Lingonberries (Vaccinium vitis-idaea L) and blueberries (Vaccinium corymbosum) contain discrete epicatechin anthocyanin derivatives linked by ethyl bridges
Abstract
BACKGROUND:
Lingonberries have been associated with a range of potential bioactivities related to their polyphenol content and composition, in particular anthocyanin and proanthocyanidin derivatives.
OBJECTIVES:
The objective of this study was to examine the anthocyanin composition of extracts of lingonberry and related Vaccinium berries with respect to previously unidentified anthocyanin-like components.
METHODS:
Extracts were prepared from lingonberries, blueberries and a commercial cranberry juice product and examined by liquid chromatography mass spectroscopic techniques (LCMSn) before and after various fractionation steps.
RESULTS:
A range of discrete anthocyanin derivatives ethyl linked to (epi)catechin units were identified in lingonberry extracts using LC-MSn methods. Analogous derivatives were detected in similar blueberry extracts, and confirmed in cranberry juices.
CONCLUSIONS:
Through a combination of fractionation techniques and LCMSn analysis, this paper provides the first evidence that anthocyanin-epicatechin derivatives linked by ethyl bridges are present in lingonberries and in blueberries. These discrete components may be the smallest in a series of proanthocyanidin derivatives with terminal anthocyanins linked through ethyl groups which have previously been inferred in cranberries using mass spectrometric techniques.
1Introduction
Lingonberry (Vaccinium vitis-idaea L.) is a popular edible berry in Scandinavian countries, and is now gaining in popularity across Europe. Lingonberries have a complex phenolic profile consisting of a mixture of anthocyanins, proanthocyanidins (PACs), flavonols, hydroxycinnamic acids and phenolic acids [1, 2]. They have a high antioxidant capacity [3] and a lingonberry extract has been found to reduce in vivo oxidative stress by 25% in a diet-induced rat model [2]. The reduction of oxidative stress may offer a plausible explanation for the potential anti-cancer effects of polyphenols from many berry sources [4–6]. Lingonberry extracts exhibit anti-proliferative activity both in vitro [7–9] and in animal models [10]. Lingonberries have also been associated with health benefits relevant to metabolic syndrome [11], vascular health [12], glycemic responses [13] and lipid digestion [14]. Lingonberries have anti-bacterial effects [15] and, similar to the related cranberry (Vaccinium oxycarpon; [16]), they have been suggested to be effective against recurring urinary tract infections in women [17]. Although a Cochrane review [18] raised doubts about the efficacy of cranberry, it has been suggested that extracts of cranberry and lingonberry standardised with respect to their polyphenol content and composition would aid in establishing such a claim [19]. Lingonberries and cranberries contain both B-type and A-type PACs and these components have been specifically associated with anti-bacterial [20] and anti-cancer [9] effectiveness. In previous work, lingonberry extracts were subjected to in vitro digestion techniques that simulated the effects of the upper gastrointestinal tract to obtain extracts whose composition should mimic those components likely to survive digestion and enter the colon [21]. These “colon-available” extracts were then assessed for their potential protective effects using in vitro models of colon cancer. The stability of the polyphenols after digestion was monitored using liquid chromatographic mass spectrometric (LC-MSn) methods to assess their likely contribution to anti-cancer effects. As a result, several potentially novel anthocyanin-like components were noted. This paper reports a combination of fractionation techniques and LC-MSn analysis which show for the first time that anthocyanin derivatives ethyl linked to (epi)catechin units are present both in lingonberries but also in blueberries.
2Experimental
2.1Berry and juice extraction
This study used lingonberries (Vaccinium vitis-idaea) obtained via Polarica AB (Haparanda, Sweden) which were wild-harvested in 2009 and 2011 and highbush blueberry (Vaccinium corymbosum) varieties, Berkley and Bluecrop, grown at the James Hutton Institute in 2011. Polyphenol-rich extracts were prepared using the method described previously [14]. Briefly, frozen berries (250 g per batch) were homogenised in an equal volume of acetonitrile containing 0.2% formic acid using a Waring Blender (3×15 sec, full power, repeated twice). The resultant puree was filtered through muslin cloth then glass sinters to obtain the extract. A portion of the extract was evaporated on a rotary evaporator to remove the acetonitrile and a polyphenol-rich fraction obtained by solid phase extraction [14]. Samples were aliquoted and dried in a Speed Vac (Thermo Scientific Ltd, Hemel Hempstead, UK).
Cranberry juice (Ocean Spray, cranberry classic light; 25% juice) was purchased locally. The sample was diluted with an equal volume of 0.2% formic acid and a polyphenol-rich fraction obtained by solid phase extraction [14]. Samples were aliquoted and dried in a Speed-Vac. Phenol content was measured using a modified Folin–Ciocalteu method (22) and quantified as gallic acid equivalents (GAE).
2.2Fractionation
A portion of the lingonberry extract was partitioned with ethyl acetate. Equal volumes of the extract (100 mL) and ethyl acetate were mixed in a separating funnel. The water fraction and the ethyl acetate fractions were separated then re-extracted twice more to ensure complete fractionation. The ethyl acetate and water fractions were aliquoted and dried to a constant phenol content using a Speed-Vac.
A portion (50 mL) of the lingonberry extract was made up to 50% (v/v) ethanol then fractionated on a 20 cm3 column of Sephadex LH-20, using the method in the Tannins Handbook (at www.users.muohio.edu/hagermae/tannin.pdf) and previously outlined (9). The run-through material plus a column volume of 50% ethanol was collected as the unbound fraction, which was enriched in anthocyanins. The column was washed with three column volumes of 50% ethanol. The bound fraction, which was enriched in proanthocyanidins (PACs), was eluted with three volumes of 80% acetone.
