A Cystatin C Cleavage ELISA Assay as a Quality Control Tool for Determining Sub-Optimal Storage Conditions of Cerebrospinal Fluid Samples in Alzheimer’s Disease Research
Abstract
Background:
An N-terminal octapeptide cleavage of the cystatin C protein was discovered by mass spectrometry when cerebrospinal fluid (CSF) was stored at –20°C for 3 months, which did not occur when CSF was stored at –80°C.
Objective:
The aim was to develop an immunoassay as quality assessment tool to detect this –20°C cleavage of cystatin C in CSF and support Alzheimer’s disease research.
Methods:
A specific monoclonal antibody and a double indirect sandwich ELISA were developed: one assay quantifies the octapeptide uncleaved protein specifically and the other quantifies the total cystatin C present in the biological fluid (both cleaved and uncleaved forms). The ratio of these concentrations was calculated to assess the extent of cleavage of cystatin C. The novel ELISA was validated and applied in a short-term (up to 4 weeks) and mid-term (up to one year) stability study of CSF stored at 4°C, –20°C, –80°C, and liquid nitrogen. Impact of freeze-thaw cycles, adsorption, and protease inhibitors were tested.
Results:
The ratio of truncated protein was modified following –20°C storage and seemed to reach a plateau after 6 months. The ratio was impacted neither by freeze-thaw cycles nor adsorption. The –20°C specific cleavage was found to be protease related.
Conclusion:
Using this novel double indirect sandwich ELISA, absolute levels of the total and uncleaved cystatin C and the ratio of truncated cystatin C can be measured. This assay is an easily applicable tool which can be used to confirm that CSF biospecimen are fit-for-purpose for Alzheimer’s disease research.
INTRODUCTION
Cerebrospinal fluid (CSF) surrounds and supports the central nervous system (CNS). As such, the composition of lumbar CSF reflects the processes of the CNS [1]. CSF therefore is a body fluid of interest for biomarker discovery in neurological conditions, such as neurodegenerative and neuroinflammatory diseases. Examples of successful implementation of CSF biomarkers in diagnostics are the presence of oligoclonal IgM bands in multiple sclerosis and abnormal amyloid-β (Aβ) concentrations in Alzheimer’s disease (AD) [2, 3].
Biomarker discovery and validation critically depend on the molecular quality of the biospecimens used. Biospecimen composition can be modified by preanalytical factors during collection, processing, and storage [4, 5]. Suboptimal long-term storage conditions of CSF can induce molecular degradation and introduce bias in biomarker studies [6, 7].
The revised diagnostic criteria of AD include the CSF concentrations of Aβ peptides in combination with the tau protein and its hyperphosphorylated forms [8]. The co-localization of cystatin C and Aβ has been observed in parenchymal and vascular amyloid deposits in brains of patients with AD [9]. The role of cystatin C in AD is unclear: on the one side, it seems to regulate the Aβ levels by binding and inhibiting the Aβ aggregation [10], on the other side it may counter Aβ degradation by inhibiting the protease cathepsin B [11, 12]. Previous work showed that the N-terminal decapeptide of cystatin C is required for effective inhibition of cathepsin B [13]. Removal of the N-terminal segment drastically affects enzyme affinity between cystatin C and cathepsin B [14]. Cystatin C has not only been studied as a brain tissue biomarker in AD but has also been suggested as an indicator of the overall quality of CSF, a biological fluid in which a plethora of other biomarkers can be found.
An N-terminal octapeptide truncation between amino acid residues 8R and 9L of the cystatin C protein was discovered by MALDI-TOF mass spectrometry when CSF was stored at –20°C for 3 months, which did not occur when CSF was stored at –80°C [15]. This was subsequently confirmed by several other studies using mass spectrometry [16–19]. The cleavage of cystatin C was initially erroneously interpreted as a biomarker for multiple sclerosis [20]. The cleavage state of cystatin C could therefore represent a biomarker to assess CSF sample storage conditions [21]. Although MALDI-TOF is an excellent method for biomarker discovery, it is too laborious and expensive to use as a routine quality assessment tool.
In the present investigation, the aim was to develop an easily applicable tool for monitoring cystatin C cleavage status which informs of CSF sample quality. As such, we developed a method of measuring the cleavage state of cystatin C based on a novel double indirect sandwich enzyme-linked immunosorbent assay (ELISA), eliminating the need of mass spectrometry. This double indirect sandwich ELISA was used to validate the hypothesis that the cystatin C protein is cleaved when CSF is stored at –20°C, but not at –80°C or in liquid nitrogen (LN). We performed a short-term stability study (up to 4 months) at room temperature (RT), 4°C, –20°C and –80°C, a mid-term stability study (up to one year) at –20°C, –80°C, and LN, and a long-term stability study (4–16 years) at –80°C. Next, we performed an adsorption study and freeze-thaw stability analysis to further validate the new method and the preanalytical sensitivity of cystatin C. In order to understand the ex-vivo mechanism inducing cystatin C cleavage, we tested the effect of protease inhibitors (PI).
