Yoga Prevents Gray Matter Atrophy in Women at Risk for Alzheimer’s Disease: A Randomized Controlled Trial
Abstract
Background:
Female sex, subjective cognitive decline (SCD), and cardiovascular risk factors (CVRFs) are known risk factors for developing Alzheimer’s disease (AD). We previously demonstrated that yoga improved depression, resilience, memory and executive functions, increased hippocampal choline concentrations, and modulated brain connectivity in older adults with mild cognitive impairment.
Objective:
In this study (NCT03503669), we investigated brain gray matter volume (GMV) changes in older women with SCD and CVRFs following three months of yoga compared to memory enhancement training (MET).
Methods:
Eleven women (mean age = 61.45, SD = 6.58) with CVRF and SCD completed twelve weeks of Kundalini Yoga and Kirtan Kriya (KY + KK) while eleven women (mean age = 64.55, SD = 6.41) underwent MET. Anxiety, resilience, stress, and depression were assessed at baseline and 12 weeks, as were T1-weighted MRI scans (Siemens 3T Prisma scanner). We used Freesurfer 6.0 and tested group differences in GMV change, applying Monte-Carlo simulations with alpha = 0.05. Region-of-interest analysis was performed for hippocampus and amygdala.
Results:
Compared to KY + KK, MET showed reductions in GMV in left prefrontal, pre- and post-central, supramarginal, superior temporal and pericalcarine cortices, right paracentral, postcentral, superior and inferior parietal cortices, the banks of the superior temporal sulcus, and the pars opercularis. Right hippocampal volume increased after yoga but did not survive corrections.
Conclusion:
Yoga training may offer neuroprotective effects compared to MET in preventing neurodegenerative changes and cognitive decline, even over short time intervals. Future analyses will address changes in functional connectivity in both groups.
INTRODUCTION
With the population aging rapidly, the global prevalence of dementia is expected to double every twenty years, with an estimated 35.6 million in 2010, 65.7 million in 2030 and a rapid rise to 115.4 million in 2050 [1]. Alzheimer’s disease (AD) is an irreversible neurodegenerative disease, and therefore, early detection and preventive intervention are critical to delaying the onset of symptoms [2]. While the AD risk gene APOE E4 is hardwired and unchangeable, focusing on modifiable risk factors promises to delay AD onset [3, 4]. Female sex, cardiovascular risk factors (CVRFs), and subjective cognitive decline (SCD) are known risk factors for developing AD [5–10]. CVRFs include older age, high blood pressure or hypertension, diabetes, high cholesterol, obesity, and a family history of cardiovascular disease [5–10]. While some of these CVRFs are modifiable (e.g., body weight, cholesterol, high blood pressure), others are not (sex, age, family history). Early prevention techniques are most urgently needed in high-risk groups. It has been argued that while objectively measured cognitive impairment using standardized neuropsychological assessments is not detectable in preclinical stages of AD, subjective cognitive decline, and neuroimaging-based biomarkers are already detectable [8]. Brain gray matter volume (GMV) atrophy, particularly in the hippocampus, responsible for memory function, can be observed decades before the onset of cognitive problems, and can serve as an early biomarker for AD [11].
Yoga has documented beneficial effects on cardiovascular functioning and is a promising intervention for stress-reduction, i.e., yoga is ideal to improve some of the modifiable AD risk factors [12]. Yoga has recently been identified as a safe practice with positive effects on cognitive functions in healthy elderly [13], older adults with mild cognitive impairment (MCI), and those with early stages of dementia [14–16]. Yoga was found to improve both mental and physical health in older adults with dementia in long-term care facilities, including blood pressure, breathing rate, cardiorespiratory fitness, body flexibility, balance, joint movement and muscle strength, and endurance [17]. Reports in the general population also show that the motivations to practice yoga include the achievement of better wellbeing, disease prevention, the improvement of fitness, energy levels, physical and mental health, immune functioning, back pain, arthritis, anxiety, and depression [18, 19]. While yoga commonly involves postures (asanas), focus on breath (pranayama), postural alignment, and movement, brief meditative practices can be helpful as well. For instance, in SCD, a yogic meditation named Kirtan Kriya (KK) improved perceived stress, psychological well-being, quality of life, and mood compared to a music listening control [20]. Yoga can be used to improve stress, memory, brain health, and subjective wellbeing in high-risk groups, as well as in the general population.
Yoga demonstrated greater overall GMV in experienced yoga practitioners compared to non-practitioners, with more years of yoga experience being associated with greater GMV in left insula, frontal operculum, and orbitofrontal cortices [21]. In addition, the same study also found that hours of weekly practice were associated with GMV increases at the borders of primary somatosensory and superior parietal cortices, the precuneus and posterior cingulate junction, and in the hippocampus and primary visual cortex. The authors therefore suggested that yoga may have neuroprotective effects. Another study found that left hippocampal volume was greater in experienced yoga practitioners compared to age- and sex-matched yoga-naïve controls [22]. Greater GMV in frontal, limbic (including the hippocampus), temporal, occipital and cerebellar regions was also found in a group of yogic meditation practitioners compared to non-practitioner controls and was associated with cognitive performance [23]. Both cognitive performance and the number of years of past yoga practice were associated with greater GMV in various brain regions also in this study. In experienced yoga meditators, global GMV was greater compared to non-meditators, in addition to regional GMV in the right ventromedial orbitofrontal and ventrolateral prefrontal cortices, inferior temporal and parietal cortices, and bilateral insula cortex [24, 25]. It was notable that non-meditators did not display greater GMV compared to meditators in any brain region. Four weeks of Sahaja yoga meditation training led to increases in GMV in a small cluster in the right inferior frontal gyrus, as well as improved well-being, fatigue and dissatisfaction compared to a waitlist control group [26]. A small uncontrolled six-month yoga trial in a group of seven older healthy volunteers reported increases in hippocampal volume, but not a control region in the occipital cortex [27].
Our own studies have previously demonstrated beneficial effects of Kundalini Yoga (KY) paired with a KK (KY + KK) homework practice on cognition, resilience, neurochemistry, and neuroplasticity in a randomized controlled trial (RCT) in older adults with MCI [28–30]. Specifically, KY + KK improved depression, resilience, and executive functions, and prevented decline in hippocampal choline concentrations [28, 30]. Since then, multiple meta-analyses have confirmed that mind-body interventions can effectively enhance various cognitive domains and resilience in healthy older adults and those with MCI [31–33]. It is important to note that existing studies reporting GMV effects with yoga are based on small samples and often specific and homogenous populations. More systematic and larger-scale studies are therefore critical to further address potential neuroplastic effects over short periods of yoga training.
KY is a form of yoga that combines movement and meditation with a greater focus on breathing intensive exercises and mental visualizations than other forms of yoga. Meditation practices can be silent or involve repetitive Sanskrit chants intended to bring peace to the mind. These meditative practices can additionally include mudras, continuous or changing positions of the hands and fingers. KY is likely to alleviate energetic blockages that may be equivalent to areas of chronic muscle tension, tightness or weakness, or any other physical discomforts. The increased intensity of breathing and mental engagement with less and more gentle movement makes this an ideal form of yoga to practice with older people, who often have mobility problems. While the evidence on the effect of yoga as a whole on cardiovascular health is abundant, the type of yoga, the duration and the control interventions vary greatly, according to a recent meta-analysis [12]. It was reported that half the included research articles did not report what form of yoga was used and only one study reported using KY. It is therefore critical to delineate the format and consistency of our program, including daily homework exercises and to study its effects systematically. It is further notable, that existing KY + KK studies are underpowered and few in number. It is therefore critical to conduct larger-scale systematic clinical research in both healthy and at-risk populations.