Cranberry juice and lingonberry extracts were fractionated using Discovery DPA-6S polyamide SPE cartridges (Supelco, Sigma-Aldrich, Poole, UK) [23]. The cartridges were pre-conditioned with 100% methanol then UPW. The sample (10 mL of juice or lingonberry extract re-dissolved in UPW) was applied and the unbound sample collected with a further wash of 10 ml UPW. This unbound fraction of the juice sample contained mainly sugars. An anthocyanin-rich fraction was eluted using 10 ml of 20% methanol. PACs were eluted using 10 mL of 80% acetone. Each fraction was aliquoted and evaporated as previously described.
2.3Liquid Chromatography-Mass Spectrometry (LC-MS)
Samples were analysed on an LCQ-Deca system, comprising Surveyor autosampler, pump and photodiode array detector (PDAD) and an ion-trap mass spectrometer (Thermo Fisher Scientific, Hemel Hempstead, UK). The PDAD scanned discrete channels at 280 nm, 365 nm and 520 nm. The samples were applied to a C18 column (Synergi Hydro C18 with polar endcapping, 2 mm×150 mm, Phenomenex Ltd.) and eluted using a gradient of 5% acetonitrile containing 0.1% formic acid to 40% acetonitrile containing 0.1% formic acid over 30 min at a rate of 200 μl/min. The LCQ-Deca LC–MS was fitted with an ESI (electrospray ionisation) interface and analysed the samples in positive and negative ion mode. There were two scan events; full scan analysis followed by data-dependent MS/MS of the most intense ions using collision energies (source voltage) of 45% . The capillary temp was set at 250°C, with sheath gas at 60 psi and auxiliary gas at 15 psi.
Certain samples were also analysed using an Accella 600 pump with a PDA detector coupled to an LTQ Orbitrap mass spectrometer (Thermo Fisher Scientific). Replicate samples were injected onto a 2×150 mm (4 μm) Synergy Hydro-RP 80 (Phenomenex Ltd, Macclesfield, UK). Sample and column temperature were maintained at 6°C and 30°C respectively. Samples were analysed at a flow rate of 0.3 ml/min using a binary mobile phase of (A) 0.1% aqueous formic acid and (B) 0.1% formic acid in acetonitrile/water (1:1, v/v) and the following gradient: 0–4 min 5% B; 4–22 min 5–50% B; 22–32 min, 50–100% B. Mass detection was carried out using an LTQ Orbitrap mass spectrometer in positive ESI mode. Two scan events were employed; full scan analysis was followed by data-dependent MS/MS of the three most intense ions using collision energies of 45 electron volts source voltage (set at 3.4 kV) in wide-band activation mode. The instrument was optimized (tuned) against cyanidin glucoside at a resolution of 30000 and a mass range of 80–2000 mass units. For optimal electrospray ionization, the source conditions were set at source temperature 300°C, sheath gas at 60 arbitrary units and auxillary gas at 5 arbitrary units. Prior to analysis, the mass accuracy of the instrument was assured by calibration following the manufacturer’s protocols.
3Results and discussion
During preparation and analysis of phenolic-enriched extracts from lingonberries (Vaccinium vitis-idaea L) for studies of their potential bioactivities with respect to colon cancer [21]), it was noted that the LC-MS-PDAD profiles contained discrete peaks that absorbed at ∼500 nm but eluted later than the main anthocyanin peaks (Fig. 1a, panel A). The initial assumption was that they were anthocyanin-like derivatives. To examine their composition further, the original extract was partitioned between ethyl acetate and water and these fractions analysed by LC-MS at equivalent phenol contents (i.e. 20 μg GAE injection; Fig. 1a, panel B & Fig. 1b, panel B). The anthocyanins were concentrated in the water fraction over the ethyl acetate fraction (compare (Fig. 1a, panel B & C). The main anthocyanin peaks, LA1, LA2 and LA3, were putatively identified as cyanidin galactoside, cyanidin glucoside and cyanidin arabinoside by comparison with previous reports (Table 1; [1, 9, 24]). The water fraction was also enriched in other anthocyanin-like peaks, LA4 to LA8. Peak LA4 was putatively identified as a cyanidin pentose, possibly a xyloside, and LA6 as a cyanidin acetyl hexose derivative by analogy to previous work [neutral loss of 204; = acetyl hexose, 25]. Peaks LA5, LA7 and LA8 yieldedλmax values shifted to higher wavelengths (∼530 nm) than the major cyanidin anthocyanins (∼515 nm). Peaks LA5 and LA7 gave a major m/z [M+H]+ at 765 and yielded MS2 signals consistent with losses of 162 (hexose), of 290 (epicatechin/catechin) and of 452 (hexose + (epi)catechin) to a possible base fragment at m/z [M+H]+ 313. In addition, peak LA8 gave a major m/z [M+H]+ signal = 735 and MS2 yielded neutral losses of 132 (pentose), 290 ((epi)catechin) and 422 (pentose + (epi)catechin) to a base fragment at M+H = 313. Peak LA9 was not obvious in the original extract but gave m/z [M+H]+ = 751 and yielded major MS2 fragments at 589 and 299, which can be construed as loss of hexose (162) and (epi)catechin + hexose (452) to a base fragment with m/z [M+H]+ 299.
