Impact of Yoga Versus Memory Enhancement Training on Hippocampal Connectivity in Older Women at Risk for Alzheimer’s Disease
Abstract
Background:
Yoga may be an ideal early intervention for those with modifiable risk factors for Alzheimer’s disease (AD) development.
Objective:
To examine the effects of Kundalini yoga (KY) training versus memory enhancement training (MET) on the resting-state connectivity of hippocampal subregions in women with subjective memory decline and cardiovascular risk factors for AD.
Methods:
Participants comprised women with subjective memory decline and cardiovascular risk factors who participated in a parent randomized controlled trial (NCT03503669) of 12-weeks of KY versus MET and completed pre- and post-intervention resting-state magnetic resonance imaging scans (yoga: n = 11, age = 61.45±6.58 years; MET: n = 11, age = 64.55±6.41 years). Group differences in parcellated (Cole-anticevic atlas) hippocampal connectivity changes (post- minus pre-intervention) were evaluated by partial least squares analysis, controlling for age. Correlations between hippocampal connectivity and perceived stress and frequency of forgetting (assessed by questionnaires) were also evaluated.
Results:
A left anterior hippocampal subregion assigned to the default mode network (DMN) in the Cole-anticevic atlas showed greater increases in connectivity with largely ventral visual stream regions with KY than with MET (p < 0.001), which showed associations with lower stress (p < 0.05). Several posterior hippocampal subregions assigned to sensory-based networks in the Cole-anticevic atlas showed greater increases in connectivity with regions largely in the DMN and frontoparietal network with MET than with KY (p < 0.001), which showed associations with lower frequency of forgetting (p < 0.05).
Conclusion:
KY training may better target stress-related hippocampal connectivity, whereas MET may better target hippocampal sensory-integration supporting better memory reliability, in women with subjective memory decline and cardiovascular risk factors.
INTRODUCTION
Early preventive interventions that focus on modifiable risk factors may delay the onset of the symptoms of Alzheimer’s disease (AD), an irreversible neurodegenerative disease, in high-risk populations [1–3]. Known modifiable risk factors include cardiovascular factors such as obesity, high blood pressure, and high cholesterol; non-modifiable risk factors include female sex, APOE E4, and family history of cardiovascular disease [4–9]. Yoga has known beneficial effects on cardiovascular functioning, including blood pressure and cardiorespiratory fitness [10–12]. Additionally, neuroimaging studies suggest that yoga has neuroprotective effects. In particular, yoga training and regular practice show positive effects on the volume of the hippocampus, a region implicated in subjective memory decline and AD [13–19]. Furthermore, yoga has been shown to be safe and have positive effects on cognitive functioning in older individuals, including those with mild cognitive impairment or early-stage dementia [20–23]. Thus, yoga may be an ideal early intervention for those with modifiable risk factors for AD development.
Kundalini yoga (KY) is a form of yoga that combines movement and meditation, with a greater focus on breathing, mantra recitation, and mental visualization compared to that in other common yoga practices (e.g., hatha), which mostly focus on postures. This combination of greater focus on breathing and mental engagement with less and more gentle movement renders KY an ideal form of yoga for older adults. Kirtan Kriya (KK) is a brief yogic meditation in the KY tradition that has been shown to improve perceived stress, psychological well-being, quality of life, and mood in individuals with subjective cognitive decline [24]. We previously reported beneficial effects of KY combined with an at-home KK practice (KY+KK) on depression, resilience, and executive functioning in a randomized controlled trial (RCT) in older adults with mild cognitive impairment [25–27]. Further, our recent RCT of KY+KK versus memory enhancement training (MET) suggested a more robust neuroprotective effect on right hippocampal volume with KY+KK than with MET in older women with subjective memory decline and cardiovascular risk factors. As resting-state connectivity of the hippocampus may be more sensitive to cognitive changes than hippocampal volumes [13], an analysis of hippocampal resting-state connectivity may further elucidate the neuroprotective effects of KY+KK. Additionally, hippocampal subregions are differentially involved in memory functions [28]. Therefore, we investigated the effect of yoga (KY+KK) versus MET on parcellated hippocampal resting-state connectivity in women with subjective cognitive decline and cardiovascular risk factors for AD. We hypothesized greater increases in hippocampal connectivity with regions implicated in memory decline with KY+KK than with MET.
MATERIALS AND METHODS
Participants
Participants comprised women with subjective memory decline and cardiovascular risk factors who participated in a parent randomized controlled trial (RCT; NCT03503669) of yoga (KY+KK) versus MET. Among the 63 participants (yoga: n = 26; MET: n = 37) who completed the parent RCT, a subsample (yoga: n = 11, mean age±standard deviation [SD] = 61.45±6.58 years; MET: n = 11, mean age±SD = 64.55±6.41 years) completed resting-state magnetic resonance imaging (rsMRI) at baseline and 12 weeks of follow up (Table 1). Patients with a self-reported decline in memory function during the previous year, one or more cardiovascular risk factors, and sufficient English proficiency and mental capacity to provide informed consent and actively participate in the study were included. Cardiovascular risk factors included an atherosclerotic cardiovascular disease risk score within the 7.5th percentile on the Cerebrovascular Risk Factor Prediction Chart, atherosclerotic findings on hematologic testing, myocardial infarction (at least 6 months prior), diabetes, and current pharmacological treatment for blood pressure or hyperlipidemia. Exclusion criteria were as follows: history of a psychiatric or neurological disorder; surgery within the 3 months prior; disabilities such as severe visual or hearing impairments; dementia; current participation in cognitive training in a therapeutic setting; current psychoactive medication use; prior experience with KY or KK; myocardial infarction within 6 months; and MRI-incompatible implants or other imaging contraindications.
Table 1
Demographic and baseline clinical data
Yoga (n = 11) | MET (n = 11) | p | |
Age (y) | 62.0 (53–59) | 64 (54–76) | 0.26 |
Education (y) | 16.0 (13–18) | 16.0 (13–18) | 0.73 |
Race, n (%) | |||
White | 7 (64) | 6 (55) | 1.00 † |
Asian | 3 (27) | 2 (18) | |
Black | 0 (0) | 2 (18) | |
Hispanic | 0 (0) | 1 (9) | |
Other | 1 (9) | 0 (0) | |
Homework (d/wk) | 4.8 (1.8–6.9) | 5.0 (2.6–6.3) | 0.89 |
Anxiety (HAMA) | 4.5 (2–10) | 4.0 (1–14) | 0.52 |
Depression (BDI) | 7.5 (1–16) | 6.0 (3–21) | 1.00 |
Frequency of forgetting (MFQ1) | 4.25 (2.3–5.8) | 4.75 (3.3–5.9) | 0.22 |
Seriousness of forgetting (MFQ2) | 3.6 (2.4–6.4) | 4.1 (2.3–6.0) | 0.29 |
Perceived stress (PSS) ‡ | 22 (20–30) | 21 (15–26) | 0.75 |
Data are presented as median (range), unless otherwise indicated. p-values were determined by the Kruskal-Wallis test, unless otherwise indicated. †Fischer’s exact test for white vs non-white. ‡One participant in the yoga group had missing PSS data. HAMA, Hamilton Anxiety Rating Scale; BDI, Beck Depression Inventory; MET, memory enhancement training; MFQ1, Memory Function Questionnaire Factor 1; MFQ2, Memory Function Questionnaire Factor 2; PSS, Perceived Stress Scale.