MATERIALS AND METHODS
Monoclonal antibodies
The mouse monoclonal anti-cystatin C Cyst28 antibody (HyTest, FI #4CC1) was used to detect the total cystatin C protein (both the cleaved and uncleaved forms of the protein). The epitope recognized by this antibody is conformational, encompassing residues 54K-60V, 85F-91L, 92K-99F, and 101I-111T [22] (Fig. 1A).
Fig. 1
Cystatin C protein and the ELISA assay. (A) Amino acid sequence of Cystatin C. The N-terminal octapeptide is highlighted in black (the preceding 26 amino acids are cleaved before the mature protein is secreted). The epitope recognized by mAb Cyst28 encompasses residues 54 -60, 85 -91, 92 -99 and 101 – 111 and is highlighted in darker grey. The sequences interacting with target proteolytic enzymes are underlined. (B) Schematic representation of secreted Cystatin C, showing the targets of the antibodies used in the ELISA. The mAb Cyst28 detects both octapeptide-uncleaved and cleaved cystatin C. The cleavage site-specific antibody recognizes specifically the octapeptide-uncleaved cystatin C.
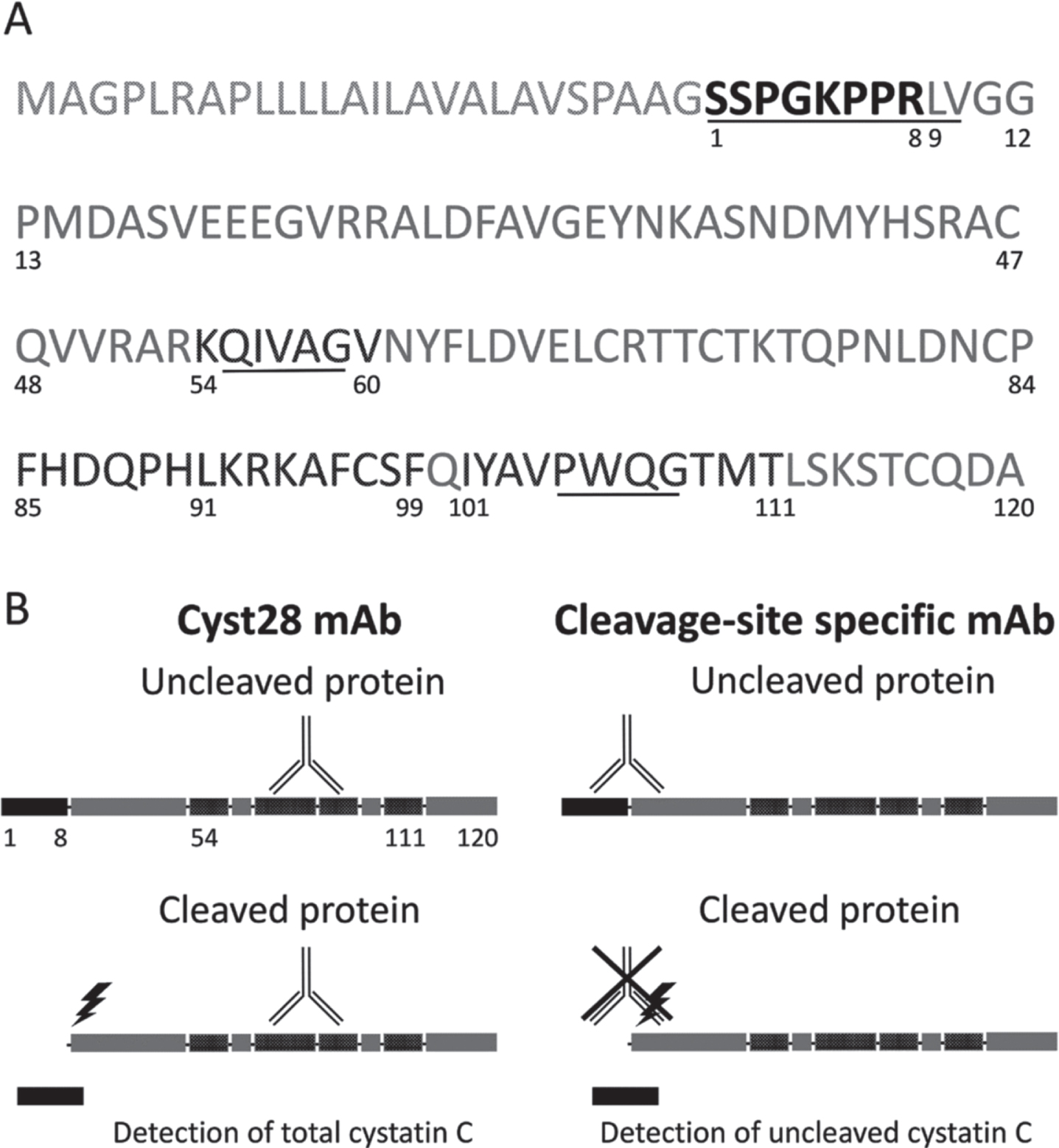
A mouse monoclonal antibody (Clone 10G6-B9-A3, IgG1,k), targeted against the octapeptide uncleaved protein was developed by our team, using Bio-Rad Laboratories as a supplier. The antibody was used for the quantification of the N-terminal uncleaved protein. Murine antibodies were generated by immunization of female 8-week-old CD-1 mice (Charles Rivers) with an octapeptide, corresponding to 1S-8R and coupled to bovine serum albumin (Bio-Rad, 15L433). The detailed description of the immunization protocol, hydridoma production with the Sp2/0Ag14 myeloma cell line (ATCC, FR #CRL 1581), and monoclonal antibody purification have been largely published [23]. Throughout the process, clone selection was done by sandwich ELISA based on capture with the anti-C terminal polyclonal antibody DP2003 (OriGene EU). Seven specific monoclonal antibodies were selected, amplified, and purified on protein A Sepharose columns (GE Healthcare). An epitope mapping test demonstrated that the mice had produced antibodies specific to the peptide 1S-8R.
Double indirect sandwich ELISA