To summarize, yoga practices offer a multitude of documented positive effects on mental and physical health, as well as neuroplastic effects in the brain in both healthy and cognitively impaired older adults. It has not been tested whether yoga can improve memory and biomarkers of brain aging in high-risk groups with SCD. We therefore investigated GMV, stress and memory changes in a group of older women with CVRFs and SCD undergoing cognitive assessments pre- and post-trial. Specifically, we tested group differences in GMV changes. As an additional exploratory analysis, we tested whether there were also group differences in change in anxiety, depression, resilience and memory.
METHODS
Screening
Between May 2018 and February 2021, 100 women were screened and randomized for the current subpart of the parent study (NCT03503669), as intended. Only 26 women (N = 13 in each group; mean age = 62.8 years, SD = 6.43) were eligible or willing to undergo an MRI scan at baseline, and 22 also completed a second scan after completion of the trial (N = 11 per group, see Fig. 1). The screening was performed by a trained staff member to assess physical and cognitive problems. Eligibility criteria were 1) SCD by report that included subjective complaints of decline in cognitive and memory function from the past level of functioning compared to the previous year; 2) the presence of one or more CVRFs, including a ≥7.5th percentile on the ASCVD risk calculator using the Cerebrovascular Risk Factor Prediction Chart and hematologic testing; 2.1) a history of myocardial infarction no less than 6 months prior; 2.2) a previous diagnosis of diabetes; 2.3) current pharmacological treatment for blood pressure (> 140/90); 2.4) current pharmacological treatment for hyperlipidemia (LDL > 160); 3) sufficient English proficiency to successfully comprehend the memory training; 4) sufficient mental capacity to provide informed consent. Exclusion criteria for the main trial included 1) a history of psychiatric conditions including psychosis, bipolar disorder, drug or alcohol dependence, or a neurological disorder; 2) surgery within the past three months or planned surgery within the next year, as well as unstable medical conditions; 3) disabilities, such as severe visual or hearing impairment that could prevent participation in the intervention; 4) insufficient English proficiency to comprehend the intervention instructions, materials and discussions; 5) a diagnosis of dementia (MMSE ≤23 or CDR ≥0.5, see below); 6) current participation in cognitive training in a therapeutic setting; 7) current treatment with psychoactive medication; 8) prior experience with KY or KK; 9) myocardial infarction within the past 6 months. Additionally, MRI exclusion criteria involved metallic implants, permanent makeup, tattoos in the head and neck region and claustrophobia. All participants provided written informed consent for participation in the trial as approved by the UCLA Institutional Review Board (IRB). This study was conducted in accordance with the Declaration of Helsinki of 1975.
Fig. 1
CONSORT diagram.
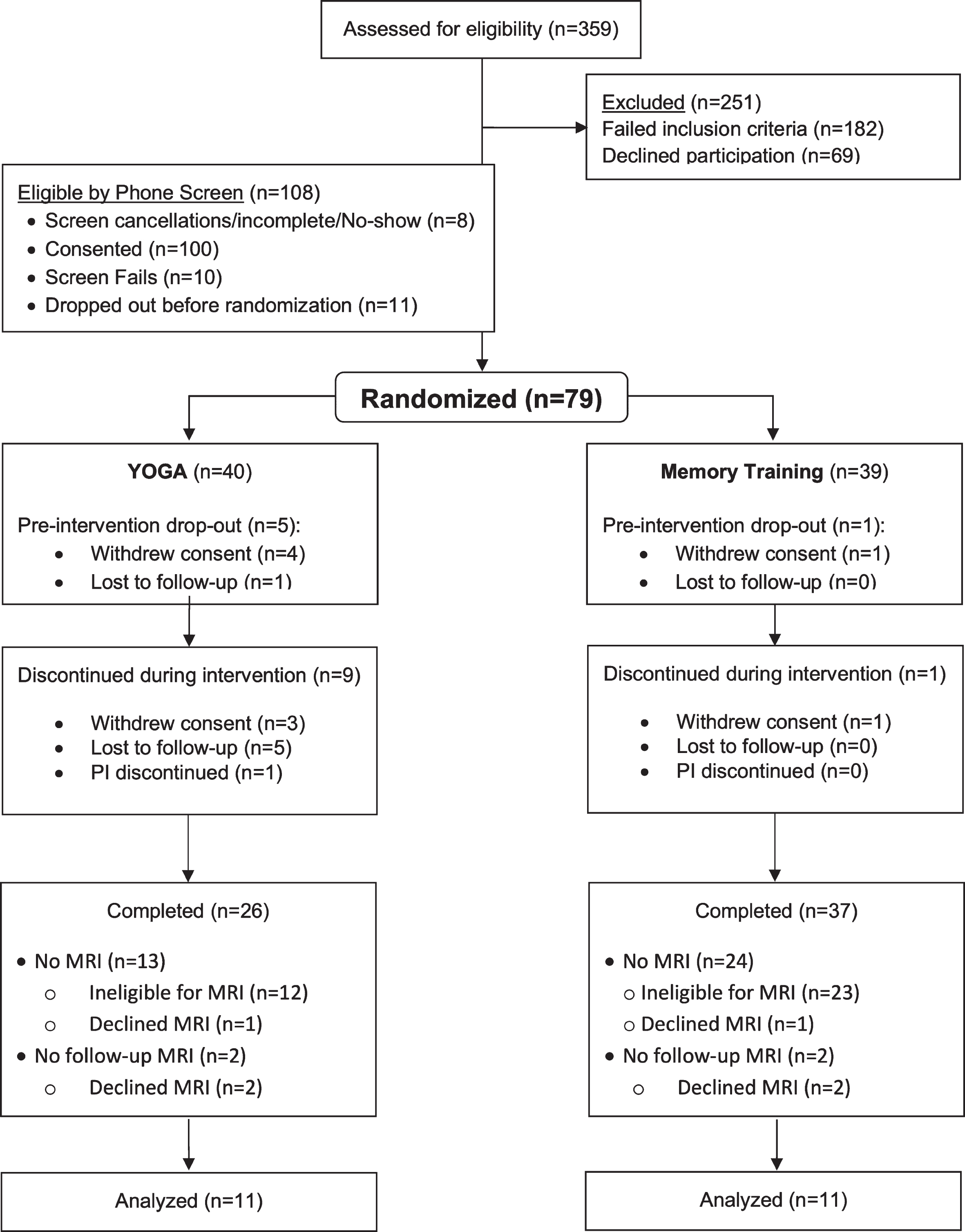
Cognitive assessment
Participants completed the Clinical Dementia Rating Scale (CDR) [34], the Mini-Mental State Examination (MMSE) [35], and the Memory Function Questionnaire (MFQ) [36]. The former two were exclusively used to screen out potential participants with AD. Two-factor scores were used in the analysis from this latter 64-item questionnaire: frequency of forgetting (MFQ1) and seriousness of forgetting (MFQ2). All tests were administered both at baseline and 12-week follow-up. SCD was defined as the subjective experience of declining memory function, despite a normal range of memory function using neuropsychological measures (for a more detailed discussion, see [37]). Note that the primary outcome variable of this study was the Hopkins Verbal Learning Test total recall score. However, the parent study tested this memory score at baseline and 6 months and therefore, there was no score available at the 3-month follow-up when we acquired MRI scans. The results will be reported in a separate report using the larger parent sample.