The study was approved by the UCLA Institutional Review Board, and written informed consent was obtained from all participants.
Study design
The study methodology is depicted in Fig. 1. Participants were randomized (1:1) to undergo yoga (KY+KK) training or MET for 12 weeks. Classes (60 min/week) were held in person, in groups of 6–10 participants, and were supplemented by daily homework/practice assignments. Participants were required to attend at least 10 classes and not participant in any other mind-body practices. Adherence to at-home assignments was monitored by self-report at each class.
In the yoga group, classes were led by a certified KY instructor under the following protocol: tuning in (5 min); warm up (15 min); breathing techniques (i.e., Pranayama, 15 min); KK (12 min); final resting pose (i.e., Savasana, 10 min); and closing (3 min). In addition, participants received a CD with 12 min of KK for daily home practice. During KK, participants sat with their eyes closed and feet flat on the floor, visualized a beam of white light entering the center of the top of the head and exiting the middle of the forehead (i.e., the third eye), and chanted while touching the thumb of each hand to the other fingers sequentially as follows: “Saa” (i.e., birth; thumb to second finger), “Taa” (i.e., life; thumb to middle finger), “Naa” (i.e., death; thumb to ring finger), and “Maa” (i.e. rebirth; thumb to fifth finger). Five rounds were completed, with the first and fifth chanted out loud, second and fourth whispered, and the third thought silently, followed by a 1-min closing comprising energetic integration and meditation.
Fig. 1
Flowchart of the study methodology.
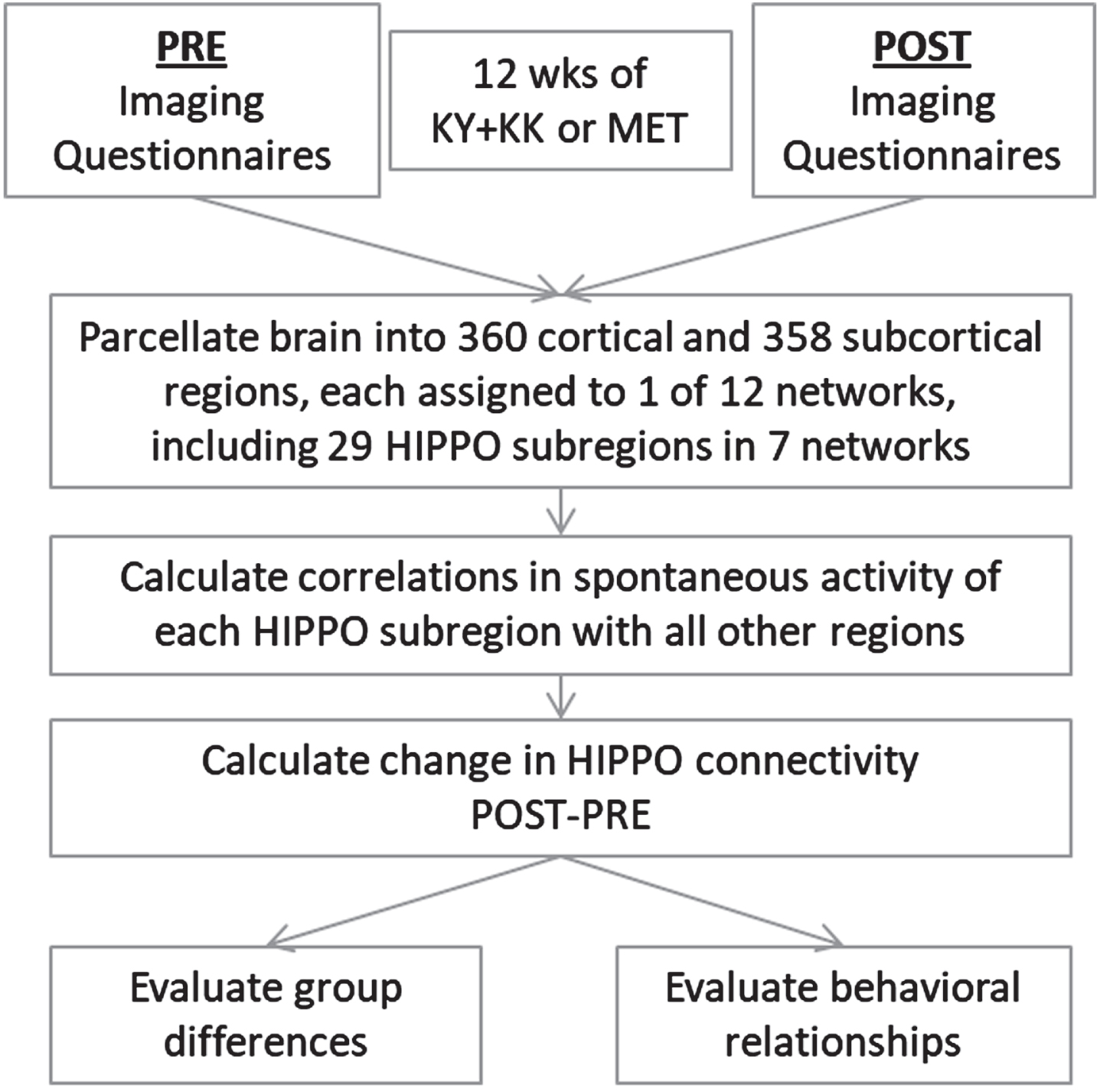
In the MET group, classes were led by a qualified memory training instructor and focused on teaching memory strategies using a scripted curriculum and companion workbook developed by researchers at the UCLA Longevity Center. Each class included a review of completed homework (15 min), and an introduction to new techniques, review of previous techniques, and group exercises (45 min). Participants were instructed to spend approximately 20 min daily on assigned homework. Specific techniques taught in the MET group include verbal associative techniques to remember lists (e.g., building a story around a list), organizational strategies (e.g., categorizing items on a grocery list), visual associative strategies to remember faces and names (adapted from [29]), strategies for remembering where an item was placed and recent activities (e.g., locking doors), and strategies for remembering future tasks (e.g., appointments).
Additional details regarding the randomization and protocols can be found in our previous publication [19].