Components with similar UV spectra and MS properties to LA5, LA7 (both M+H = 765) and LA8 (M+H = 735) have been noted in cranberry (Vaccinium macrocarpon Ait.) extracts [26], and were suggested to be derivatives composed of epicatechin ethyl bridged to cyanidin anthocyanins. In negative mode, LA7 gave a major m/z [M-H]– at 763 which yielded MS2 fragments at 611 (loss of 152), 601 (loss of 162 = hexose), 473 (loss of 290, (epi)catechin), 447 (loss of 316, (epi)catechin +26), and 311 (loss of (epi)catechin and hexose) and 285 (loss of 478; loss of (epi)catechin, hexose + 26) (Fig. 2). These losses match the MS2 data provided for similar compounds in cranberry [26]. The loss of 152 is characteristic of retro-Diels Alder (RDA) fragmentation of the epicatechin group and the major fragments at 463 and 447 can be assigned to vinyl cyanidin hexose and cyandin hexose respectively (see Diagram 1). LC-MSn analysis on an Orbitrap MS system also identified two main peaks with m/z [M+H]+ 765 both with similar MS2 profiles. The exact mass of the component at m/z [M+H]+ 765.2014 gave a predicted structural formula of C38H37O17 with a Δ ppm <1. In addition, the m/z [M+H]+ 735 signal gave an exact mass of 735.1911 which gave a predicted structural formula of C37H35O16 with a Δ ppm <1. For the m/z [M+H]+ 765 component, the predicted structural formula (C38H37O17) can be reconstructed from cyandin hexose (C21H21O11) plus (epi)catechin (C15H14O6) with a remainder of C2H2. The 26 amu (C2H2) difference between the fragment at M+H 313 and cyanidin (M+H = 287) would be explained by the production of a vinyl group (-CH = CH2) from an ethyl bridge after fragmentation between the (epi)catechin and cyanidin groups (see Diagram 1). The MS2 properties of Peak LA9, m/z [M+H]+ 751 with neutral losses of 162 (hexose), 290 ((epi)catechin) and 452 (hexose + (epi)catechin), fits with an ethyl linked epicatechin anthocyanin derivative but the nature of the “anthocyanidin” (m/z [M+H]+ 299) is not known. Apart from this last component, it is notable that the putative ethyl linked (epi)catechin derivatives have the same underlying structure as the main lingonberry anthocyanins, i.e. cyanidin hexoses and pentoses.
Analogous ethyl-linked epicatechin anthocyanin derivatives were confirmed in a commercial cranberry juice using similar LCMSn techniques (results not shown). Like lingonberry, the structures of these components mirrored the major anthocyanins, in this case being ethyl linked epicatechin derivatives of cyanidin and peonidin hexoses and pentoses. Previous work [27, 28] highlighted the possibility that cranberries (Vaccinium macrocarpon Ait.) contained a range of proanthocyanidin-like components with terminal cyanidin groups which were linked through an ethyl group to the flavan-3-ol units (see Diagram 1). The lingonberry and cranberry components noted in this study using LCMSn may represent the simplest form of this class of compounds. The putative ethyl-linked (epi)catechin derivatives in both lingonberry and cranberry had λmax values shifted upwards from their parent molecules (from 515 nm to ∼530 nm) and pronounced shoulders at ∼450 nm which was also noted for synthesized ethyl-catechin cyanidin galactosides [29].
The putative anthocyanin-ethyl linked (epi)catechin components with m/z [M+H]+ = 765 from lingonberry did not bind to Sephadex LH20 and was enriched in the anthocyanin-rich unbound fraction (Fig. 3) under conditions were proanthocyanidins (PACs) bound and were removed [9]. In addition, this putative anthocyanin-ethyl linked (epi)catechin component remained soluble when gelatin was added to remove the PACs by fining ([30]; results not shown).
As previously reported [23], fractionation on polyamide SPE units led to an enrichment of the major anthocyanins in the methanol fraction and allowed the selective fractionation of PACs in the acetone fraction. The ethyl linked (epi)catechin anthocyanin derivatives from cranberry juice and lingonberry extracts were essentially equally fractionated between the methanol and acetone fractions, which may reflect the “composite” nature of these derivatives. However, these derivatives gave clearer MS spectra in the methanol fraction probably due to the removal of co-eluting PACs. In all cases, the ethyl (epi)catechin anthocyanin derivatives existed in at least two separate peaks, which might represent isomers arising from different points of attachment of the ethyl-EC units to the anthocyanin terminal unit.
Putative ethyl-linked (epi)catechin anthocyanin derivatives were also detected for the first time in polyphenol-enriched extracts prepared from fruit of highbush blueberry cultivars. As was seen in the original lingonberry extracts (Fig. 1b, panel A), late eluting anthocyanin-like peaks could be discerned (Fig. 4, panel A) in polyphenol-enriched extracts of variety Bluecrop without further fractionation. The two anthocyanin-like peaks (BbA1 and BbA2; Fig. 4. panels A & B) gave MS data consistent with isomers of malvidin ethyl (epi)catechin acetyl hexose [m/z [M+H]+ = 851 with an in-source fragment of 357 (Fig. 4, panel C); MS2 = 699, (loss of 152 through RDA fragmentation of (epi)catechin), 647 (loss of 204; acetyl hexose), 561 (loss of 290, (epi)catechin) and 357 (loss of 494, acetyl hexose and (epi)catechin; Fig. 4, panel D)]. However in polyphenol-enriched extracts of variety Berkley, the only detectable derivative gave MS data consistent with a malvidin ethyl (epi)catechin hexose derivative [m/z [M+H]+ = 809, MS2 = 647 (loss of hexose), 519 (loss of (epi)catechin) and 357 (loss of hexose and (epi)catechin); results not shown]. Other ethyl linked (epi)catechin anthocyanin derivatives may be present in these blueberry extracts but these represent the major and therefore most readily detectable components. Further fractionation work is required to define the scope of these components. The difference between the varieties is probably related to their overall anthocyanin composition as Berkley has few acetylated anthocyanins whereas they make up a considerable proportion of the total in Bluecrop. Substantial variation in acetylated anthocyanins has been previously noted in blueberry genotypes [31].