Clinical assessment
Anxiety, depression, stress, and resilience were selected as the main clinical outcomes for the current study and were measured at baseline and 12-week follow-up using the Beck Depression Inventory (BDI) [38], Hamilton Anxiety Scale (HAMA) [39], the Connor-Davidson Resilience Scale (CDRISC) [40], and the Perceived Stress Scale (PSS) [41]. Side effects and adverse events were monitored using the UKU Side Effect Rating Scale [42].
Yoga intervention
The KY + KK intervention consisted of weekly, 60-min in-person lessons with a certified KY instructor with 6–10 participants per class. Each class followed the same structure: 1) tuning in (5 min); 2) warm up (15 min); 3) breathing techniques “Pranayama” (15 min); 4) KK (12 min); 5) final resting pose “Savasana” (10 min) and closing (3 min). In addition, each participant received a CD containing a 12-min KK recording with gentle background music and guidance for the exercise sequence. Participants performed this exercise at home every day. They were instructed to chant along with their eyes closed in a seated position, the feet flat on the floor (i.e., relaxed with a straight spine), to visualize a beam of white light entering the center of the top of the head and exiting the middle of the forehead, which is spiritually considered the third eye. While chanting, the thumb of each hand would touch the other fingers sequentially (“mudras”) along with the words “Saa” (thumb touches second finger), “Taa” (middle finger), “Naa” (ring finger), and “Maa” (fifth finger). “Saa Taa Naa Maa” translates to “Birth, Life, Death, and Rebirth.” The first round is chanted out loud, the next round whispered, the third is thought silently, the fourth is also whispered, and the fifth round is chanted out loud again. This sequence is repeated for 11 min and closes with a last minute of energetic integration and meditation. This technique is thought to engage different senses simultaneously (visualization, vocalization, motor, and sensory stimulation). Furthermore, the chanting and breathing pattern modulate respiratory muscles, lung volume, cardiovascular and autonomic nervous system functions (for a narrative review on singing, see [43]).
Memory enhancement training (MET)
MET involved 12 weekly in-person group classes presented by a qualified memory training instructor. The classes aimed to teach memory strategies, while participants completed weekly homework assignments and handed them in to ascertain participant compliance. MET was developed by researchers at the UCLA Longevity Center. This MET program involves a scripted curriculum for the trainer and a companion workbook for each participant. The detailed standard protocol for MET was derived from evidence-based techniques that use verbal and visual association, as well as practical strategies for memory learning [44, 45]. MET is performed in small group sessions of 6–10 people and includes 1) education about memory; 2) introduction to memory strategies (described below); 3) instruction of the use of specific memory strategies; 4) home practice along with logs to track activity; 5) the discussion of non-cognitive factors, such as self-confidence, anxiety, and negative expectations. Each weekly session has the same structure; trainers 1) document the number of participants per session, engagement in alternative treatments, and collect homework completion logs; 2) review the previous homework exercises to reinforce learned techniques; 3) teach new techniques, review, and conduct exercises in the group session; and 4) assign new homework for the following week. Participants were directed to spend approximately 20 min daily on homework and document their activity in their logs. Each group session was devoted to learning and practicing memory techniques, and 15 min were reserved for reviewing the completed homework. Specific techniques taught include the following: verbal associative techniques (such as the use of stories) to remember lists; organizational strategies (categorizing items on a grocery list); visual associative strategies for learning faces and names (adapted from [46]); learning to implement memory habits to recall where the person placed an item, what recent activities they performed (e.g. locking doors, turning off appliances); and how they can remember future tasks (i.e. appointments).
Adherence and attendance
Staff members tracked the attendance of participants for their weekly in-person training classes. Each participant was allowed a maximum of two missed classes. Participants self-reported if they had completed their homework of KK or their memory training tasks. Completed homework sheets had to be handed in to a staff member each time the participants came to the lab for their classes or testing. Additionally, participants were asked not to participate in any other mind-body practices during the trial period, such as Tai Chi, Qi Gong, or yoga. This adherence was also monitored at each class session.
Neuroimaging protocol
A high-resolution structural T1-weighted image was collected for each participant using a 3T Sie-mens Prisma scanner (Siemens, Erlangen, Germany) with a 32-channel head coil at the UCLA Ahman-son & Lovelace Brain Mapping Center (ALBMC). This was part of a 90-min protocol that also included a T2 scan, fMRI scans for resting state and two memory tasks, diffusion-weighted imaging, single-voxel spectroscopy and a high-resolution scan of the hippocampus. Due to reasons of timing, comfort and technical difficulties, not all participants completed all scans. However, each participant who under-went MRI scanning, completed the T1 scan. Para-meters were as follows: a multi-echo MPRAGE scan 0.8 mm3 with isotropic voxel dimensions, 208 slices, TR = 2,500 ms, TE = 1.81, 3.6, 5.39 and 7.18 ms, TI = 1,000, FOV = 256 mm, matrix size =256×256 mm, and a flip angle = 8 degrees. We performed automatic cortical reconstruction using Freesurfer version 6.0 (https://surfer.nmr.mgh.harvard.edu/). Preprocessing included the correction of magnetic field inhomogeneities, the extraction of brain volume from other tissue types, the segmentation of subcortical gray matter and cortical surface parcellation according to the Desikan-Killany atlas [47]. Subsequently, subject-specific templates were created using the automated longitudinal reconstruction pipeline. Baseline and post-treatment images were then registered to each participant’s average template [48]. Resulting scans were carefully inspected by the same researcher for tissue misclassifications and misalignments between baseline and post-treatment scan. Corrections were performed manually. Cortical maps were smoothed using a Gaussian kernel of 10 mm full-width half-maximum. Subcortical volumes for the hippocampus and amygdala for each hemisphere were extracted for further analysis using statistical software (see Statistics).
Statistics
Baseline differences, as well as between-group differences in change in clinical scores and subcortical regions of interest (ROIs) (left and right amygdala and hippocampus) were tested using the Kruskal-Wallis test. Signed rank tests were used to examine within-group changes. The significance level for two-tailed testing was set to an alpha of 5%. Results for subcortical ROIs were corrected for multiple comparisons using the Benjamini-Hochberg procedure (with a false discovery rate of 10%). Q plots and Levene’s test were used to assess normality and heteroscedasticity. Because of the small sample size (n = 11 in each of the two groups), non-parametric tests were used for all analyses. We tested group differences in whole-brain symmetrized percent change (SPC), the change rate with respect to the average GMV [i.e., 100 * (annualized rate between time point 1 and time point 2)/average] GMV using the longitudinal volume-based two-stage voxel-wise general linear model using qdec (https://www.surfer.nmr.mgh.harvard.edu). SPC does not rely on the order of time points entered into the model and takes into account between-subject variation in inter-time point intervals [49]. Age was used as a covariate in the model. The voxel threshold and Monte-Carlo corrected cluster thresholds were set to p < 0.05. Additionally, we extracted GMV change values for each participant from the clusters and performed a post-hoc signed rank test (S-statistic) to test whether the change within each group was significantly different from zero. Age served as a covariate in this analysis as well. While the whole-brain model determined whether and where there were group differences in the brain changes, the signed rank test revealed which group contributed to the difference.
