructose and glucose, according to the literature [29, 34, 35], which were found at similar concentrations, while only traces of sucrose were detected. The concentration of the sugars were in good correlation with that of total soluble solids. It was clear that ANS8 had the least soluble sugars concentration, while ANS10 the highest, justifying its higher sweetness index.
The PCA and discriminant analysis clearly showed that most of the genotypes assayed here were different from the hybrid Fengchisang, with ANS8 differentiating from all the rest, as it presented the best antioxidant values and the highest phenolic compound concentrations. It was quite interesting the fact that PCA vectors distinguished the cultivars based on their high content in either products of primary metabolism (sugars) or of secondary metabolsim (phenolic compounds). The genotypes located at the right side of component 1 presented higher concentration of secondary metabolite’s products, while those at the left side, higher concentration of products of the primary metabolism, indicating probably the genetic differences concerning the preference of genotypes in metabolic investment. The cluster analysis further discriminated ANS8 from the other genotypes.
Berries contain significant amounts of metabolites, including polyphenols, which are reported to inhibit oxidative stress [36], reduce coronary heart disease, cancer risk and other diseases [22]. These effects are mainly attributed to the antioxidant capacity of phenolic compounds found in berries [22, 37, 38]. During the last years, many in vitro studies investigated the prooxidative and oxidative effects of polyphenols against cancer cell proliferation and survival [39– 41]. Various berry extracts have been used to assess their effects on the proliferation of different cancer cell types such as colon, breast, cervical, melanoma [42– 44]. In this work, a cell proliferation assay was performed in order to assess the effects of berry extracts from different genotypes on the viability of cultured breast and cervical cancer cells. Using the MTT uptake viability assay, increased cellular fitness in both cell lines treated with berry extracts for 24 h was observed, leading to a hormetic dose-viability graph (especially significant at MCF-7 cells). The observed increased of MTT uptake, especially by Fengchisang, proportionally to the concentration (55% at 2.5 mg/mL), could possibly be explained by the common phenomenon of hormesis a dose-response relationship characterized by low-dose stimulation and high-dose inhibition, consistent with the Hueppe’s rule, Arndt-Schulz law, and other terms describing a beneficial stimulation at low concentrations [45]. Nonetheless, at 48 h, higher concentrations (1.5– 2.5 mg/mL) tended to produce cytotoxic effects, revealing possible anti-carcinogenic properties of both genotypes, which were most evident in ANS8 ethanolic extractions.
5Conclusions
It can be safely reported here, that ANS8 is a promising black mulberry genotype, as it combines many advantages for both the nursery industry (easy rooting and high rooting percentages with both leafy and hardwood cuttings) and market needs (high antioxidant capacity and phenol content and stronger inhibition of cancer cell viability compared to the elite genotype Fengchisang). It must be noted though, that this specific genotype presented the lowest TSS/TA ratio and sweetness index, characteristics which may rank it low in consumers’ preference [22]. Nonetheless, there are cultivation techniques which may help increase the sugar content and sweetness of the fruit (such as girdling, potassium fertilization etc), while on the other hand, the fruits could be of interest in the pharmacology and the food industry, or in future breeding programms. It is clear that further research is needed, in order to evaluate more horticultural and phytochemical characteristics of this promising genotype and to investigate possible alternative uses of it such as in pharmacology, food industry etc.
Funding
The authors report no funding.
Conflict of interest
The authors have no conflict of interest to report.
Acknowledgments
The authors would like to acknowledge the valuable help in the field of Mr Souchlas Vassilios and Mrs Laskou Dimitra.