The mechanism of formation of these components is unknown. The close match between the structures of the discrete ethyl linked (epi)catechin derivatives noted in this paper with the major anthocyanins of the different berries suggests that they are formed from the major anthocyanins. The low levels of these components (the largest A7 in lingonberries was ∼2% of the total anthocyanin content by peak area) also supports their formation as a side reaction of the major anthocyanins. Ethyl linked condensation products of anthocyanins and flavan-3-ols have been noted in stored and aged red wines [32, 33] where the ethyl link is formed from acetaldehyde produced during fermentation. Such components identified in rose cider have been synthesised in model systems reacting acetaldehyde, cyanidin galactoside and epicatechin [29]. Indeed, previous MALDI-TOF studies suggested that juicing and storage altered the content and composition of ethyl linked epicatechin anthocyanin derivatives in cranberry products [27]. However, acetaldehyde can be formed during berry ripening [28] and these derivatives could be formed during this phase. A study of berries through ripening may confirm if these components are produced in vivo or as a result of post-harvest processing.
In conclusion, discrete ethyl-linked epicatechin anthocyanin components were detected for the first time in lingonberries and blueberries and confirmed in cranberry juices. They may represent the simplest of a class of “hybrid” anthocyanin-proanthocyanidin components up to hexamer in extension already inferred in cranberry [26]. Although present in fruit of these members of the Vaccinium family, it is not currently known if these components are present in berries from other Vaccinium species or indeed in other families. Although they are minor components compared to anthocyanins, they could be ingested and their metabolic fate in humans and potential bioactivities are unknown. Initial studies showed that these components were as stable to simulated gastrointestinal digestion as the main lingonberry anthocyanins [23; results not shown] and may be available in the colon where they could contribute to effects on human health.
Acknowledgments
The authors acknowledge Lara Hilley, Mohammed Al-Khairulla and Sandra Bacon who produced extracts that were subsequently analysed to identify some of the compounds mentioned in this paper.
References
[1] | Ek S , Kartimo H , Mattila S , Tolonen A . Characterization of phenolic compounds from lingonberry (Vaccinium vitis-idaea). J Agric Food Chem. (2006) ;54: :9834–42. |
[2] | Mane C , Loonis M , Juhel C , Dufour C , Malien-Aubert C . Food grade lingonberry extract: Polyphenolic composition and in vivo protective effect against oxidative stress. J Agric Food Chem. (2011) ;59: :3330–9. |
[3] | Zheng W , Wang SY . Oxygen radical absorbing capacity of phenolics in blueberries, cranberries, chokeberries, and lingonberries. J Agric Food Chem. (2003) ;51: :502–9. |
[4] | Seeram NP . Berry fruits for cancer prevention: Current status and future prospects. J Agric Food Chem. (2008) ;56: :630–5. |
[5] | Brown EM , McDougall GJ , Stewart D , Pereira-Caro G , Gonzalez-Barrio R , Allsopp P , Magee P , Crozier A , Rowland I , Gill CIR . Persistence of anticancer activity in berry extracts after simulated gastrointestinal digestion and colonic fermentation. PLoS One. (2012) ;7: :1–10. |
[6] | Brown EM , Latimer C , Allsopp P , Ternan NG , McMullan G , McDougall GJ , Stewart D , Crozier A , Rowland I , Gill CIR . In vitro and in vivo models of colorectal cancer: Antigenotoxic activity of berries. J Agric Food Chem. (2014) ;62: :3852–66. |
[7] | Olsson ME , Gustavsson KE , Andersson S , Nilsson A , Duan RD . Inhibition of cancer cell proliferation in vitro by fruit and berry extracts and correlations with antioxidant levels. J Agric Food Chem. (2004) ;52: :7264–71. |
[8] | Wu QK , Koponen JM , Mykkänen HM , Törrönen AR . Berry phenolic extracts modulate the expression of p21(WAF1) and Bax but not Bcl-2 in HT-29 colon cancer cells. J Agric Food Chem. (2007) ;55: :1156–63. |
[9] | McDougall GJ , Ross HA , Ikeji M , Stewart D . Berry extracts exert different antiproliferative effects against cervical and colon cancer cells grown in vitro. J Agric Food Chem. (2008) ;56: :3016–23. |
[10] | Misikangas M , Pajari AM , Päivärinta E , Oikarinen SI , Rajakangas J , Marttinen M , Tanayama H , Törrönen R , Mutanen M . Three Nordic berries inhibit intestinal tumorigenesis in multiple intestinal neoplasia/+ mice by modulating beta-catenin signaling in the tumor and transcription in the mucosa. J Nutr. (2007) ;137: :2285–90. |
[11] | Heyman L , Axling U , Blanco N , Sterner O , Holm C , Berger K . Evaluation of beneficial metabolic effects of berries in high-fat fed C57BL/6J mice. J Nutrition & Metab. (2014) ;17. article 403041. |