RESULTS
Clinical effects
The CONSORT diagram for the study is shown in Fig. 1. After screening 359 potential participants, 251 had to be excluded who either did not meet the inclusion criteria or declined to participate. Out of the remaining 108 participants who were screened over the phone, 79 were randomized to yoga or MET using a computer-generated assignment scheme, which assigns participants in a 1:1 ratio to each group. Twenty-six completed KY, while 37 completed MET. However, only 13 participants in each group were eligible or willing to also perform an MRI scan. Of the total of 26 participants tested at baseline, four declined to complete the second scan. The final sample consisted of 22 participants, 11 in each group. There were no significant differences in demographics, clinical or memory scores between groups at baseline (Table 1, Supplementary Table 1). None of the changes in the clinical scores (BDI, HAMA, CDRISC, PSS, and MFQ Factors 1 and 2) were significantly different between groups (Table 2). Anxiety and depression improved significantly within the yoga group but did not change in the MET group (Table 2). No side effects were reported.
Table 1
Participant demographics, clinical, and memory scores at baseline
Variable | Yoga | Memory training | Kruskal-Wallis test | ||
Median | Range | Median | Range | Statistic (p) | |
Demographics and clinical scores | |||||
Age | 62.0 | 53–69 | 64.0 | 54–76 | 1.26 (0.26) |
Education | 16.0 | 13–18 | 16.0 | 13–18 | 0.12 (0.73) |
Body mass index | 25.65 | 20.1–39.9 | 23.15 | 18.6–41.6 | 0.16 (0.69) |
Anxiety | 4.5 | 2–10 | 4.0 | 1–14 | 0.41 (0.52) |
Depression | 7.5 | 1–16 | 6.0 | 3–21 | < 0.0001 (1.0) |
Resilience | 70.0 | 54–90 | 70.0 | 50–98 | < 0.0001 (1.0) |
Perceived Stress | 22.0 | 20–30 | 22.0 | 15–26 | 0.1 (0.75) |
Memory Function Questionnaire | |||||
Frequency of Forgetting | 4.25 | 2.3–5.8 | 4.75 | 3.3–5.9 | 1.48 (0.22) |
Seriousness of Forgetting | 3.6 | 2.4–6.4 | 4.1 | 2.3–6.0 | 1.11 (0.29) |
Gray matter volume | |||||
Estimated total intracranial volume | 1491456.34 | 1055761.8–1708017.45 | 1456013.1 | 1055410.9–1673511.8 | 0.13 (0.72) |
Left hippocampus | 3538.70 | 3007.1–4253.2 | 3530.5 | 3248.6–4241.1 | 0.03 (0.87) |
Right hippocampus | 3800.4 | 3132.7–4443.0 | 3512.2 | 3303.0–4261.6 | 0.31 (0.58) |
Left amygdala | 1262.4 | 1007.1–1505.0 | 1310.9 | 1101.9–1412.0 | 0.79 (0.38) |
Right amygdala | 1486.7 | 1227.4–1643.0 | 1478.2 | 1215.1–1701.3 | 0.05 (0.82) |
Neither demographics and clinical, nor ROI-based GMV differed between the groups at baseline (p-values in parentheses).
Table 2
Change in clinical scores (means and standard deviations)
Variable | Yoga | Memory training | Between-group difference in change | ||
Mean change (standard deviation) | Signed rank test statistic (p) | Mean change (standard deviation) | Signed rank test statistic (p) | Kruskal-Wallis test statistic (p) | |
Anxiety | –3.1 (2.89) | –23.0 (0.02*) | 0.0 (5.88) | 0.5 (0.99) | 3.65 (0.06) |
Depression | –3.4 (4.12) | –20.5 (0.02*) | –1.27 (5.44) | –5.0 (0.6) | 1.62 (0.2) |
Resilience | 1.0 (12.1) | 1.0 (0.94) | 2.91 (9.84) | 10.0 (0.32) | 0.24 (0.62) |
Perceived Stress | 1.2 (9.74) | –0.5 (1.0) | 0.27 (4.92) | 4.5 (0.71) | 0.18 (0.67) |
Memory Function Questionnaire | |||||
Frequency of Forgetting | 0.73 (1.02) | 18.5 (0.06) | 0.003 (0.39) | –0.5 (0.98) | 1.61 (0.2) |
Seriousness of Forgetting | 0.51 (1.53) | 10.5 (0.32) | –0.47 (1.08) | 8.0 (0.52) | 2.4 (0.12) |
*p < 0.05.
GMV effects
Various large clusters across both hemispheres demonstrated a greater decrease in GMV in the MET compared to the yoga group (Fig. 2). The clusters were located in the left middle to superior temporal lobe, supramarginal extending down to the superior temporal cortex, the precentral cortex, medial superior frontal and lateral occipital cortices, as well as the right lateral postcentral cortex extending into the supramarginal cortex, superior parietal and precuneus, precentral stretching into pars opercularis, inferior parietal, superior temporal including the banks of the superior temporal sulcus, and the paracentral cortex (see cluster details in Table 3). The within-group change signed rank tests demonstrated that MET had significant GMV atrophy in all clusters, while the yoga group showed no significant changes in all but two clusters (for details see Table 4, Supplementary Table 2). Specifically, in the left precentral and left lateral occipital cortices, the yoga group showed significant increases in GMV. Further, the Yoga group showed a significant increase in the right hippocampus (see Table 4). This change was not significantly different from the change in the MET group when corrected for multiple comparisons (Kruskal-Wallis test statistic = 4.0, uncorrected p-value = 0.05, corrected p-value = 0.2). The other subcortical regions did not show significant changes in either group.
Fig. 2
Clusters showing group differences in GMV change. The MET group showed reductions in GMV in all eleven clusters across the brain over a 3-month time frame, while the yoga group showed significant increases in the left precentral (1) and lateral occipital cortices or GMV remained stable pre-to-post intervention (5).