To assess the use of cystatin C as a tool for quality control, two indirect sandwich ELISAs were developed: one assay quantifies specifically the uncleaved protein only, while the other quantifies both the cleaved and the uncleaved forms of cystatin C (“total cystatin C”) (Fig. 1B). The truncation state of cystatin C is measured by the ratio between the concentration of uncleaved protein and the concentration of “total cystatin C” protein. This ratio is referred to as “cystatin C ratio”. A high ratio indicates few or no truncated protein molecules are present in the CSF, while a low ratio indicates that many cleaved protein molecules are present. Standards and samples were run on both ELISAs.
The cystatin C capture polyclonal antibody DP2003 was diluted to 1μg/mL in 1X PBS (Thermo Fisher Scientific, EU #14190094). A 96-well plate (Thermo Fisher Scientific, EU #439454) was coated with 100μl per well and incubated overnight at 4°C. The plate was washed three times with 300μL per well of T-PBS (1X PBS 0.1% TWEEN®20 Detergent (Merck, 655205)). The plate was saturated with 200μL per well of blocking solution consisting of 1%skim milk (Régilait) in 1X PBS and incubated 1h in a 37°C incubator. T-PBS-diluted standard curve and test samples were tested in duplicate. The recombinant protein (Cell Signaling Technologies, EU #5717-no carrier) was used to create the standard curve, ranging from 25 ng/mL to 0.7815 ng/mL by sequential dilutions. The typical CSF sample dilutions were 750× or 1500×. The plate was incubated for 1 h 30 min at 37°C. The plate was washed three times as described previously. 100μl per well of 1μg/ml in T-PBS of the specific monoclonal antibodies (Cyst28 or cleavage site-specific) were dispensed and the plate was incubated for 1 h 30 min at 37°C, then washed three times. 100μL per well of an anti-Mouse IgG (γ-chain specific)-Peroxidase antibody (Sigma-Aldrich, BE #A3673) diluted 3000 times in T-PBS was dispensed. The plate was incubated for 1 h at 37°C, then washed four times. 100μl per well of TMB Peroxidase EIA Substrate solution (Bio-Rad, FR #1721066) was added and incubated in the dark for 20 min. The reaction was stopped with the addition of 100μl per well of 1N sulphuric acid solution (Sigma-Aldrich, BE # 339741). OD450 nm spectrophotometry was performed on a Synergy Mx (Bio-Tek Instruments, EU).
