Retromer Stabilization Improves Cognitive Function and Synaptic Plasticity in a Mouse Model of Down Syndrome
Abstract
Background:
Retromer complex proteins are decreased in postmortem brain tissues from Down syndrome subjects and inversely correlate with the Alzheimer’s disease-like neuropathology. However, whether targeting in vivo the retromer system affects cognitive deficits and synaptic function in Down syndrome remains unknown.
Objective:
The aim of the current study was to examine the effects of pharmacological retromer stabilization on cognitive and synaptic functions in a mouse model of Down syndrome.
Methods:
Ts65dn mice were administered the pharmacological chaperone, TPT-172, or vehicle from 4 to 9 months of age and then assessed for changes in cognitive function. To assess the effects of TPT-172 on synaptic plasticity, hippocampal slices from Ts65dn mice were incubated in TPT-172 and used for field potential recordings.
Results:
Chronic TPT-172 treatment improved performance in cognitive function tests, its incubation with hippocampal slices ameliorated synaptic function response.
Conclusion:
Pharmacological stabilization of the retromer complex improves synaptic plasticity and memory in a mouse model of Down syndrome. These results support the therapeutic potential of pharmacological retromer stabilization for individual with Down syndrome.
INTRODUCTION
Down syndrome (DS) is a congenital condition caused by the presence of extra genetic material originating from an extra copy of the human chromosome 21 (HSA21) and occurs in about 1 in 800 births worldwide. Trisomy of chromone 21 is one of the most significant risk factors for the development of early onset Alzheimer’s disease (AD) [1], and people with DS develop the neuropathological hallmarks of AD and severe cognitive decline by age 40 [2]. However, the onset of clinical symptoms varies, suggesting that factors outside of HSA21 modulate the transition from preclinical stages of the disease to full AD-like dementia.
In recent years, genetic and functional studies have highlighted the endo-lysosomal network as a key biological pathway central to AD pathogenesis [3–5]. Within this network, the retromer complex is a multi-protein endosomal sorting system that regulates sorting of cargoes from early endosomes and, consequently, is an essential regulator of intracellular protein trafficking and homeostasis. The retromer cargo recognition core, composed of vacuolar protein sorting (VPS) 35, VPS26, and VPS29, is the central functional unit of the retromer complex system that directs cargoes via three distinct trafficking routes: to the plasma membrane for recycling, retrograde to the trans-Golgi, or to the lysosome for degradation. Regulated and balanced coordination of these three trafficking pathways is crucial for neuronal health and physiology. Conversely, dysregulation of this system leads to aberrant endosomal protein sorting and trafficking, and consequently, vulnerability to neurodegenerative processes.
Dysfunction of the retromer complex, secondary to decreases in VPS35 and VPS26 proteins, has been reported brains of human patients with AD [6], and enhancing retromer function has been shown to influence both amyloid and tau neuropathology in models of the disease [7, 8]. We previously showed that the retromer complex proteins are also reduced in postmortem brain tissues and induced pluripotent stem cell (iPSC) derived neurons from subjects with DS [9, 10]. Furthermore, we demonstrated that in vitro enhancement of retromer complex function significantly reduces classical AD-like pathology measures in DS iPSC-derived neurons [9]. To expand upon our previous findings, in the current study we utilize the Ts65dn mouse model of DS to examine the biological effects of chronic in vivo retromer stabilization on memory and synaptic function. Herein we report that stabilization of the retromer complex by a small pharmacological chaperone results in improved cognition and synaptic function in DS mice.
METHODS
Animals and treatments
All animal care and procedures were approved by the Institutional Animal Care and Usage Committee in accordance with the US National Institutes of Health guidelines. Ts65dn mice (stock 005252) were purchased from Jackson laboratories. Both males and females were used in this study. Ts65dn mice were oral gavaged 3 times weekly from 4 to 9 months of age with 50 mg/kg of TPT-172 (Benjamin Blass, School of Pharmacy, Temple University) or vehicle. Mice were monitored for changes in weight, health, and signs of toxicity.