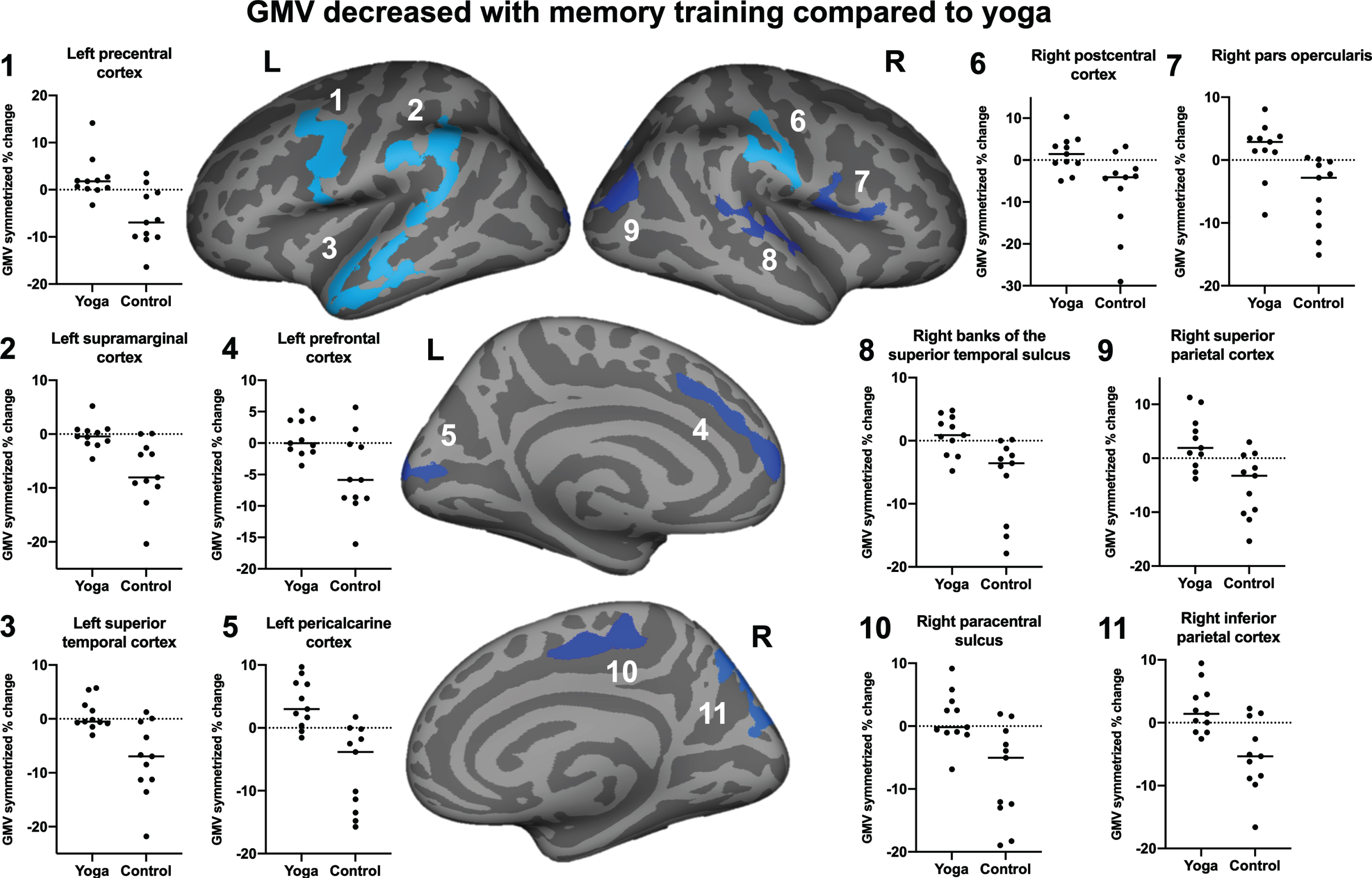
Table 3
Clusters reflecting group differences in GMV change
Cluster region | Cluster size in voxels | Talairach coordinates | p | p range | ||
X | Y | Z | ||||
Left middle temporal | 1,836.39 | –53.1 | –24.0 | –4.0 | 0.0001 | < 0.00001 –0.0002 |
Left supramarginal | 1,805.37 | –49.6 | –48.0 | 44.6 | 0.0001 | < 0.00001 –0.0002 |
Left precentral | 1,391.56 | –52.5 | –6.6 | 38.5 | 0.0001 | < 0.00001 –0.0002 |
Left superior frontal | 709.9 | –7.5 | 36.5 | 29.0 | 0.007 | 0.006 –0.008 |
Left lateral occipital | 703.46 | –12.6 | –85.5 | 9.2 | 0.007 | 0.006 –0.008 |
Right postcentral | 1,018.2 | 62.1 | –9.6 | 28.9 | 0.0001 | < 0.00001 –0.0002 |
Right precuneus | 892.05 | 19.2 | –81.2 | 36.4 | 0.0009 | 0.0009 –0.002 |
Right precentral | 760.49 | 44.9 | 8.0 | 6.8 | 0.005 | 0.004 –0.006 |
Right inferior parietal | 695.18 | 44.0 | –77.0 | 18.8 | 0.009 | 0.007 –0.01 |
Right superior temporal | 617.64 | 58.4 | –40.1 | 14.2 | 0.02 | 0.017 –0.021 |
Right paracentral | 555.49 | 8.5 | –26.3 | 49.7 | 0.04 | 0.036 –0.041 |
Cluster regions, size, and coordinates identified across various brain regions in both hemispheres.
Table 4
Within-group differences in GMV change
Variable | Yoga | Memory training | ||
Median change (range) | Signed rank test statistic (p) | Median change (range) | Signed rank test statistic (p) | |
Clusters | ||||
Left middle temporal | –0.5 (–3.03–5.73) | 3.0 (0.83) | –6.95 (–21.81–1.26) | –29.0 (0.007**) |
Left supramarginal | –0.42 (–4.62–5.21) | –6.0 (0.64) | –8.05 (–20.37–0.1) | –30.0 (0.005**) |
Left precentral | 1.78 (–3.26–14.17) | 23.0 (0.04*) | –6.94 (–16.37–3.45) | –26.0 (0.02*) |
Left superior frontal | –0.008 (–3.58–5.14) | 7.0 (0.58) | –5.85 (–16.06–5.69) | –26.0 (0.02*) |
Left lateral occipital | 2.98 (–1.55–9.68) | 28.0 (0.01*) | –3.84 (–15.73–1.73) | –30.0 (0.005**) |
Right postcentral | 1.46 (–4.98–10.33) | 10.0 (0.41) | –4.1 (–29.01–3.24) | –28.0 (0.01*) |
Right precuneus | 1.91 (–3.8–11.27) | 18.0 (0.12) | –3.26 (–15.39–3.0) | –25.0 (0.02*) |
Right precentral | 2.9 (–8.7–8.09) | 15.0 (0.21) | –2.82 (–15.08–0.38) | –29.0 (0.007**) |
Right inferior parietal | 1.42 (–2.56–9.45) | 17.0 (0.15) | –5.34 (–16.6–2.26) | –27.0 (0.01*) |
Right superior temporal | 0.9 (–4.78–4.78) | 12.0 (0.32) | –3.57 (–17.86–0.17) | –30.0 (0.005**) |
Right paracentral | –0.16 (–6.86–9.16) | 8.0 (0.52) | –5.03 (–18.97–1.94) | –28.0 (0.01*) |
Regions of interest | ||||
Left hippocampus | 38.1 (–116.1–117.3) | 11.0 (0.37) | –2.8 (–84.4–71.0) | –8.0 (0.52) |
Right hippocampus | 32.4 (–29.2–101.2) | 24.0 (0.03*) | –13.6 (–111.1–54.1) | –15.0 (0.20) |
Left amygdala | –22.2 (–51.0–97.2) | –6.0 (0.63) | 5.3 (–57.7–68.0) | 12.0 (0.32) |
Right amygdala | 37.7 (–67.2–79.9) | 9.0 (0.46) | –0.9 (–79.2–155.7) | –2.0 (0.90) |
Cluster and ROI changes in GMV within the yoga and the MET groups. *p < 0.05; **p < 0.01.