To verify the specificity of these monoclonal antibodies against cystatin C, cell supernatant of HAP1 cells (Horizon™), wild-type and CST3-knockout, was tested. A signal was obtained using both antibodies for the wild-type cell supernatant while no signal was obtained for the knockout cell supernatant with media incubated with cells for 48 h. This confirms that both antibodies detect cystatin C.
Cerebrospinal fluid samples
CSF surplus samples (e.g., samples having inadequate clinical information for use in clinical validation) from the Amsterdam Dementia Cohort (ADC) were used for this study [24]. The protocol was approved by the institutional review board (reference number: 2017.315) and subjects gave written consent. ADC CSF was collected by lumbar puncture in 10 mL polypropylene tubes (Sarstedt, EU # 62.610.018). CSF samples were centrifuged at 1800 to 2100 g for 10 min at 4°C within 2 h after collection, aliquoted into polypropylene tubes (1.5 or 2.0 mL; Sarstedt, EU 72.703 or 72.694.007) in 500μL volumes [25]. Subsequently, either freshly collected or CSF surplus samples stored at –80°C, were used to perform the specific experimental sample treatments as described below.
Short-term storage stability study (up to 4 months)
Frozen CSF surplus samples were thawed and used to prepare three pools for short-term storage. Aliquots of 350μl of three different CSF pools were stored in 1.5 ml polypropylene tubes (Sarstedt, EU 72.703). The aliquoted CSF pools were exposed to storage conditions as described in a published protocol for sample stability [26], i.e., storage for up to 1 week at either 4°C or room temperature (RT) or storage at –20°C for up to 1 or 4 months, before final storage at –80°C.
Mid-term storage stability study (up to one year)
Ten fresh CSF surplus samples from the ADC were centrifuged at 1800 to 2100 g for 10 min at 4°C within 2 h after collection, and divided into 100μl aliquots (polypropylene tubes, 1.5 ml Sarstedt, EU #72.703). Aliquots were directly snap-frozen in LN, or directly stored at –80°C or –20°C. The reference aliquot was stored directly at –80°C at time point zero. LN-stored aliquots were measured at baseline, 4 weeks, 8 weeks, 4 months, and 6 months. Aliquots stored at –80°C, or –20°C were placed into LN storage after 6 weeks, 10 weeks, 4 months, and 6 months and measured in one run at the end of the 6 months storage.
Long-term storage stability study (4–16 years)
The long-term storage study design was described before [27]. Frozen CSF samples from 116 AD patients from the ADC were selected. The patients had a narrow age range [Median: 68.3, range: 64–72 years], equally distributed APOE genotype [ɛ3/ɛ3, ɛ3/ɛ4, ɛ4/ɛ4: 28, 54, 34] and equivalent gender distribution across the storage duration [male, female: 52, 64] [27]. Sample storage duration at –80°C ranged between 4 and 16.4 years.
Adsorption on tube walls and freeze-thaw stability
Frozen CSF surplus samples were thawed and used to prepare pools for preanalytical experiments. Three CSF pools were aliquoted in 150μl volumes and transferred 0 or 4 times into a new tube of the same type (polypropylene tubes, 2.0 ml Sarstedt, EU #72.694.007) with an “incubation” time of 1 min at RT between transfers. A new pipette tip was used for every transfer. Three CSF pools were exposed to 1 and 7 freeze/thaw cycles as described in a published protocol for sample stability [26].
Mechanism of cystatin C truncation
Ten fresh CSF surplus samples from the ADC were divided in 96μL aliquots (polypropylene tubes, 2 mL Sarstedt, EU #72.694.007). One tablet Complete® protease inhibitor cocktail of proprietary composition (Roche Diagnostics GmbH, EU #04693116001) was dissolved in 2 mL of nuclease-free water (stock solution), and 4μl of the PI stock solution or 4μl of nuclease-free water was added to the CSF aliquots. Aliquots were directly snap-frozen in LN, or directly stored at –80°C or –20°C. Aliquots were measured at baseline, and after 4 and 8 weeks of storage.
Statistical analysis
Friedman Test with a post-hoc Conover test was used for the mid-term stability study, linear mixed models were used for the long-term stability study and Student’s t-test was used for the adsorption and mechanism study with significance level p < 0.05. The Friedman Test was done in RStudio version 1.1.463. The linear mixed model was done using Analyse-it version 4.90.1. The Student’s t-test and all graphs were done in Excel version 2003. The standard deviation (SD) of the ratio Y was calculated as follows: SD(Y) = SQRT(Y∧2*((((CVuncleaved*CCuncleaved/100) / CCuncleaved)∧2 + ((CCtotal*CVtotal/100) / CCtotal)∧2))-(2*R*CCuncleaved*((CVuncleaved*CCuncleaved / 100))*((CCtotal*CVtotal /100))/CCtotal∧3)), where R is the correlation coefficient between the concentrations (CC) of uncleaved and total cystatin C, and CV is the coefficient of variation.