Behavioral tests
Ts65dn mice treated with TPT-172 or vehicle underwent behavioral assessment between 8 and 9 months of age. All mice were handled for 3-4 consecutive days prior to behavioral testing. The Morris water maze (MWM), Y-Maze, and Fear Conditioning behavioral paradigms were conducted, recorded, and analyzed as previously described [11, 12].
Electrophysiology slice preparation
Hippocampal slices were prepared as previously described [11]. To assess the effect of TPT-172 on synaptic activity, several hippocampal slices from each animal were incubated in 25μM TPT-172 for 2 h following the recovery period. Both control and TPT-172 treated slices from each animal were used for field potential recordings.
Field potential recordings
Field excitatory postsynaptic potentials (fEPSPs) were evoked via stimulation of hippocampal Schaffer collaterals as previously described [11]. To study basal synaptic activity, the slices were stimulated with current pulses of differing intensities and constant increments of 20 second inter-pulse intervals. The input to output curve was generated from the value of the fEPSP slope over the amount of injected current. Short-term plasticity (STP) and long-term plasticity (LTP) were assessed by the intensity of stimulation necessary to evoke an fEPSP at 1/3 of the maximal response, as determined by analysis of the input-output curve. STP was evaluated with time dependency of the paired-pulse facilitation protocol. In the paired-pulse facilitation protocol, paired current pulses at differing inter-pulse intervals were used to evoke paired fEPSPs. The ratio of the fEPSP slope at pulse 2 to the fEPSP slope at pulse 1 was determined using respective inter-pulse interval time points. The high-frequency stimulation protocol was used to induce LTP. The baseline response to a continuous stimulation was recorded for 20 minutes prior to LTP induction. Three consecutive tetanic pulses of 1-s duration and 100 Hz were applied at 60-s inter-pulse intervals to induce LTP. The value of the fEPSP slope following LTP induction was normalized to the baseline fEPSP slope. Data acquisition and analysis for was performed using the Clampfit 10.7 software from the PClamp 10.7 software suite (Molecular Devices, USA).
General statistical analysis
Data are expressed as mean±standard error of the mean (SEM). For comparison of two groups, the unpaired two-tailed student t test was used. Statistical significance was set at p < 0.05. GraphPad Prism for Mac and for Windows version 9.00 was used for the above data analyses.
RESULTS
Pharmacological retromer stabilization improves cognition in Ts65dn mice
The main goal of the current study was to examine whether pharmacological stabilization of the retromer complex via chronic in vivo administration of TPT-172 would improve cognitive function in a DS mouse model. Thus, Ts65dn mice were administered with TPT-172 or vehicle via oral-gavage 3 times weekly from approximately 4 to 9 months of age. No significant changes in general health, weight, or survival were detected between mice receiving vehicle or TPT-172 (Fig. 1A, B). During the last month of treatment (from about 8–9 months of age) mice were subjected to behavioral learning and memory paradigms. In the Y-maze test, mice did not differ in the overall number of arm entries, however, mice receiving TPT-172 had a significant increase in percent alternations (Fig. 1C). No significant differences were observed in the MWM test between the two groups, though there were trends toward improvement in both the training and probe phases in mice receiving TPT-172 (Fig. 1D). In the fear conditioning paradigm, there were no baseline differences in freezing response in the training phase, but there was a significant increase of the freezing response in the contextual recall test, and a trend toward improvement in the cued recall in mice administered TPT-172 (Fig. 1E). Thus, overall, chronic administration of TPT-172 improved cognitive impairments in Ts65dn mice.
Fig. 1
Chronic TPT-172 administration in Ts65dn mice improves performance in learning and memory behavioral paradigms. A) Average weight of Ts65dn mice receiving vehicle (CTR) or TPT-172 (TPT) throughout the study. B) Percent survival of Ts65dn mice receiving vehicle (CTR) or TPT-172 (TPT) throughout the study until the time of sacrifice (9 months of age). C) Number of entries and percent alternations in the Y-maze paradigm. D) Latency to reach the hidden platform over 4 consecutive days of training in the Morris water maze training phase, and latency to reach the platform zone and number of entries into the platform zone in the Morris water maze probe test. E) Percent freezing in the training, contextual, and cued phases of the fear conditioning paradigm. (n = 11 CTR, 13 TPT). Values represent mean±standard error of the mean, *p < 0.05.