DISCUSSION
In a three-month clinical trial of yoga on memory and brain morphometry in women with an increased risk of developing AD, we found that weekly KY sessions paired with daily homework of KK preserved GMV in various regions across the brain compared to an active memory training control group and additionally led to increased left precentral and lateral occipital cortex volume. The active control MET group showed no increases in any region compared to the yoga group. Furthermore, we found that the yoga group improved in anxiety and depression, although the change in scores was not significantly different from the change in the MET group. We found no effects of yoga on memory, perceived stress, or resilience, likely due to the short intervention duration and the fact that this group of women was not clinically impaired. The small sample size may also have contributed to this lack of clinical effects. It is possible that GMV change occurs on a different time trajectory than behavioral or clinical changes. However, it is also plausible that the effect of yoga on GMV was mediated by variables we have not considered in this study. For example, our yoga training was relatively complex, including weekly group practices involving poses, meditation and chanting, interspersed with the daily home practice of meditative chanting with mental visualizations. While the individual components of this practice may have affected very specific cognitive, sensory, and motor functions and domains, they may have had little influence on memory, subjective stress, and resilience. In a previous study using the same yoga versus MET paradigm in a group of mixed older men and women with MCI, we found memory improvements in both intervention groups [29].
The regions, in which we observed GMV decline with memory training, were widespread across the cortex. The clusters are consistent with maps of age-related cortical thinning and volume loss [50]. Whole-brain longitudinal effects of yoga intervention paradigms, including a movement practice, are not yet available to our best knowledge. In a similar mixed-sex population, we previously demonstrated a trend in increased dorsal anterior cingulate cortex increases in the memory training group compared to yoga, but no effects in the hippocampus [30]. While the mechanisms of the observed preservation of GMV in the yoga group are unclear, we hypothesize that it might be due to the multi-modal nature of our intervention. For example, the cluster in the left precentral cortex covered a large portion of the primary motor cortex, while the cluster in the right postcentral cortex largely overlaps with the primary somatosensory cortex. Both movement and sensation are strong components of our yoga training, which consisted of weekly posture classes but also the daily homework sessions included touching each finger to the thumb sequentially (motor and somatosensory) and chanting (motor). Other aspects of our training included meditation, visualization, motor planning and coordination, auditory stimulation, relaxation, attention, and verbal expression (i.e., chanting mantra). KK has previously been shown to increase cerebral blood flow in the right temporal cortex and the right posterior cingulate gyrus, while it decreased blood flow in left temporo-parietal and occipital gyri compared to rest, using single-photon emission tomography [51].
We observed right hippocampal volume increases in the yoga group. While this effect did not survive corrections for multiple comparisons, it was a hypothesis-driven specific analysis, based on, and in line with previous findings [26, 27, 30]. We believe that hippocampal growth in this population is likely to occur. For instance, a postmortem study found that healthy humans have the capacity for hippocampal neurogenesis until at least their 8th decade of life [52]. According to the authors, angiogenesis and neuroplasticity decrease at older age in certain subparts of the hippocampus. It is therefore possible that even though our effect did not remain significant, beneficial neuroplastic changes may have occurred. Future studies should investigate the effect of longer yoga interventions on hippocampal growth in this population. For example, preserved hippocampal volume was found in a variety of exercise interventions, reposted by a recent large-scale meta-analysis [53]. The authors found that while the exercise groups consisting of aerobic exercise types across various healthy, psychiatric and dementia-type populations showed no change in hippocampal volume, the respective control groups demonstrated significant decreases that were in line with typical annual rates of age-related atrophy. What was surprising is that the hippocampal volume effects were significant for older samples over the age of 65. Furthermore, only interventions longer than 39 weeks were successful, and the effects were for across-hemisphere, but not lateralized hippocampal volume. While our current study was not specifically an aerobic exercise program, certain KY exercises and rapid breathing techniques may have aerobic components. It is possible that we would be able to detect significant hippocampal preservation with longer training periods, assuming that the age-related hippocampal decline would be sharper after 6 or 12 months.
We believe that our results demonstrate real GMV changes, despite the short intervention period of 12 weeks. While longitudinal research reporting the rates of cortical changes in different age and medical populations and across shorter time periods are scarce, there is evidence that the annual gray matter loss in healthy adults over 50 is more than 1% [54]. It has also been demonstrated in cross-sectional post-mortem studies that even with advanced age, the capacity to form new tissue in the medial temporal lobe is still present [52]. In younger adults, early neuroplasticity training studies have shown that 12 weeks of juggling training was sufficient to increase cortical volume in visuospatial regions, which, after a small decrease, still remained far above the baseline level after a further 12-week follow-up [55]. Later, the same group demonstrated that such gray matter increases are already detectable after only seven days of juggling training, which appeared to be less likely due to performance or exercise, but more due to the fact that participants were acquiring a new skill [56]. It is therefore plausible, yet not systematically investigated, that older women with an increased risk of AD may undergo observable GMV decline across 12 weeks.
This study has several limitations: first, the sample size was small and relatively homogenous in terms of participants’ levels of education and socioeconomic status. Since lower educational levels are a risk factor for AD [57], our more highly educated and relatively healthy sample may not be representative of the general population. While we used non-parametric statistical methods that are robust to smaller sample sizes, additional effects of yoga on clinical or brain data are likely to be masked by these sample-size limitations. Second, the dropout rates differed between the groups for different reasons. Prior and during the intervention, 14 participants discontinued in the yoga group, while only two discontinued in the MET group. MRI eligibility was only half as problematic in the yoga group compared to the MET group. Eventually, the same number of participants was available for analysis in both groups. The difficulty with MRI scanning in this population is that older people have more implanted devices, which cannot be verified as safe due to the loss of medical record keeping if the surgery was performed more than twenty years prior. Similarly, some participants were able to undergo scanning at baseline, but had implant surgery during the intervention period and were no longer eligible for safety reasons at follow-up. In addition, some participants were reluctant to perform an MRI scan or to participate for other personal reasons. Third, we may be observing a “ceiling effect” in healthy women without significant cognitive impairment at baseline. Fourth, the duration of 12 weeks of intervention may not be sufficient to detect cognitive benefits. Longer interventions may have more beneficial effects on cognition. Furthermore, while our target population consisted of healthy older women with SCD and CVRFs, we did not compare their brain GMV and clinical scores to age- and education-matched controls without cognitive problems or CVRFs. We did not use a placebo or a usual care group to document natural progression of brain aging that may have supported observed GMV decreases in the MET group. The effect of yoga versus MET on low AD risk profile women is therefore still unknown and remains to be investigated.
To conclude, we were able to demonstrate that three months of KY training combined with a daily practice of KK had protective effects on brain regions known to undergo age-related cortical decline and may lead to improvements in anxiety and depression in older women with subjective cognitive decline and CVRFs. While the long-term effects of this intervention and its efficacy in AD prevention or delay remain to be established, our results suggest that yoga can have beneficial effects on brain and mental health in this high-risk group. Since yoga is considered safe and has shown positive effects on a variety of stressors, the brain and mental health, yoga is a promising practice for older women with increased AD risk. Whether yoga has the capacity to delay the onset of AD remains to be established in the future.
ACKNOWLEDGMENTS
Funding for this study was provided by the Alzheimer’s Research and Prevention Foundation and was partially supported by the K24 AT009198 to HL.
We thank the MRI technicians at the UCLA Brain Mapping Center.