[12] | Kivimaki AS , Siltari A , Ehlers P , Korpela R , Vapaatalo H . Lingonberry juice lowers blood pressure of spontaneously hypertensive rats (SHR). J Functional Foods. (2013) ;5: :1432–40. |
[13] | Torronen R , Kolehmainen M , Sarkkinen E , Mykkanen H , Niskanen L . Postprandial glucose, insulin, and free fatty acid responses to sucrose consumed with blackcurrants and lingonberries in healthy women. Amer J Clin Nutr. (2012) ;96: :527–33. |
[14] | McDougall GJ , Kulkarni NN , Stewart D . Berry polyphenols inhibit pancreatic lipase activity in vitro . Food Chem. (2009) ;115: :193–9. |
[15] | Toivanen M , Huttunen S , Lapinjoki S , Tikkanen-Kaukanen C . Inhibition of adhesion of Neisseria meningitidis to human epithelial cells by berry juice polyphenolic fractions. Phytother Res. (2011) ;25: :828–32. |
[16] | Howell AB . Cranberry proanthocyanidins and the maintenance of urinary tract health. Crit Rev Food Sci Nutr. (2002) ;42: :273–8. |
[17] | Kontiokari T , Sundqvist K , Nuutinen M , Pokka T , Koskela M , Uhari M . Randomised trial of cranberry-lingonberry juice and Lactobacillus GG drink for the prevention of urinary tract infections in women. Brit Med J. (2001) ;322: :1571–3. |
[18] | Jepson RG , Williams G , Craig JC . Cranberries for preventing urinary tract infections. Cochrane Database Syst Rev. (2012) ;17: :10:CD001321. doi: 10.1002/14651858.CD001321.pub5 |
[19] | Davidson E , Zimmermann BF , Jungfer E , Chrubasik-Hausmann S . Prevention of urinary tract infections with Vaccinium products. Phytother Res. (2014) ;28: :465–70. |
[20] | Howell AB , Reed JD , Krueger CG , Winterbottom R , Cunningham DG , Leahy M . A-type cranberry proanthocyanidins and uropathogenic bacterial anti-adhesion activity. Phytochemistry. (2005) ;66: :2281–91. |
[21] | Brown EM , Nitecki S , Pereira-Caro G , McDougall GJ , Stewart D , Rowland I , Crozier A , Gill CIR . Comparison of in vivo and in vitro digestion on polyphenol composition in lingonberries: Potential impact on colonic health. Biofactors. (2014) ;40: :611–23. |
[22] | Deighton N , Brennan R , Finn C , Davies HV . Antioxidant properties of domesticated and wild Rubus species. J Sci Food Agric. (2000) ;80: :1307–13. |
[23] | Hellström JK , Mattila PH . HPLC determination of extractable and unextractable proanthocyanidins in plant materials. J Agric Food Chem. (2008) ;56: :7617–24. |
[24] | Andersen ØM . Chromatographic separation of anthocyanins in cowberry (Lingonberry, Vaccinium vitis-idaea L). J Food Sci. (1985) ;50: :1230–2. |
[25] | Cho MJ , Howard LR , Prior RL , Clark JR . Flavonoid glycosides and antioxidant capacity of various blackberry, blueberry and red grape genotypes determined by high-performance liquid chromatography/mass spectrometry. J Sci Food Agric. (2004) ;84: :1771–82. |
[26] | Tarascou I , Mazauric JP , Meudec E , Souquet JM , Cunningham D , Nojeim S , Cheynier V , Fulcrand H . Characterisation of genuine and derived cranberry proanthocyanidins by LC-ESI-MS. Food Chemistry. (2011) ;128: :802–10. |
[27] | Krueger CG , Vestling MM , Reed JD . Matrix-assisted laser desorption-ionization time-of-flight mass spectrometry of anthocyanin-poly-flavan-3-ol oligomers in cranberry fruit (Vaccinium macrocarpon, Ait.) and spray-dried cranberry juice. In: Red Wine Colour, ACS Symposium Series 886. American Chemical Society. (2004) , pp. 232–46. |
[28] | Reed JD , Krueger CG , Vestling MM . MALDI-TOF mass spectrometry of oligomeric food polyphenols. Phytochemistry. (2005) ;66: :2248–63. |
[29] | McDougall GJ , Shpiro F , Dobson P , Smith P , Blake A , Stewart D . Different polyphenolic components of soft fruits inhibit α-amylase and α-glucosidase. J Agric Food Chem. (2005) ;53: :2760–66. |
[30] | Yousef GG , Brown AF , Funakoshi Y , Mbeunkui F , Grace MH , Ballington JR , Loraine A , Lila MA . Efficient quantification of the health-relevant anthocyanin and phenolic acid profiles in commercial cultivars and breeding selections of blueberries (Vaccinium spp.). J Agric Food Chem. (2013) ;61: :4806–15. |
[31] | Timberlake CF , Bridle P . Interactions between anthocyanins, phenolic compounds, and acetaldehyde and their significance in red wines. Amer J Enol Vitic. (1976) ;27: :97–105. |
[32] | Shoji T , Goda Y , Toyoda M , Yanagida A , Kanda T . Characterization and structures of anthocyanin pigments generated in rosé cider during vinification. Phytochemistry. (2002) ;59: :183–9. |
[33] | Mateus N , Silva AM , Santos-Buelga C , Rivas-Gonzalo JC , de Freitas V . Identification of anthocyanin-flavanol pigments in red wines by NMR and mass spectrometry. J Agric Food Chem. (2002) ;50: :2110–6. |
Figures and Tables
Diagram1
Structure of putative ethyl linked anthocyanin (epi)catechin component. Adapted from structures in Kruger et al. (2004). R = hexose moiety shows main fragmentations in positive mode; loss of hexose (R) and loss of epicatechin to yield a vinyl cyanidin group. Additional fragmentations only seen in negative mode.