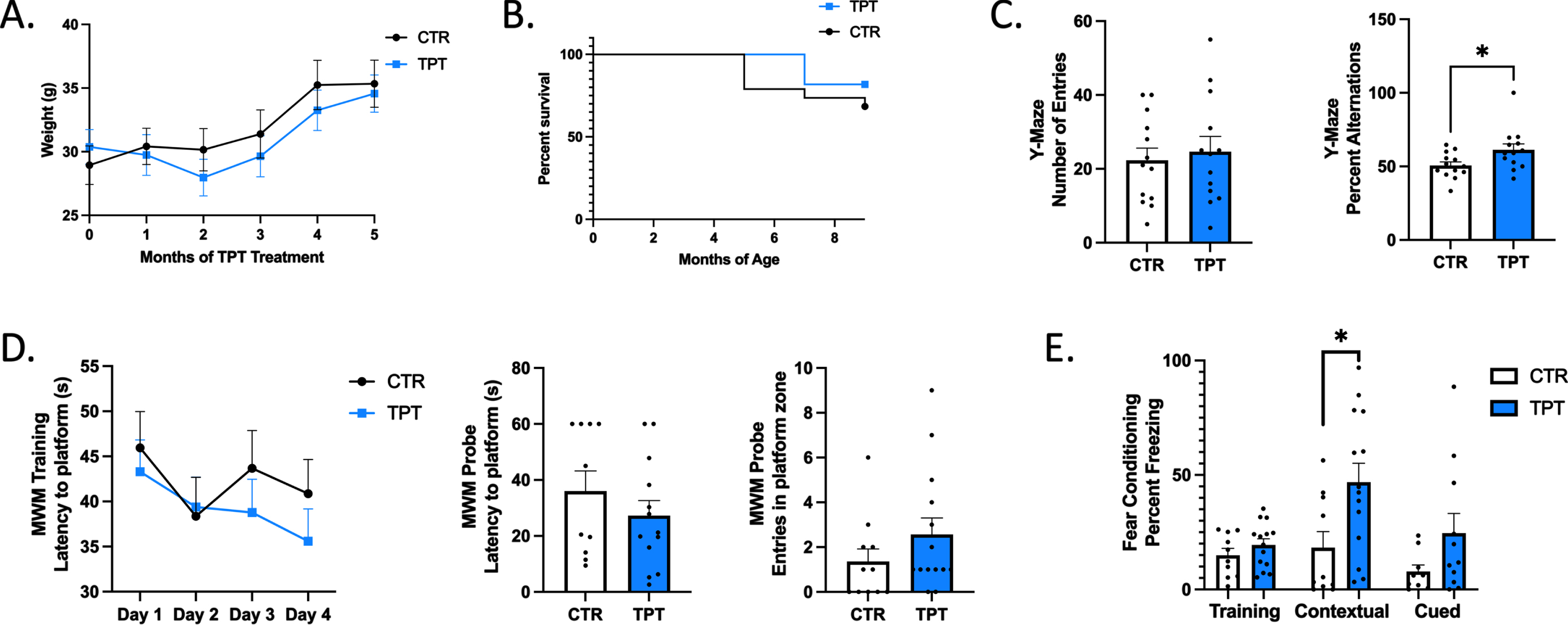
TPT-172 improves synaptic plasticity
Given that we observed improved cognitive performance in the learning and memory behavioral paradigms following pharmacological stabilization of the retromer complex, we next wanted to investigate directly whether the drug influenced synaptic plasticity. To this end, we examined basal synaptic activity, STP, and LTP in the CA1 region of hippocampal slices from 9-month-old Ts65dn mice incubated with vehicle or TPT-172. To assess synaptic activity, we stimulated Schaffer collaterals and fEPSPs were recorded from the stratum radiatum in the CA1 region (Fig. 2). Basal synaptic activity did not differ between groups (Fig. 2A); however, the early-phase LTP response was trending toward improvement (Fig. 2C), and we saw a significant increase in paired-pulse facilitation (STP) at 25 and 100 ms inter-pulse intervals in hippocampal slices that were incubated with TPT-172 (Fig. 2B).