Authors’ disclosures available online (https://www.j-alz.com/manuscript-disclosures/21-5563r2).
SUPPLEMENTARY MATERIAL
[1] The supplementary material is available in the electronic version of this article: https://dx.doi.org/10.3233/JAD-215563.
REFERENCES
[1] | Prince M , Bryce R , Albanese E , Wimo A , Ribeiro W , Ferri CP ((2013) ) The global prevalence of dementia: A systematic review and metaanalysis. Alzheimers Dement 9: , 63–75.e62. |
[2] | Reiman EM , Langbaum JB , Tariot PN ((2010) ) Alzheimer’s prevention initiative: A proposal to evaluate presymptomatic treatments as quickly as possible. Biomark Med 4: , 3–14. |
[3] | Wisniewski T , Drummond E ((2020) ) APOE-amyloid interaction: Therapeutic targets. Neurobiol Dis 138: , 104784. |
[4] | Crous-Bou M , Minguillón C , Gramunt N , Molinuevo JL ((2017) ) Alzheimer’s disease prevention: From risk factors to early intervention. Alzheimers Res Ther 9: , 71. |
[5] | Snyder HM , Shineman DW , Friedman LG , Hendrix JA , Khachaturian A , Le Guillou I , Pickett J , Refolo L , Sancho RM , Ridley SH ((2016) ) Guidelines to improve animal study design and reproducibility for Alzheimer’s disease and related dementias: For funders and researchers. Alzheimers Dement 12: , 1177–1185. |
[6] | Ferretti MT , Iulita MF , Cavedo E , Chiesa PA , Schumacher Dimech A , Santuccione Chadha A , Baracchi F , Girouard H , Misoch S , Giacobini E , Depypere H , Hampel H ((2018) ) Sex differences in Alzheimer disease - the gateway to precision medicine. Nat Rev Neurol 14: , 457–469. |
[7] | Ou YN , Tan CC , Shen XN , Xu W , Hou XH , Dong Q , Tan L , Yu JT ((2020) ) Blood pressure and risks of cognitive impairment and dementia: A systematic review and meta-analysis of 209 prospective studies. Hypertension 76: , 217–225. |
[8] | Lin Y , Shan PY , Jiang WJ , Sheng C , Ma L ((2019) ) Subjective cognitive decline: Preclinical manifestation of Alzheimer’s disease. Neurol Sci 40: , 41–49. |
[9] | Qu Y , Hu HY , Ou YN , Shen XN , Xu W , Wang ZT , Dong Q , Tan L , Yu JT ((2020) ) Association of body mass index with risk of cognitive impairment and dementia: A systematic review and meta-analysis of prospective studies. Neurosci Biobehav Rev 115: , 189–198. |
[10] | Snyder HM , Asthana S , Bain L , Brinton R , Craft S , Dubal DB , Espeland MA , Gatz M , Mielke MM , Raber J ((2016) ) Sex biology contributions to vulnerability to Alzheimer’s disease: A think tank convened by the Women’s Alzheimer’s Research Initiative. Alzheimers Dement 12: , 1186–1196. |
[11] | Jack CR , Knopman DS , Jagust WJ , Petersen RC , Weiner MW , Aisen PS , Shaw LM , Vemuri P , Wiste HJ , Weigand SD , Lesnick TG , Pankratz VS , Donohue MC , Trojanowski JQ ((2013) ) Update on hypothetical model of Alzheimer’s disease biomarkers. Lancet Neurol 12: , 207–216. |
[12] | Cramer H , Lauche R , Haller H , Steckhan N , Michalsen A , Dobos G ((2014) ) Effects of yoga on cardiovascular disease risk factors: A systematic review and meta-analysis. Int J Cardiol 173: , 170–183. |
[13] | Bhattacharyya KK , Andel R , Small BJ ((2021) ) Effects of yoga-related mind-body therapies on cognitive function in older adults: A systematic review with meta-analysis. Arch Gerontol Geriatr 93: , 104319. |
[14] | Brenes GA , Sohl S , Wells RE , Befus D , Campos CL , Danhauer SC ((2019) ) The effects of yoga on patients with mild cognitive impairment and dementia: A scoping review. Am J Geriatr Psychiatry 27: , 188–197. |
[15] | Hariprasad VR , Koparde V , Sivakumar PT , Varambally S , Thirthalli J , Varghese M , Basavaraddi IV , Gangadhar BN ((2013) ) Randomized clinical trial of yoga-based intervention in residents from elderly homes: Effects on cognitive function. Indian J Psychiatry 55: , S357–363. |
[16] | Chobe S , Chobe M , Metri K , Patra SK , Nagaratna R ((2020) ) Impact of Yoga on cognition and mental health among elderly: A systematic review. Complement Ther Med 52: , 102421. |
[17] | Fan JT , Chen KM ((2011) ) Using silver yoga exercises to promote physical and mental health of elders with dementia in long-term care facilities. Int Psychogeriatr 23: , 1222–1230. |
[18] | Cartwright T , Mason H , Porter A , Pilkington K ((2020) ) Yoga practice in the UK: A cross-sectional survey of motivation, health benefits and behaviours. BMJ Open 10: , e031848. |
[19] | Cramer H , Ward L , Steel A , Lauche R , Dobos G , Zhang Y ((2016) ) Prevalence, patterns, and predictors of yoga use: Results of a U.S. nationally representative survey. Am J Prev Med 50: , 230–235. |
[20] | Innes KE , Selfe TK , Khalsa DS , Kandati S ((2016) ) Effects of meditation versus music listening on perceived stress, mood, sleep, and quality of life in adults with early memory loss: A pilot randomized controlled trial. J Alzheimers Dis 52: , 1277–1298. |
[21] | Villemure C , Čeko M , Cotton VA , Bushnell MC ((2015) ) Neuroprotective effects of yoga practice: Age-, experience-, and frequency-dependent plasticity. Front Hum Neurosci 9: , 281–281. |
[22] | Gothe NP , Hayes JM , Temali C , Damoiseaux JS ((2018) ) Differences in brain structure and function among yoga practitioners and controls. Front Integr Neurosci 12: , 26. |
[23] | Froeliger B , Garland EL , McClernon FJ ((2012) ) Yoga meditation practitioners exhibit greater gray matter volume and fewer reported cognitive failures: Results of a preliminary voxel-based morphometric analysis. Evid Based Complement Alternat Med 2012: , 821307. |
[24] | Hernández SE , Suero J , Barros A , González-Mora JL , Rubia K ((2016) ) Increased grey matter associated with long-term Sahaja yoga meditation: A voxel-based morphometry study. PLoS One 11: , e0150757. |
[25] | Hernández SE , Dorta R , Suero J , Barros-Loscertales A , González-Mora JL , Rubia K ((2020) ) Larger whole brain grey matter associated with long-term Sahaja Yoga Meditation: A detailed area by area comparison. PLoS One 15: , e0237552. |
[26] | Dodich A , Zollo M , Crespi C , Cappa SF , Laureiro Martinez D , Falini A , Canessa N ((2019) ) Short-term Sahaja Yoga meditation training modulates brain structure and spontaneous activity in the executive control network. Brain Behav 9: , e01159. |