References
[1] | Gerasopoulos D , Stavroulakis G . Quality characteristics of four mulberry (Morus sp) cultivars in the area of Chania, Greece. J Sci Food Agric. (1997) ;73: (2):261–4. |
[2] | Ercisli S , Orhan E . Chemical composition of white (Morus alba), red (Morus rubra) and black (Morus nigra) mulberry fruits. Food Chem. (2007) ;103: (4):1380–4. |
[3] | Gecer MK , Akin M , Gundogdu M , Eyduran SP , Ercisli S , Eyduran E . Organic acids, sugars, phenolic compounds, and some horticultural characteristics of black and white mulberry accessions from Eastern Anatolia. Cana J Plant Sci. (2016) ;96: (1):27–33. |
[4] | Kamiloglu S , Serali O , Unal N , Capanoglu E . Antioxidant activity and polyphenol composition of black mulberry (Morus nigra L.) products. J Berry Res. (2013) ;3: (1):41–51. |
[5] | Özgen M , Serçe S , Kaya C . Phytochemical and antioxidant properties of anthocyanin-rich Morus nigra and Morus rubra fruits. Sci Hortic. (2009) ;119: (3):275–9. |
[6] | Ercisli S , Orhan E . Some physico-chemical characteristics of black mulberry (Morus nigra L.) genotypes from Northeast Anatolia region of Turkey. Sci Hortic. (2008) ;116: (1):41–6. |
[7] | Donno D , Cerutti A , Prgomet I , Mellano M , Beccaro G . Foodomics for mulberry fruit (Morus spp.): Analytical fingerprint as antioxidants’ and health properties’ determination tool. Food Res Intern. (2015) ;69: :179–88. |
[8] | Oki T , Kobayashi M , Nakamura T , Okuyama A , Masuda M , Shiratsuchi H , et al. Changes in radical-scavenging activity and components of mulberry fruit during maturation. J Food Sci.. (2006) ;71: (1):C18–C22. |
[9] | Sánchez EM , Calín-Sánchez Á , Carbonell-Barrachina ÁA , Melgarejo P , Hernández F , Martínez-Nicolás JJ . Physicochemical characterisation of eight S panish mulberry clones: processing and fresh market aptitudes. Intern J Food Sci & Techn. (2014) ;49: (2):477–83. |
[10] | Zenginbal H , Eşitken A . Effects of the application of various substances and grafting methods on the grafting success and growth of black mulberry (Morus nigra L. ). Acta Scien Polon-Hort Cultus. (2016) ;15: (4):99–109. |
[11] | Kalyoncu I , Ersoy N , Yılmaz M , Aydın M . Effects of humidity level and IBA dose application on the softwood top cuttings of white mulberry (Morus alba L.) and black mulberry (Morus nigra L.) types. Afr J Biotechn. (2009) ;8: (16):3754–60. |
[12] | Makavelou M , Kafkaletou M , Tsantili E , Harizanis P . Physiological and quality characteristics in four genotypes of mulberry fruit (Morus alba L.) during ripening. Acta Hortic. (2011) ;981: :625–30. |
[13] | Roussos P , Denaxa NK , Damvakaris T . Strawberry fruit quality attributes after application of plant growth stimulating compounds. Sci Hortic. (2009) ;119: (2):138–46. |
[14] | Meyers KJ , Watkins CB , Pritts MP , Liu RH . Antioxidant and antiproliferative activities of strawberries. J Agric Food Chem. (2003) ;51: (23):6887–92. |
[15] | Arnous A , Makris DP , Kefalas P . Correlation of pigment and flavanol content with antioxidant properties in selected aged regional wines from Greece. J Food Compos Anal. (2002) ;15: (6):655–65. |
[16] | da Silva Pinto M , Lajolo FM , Genovese MI . Bioactive compounds and quantification of total ellagic acid in strawberries (Fragaria x ananassa Duch.). Food Chem. (2008) ;107: (4):1629–35. |
[17] | Mosmann TJ . Rapid colorimetric assay for cellular growth and survival: application to proliferation and cytotoxicity assays. J Immunol Methods. (1983) ;65: (1-2):55–63. |
[18] | Singh K , Jat DK , Mehta S . Rootability of hardwood cuttings of mulberry (Morus alba L.) influenced by planting time and growing conditions under valley condition of Garhwal Himalayas. Plant Arch. (2015) ;15: (2):1031–6. |
[19] | Koyuncu F , Senel E . Rooting of black mulberry (Morus nigra L.) hardwood cuttings. J Fruit Ornam Plant Res. (2003) ;11: (1/4):53–8. |
[20] | Denaxa N-K , Vemmos SN , Roussos PA . The role of endogenous carbohydrates and seasonal variation in rooting ability of cuttings of an easy and a hard to root olive cultivars (Olea europaea L.). Sci Horticulturae. (2012) ;143: :19–28. |
[21] | Oliveira JAA , Bruckner CH , Silva DFPd , Santos CEMd , Penso GA , Aquino CF . Estimation of genetic parameters and selection for rooting capacity in peach. Crop Breed App Biot. (2018) ;18: (3):320–4. |
[22] | Di Vittori L , Mazzoni L , Battino M , Mezzetti B . Pre-harvest factors influencing the quality of berries. Sci Hortic. (2018) ;233: :310–22. |
[23] | Koyuncu F , Çetinbas M , Erdal İ . I. Nutritional constituents of wild-grown black mulberry (Morus nigra L.). J Appl Bot Food Qual. (2014) ;87: :93–6. |
[24] | Okatan V . Phenolic compounds and phytochemicals in fruits of black mulberry (Morus nigra L. ) genotypes from the Aegean region in Turkey. Folia Hortic. (2018) ;30: (1):93–101. |