Fig. 2
Acute TPT-172 administration improves synaptic plasticity. A) The input-output curve of the fEPSP slope versus the injected current. B) Time dependence of paired-pulse facilitation of the fEPSP slope, presented as a ratio of the fEPSP slope at pulse 1 to the fEPSP slope at pulse 2, for different time-points. C) LTP induced with 3 consecutive 1-s high-frequency stimulation pulses of 100 Hz at a 20-s inter-pulse interval. The values of the fEPSP slope are presented as the percentage of response after induction of LTP to the averaged baseline response. Ts65dn hippocampal slices incubated for 2 h in vehicle [(n = 7 CTR), or TPT-172 (n = 7 TPT)]. Values represent mean±standard error of the mean, *p < 0.05.
![Acute TPT-172 administration improves synaptic plasticity. A) The input-output curve of the fEPSP slope versus the injected current. B) Time dependence of paired-pulse facilitation of the fEPSP slope, presented as a ratio of the fEPSP slope at pulse 1 to the fEPSP slope at pulse 2, for different time-points. C) LTP induced with 3 consecutive 1-s high-frequency stimulation pulses of 100 Hz at a 20-s inter-pulse interval. The values of the fEPSP slope are presented as the percentage of response after induction of LTP to the averaged baseline response. Ts65dn hippocampal slices incubated for 2 h in vehicle [(n = 7 CTR), or TPT-172 (n = 7 TPT)]. Values represent mean±standard error of the mean, *p < 0.05.](https://content.iospress.com:443/media/jad/2023/94-2/jad-94-2-jad230205/jad-94-jad230205-g002.jpg)
DISCUSSION
Our study shows that pharmacological stabilization of the retromer complex system results in an amelioration of cognitive function and synaptic plasticity in a mouse model of DS. The retromer system is an endosomal sorting complex that regulates numerous aspects of normal inter- and intracellular trafficking by sorting endocytic cargoes into the retrograde, recycling, or degradation pathways [13]. Because of its actions on these three major routes, by targeting the retromer complex system in the current study we have implemented a whole cell biology approach to address the effect on cognitive deficits in a mouse model of DS. Through trafficking of the protease, cathepsin D, the retromer complex can regulate lysosomal degradation of pathogenic proteins, while also limiting amyloidogenic processing of AβPP in the early endosomes and by doing so it could also improve synaptic function and behavioral impairments. Furthermore, the retromer’s actions in the recycling pathway towards the cell membrane can facilitate synaptic plasticity by transporting glutamate receptors to the synapse [4, 14, 15].
As a follow up to our previous studies demonstrating the role of in vitro retromer stabilization in reducing AD-like pathology in DS iPSC-derived neurons, we now wanted to determine the biological and functional consequences of chronic modulation of the retromer function in an animal model of DS. To increase retromer function in Ts65dn mice, we utilized a small molecule pharmacological chaperone, TPT-172, which is known to stabilize the retromer complex protein [9]. Thus, this molecule enhances retromer complex function by increasing stability of the VPS35-VPS29 binding domain, the weakest link of the VPS35-VPS26-VPS29 heterotrimer, and consequently protecting the recognition core from denaturation [9,16]. To this end, we treated Ts65dn mice with TPT-172 for 5 months starting at 4 months of age; a time just prior to significant development of aging related cognitive and neuropathological changes in this model [17]. This chronic stabilization of the retromer complex resulted in improved performance in learning and memory tasks, showing that TPT-172 can indeed improve cognition. Although we do not have data showing that the pharmacological chaperone we used in our study has crossed the blood-brain barrier in our mice, we are confident that this is the case based on the similarity with other small pharmacological chaperones, most of which are predicted to have a good brain permeability [18, 19]. Additionally, the same drug was previously shown to be effective in reducing in vivo the classical AD-like neuropathology in mice. Thus, our current results agree with our previous studies in which TPT-172 was chronically administered to a mouse model of AD with amyloid plaques and tangles, the 3xTg mice [7, 8]. However, by contrast with that study in the current one we did not investigate a possible effect of the pharmacologic chaperone on the neuropathology of the mice. Based on the obtained results, future studies are planned to address this important biological question.