RESULTS
Double indirect sandwich cystatin C ELISA development
The average background of blank samples at OD450 was 0.174 for the total and 0.172 for the uncleaved protein. The calibration curve, obtained with a four-parameter logistic curve, covered a range of 0.78 ng/ml to 25 ng/mL, had R2 = 0.999 for total cystatin C and R2 = 0.999 for uncleaved cystatin C. The correlation coefficient between the concentrations of uncleaved and total cystatin C is 0.94.
To assess the intra- and inter-plate variation, an internal quality control CSF material was included in each ELISA assay. The quality control material was stored in LN throughout the project and was tested in the typical CSF sample dilutions (750× and 1500×) in each ELISA assay. The variation within plate corresponded to a coefficient of variation (CV) of 1.8%for the total protein, 1.6%for the uncleaved protein and 1.9%for the cystatin C ratio. The variation between plates corresponded to a CV of 31.9%for the total protein concentration, 30.7%for the uncleaved protein concentration and 10.9%for the cystatin C ratio.
Short-term storage stability
As shown in Fig. 2A, no difference in the mean cystatin C ratio was observed when CSF was stored up to one week at RT or at 4°C. At one week, the mean ratio was already decreasing for CSF stored at –20°C (Fig. 2A). As storage time at –20°C increased from one week up to one month, a mean 31.2%reduction of the mean cystatin C ratio was observed (Fig. 2B).
Fig. 2