[27] | Hariprasad VR , Varambally S , Shivakumar V , Kalmady SV , Venkatasubramanian G , Gangadhar BN ((2013) ) Yoga increases the volume of the hippocampus in elderly subjects. Indian J Psychiatry 55: , S394–396. |
[28] | Eyre HA , Acevedo B , Yang H , Siddarth P , Van Dyk K , Ercoli L , Leaver AM , Cyr NS , Narr K , Baune BT , Khalsa DS , Lavretsky H ((2016) ) Changes in neural connectivity and memory following a yoga intervention for older adults: A pilot study. J Alzheimers Dis 52: , 673–684. |
[29] | Eyre HA , Siddarth P , Acevedo B , Van Dyk K , Paholpak P , Ercoli L , St Cyr N , Yang H , Khalsa DS , Lavretsky H ((2017) ) A randomized controlled trial of Kundalini yoga in mild cognitive impairment. Int Psychogeriatr 29: , 557–567. |
[30] | Yang H , Leaver AM , Siddarth P , Paholpak P , Ercoli L , St Cyr NM , Eyre HA , Narr KL , Khalsa DS , Lavretsky H ((2016) ) Neurochemical and neuroanatomical plasticity following memory training and yoga interventions in older adults with mild cognitive impairment. Front Aging Neurosci 8: , 277. |
[31] | Farhang M , Miranda-Castillo C , Rubio M , Furtado G ((2019) ) Impact of mind-body interventions in older adults with mild cognitive impairment: A systematic review. Int Psychogeriatr 31: , 643–666. |
[32] | Zou Y , Zhao X , Hou YY , Liu T , Wu Q , Huang YH , Wang XH ((2017) ) Meta-analysis of effects of voluntary slow breathing exercises for control of heart rate and blood pressure in patients with cardiovascular diseases. Am J Cardiol 120: , 148–153. |
[33] | Zhang Y , Li C , Zou L , Liu X , Song W ((2018) ) The effects of mind-body exercise on cognitive performance in elderly: A systematic review and meta-analysis. Int J Environ Res Public Health 15: , 2791. |
[34] | Hughes CP , Berg L , Danziger W , Coben LA , Martin RL ((1982) ) A new clinical scale for the staging of dementia. Br J Psychiatry 140: , 566–572. |
[35] | Folstein MF , Folstein SE , McHugh PR ((1975) ) “Mini-mental state”: A practical method for grading the cognitive state of patients for the clinician. J Psychiatr Res 12: , 189–198. |
[36] | Gilewski MJ , Zelinski EM , Schaie KW ((1990) ) The Memory Functioning Questionnaire for assessment of memory complaints in adulthood and old age. Psychol Aging 5: , 482–490. |
[37] | Molinuevo JL , Rabin LA , Amariglio R , Buckley R , Dubois B , Ellis KA , Ewers M , Hampel H , Klöppel S , Rami L , Reisberg B , Saykin AJ , Sikkes S , Smart CM , Snitz BE , Sperling R , van der Flier WM , Wagner M , Jessen F ((2017) ) Implementation of subjective cognitive decline criteria in research studies. Alzheimers Dement 13: , 296–311. |
[38] | Beck AT , Ward CH , Mendelson M , Mock J , Erbaugh J ((1961) ) An inventory for measuring depression. Arch Gen Psychiatry 4: , 561–571. |
[39] | Hamilton M ((1959) ) The assessment of anxiety states by rating. Br J Med Psychol 32: , 50–55. |
[40] | Connor KM , Davidson JR ((2003) ) Development of a new resilience scale: The Connor-Davidson Resilience Scale (CD-RISC). Depress Anxiety 18: , 76–82. |
[41] | Cohen S , Kamarck T , Mermelstein R ((1983) ) A global measure of perceived stress. J Health Soc Behav 24: , 385–396. |
[42] | Lingjaerde O , Ahlfors UG , Bech P , Dencker SJ , Elgen K ((1987) ) The UKU side effect rating scale. A new comprehensive rating scale for psychotropic drugs and a cross-sectional study of side effects in neuroleptic-treated patients. Acta Psychiatr Scand Suppl 334: , 1–100. |
[43] | Kang J , Scholp A , Jiang JJ ((2018) ) A review of the physiological effects and mechanisms of singing. J Voice 32: , 390–395. |
[44] | Verhaeghen P , Marcoen A , Goossens L ((1992) ) Improving memory performance in the aged through mnemonic training: A meta-analytic study. Psychol Aging 7: , 242–251. |
[45] | Gross AL , Rebok GW ((2011) ) Memory training and strategy use in older adults: Results from the ACTIVE study. Psychol Aging 26: , 503–517. |
[46] | McCarty DL ((1980) ) Investigation of a visual imagery mnemonic device for acquiring face–name associations. J Exp Psychol Hum Learn 6: , 145–155. |
[47] | Desikan RS , Ségonne F , Fischl B , Quinn BT , Dickerson BC , Blacker D , Buckner RL , Dale AM , Maguire RP , Hyman BT , Albert MS , Killiany RJ ((2006) ) An automated labeling system for subdividing the human cerebral cortex on MRI scans into gyral based regions of interest. Neuroimage 31: , 968–980. |
[48] | Reuter M , Schmansky NJ , Rosas HD , Fischl B ((2012) ) Within-subject template estimation for unbiased longitudinal image analysis. Neuroimage 61: , 1402–1418. |
[49] | Reuter M , Fischl B ((2011) ) Avoiding asymmetry-induced bias in longitudinal image processing. Neuroimage 57: , 19–21. |
[50] | Salat DH , Buckner RL , Snyder AZ , Greve DN , Desikan RS , Busa E , Morris JC , Dale AM , Fischl B ((2004) ) Thinning of the cerebral cortex in aging. Cereb Cortex 14: , 721–730. |
[51] | Khalsa DS , Amen D , Hanks C , Money N , Newberg A ((2009) ) Cerebral blood flow changes during chanting meditation. Nucl Med Commun 30: , 956–961. |
[52] | Boldrini M , Fulmore CA , Tartt AN , Simeon LR , Pavlova I , Poposka V , Rosoklija GB , Stankov A , Arango V , Dwork AJ , Hen R , Mann JJ ((2018) ) Human hippocampal neurogenesis persists throughout aging. Cell Stem Cell 22: , 589–599.e585. |
[53] | Wilckens KA , Stillman CM , Waiwood AM , Kang C , Leckie RL , Peven JC , Foust JE , Fraundorf SH , Erickson KI ((2021) ) Exercise interventions preserve hippocampal volume: A meta-analysis. Hippocampus 31: , 335–347. |
[54] | Raz N , Rodrigue KM , Head D , Kennedy KM , Acker JD ((2004) ) Differential aging of the medial temporal lobe: A study of a five-year change. Neurology 62: , 433–438. |
[55] | Draganski B , Gaser C , Busch V , Schuierer G , Bogdahn U , May A ((2004) ) Changes in grey matter induced by training. Nature 427: , 311–312. |
[56] | Driemeyer J , Boyke J , Gaser C , Büchel C , May A ((2008) ) Changes in gray matter induced by learning—revisited. PLoS One 3: , e2669. |
[57] | Hersi M , Irvine B , Gupta P , Gomes J , Birkett N , Krewski D ((2017) ) Risk factors associated with the onset and progression of Alzheimer’s disease: A systematic review of the evidence. Neurotoxicology 61: , 143–187. |