Questionnaires
Participants completed questionnaires, including the Perceived Stress Scale (PSS) [30], Memory Function Questionnaire (MFQ) [31], Hamilton Anxiety Rating Scale [32], and Beck Depression Inventory [33], pre-intervention and post-intervention (at baseline and the 3-month follow up, respectively). The MFQ assesses subjective memory functioning and includes 4 components. In the present study, we focused on the first factor, namely the frequency of forgetting (MFQ1), which has been shown to more robustly reflect AD pathology than other MFQ components [34]; higher scores indicate better functioning. The PSS is a 10-item questionnaire that assesses feelings of stress during the prior month; higher scores indicate more perceived stress.
Neuroimaging
MRI-eligible participants underwent scanning at baseline (pre-intervention) and the 3-month follow up (post-intervention). High-resolution T1-weighted (multi-echo MPRAGE) and T2-weighted MR images and resting-state BOLD images were collected using a Prisma-fit system (Siemens, Erlangen, Germany) with a 32-channel head coil. Multi-echo MPRAGE scans were acquired with the following parameters: isotropic 0.8-mm3 voxels; 208 slices; TR: 2500 ms; TE: 1.81, 3.6, 5.39, and 7.18 ms; TI: 1000 ms; FOV: 256 mm; matrix size: 256×256 mm; and flip angle: 8 degrees. T2-weighted scans were acquired with the following parameters: isotropic 0.8-mm3 voxels; 208 slices; TR: 3200 ms; TE: 564 ms; FOV: 256 mm; matrix size: 256×256 mm; and flip angle: 120 degrees. Resting-state scans (eyes open, with a fixation cross) were collected in both anterior-posterior and posterior-anterior directions (6.4 min each; total scan time, 12.8 min) with the following parameters: isotropic 2.0-mm3 voxels; 72 slices; TR: 800 ms; TE: 37.0 ms; FOV: 208 mm; matrix size: 208×208 mm; and flip angle: 52 degrees. Fieldmaps were acquired in both anterior-posterior and posterior-anterior directions before resting-state scans.
Imaging data were pre-processed with the minimal Human Connectome Project (HCP) pipeline, including structural preprocessing of the T1-weighted and T2-weighted images by PreFreeSurfer, FreeSurfer, and PostFreeSurfer and functional preprocessing of the BOLD images by fMRIVolume and fMRISurface, followed by denoising using multi-run ICA-FIX [35–39].
Denoised and normalized resting-state images were parcellated according to the Cole-Anticevic Brain Network Parcellation (CAB-NP) atlas [40]. This atlas extends the HCPMMP atlas by including a fine-grained parcellation of subcortical regions. The CAB-NP atlas comprises 360 cortical and 358 subcortical parcels, with each parcel assigned to one of 12 major networks (specifically, the default mode network [DMN], auditory network [AN], frontoparietal network [FPN], language network, dorsal attention network, cingulo-opercular network, somatomotor network [SMN], posterior multimodal network [PMM], ventral multimodal network, primary visual network, and secondary visual network) [40]. The CAB-NP atlas includes 29 hippocampal parcels (17 and 12 in the left and right hemispheres, respectively), with 4 assigned to the DMN, 6 each assigned to the AN and SMN, 1 assigned to the FPN, 2 each assigned to the ventral multimodal and primary visual networks, and 8 assigned to the secondary visual network, which is consistent with the many roles of the hippocampus in learning and memory, spatial navigation, sensory integration, and emotion processing. For each participant, normalized (Fisher’s z-transformed) pairwise correlations between each hippocampal parcel timeseries and the timeserieses of all remaining parcels were computed, creating 29 hippocampal subregion connectivity maps for each scan (pre- and post-intervention). The change in connectivity for each hippocampal subregion was calculated by subtracting the post-intervention map from pre-the intervention map.
Statistical analysis
Group differences in demographic and baseline clinical characteristics were evaluated using the non-parametric Kruskal-Wallis test for continuous variables and Fisher’s exact test for categorical variables. Statistical analyses were performed using SPSS version 26 (IBM Corp. Albany, NY).
Group differences in hippocampal subregion connectivity changes with intervention were evaluated by non-rotated partial least squares analysis [41], implemented in Matlab using plscmd scripts, with and boot-strap estimation (5000 samples) (https://www.rotman-baycrest.on.ca). p-values <0.001 were considered significant.
To aid in the interpretation of the group difference, relationships between post-intervention hippocampal subregion connectivity and the MFQ1 and PSS were tested by Spearman partial correlational analysis across both groups (controlling for age) for those connections showing significance on the above-mentioned partial least squares analysis. As one participant in the yoga group had missing MFQ/PSS post-intervention data, the correlational analysis was performed on 21 participants. p-values <0.05 were considered significant.
Table 2
Change in clinical scores
Yoga | MET | p for group difference | |||
Change | p | Change | p | ||
HAMA | –3.10 (2.89) | 0.02 | 0.0 (5.88) | 0.99 | 0.06 |
BDI | –3.40 (4.12) | 0.02 | –1.27 (5.44) | 0.60 | 0.20 |
MFQ1 | 0.73 (1.02) | 0.06 | 0.003 (0.39) | 0.98 | 0.20 |
MFQ2 | 0.51 (1.53) | 0.32 | –0.47 (1.08) | 0.52 | 0.12 |
PSS | 1.20 (9.74) | 1.0 | 0.27 (4.92) | 0.71 | 0.67 |
HAMA, Hamilton Anxiety Rating Scale; BDI, Beck Depression Inventory; MET, memory enhancement training; MFQ1 Memory Function Questionnaire Factor 1 (frequency of forgetting); MFQ2, Memory Function Questionnaire Factor 2 (seriousness of forgetting); PSS, Perceived Stress Scale.
RESULTS
Demographic and clinical data
Baseline demographics and clinical data are summarized in Table 1. There were no significant differences between groups in baseline demographic and clinical characteristics. Additionally, there was no significant difference between groups in homework compliance (average number of days per week). As previously reported, while none of the changes in the clinical scores (BDI, HAMA, PSS, and MFQ1 MFQ2) were significantly different between groups, anxiety and depression scores improved significantly in the yoga group, but not in the MET group; changes in other scores did not reach significance (Table 2) [19].
Hippocampal subregion connectivity changes
Four hippocampal subregions showed significant differences in connectivity changes between the two groups (p < 0.001) (Fig. 2b). Specifically, an anterior hippocampal subregion assigned to the DMN (centered at – 10 – 8 – 21) showed significantly greater connectivity increases with 12 brain regions, including visual system regions (right V2, V8, fusiform face complex, and V4t) and a posterior hippocampal subregion (centered at – 23 – 40 0), in the yoga group than in the MET group (p < 0.001; Fig. 3a).