Mean ratio of uncleaved to total cystatin C protein of three CSF sample pools tested in a short-term storage stability study. Dashed line indicates sample storage at RT, dotted line at 4°C and solid line at –20°C for up to one week (A). Solid line indicates sample storage at –20°C from 1 week to 4 months (B). Error bars are one standard deviation.
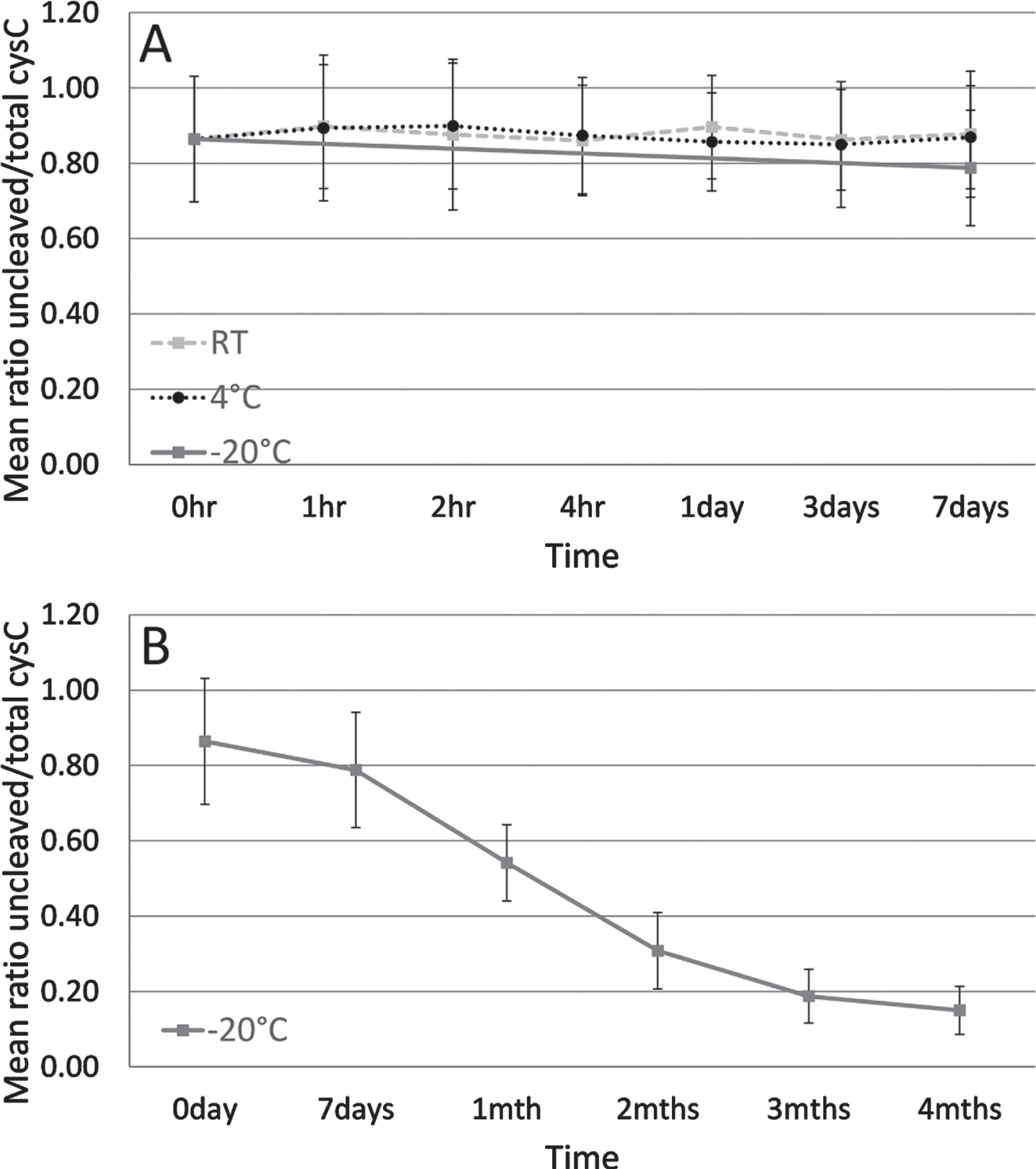
Mid-term storage stability
Storage from 6 to 52 weeks at –20°C caused a mean 85.4%reduction of the mean cystatin C ratio, reaching a bottom plateau at week 26. The samples stored at –80°C or LN remained stable or even showed a slight increase in the mean cystatin C ratio after 52 weeks. Friedman test showed a significant difference between the tested temperatures (p = 0.02) as shown in Fig. 3. The Conover analysis showed that the difference was due to the difference between LN and –20°C (p = 0.003), and between –80°C and –20°C (p = 0.003).
Fig. 3
Cystatin C ratio in a mid-term storage stability study. Samples were analyzed at 6, 10, 17, 26, and 52 weeks when stored at –20°C (solid line) and at –80°C (dashed line); and at 4, 8, 19, 26, and 52 weeks when stored in LN (dotted line). The reference sample is shown by a triangle dot. Error bars are one standard deviation.
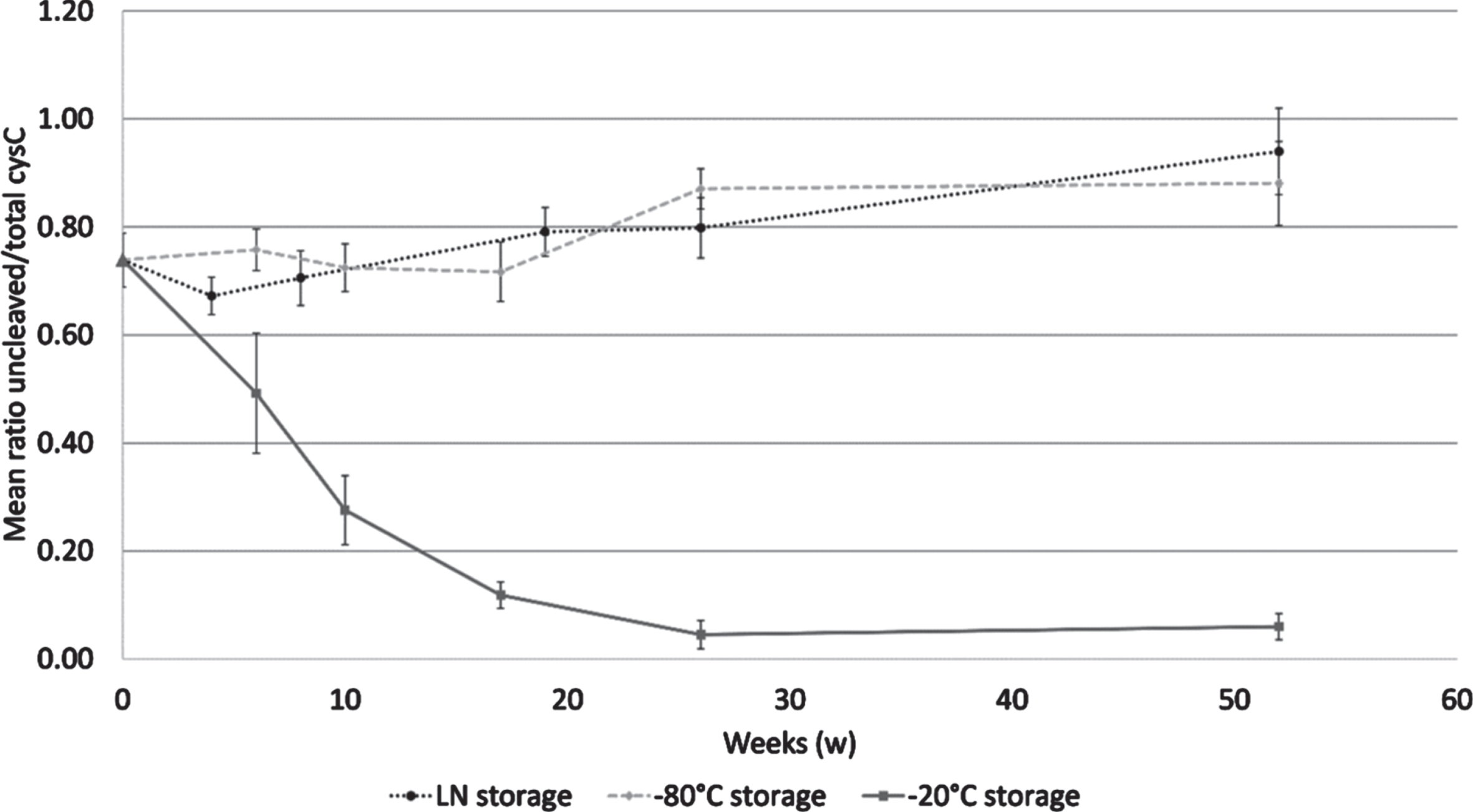
Long-term storage stability
As shown in Fig. 4, the mean concentration of total and uncleaved cystatin C in CSF from patients with AD (n = 116) was 17.30±5.71 mg/L (range: 5.95–29.90) and 13.05±4.31 mg/L (range: 4.46–25.53) respectively. The same pattern of distribution of total versus uncleaved cystatin C concentration was seen for each sample. Linear mixed model analysis showed