Considering that TPT-172 treatment was able to improve performance in learning and memory tasks, we next wanted to directly assess the effects of retromer stabilization on synaptic plasticity. To this end, we incubated hippocampal slices from 9-month-old Ts65dn mice in TPT-172 or vehicle prior to electrophysiological assessment. Acute TPT-172 treatment significantly enhanced short-term plasticity, aligning with the role of the retromer complex in regulating synaptic function through the recycling of synaptic proteins [20, 21]. Meanwhile acute hippocampal slice treatment with TPT-172 did not show any changes in basal synaptic activity and showed insignificant trends toward improvement in the early phase of LTP. It is likely that significant effects of acute treatment with TPT-172 on short-term plasticity along with insignificant changes in LTP may indicate on the importance of treatment duration with retromer complex stabilization, however additional studies are needed to address this hypothesis. Nevertheless, enhancing retromer stability via chronic administration TPT-172 improved cognition, suggesting that this approach facilitates synaptic plasticity in Ts65dn mice. Interestingly, our findings are supported by recent studies which have demonstrated the role of the retromer complex system in the regulation of pre-synaptic vesicle release [22, 23] and post-synaptic trafficking of AMPA receptors [23].
In conclusion, the results presented in the current study demonstrate that stabilization of the retromer complex in DS addresses aging-related cognitive deficits in the Ts65dn mouse model of DS. Our study provides further support for the role of pharmacological stabilization of the retromer complex as a viable therapeutic approach against cognitive decline in DS subjects.
ACKNOWLEDGMENTS
Domenico Praticò is the Scott Richards North Star Charitable Foundation Chair for Alzheimer’s disease research.
FUNDING
The work presented in this article was in part funded by grants from the National Institute of Health (AG055707 and AG056689).
CONFLICT OF INTEREST
Domenico Pratico is an Editorial Board Member of this journal but was not involved in the peer-review process nor had access to any information regarding its peer-review.
The authors have no conflicting financial interests to disclose.
DATA AVAILABILITY
The data supporting the findings of this study are available on request from the corresponding author.
REFERENCES
[1] | Wiseman FK , Al-Janabi T , Hardy J , Karmiloff-Smith A , Nizetic D , Tybulewicz VL , Fisher EMC , Strydom A ((2015) ) A genetic cause of Alzheimer disease: Mechanistic insights from Down syndrome. Nat Rev Neurosci 16: , 564–574. |
[2] | Head E , Powell D , Gold BT , Schmitt FA ((2012) ) Alzheimer’s disease in Down syndrome. Eur J Neurodegener Dis 1: , 353–364. |
[3] | Karch CM , Goate AM ((2015) ) Alzheimer’s disease risk genes and mechanisms of disease pathogenesis. Biol Psychiatry 77: , 43–51. |
[4] | Vagnozzi AN , Pratico D ((2019) ) Endosomal sorting and trafficking, the retromer complex and neurodegeneration. Mol Psychiatry 24: , 857–868. |
[5] | Wong CO ((2020) ) Endosomal-lysosomal processing of neurodegeneration-associated proteins in astrocytes. Int J Mol Sci 21: , 5149. |