In addition, one posterior hippocampal subregion assigned to the AN (centered at – 25 – 32 – 12) and two posterior hippocampal assigned to the SMN (centered at – 23 – 40 0 and 21 – 40 2) showed significantly greater connectivity increases with 13, 28, and 49 brain regions, respectively, in the MET group than in the yoga group (p < 0.001; Fig. 3b). These regions were concentrated in the DMN and FPN.
Fig. 2
Hippocampal subregions. a) Hippocampal parcellations in the CAB-NP atlas color-coded according to assigned network (note: not all parcellations are visible). b) Location of hippocampal subregions with significance in the connectivity analysis. Red, default mode network; magenta, auditory network; cyan, somatomotor network; blue, primary visual network; CAB-NP, Cole-Anticevic brain network parcellation.
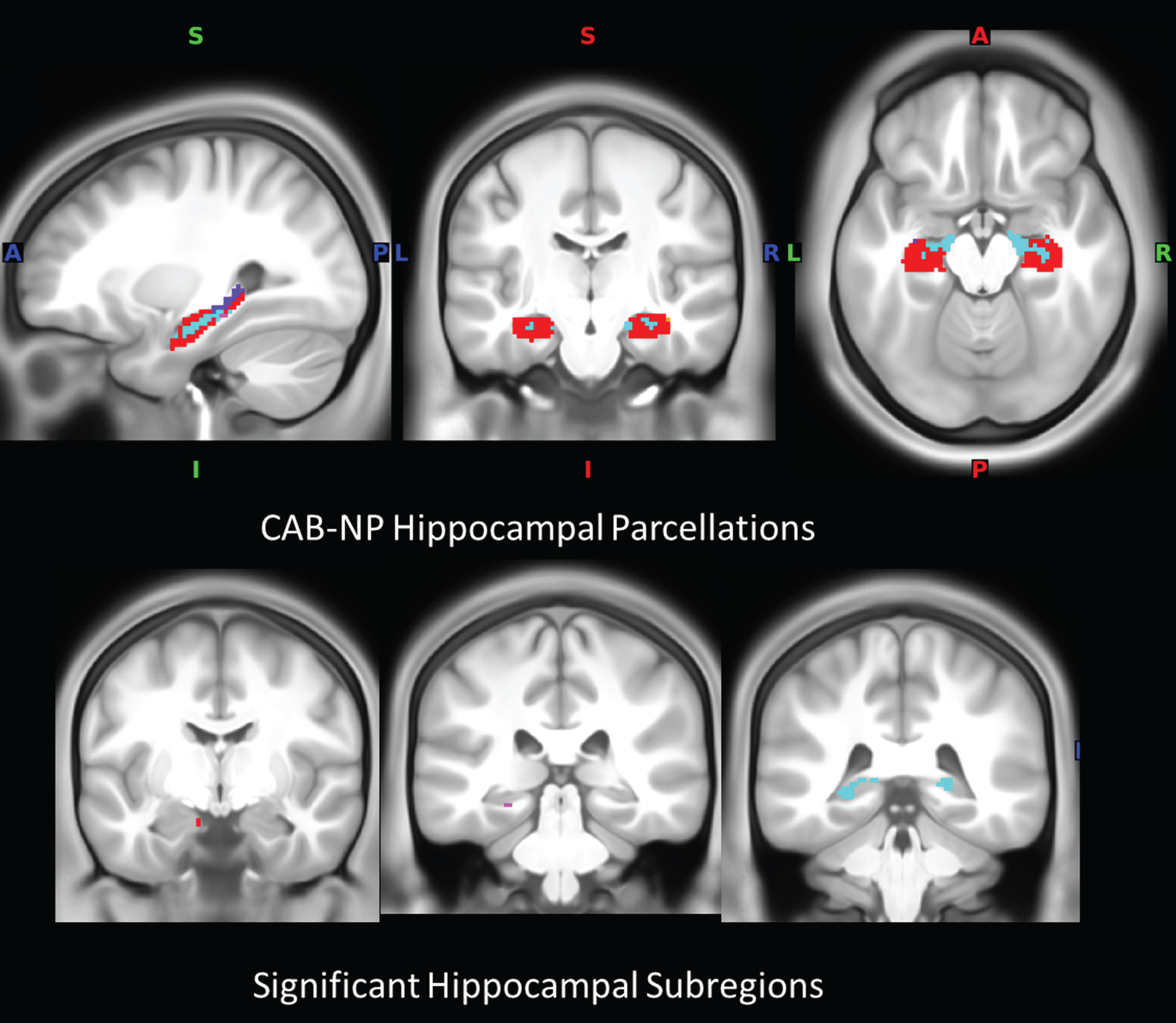
Associations between post-intervention hippocampal subregion connectivity and perceived stress/memory functioning
Among the 12 connections showing greater increases in the yoga group than in the MET group, 3 (involving connectivity between the left anterior DMN hippocampal subregion and the right V8, medial temporal area, and V4t) were significantly and negatively correlated with the post-intervention PSS score (p < 0.05; Fig. 2a) There were no significant correlations with the post-intervention MFQ1 score.
Among the 90 connections showing greater increases in the MET group than in the yoga group, 15 were significantly and positively correlated with the post-intervention MFQ1 score (i.e., better functioning in terms of the frequency of forgetting), mainly involving connectivity between the right posterior hippocampal subregion assigned to the SMN and regions in the DMN (p < 0.05; Fig. 2b). There were no significant correlations with the post-intervention PSS score.
DISCUSSION
The present study found differential changes in the resting-state connectivity of hippocampal subregions under two training conditions, one directly targeting memory strategies (MET) and one combining yoga classes and at-home yogic meditation (KY+KK), in women with subjective memory decline and cardiovascular risk factors. A fine-grained analysis was performed, using 29 hippocampal subregions assigned to 7 major resting-state networks in the CAB-NP atlas. While the yoga group showed greater connectivity increases involving an anterior hippocampal subregion assigned to the DMN, the MET group showed greater widespread connectivity increases involving posterior hippocampal subregions assigned to sensory-based networks; specifically, the SMN and AN. Further, connections more robustly impacted by yoga than by MET showed associations with lower perceived stress; specifically, greater connectivity between the anterior hippocampal subregion assigned to the DMN and regions in the visual system was associated with lower PSS scores. In contrast, connections more robustly impacted by MET than by yoga showed associations with better memory functioning; specifically, greater connectivity between the right posterior hippocampal subregion assigned to the SMN and regions mainly in the DMN was associated with higher MFQ1 scores (indicating less frequency of forgetting). These results partially support our hypothesis of greater hippocampal increases with memory-related regions with yoga than with MET, as discussed below.