= loss of RDA fragment from epicatechin (-152).
= loss of vinyl (epi)catechin group (-316).
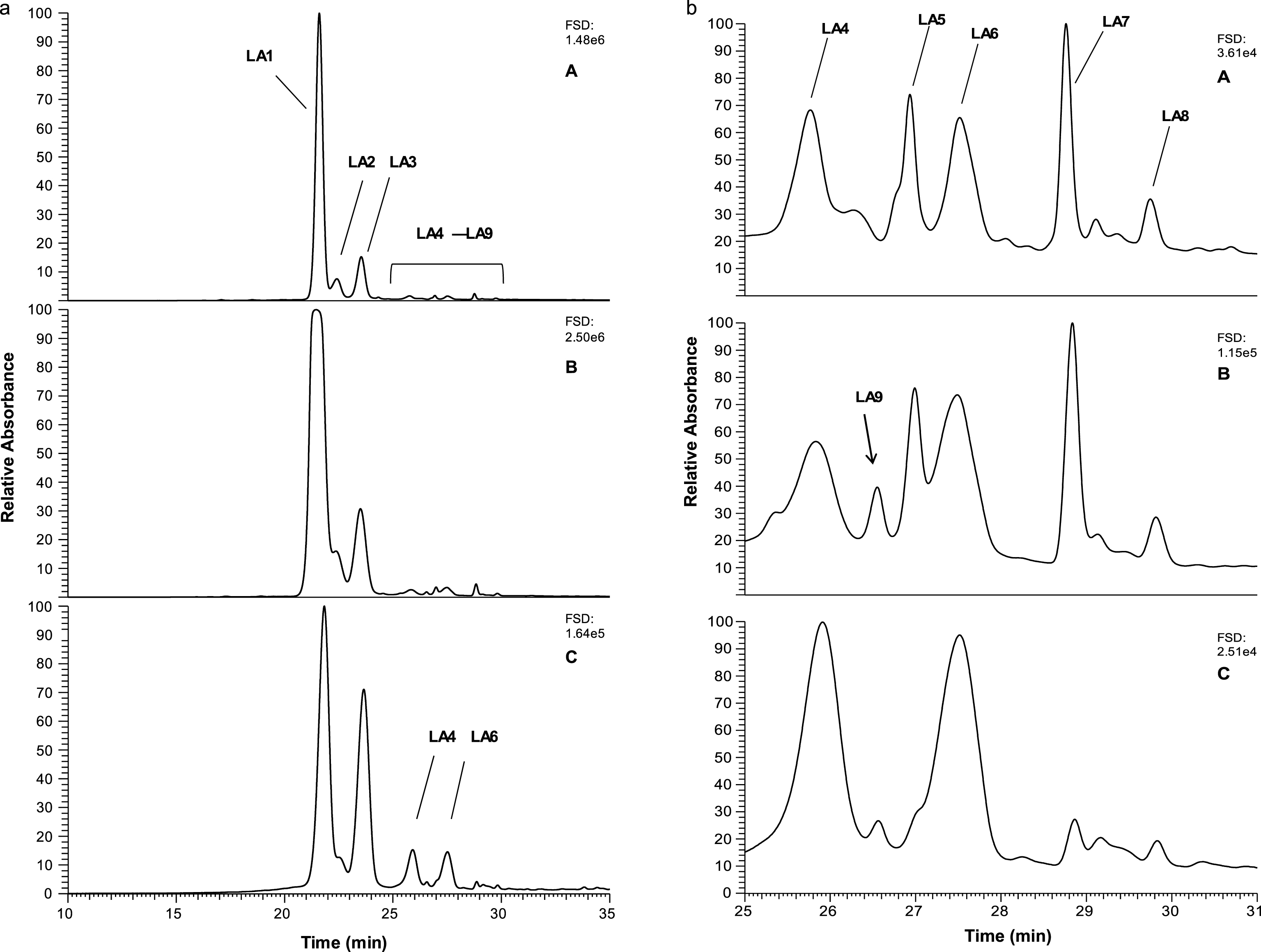
Fig.1
a) Anthocyanin-like peaks in lingonberry extracts and fractions. Figure 1a, Panel A shows the PDA profile at 520 nm of the lingonberry original extract, Panel B shows the PDA profile at 520 nm of the water fraction. Panel C shows the PDA profile at 520 nm of the ethyl acetate fraction. b) Anthocyanin-like peaks in lingonberry extracts and fractions. Figure 1b shows the area from 25 to 31 min expanded for each sample in panels A-C. The figures in the top right corner are the full scale deflections for the PDA detector at 520 nm. Annotated peaks are discussed in the text and in Table 1.