[6] | Wang X , Huang T , Bu G , Xu H ((2014) ) Dysregulation of protein trafficking in neurodegeneration. Mol Neurodegener 9: , 31. |
[7] | Li JG , Blass BE , Pratico D ((2023) ) Beneficial effect of a small pharmacologic chaperone on the established Alzheimer’s disease phenotype. J Alzheimers Dis 91: , 463–469. |
[8] | Li JG , Chiu J , Ramanjulu M , Blass BE , Pratico D ((2020) ) A pharmacological chaperone improves memory by reducing Abeta and tau neuropathology in a mouse model with plaques and tangles. Mol Neurodegener 15: , 1. |
[9] | Curtis ME , Smith T , Blass BE , Pratico D ((2022) ) Dysfunction of the retromer complex system contributes to amyloid and tau pathology in a stem cell model of Down syndrome. Alzheimers Dement (N Y) 8: , e12334. |
[10] | Curtis ME , Yu D , Pratico D ((2020) ) Dysregulation of the retromer complex system in Down syndrome. Ann Neurol 88: , 137–147. |
[11] | Lauretti E , Nenov M , Dincer O , Iuliano L , Pratico D ((2020) ) Extra virgin olive oil improves synaptic activity, short-term plasticity, memory, and neuropathology in a tauopathy model. Aging Cell 19: , e13076. |
[12] | Di Meco A , Pratico D ((2019) ) Early-life exposure to high-fat diet influences brain health in aging mice. Aging Cell 18: , e13040. |
[13] | Burd C , Cullen PJ ((2014) ) Retromer: A master conductor of endosome sorting. Cold Spring Harb Perspect Biol 6: , a016774. |
[14] | Qureshi YH , Baez P , Reitz C ((2020) ) Endosomal trafficking in Alzheimer’s disease, Parkinson’s disease, and neuronal ceroid lipofuscinosis. Mol Cell Biol 40: , e00262-e00220. |
[15] | Li C , Shah SZ , Zhao D , Yang L ((2016) ) Role of the retromer complex in neurodegenerative diseases. Front Aging Neurosci 8: , 42. |
[16] | Mecozzi VJ , Berman DE , Simoes S , Vetanovetz C , Awal MR , Patel VM , Scheider RT , Petsko GA , Ringe D , Small SA ((2014) ) Pharmacological chaperones stabilize retromer to limit APP processing. Nat Chem Biol 10: , 443–449. |
[17] | Hamlett ED , Boger HA , Ledreux A , Kelley CM , Mufson EJ , Falangola MF , Guilfoyle DN , Nixon RA , Patterson D , Duval N , Granholm A-C E ((2016) ) Cognitive impairment, neuroimaging, and Alzheimer’s neuropathology in mouse models of Down syndrome. Curr Alzheimer Res 1: , 335-e52. |
[18] | DeWeerdt S ((2016) ) Drug development: Through the barrier. Nature 537: , S154–S157. |
[19] | Tran ML , Genisson Y , Ballereau S , Dehoux C ((2020) ) Second-generation pharmacological chaperones: Beyond inhibitors. Molecules 25: , 3145. |
[20] | Brodin L , Shupliakov O ((2018) ) Retromer in synaptic function and pathology. Front Synaptic Neurosci 10: , 37. |
[21] | Ye H , Ojelade SA , Li-Kroeger D , Zuo Z , Wang L , Li Y , Gu JYJ , Tepass U , Rodal AA ((2020) ) Retromer subunit, VPS29, regulates synaptic transmission and is required for endolysosomal function in the aging brain. Elife 9: , e51977. |
[22] | Inoshita T , Arano T , Hosaka Y , Meng H , Umezaki Y , Kosugi S , Morimoto T , Koike M , Chang H-Y , Imai Y , Hattori N ((2017) ) Vps35 in cooperation with LRRK2 regulates synaptic vesicle endocytosis through the endosomal pathway in Drosophila. Hum Mol Genet 26: , 2933–2948. |
[23] | Temkin P , Morishita W , Goswami D , Arendt K , Chen L , Malenka R ((2017) ) The retromer supports AMPA receptor trafficking during LTP. Neuron 94: , 74-82 e85. |