Fig. 3
Hippocampal subregion connectivity differentially impacted by KY+KK and MET intervention, and behavioral relationships. a) Hippocampal subregion connectivity showing a greater increase with KY+KK than with MET. b) Hippocampal subregion connectivity showing a greater increase with MET than with KY+KK. Lines between regions indicate a significant group difference in the change in connectivity (post-invervention – pre-intervention). Colored lines represent connections with a significant relationship to behavioral scores across both groups (dark purple, PSS; dark green, MFQ1). Hippocampal subregions are indicated by a black band in the ideogram, and the CAB-NP atlas name is shown for all significant connections. Significant connections are also listed in Supplementary Table 1. V1, primary visual; V2, secondary visual; CO, cingulo-opercular; DMN, default mode network; FPN, frontoparietal network; PMM, posterior multimodal; SMN, somatosensory network; DAN, dorsal attention network; OA, orbito-affective; VMM, ventral multimodal; LN, language network; AN, auditory network; CAB-NP, Cole-Anticevic brain network parcellation; KY+KK, Kundalini yoga combined with Kirtan Kriya; MFQ1, Memory Function Questionnaire Factor 1; PSS, Perceived Stress Scale.
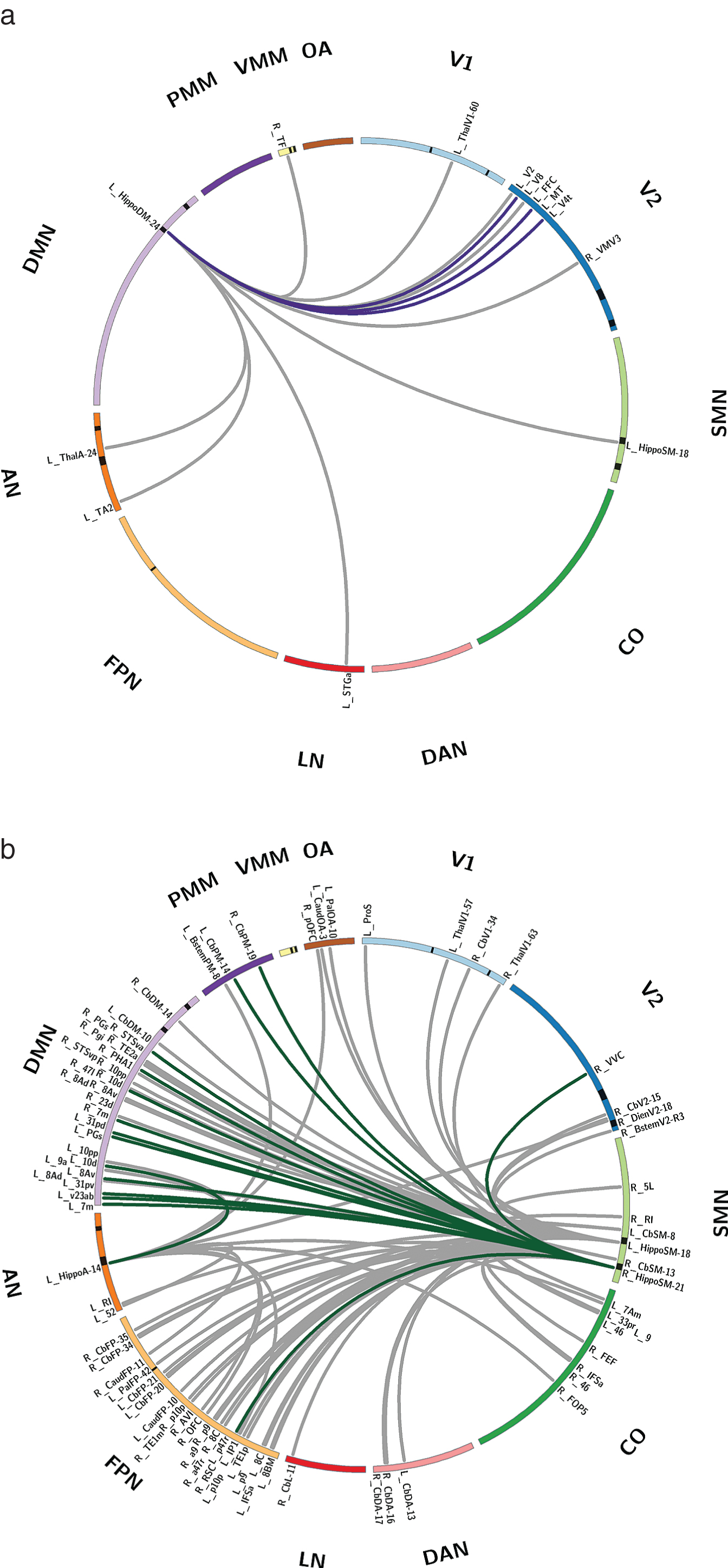
Although the DMN is best known as involved in self-referential processing, including autobiographical processes, it is associated many functions such as semantic processing, mental simulation and future planning, and social cognition [42–46]. The core brain regions of the DMN traditionally comprise the medial prefrontal, posterior cingulate, and inferior parietal cortices, and hippocampus [47]. However, the CAP-NB atlas refines the subcortical contribution to the DMN, as well as other resting-state networks, based on multimodal information, assigning 4 of 29 hippocampal subregions to the DMN [40]. The DMN and hippocampus have both been implicated in cognitive decline and AD. For example, previous studies have shown that the severity of subjective memory AD progression severity is associated with decreased DMN functional connectivity, which may be preceded by reduced signal complexity in the DMN, especially in the hippocampus [13, 48–50]. Additionally, hippocampal volume reduction and hypoconnectivity are associated with subjective memory decline and AD [13, 14]. The two interventions may differentially address this hypoconnectivity and the course of memory decline.
KY+KK engages different senses simultaneously (visualization, vocalization, motor, and sensory stimulation), and the chanting component may modulate respiratory muscles, lung volume, and cardiovascular and autonomic nervous system functions [51]. Further, KK has been shown to improve perceived stress [24]. Consistent with this, KY+KK training preferentially increased connectivity of the anterior hippocampal subregion assigned to the DMN with visual system regions, including several stress-sensitive connections. Stress, particularly persistent stress, is known to impair hippocampal-dependent memory and spatial navigation functioning [52] and alters the functional modular organization of the brain towards less coupling between the hippocampus and visual (occipital) regions [53]. Additionally, decoupling between the DMN and visual system is associated with mind-wandering, which interferes with reading comprehension and memory for text [54, 55]. Further, hippocampal-fusiform coupling is important to memory consolidation of faces and recall performance [56, 57]. Thus, KY+KK training may better target hippocampal subregion connectivity impacted by stress, which may aid in processing information, including facial information, into memory. However, at the group level, there was no significant net change in the PSS score (Table 2). KY+KK may improve stress-impacted hippocampal connectivity in a subset of women with subjective memory decline and cardiovascular risk factors. Further studies with larger sample sizes are needed to characterize those who would benefit most from KY+KK.