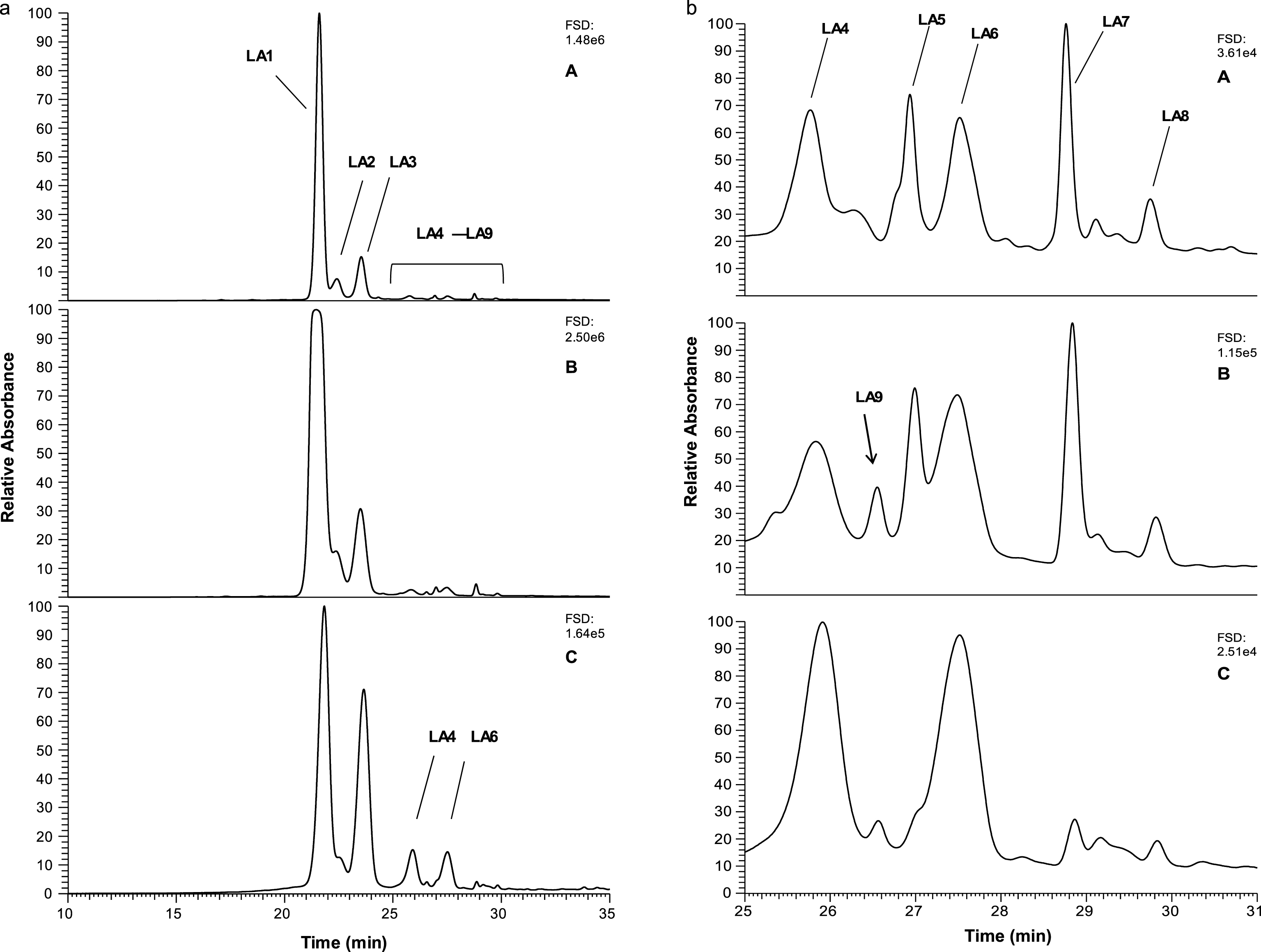
Fig.2
MS2 of peak LA7: Negative mode. The figure in the top right corner is the full scale deflection of the MS detector. MS signals in bold are discussed in the text.
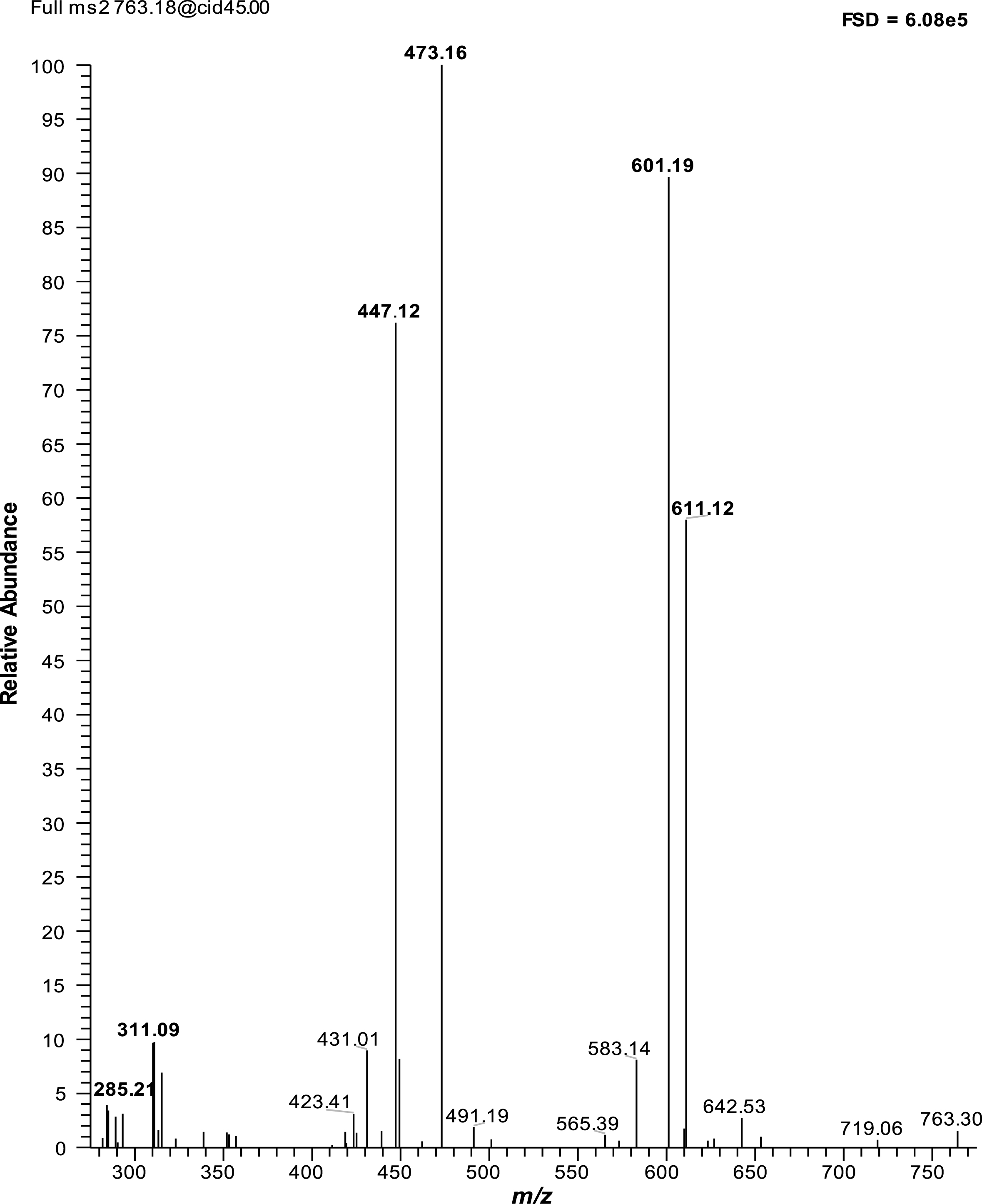
Fig.3
Fractionation of lingonberry m/z 765 component on Sephadex LH-20. Panel A shows the MS profile of original lingonberry sample at m/z [M+H]+ 765, Panel B shows the MS profile of LH-unbound sample at m/z [M+H]+ 765, Panel C shows the MS spectra & Panel D shows the MS2 spectra of m/z [M+H]+ 765. Data for panels C & D was taken from the LH-20 unbound fraction.
![Fractionation of lingonberry m/z 765 component on Sephadex LH-20. Panel A shows the MS profile of original lingonberry sample at m/z [M+H]+ 765, Panel B shows the MS profile of LH-unbound sample at m/z [M+H]+ 765, Panel C shows the MS spectra & Panel D shows the MS2 spectra of m/z [M+H]+ 765. Data for panels C & D was taken from the LH-20 unbound fraction.](https://content.iospress.com:443/media/jbr/2016/6-1/jbr-6-1-jbr108/jbr-6-jbr108-g003.jpg)
Fig.4
Ethyl linked anthocyanin (epi)catechin derivatives in blueberry extracts. Panel A shows the PDA profile at 520 nm of the blueberry extract from variety Bluecrop. Panel B shows the MS profile at m/z [M+H]+ 851 with peaks BbA1 and BbA2 denoted; Panel C shows the MS spectra under peak BbA1 & Panel D shows the MS2 spectra of m/z [M+H]+ 851. Figures in the top right hand corner are the full scale deflections (FSD) of the detectors.