a slight decrease of the mean concentration of total cystatin C with –80°C storage time (β[slope±SEM] = –367.9±156.2 ng/mL per year, p = 0.02). There was a slight decrease, but not statistically significant, of the mean concentration of uncleaved cystatin C with –80°C storage time. There was a slight but steady increase of the cystatin C ratio with –80°C storage time (β[slope±SEM] = 0.0039±0.0013 ng/mL per year, p = 0.004).
Fig. 4
Long-term –80°C storage stability study of total (A) and uncleaved (B) cystatin C concentration and ratio (C) using 116 Alzheimer’s disease patient CSF samples. Dotted line indicates linear trend line. The arrows indicate the concentrations in three selected samples (a, b, and c), showing the similar pattern of distribution of total and uncleaved cystatin C concentrations
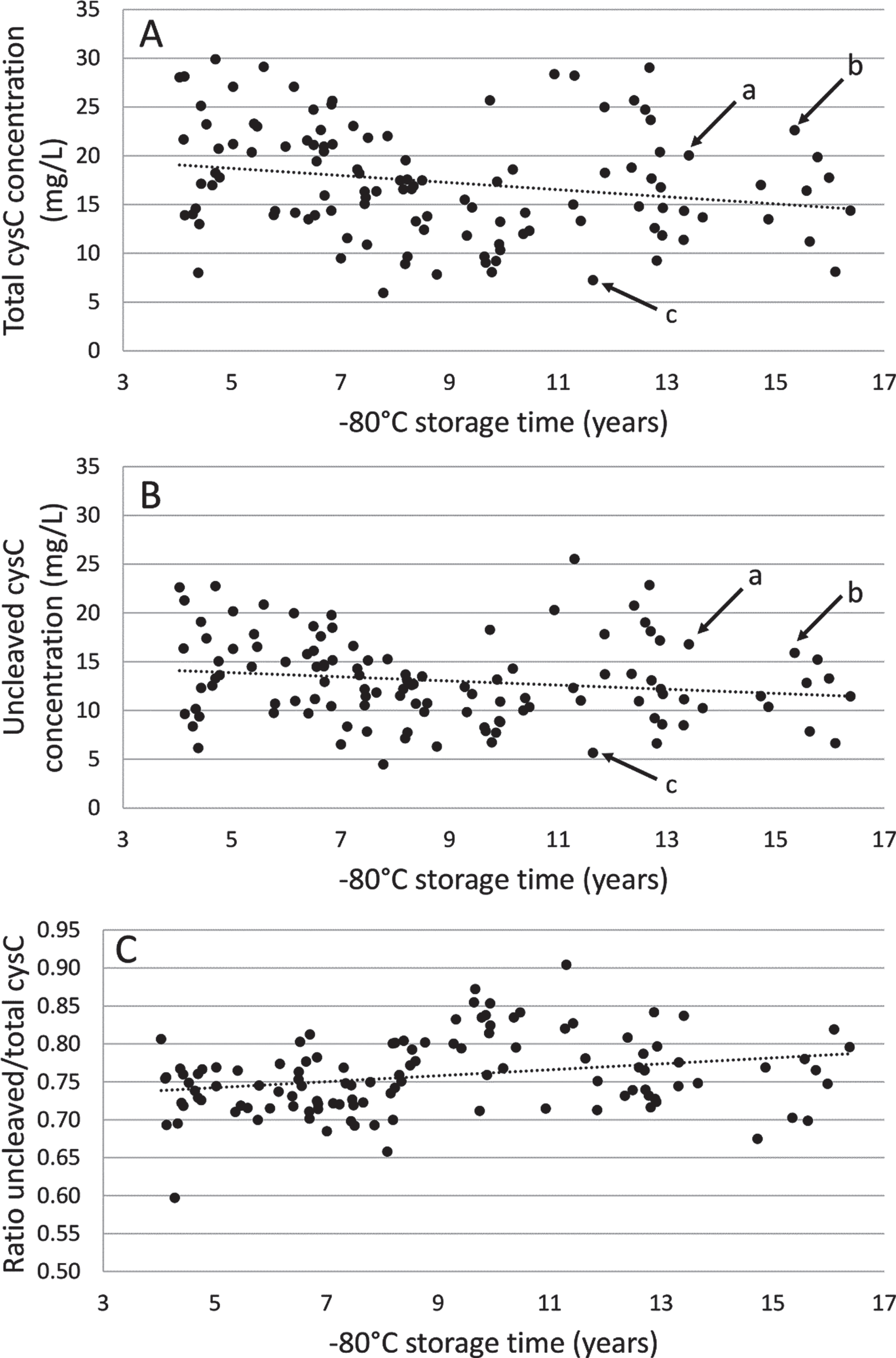
Adsorption on tube walls and freeze-thaw stability
We next studied the adsorption and the freeze-thaw stability of the cystatin C protein as well as their impact on the cystatin C ratio. As shown in Table 1, a mean 40.3 %reduction in the total cystatin C concentration and a mean 41.9%reduction in the uncleaved cystatin C concentration was detected between condition 1 (0 transfer step) and 2 (4 transfer steps) for three CSF sample pools. There was no significant difference between the cystatin C ratio values in condition 1 and condition 2 for the three CSF sample pools.
Table 1
Effect of adsorption and freeze-thaw cycles on mean levels of uncleaved cystatin C, total cystatin C and ratio of these two measurements