KY+KK training also impacted anterior-posterior hippocampal subregion connectivity to a greater extent than MET. In a previous imaging study, lower resting-state connectivity between the anterior and posterior hippocampi was associated with a decline in episodic memory function over a period of 2 years [58]. As the anterior and posterior hippocampi differentially contribute to complementary memory functions (the anterior hippocampus is more related to encoding, response to novelty, and global information, while the posterior hippocampus is more related to retrieval and detailed information), greater connectivity between anterior and posterior hippocampi may create a more integrated representation of an event, improving memory for the event [58–61]. Thus, the observed greater increased connectivity between anterior and posterior hippocampal subregions with KY+KK training than with MET may suggest superior long-term neuroprotective benefits in terms of vulnerable hippocampal connections critical to episodic memory with KY+KK training. Further studies with longer follow up are needed.
The MET protocol was derived from evidence-based techniques that use verbal and visual association, as well as practical strategies for memory learning [62, 63]. We found more widespread connectivity increases with MET than with KY+KK training, which involved hippocampal subregions assigned to sensory-based networks (i.e., AN and SMN). The hippocampus must integrate information from different sensory modalities to adapt to the changing environment, as a component in its role in learning and memory [64]. The various mnemonic strategies in MET, including verbal, visual, and spatial associative techniques, generally aim to enhance multimodal sensory integration into memory processes. Thus, MET may show superiority to KY+KK in terms of hippocampal sensory-integration important to memory. Consistent with this, increased connectivity between the right posterior hippocampal subregion assigned to the SMN and 15 regions in the DMN, PMM, and FPN (involved in working memory and other cognitive functions) was significantly correlated with improvement in the MFQ1 score (i.e., reduction in the frequency of forgetting). However, at the group level, there was no significant net change in the MFQ1 score (Table 2). MET may improve hippocampal connectivity along with memory function in a subset of women with subjective memory decline and cardiovascular risk factors. Further studies with larger sample sizes are needed to characterize those who would benefit most from MET.
The present study has several limitations to acknowledge. The sample size was small because some patients who enrolled in the RCT were unable to participate in the neuroimaging component due to contraindications (e.g., implants); additionally, some participants dropped out of the parent study and did not complete follow-up scanning. While we used non-parametric statistical methods that are robust to smaller sample sizes, additional effects may have been masked by these sample-size limitations. Additionally, as a non-treatment/waiting-list group was not included in the study design, whether the observed connectivity changes differ from the naturalistic course is unclear. Future large-scale studies that include a placebo group/control arm are necessary to further clarify the beneficial effects of KY+KK and MET on hippocampal connectivity and memory.
Conclusions
KY+KK training may better target hippocampal subregion connectivity impacted by stress and associated with long-term episodic memory decline, whereas MET may better target hippocampal sensory-integration supporting better memory reliability, in women with subjective memory decline and cardiovascular risk factors. These results add to the literature on the benefits of yoga for brain health and further indicate yoga as a promising practice for older women with increased AD risk, especially those with greater perceived stress.
ACKNOWLEDGMENTS
The authors have no acknowledgments to report.
FUNDING
This work was funded by the Alzheimer’s Research and Prevention Foundation and partially by NIH grant AT009198 to HL, and further supported by the National Center for Advancing Translational Science (NCATS) UCLA CTSI Grant Number UL1TR001881.
CONFLICT OF INTEREST
Dharma S. Khalsa is an Editorial Board Member of this journal but was not involved in the peer-review process nor had access to any information regarding its peer-review.
All other authors have no conflict of interest to report.
DATA AVAILABILITY
The data supporting the findings of this study are available within the article and/or its supplementary material.
SUPPLEMENTARY MATERIAL
[1] The supplementary material is available in the electronic version of this article: https://dx.doi.org/10.3233/JAD-221159.
REFERENCES
[1] | Reiman EM , Langbaum JB , Tariot PN ((2010) ) Alzheimer’s prevention initiative: A proposal to evaluate presymptomatic treatments as quickly as possible. Biomark Med 4: , 3–14. |
[2] | Wisniewski T , Drummond E ((2020) ) APOE-amyloid interaction: Therapeutic targets. Neurobiol Dis 138: , 104784. |
[3] | Crous-Bou M , Minguillon C , Gramunt N , Molinuevo JL ((2017) ) Alzheimer’s disease prevention: From risk factors to early intervention. Alzheimers Res Ther 9: , 71. |
[4] | Snyder HM , Shineman DW , Friedman LG , Hendrix JA , Khachaturian A , Le Guillou I , Pickett J , Refolo L , Sancho RM , Ridley SH ((2016) ) Guidelines to improve animal study design and reproducibility for Alzheimer’s disease and related dementias: For funders and researchers. Alzheimers Dement 12: , 1177–1185. |
[5] | Ferretti MT , Iulita MF , Cavedo E , Chiesa PA , Schumacher Dimech A , Santuccione Chadha A , Baracchi F , Girouard H , Misoch S , Giacobini E , Depypere H , Hampel H , Women’s Brain P , the Alzheimer Precision Medicine I ((2018) ) Sex differences in Alzheimer disease - the gateway to precision medicine. Nat Rev Neurol 14: , 457–469. |
[6] | Qu Y , Hu HY , Ou YN , Shen XN , Xu W , Wang ZT , Dong Q , Tan L , Yu JT ((2020) ) Association of body mass index with risk of cognitive impairment and dementia: A systematic review and meta-analysis of prospective studies. Neurosci Biobehav Rev 115: , 189–198. |