![Ethyl linked anthocyanin (epi)catechin derivatives in blueberry extracts. Panel A shows the PDA profile at 520 nm of the blueberry extract from variety Bluecrop. Panel B shows the MS profile at m/z [M+H]+ 851 with peaks BbA1 and BbA2 denoted; Panel C shows the MS spectra under peak BbA1 & Panel D shows the MS2 spectra of m/z [M+H]+ 851. Figures in the top right hand corner are the full scale deflections (FSD) of the detectors.](https://content.iospress.com:443/media/jbr/2016/6-1/jbr-6-1-jbr108/jbr-6-jbr108-g004.jpg)
Table 1
Anthocyanin-like peaks in lingonberry extracts
Peak No. | TR | PDA | m/z+ | MS2 | Putative Identity |
LA1 | 21.60 | 515, 280 | 448.99, 287.27 | 287.27 | Cyanidin galactoside |
LA2 | 22.41 | 515, 280 | 449.04, 287.30 | 287.27 | Cyanidin glucoside |
LA3 | 23.54 | 515, 280 | 419.01, 287.27 | 287.20 | Cyanidin arabinoside |
LA4 | 25.77 | 520, 280 | 419.01, 287.27 | 287.20 | Cyanidin pentose |
LA5 | 26.93 | 530, 280 | 765.13, 475.01, 313.27, 287.27 | 603.08, 474.95, 313.19 | Cyanidin-ethyl epicatechin hexose |
LA6 | 27.50 | 520, 280 | 491.04, 287.30 | 287.27 | Cyanidin acetyl hexose |
LA7 | 28.76 | 530, 280 | 765.12, 475.05, 313.26 | 602.98, 474.97, 313.18 | Cyanidin-ethyl epicatechin hexose |
LA8 | 29.75 | 530, 280 | 735.10, 313.27 | 602.98, 444.96, 313.18 | Cyanidin-ethyl epicatechin pentose |
LA9 | 26.55 | 530, 280 | 751.15 | 589.06, 299.14 | Anthocyanin ethyl epicatechin derivative |
All retention times and MS data taken from the water extract sample. Figures in bold represent the dominant m/z or MS2 values. Assignments are supported by published data [1, 9, 24].