[25] | Sans S , Casals J , Simó J . Improving the Commercial Value of the ‘CalÇot’(Allium cepa L. ) Landrace: Influence of Genetic and Environmental Factors in Chemical Composition and Sensory Attributes. Front Plant Sci. (2018) ;9: :1–12. |
[26] | Kyriacou MC , Leskovar DI , Colla G , Rouphael Y . Watermelon and melon fruit quality: The genotypic and agro-environmental factors implicated. Sci Hortic. (2018) ;234: :393–408. |
[27] | Tripodi P , Cardi T , Bianchi G , Migliori CA , Schiavi M , Rotino GL , et al. Genetic and environmental factors underlying variation in yield performance and bioactive compound content of hot pepper varieties (Capsicum annuum) cultivated in two contrasting Italian locations. Eur Food Res Techn. (2018) )1–13. |
[28] | Tsimogiannis DI , Oreopoulou V . The contribution of flavonoid C-ring on the DPPH free radical scavenging efficiency. A kinetic approach for the 3′, 4′-hydroxy substituted members. Inn Food Sci & Emerg Techn. (2006) ;7: (1-2):140–6. |
[29] | Sánchez-Salcedo EM , Mena P , García-Viguera C , Martínez JJ , Hernández F . Phytochemical evaluation of white (Morus alba L.) and black (Morus nigra L.) mulberry fruits, a starting point for the assessment of their beneficial properties. J Funct Foods. (2015) ;12: :399–408. |
[30] | Ercisli S , Akin M , Eyduran S . Organic acids, sugars, vitamin C, antioxidant capacity, and phenolic compounds in fruits of white (Morus alba L.) and black (Morus nigra L.) mulberry genotypes. J App Bot Food Qual. (2015) ;88: :134–8. |
[31] | Akin M , Eyduran SP , Ercisli S , Yilmaz I , Cakir O . Phytochemical profiles of wild grown blackberry and mulberry in Turkey. Acta Sci. Pol. Hort Cultus. (2016) ;15: :3–12. |
[32] | Koyuncu F . Organic acid composition of native black mulberry fruit. Chem Nat Comp. (2004) ;40: (4):367–9. |
[33] | Etienne A , Génard M , Lobit P , Mbeguiée-A-Mbeguiée D , Bugaud C . What controls fleshy fruit acidity? A review of malate and citrate accumulation in fruit cells. J Exp Bot. (2013) ;64: (6):1451–69. |
[34] | Eyduran SP , Ercişli S , Akın M , Beyhan Ö , GeÇer MK , Eyduran E , et al. Organic acids, sugars, vitamin C, antioxidant capacity, and phenolic compounds in fruits of white (Morus alba L.) and black (Morus nigra L.) mulberry genotypes. J App Bot Food Qual. (2015) ;88: :134–8. |
[35] | Gundogdu M , Muradoglu F , Sensoy RG , Yilmaz H . Determination of fruit chemical properties of Morus nigra L., Morus alba L. and Morus rubra L. by HPLC. Sci Hortic. (2011) ;132: :37–41. |
[36] | Forbes-Hernández TY , Afrin S , Cianciosi D , Manna PP , Zhang J , Gasparrini M , Reboredo-Rodriguez P . Strawberry extract attenuates oxidative stress in 3T3-L1 cells. J Berry Res. (2018) ;8: :193–203. |
[37] | Amatori S , Mazzoni L , Alvarez-Suarez JM , Giampieri F , Gasparrini M , Forbes-Hernandez TY , et al. Polyphenol-rich strawberry extract (PRSE) shows in vitro and in vivo biological activity against invasive breast cancer cells. Sci Rep. (2016) ;6: :30917. |
[38] | Li X , Liu H , Lv L , Yan H , Yuan YJ . Antioxidant activity of blueberry anthocyanin extracts and their protective effects against acrylamide-induced toxicity in HepG2 cells. Int J Food Sci Tech. (2018) ;53: (1):147–55. |
[39] | Jin S , Zhang Q , Kang X , Wang J , Zhao WJ . Daidzein induces MCF-7 breast cancer cell apoptosis via the mitochondrial pathway. Ann Oncol. (2009) ;21: (2):263–8. |
[40] | Kim KK , Singh AP , Singh RK , DeMartino A , Brard L , Vorsa N , et al. Anti-angiogenic activity of cranberry proanthocyanidins and cytotoxic properties in ovarian cancer cells. Int J Oncol. (2012) ;40: (1):227–35. |
[41] | Yang X , Belosay A , Hartman JA , Song H , Zhang Y , Wang W , et al. Dietary soy isoflavones increase metastasis to lungs in an experimental model of breast cancer with bone micro-tumors. Clin Exp Metast. (2015) ;32: (4):323–33. |
[42] | Brown EM , McDougall GJ , Stewart D , Pereira-Caro G , González-Barrio R , et al. Persistence of anticancer activity in berry extracts after simulated gastrointestinal digestion and colonic fermentation. PLOS ONEe. (2012) ;7: (11):49740. |
[43] | Olsson ME , Gustavsson K-E , Andersson S , Nilsson Å , Duan R-D . Inhibition of cancer cell proliferation in vitro by fruit and berry extracts and correlations with antioxidant levels. J Agric Food Chem. (2004) ;52: (24):7264–71. |
[44] | Wang E , Liu Y , Xu C , Liu J . Antiproliferative and proapoptotic activities of anthocyanin and anthocyanidin extracts from blueberry fruits on B16-F10 melanoma cells. Food Nutr Res. (2017) ;61: (1):1325308. |
[45] | Calabrese EJ . Hormesis: from mainstream to therapy. J Cell Commun. Signal. (2014) ;8: (4):289–91. |