[7] | Lin Y , Shan PY , Jiang WJ , Sheng C , Ma L ((2019) ) Subjective cognitive decline: Preclinical manifestation of Alzheimer’s disease. Neurol Sci 40: , 41–49. |
[8] | Ou YN , Tan CC , Shen XN , Xu W , Hou XH , Dong Q , Tan L , Yu JT ((2020) ) Blood pressure and risks of cognitive impairment and dementia: A systematic review and meta-analysis of 209 prospective studies. Hypertension 76: , 217–225. |
[9] | Snyder HM , Asthana S , Bain L , Brinton R , Craft S , Dubal DB , Espeland MA , Gatz M , Mielke MM , Raber J , Rapp PR , Yaffe K , Carrillo MC ((2016) ) Sex biology contributions to vulnerability to Alzheimer’s disease: A think tank convened by the Women’s Alzheimer’s Research Initiative. Alzheimers Dement 12: , 1186–1196. |
[10] | Cramer H , Lauche R , Haller H , Steckhan N , Michalsen A , Dobos G ((2014) ) Effects of yoga on cardiovascular disease risk factors: A systematic review and meta-analysis. Int J Cardiol 173: , 170–183. |
[11] | Fan JT , Chen KM ((2011) ) Using silver yoga exercises to promote physical and mental health of elders with dementia in long-term care facilities. Int Psychogeriatr 23: , 1222–1230. |
[12] | Sovova E , Cajka V , Pastucha D , Malincikova J , Radova L , Sovova M ((2015) ) Positive effect of yoga on cardiorespiratory fitness: A pilot study. Int J Yoga 8: , 134–138. |
[13] | Zajac L , Koo BB , Tripodis Y , Mian A , Steinberg E , Mez J , Alosco ML , Cervantes-Arslanian A , Stern R , Killiany R ((2020) ) Hippocampal resting-state functional connectivity patterns are more closely associated with severity of subjective memory decline than whole hippocampal and subfield volumes. Cereb Cortex Commun 1: , tgaa019. |
[14] | Jack CR Jr , Knopman DS , Jagust WJ , Petersen RC , Weiner MW , Aisen PS , Shaw LM , Vemuri P , Wiste HJ , Weigand SD , Lesnick TG , Pankratz VS , Donohue MC , Trojanowski JQ ((2013) ) Tracking pathophysiological processes in Alzheimer’s disease: An updated hypothetical model of dynamic biomarkers. Lancet Neurol 12: , 207–216. |
[15] | Villemure C , Ceko M , Cotton VA , Bushnell MC ((2015) ) Neuroprotective effects of yoga practice: Age-, experience-, and frequency-dependent plasticity. Front Hum Neurosci 9: , 281. |
[16] | Gothe NP , Hayes JM , Temali C , Damoiseaux JS ((2018) ) Differences in brain structure and function among yoga practitioners and controls. Front Integr Neurosci 12: , 26. |
[17] | Froeliger B , Garland EL , McClernon FJ ((2012) ) Yoga meditation practitioners exhibit greater gray matter volume and fewer reported cognitive failures: Results of a preliminary voxel-based morphometric analysis. Evid Based Complement Alternat Med 2012: , 821307. |
[18] | Hariprasad VR , Varambally S , Shivakumar V , Kalmady SV , Venkatasubramanian G , Gangadhar BN ((2013) ) Yoga increases the volume of the hippocampus in elderly subjects. Indian J Psychiatry 55: , 394–396. |
[19] | Krause-Sorio B , Siddarth P , Kilpatrick L , Milillo MM , Aguilar-Faustino Y , Ercoli L , Narr KL , Khalsa DS , Lavretsky H ((2022) ) Yoga prevents gray matter atrophy in women at risk for Alzheimer’s disease: A randomized controlled trial. J Alzheimers Dis 87: , 569–581. |
[20] | Bhattacharyya KK , Andel R , Small BJ ((2021) ) Effects of yoga-related mind-body therapies on cognitive function in older adults: A systematic review with meta-analysis. Arch Gerontol Geriatr 93: , 104319. |
[21] | Brenes GA , Sohl S , Wells RE , Befus D , Campos CL , Danhauer SC ((2019) ) The effects of yoga on patients with mild cognitive impairment and dementia: A scoping review. Am J Geriatr Psychiatry 27: , 188–197. |
[22] | Hariprasad VR , Koparde V , Sivakumar PT , Varambally S , Thirthalli J , Varghese M , Basavaraddi IV , Gangadhar BN ((2013) ) Randomized clinical trial of yoga-based intervention in residents from elderly homes: Effects on cognitive function. Indian J Psychiatry 55: , S357–363. |
[23] | Chobe S , Chobe M , Metri K , Patra SK , Nagaratna R ((2020) ) Impact of Yoga on cognition and mental health among elderly: A systematic review. Complement Ther Med 52: , 102421. |
[24] | Innes KE , Selfe TK , Khalsa DS , Kandati S ((2016) ) Effects of meditation versus music listening on perceived stress, mood, sleep, and quality of life in adults with early memory loss: A pilot randomized controlled trial. J Alzheimers Dis 52: , 1277–1298. |
[25] | Eyre HA , Acevedo B , Yang H , Siddarth P , Van Dyk K , Ercoli L , Leaver AM , Cyr NS , Narr K , Baune BT , Khalsa DS , Lavretsky H ((2016) ) Changes in neural connectivity and memory following a yoga intervention for older adults: A pilot study. J Alzheimers Dis 52: , 673–684. |
[26] | Eyre HA , Siddarth P , Acevedo B , Van Dyk K , Paholpak P , Ercoli L , St Cyr N , Yang H , Khalsa DS , Lavretsky H ((2017) ) A randomized controlled trial of Kundalini yoga in mild cognitive impairment. Int Psychogeriatr 29: , 557–567. |
[27] | Yang H , Leaver AM , Siddarth P , Paholpak P , Ercoli L , St Cyr NM , Eyre HA , Narr KL , Khalsa DS , Lavretsky H ((2016) ) Neurochemical and neuroanatomical plasticity following memory training and yoga interventions in older adults with mild cognitive impairment. Front Aging Neurosci 8: , 277. |
[28] | Seok JW , Cheong C ((2020) ) Functional dissociation of hippocampal subregions corresponding to memory types and stages. J Physiol Anthropol 39: , 15. |
[29] | McCarty DL ((1980) ) Investigation of a visual imagery mnemonic device for acquiring face–name associations. J Exp Psychol Hum Learn 6: , 145–155. |
[30] | Cohen S , Kamarck T , Mermelstein R ((1983) ) A global measure of perceived stress. J Health Soc Behav 24: , 385–396. |
[31] | Gilewski MJ , Zelinski EM , Schaie KW ((1990) ) The Memory Functioning Questionnaire for assessment of memory complaints in adulthood and old age. Psychol Aging 5: , 482–490. |
[32] | Hamilton M ((1959) ) The assessment of anxiety states by rating. Br J Med Psychol 32: , 50–55. |
[33] | Beck AT , Ward CH , Mendelson M , Mock J , Erbaugh J ((1961) ) An inventory for measuring depression. Arch Gen Psychiatry 4: , 561–571. |
[34] | Merrill DA , Siddarth P , Saito NY , Ercoli LM , Burggren AC , Kepe V , Lavretsky H , Miller KJ , Kim J , Huang SC , Bookheimer SY , Barrio JR , Small GW ((2012) ) Self-reported memory impairment and brain PET of amyloid and tau in middle-aged and older adults without dementia. Int Psychogeriatr 24: , 1076–1084. |
[35] | Glasser MF , Coalson TS , Bijsterbosch JD , Harrison SJ , Harms MP , Anticevic A , Van Essen DC , Smith SM ((2018) ) Using temporal ICA to selectively remove global noise while preserving global signal in functional MRI data. Neuroimage 181: , 692–717. |
[36] | Glasser MF , Coalson TS , Robinson EC , Hacker CD , Harwell J , Yacoub E , Ugurbil K , Andersson J , Beckmann CF , Jenkinson M , Smith SM , Van Essen DC ((2016) ) A multi-modal parcellation of human cerebral cortex. Nature 536: , 171–178. |
[37] | Glasser MF , Smith SM , Marcus DS , Andersson JL , Auerbach EJ , Behrens TE , Coalson TS , Harms MP , Jenkinson M , Moeller S , Robinson EC , Sotiropoulos SN , Xu J , Yacoub E , Ugurbil K , Van Essen DC ((2016) ) The Human Connectome Project’s neuroimaging approach. Nat Neurosci 19: , 1175–1187. |
[38] | Glasser MF , Sotiropoulos SN , Wilson JA , Coalson TS , Fischl B , Andersson JL , Xu J , Jbabdi S , Webster M , Polimeni JR , Van Essen DC , Jenkinson M , Consortium WU-MH ((2013) ) The minimal preprocessing pipelines for the Human Connectome Project. Neuroimage 80: , 105–124. |
[39] | Robinson EC , Garcia K , Glasser MF , Chen Z , Coalson TS , Makropoulos A , Bozek J , Wright R , Schuh A , Webster M , Hutter J , Price A , Cordero Grande L , Hughes E , Tusor N , Bayly PV , Van Essen DC , Smith SM , Edwards AD , Hajnal J , Jenkinson M , Glocker B , Rueckert D ((2018) ) Multimodal surface matching with higher-order smoothness constraints. Neuroimage 167: , 453–465. |
[40] | Ji JL , Spronk M , Kulkarni K , Repovs G , Anticevic A , Cole MW ((2019) ) Mapping the human brain’s cortical-subcortical functional network organization. Neuroimage 185: , 35–57. |
[41] | McIntosh AR , Lobaugh NJ ((2004) ) Partial least squares analysis of neuroimaging data: Applications and advances.S. Neuroimage 23: (Suppl 1), 250–263. |
[42] | Mancuso L , Cavuoti-Cabanillas S , Liloia D , Manuello J , Buzi G , Cauda F , Costa T ((2022) ) Tasks activating the default mode network map multiple functional systems. Brain Struct Funct 227: , 1711–1734. |
[43] | Gerlach KD , Spreng RN , Gilmore AW , Schacter DL ((2011) ) Solving future problems: Default network and executive activity associated with goal-directed mental simulations. Neuroimage 55: , 1816–1824. |
[44] | Lanzoni L , Ravasio D , Thompson H , Vatansever D , Margulies D , Smallwood J , Jefferies E ((2020) ) The role of default mode network in semantic cue integration. Neuroimage 219: , 117019. |
[45] | Mwilambwe-Tshilobo L , Spreng RN ((2021) ) Social exclusion reliably engages the default network: A meta-analysis of Cyberball. Neuroimage 227: , 117666. |
[46] | Yeshurun Y , Nguyen M , Hasson U ((2021) ) The default mode network: Where the idiosyncratic self meets the shared social world. Nat Rev Neurosci 22: , 181–192. |
[47] | Buckner RL , Andrews-Hanna JR , Schacter DL ((2008) ) The brain’s default network: Anatomy, function, and relevance to disease. Ann N Y Acad Sci 1124: , 1–38. |
[48] | Grieder M , Wang DJJ , Dierks T , Wahlund LO , Jann K ((2018) ) Default Mode Network Complexity and Cognitive Decline in Mild Alzheimer’s Disease. Front Neurosci 12: , 770. |
[49] | Zhang HY , Wang SJ , Liu B , Ma ZL , Yang M , Zhang ZJ , Teng GJ ((2010) ) Resting brain connectivity: Changes during the progress of Alzheimer disease. Radiology 256: , 598–606. |
[50] | Greicius MD , Krasnow B , Reiss AL , Menon V ((2003) ) Functional connectivity in the resting brain: A network analysis of the default mode hyothesis. Proc Natl Acad Sci U S A 100: , 253–258. |
[51] | Kang J , Scholp A , Jiang JJ ((2018) ) A Review of the Physiological Effects and Mechanisms of Singing. J Voice 32: , 390–395. |
[52] | Kim EJ , Pellman B , Kim JJ ((2015) ) Stress effects on the hippocampus: A critical review. Learn Mem 22: , 411–416. |
[53] | Zhang Y , Dai Z , Hu J , Qin S , Yu R , Sun Y ((2020) ) Stress-induced changes in modular organizations of human brain functional networks. Neurobiol Stress 13: , 100231. |
[54] | Zhang M , Bernhardt BC , Wang X , Varga D , Krieger-Redwood K , Royer J , Rodriguez-Cruces R , Vos de Wael R , Margulies DS , Smallwood J , Jefferies E ((2022) ) Perceptual coupling and decoupling of the default mode network during mind-wandering and reading. Elife 11: . |
[55] | Zhang M , Savill N , Margulies DS , Smallwood J , Jefferies E ((2019) ) Distinct individual differences in default mode network connectivity relate to off-task thought and text memory during reading. Sci Rep 9: , 16220. |
[56] | Geiger MJ , O’Gorman Tuura R , Klaver P ((2016) ) Inter-hemispheric connectivity in the fusiform gyrus supports memory consolidation for faces. Eur J Neurosci 43: , 1137–1145. |
[57] | Dickerson BC , Miller SL , Greve DN , Dale AM , Albert MS , Schacter DL , Sperling RA ((2007) ) Prefrontal-hippocampal-fusiform activity during encoding predicts intraindividual differences in free recall ability: An event-related functional-anatomic MRI study. Hippocampus 17: , 1060–1070. |
[58] | Oren N , Ash EL , Shapira-Lichter I , Elkana O , Reichman-Eisikovits O , Chomsky L , Lerner Y ((2019) ) Changes in resting-state functional connectivity of the hippocampus following cognitive effort predict memory decline at older age-A longitudinal fMRI study. Front Aging Neurosci 11: , 163. |
[59] | Poppenk J , Evensmoen HR , Moscovitch M , Nadel L ((2013) ) Long-axis specialization of the human hippocampus. Trends Cogn Sci 17: , 230–240. |
[60] | Strange BA , Witter MP , Lein ES , Moser EI ((2014) ) Functional organization of the hippocampal longitudinal axis. Nat Rev Neurosci 15: , 655–669. |
[61] | Moscovitch M , Cabeza R , Winocur G , Nadel L ((2016) ) Episodic memory and beyond: The hippocampus and neocortex in transformation. Annu Rev Psychol 67: , 105–134. |
[62] | Verhaeghen P , Marcoen A , Goossens L ((1992) ) Improving memory performance in the aged through mnemonic training: A meta-analytic study. Psychol Aging 7: , 242–251. |
[63] | Gross AL , Rebok GW ((2011) ) Memory training and strategy use in older adults: Results from the ACTIVE study. Psychol Aging 26: , 503–517. |
[64] | Del Rio-Bermudez C , Blumberg MS ((2022) ) Sleep as a window on the sensorimotor foundations of the developing hippocampus. Hippocampus 32: , 89–97. |