Adsorption | Freeze-thaw cycles | |||||
Condition 1 (0 transfer steps prior –80°C storage) | Condition 2 (4 transfer steps prior –80°C storage) | Reduction (%) between the two conditions | Condition 1 (0 freeze-thaw cycles) | Condition 2 (7 freeze-thaw cycles) | Reduction (%) between the two conditions | |
Total cystatin C concentration (mg/L) | 5.9±1.7 | 3.6±1.2 | 40.3 | 21.1±6.8 | 20.8±8.2 | 2.2 |
Uncleaved cystatin C concentration (mg/L) | 5.2±1.5 | 3.1±1.1 | 41.9 | 17.8±4.9 | 16.4±3.6 | 6.8 |
Cystatin C ratio | 0.88±0.04 | 0.86±0.06 | 2.9 | 0.86±0.17 | 0.83±0.21 | 4.11 |
Next, the stability of the ratio upon several freeze-thaw cycles was tested. As shown in Table 1, a mean 7.2%reduction in the total cystatin C concentration and a mean 9.0%reduction in the uncleaved cystatin C concentration was detected between condition 1 (0 free-thaw cycle) and 2 (7 freeze-thaw cycles) for three CSF sample pools. There was no significant difference between the cystatin C ratio after 0 (condition 1) and 7 freeze-thaw cycles (condition 2), for the three CSF sample pools.
Effect of protease inhibitors (PI) on cystatin C ratio
As shown in Fig. 5, a statistically significant decrease in the mean cystatin C ratio of 10 CSF sample pools without PI was observed when samples were stored in a –20°C freezer for 8 weeks (p = 0.003), but not for 4 weeks. Similarly, there were no statistically significant differences in the mean cystatin C ratio of the CSF sample pools with or without PI when stored in a –80°C freezer for 4 and 8 weeks. Treatment of the CSF sample pools with PI before storage in a –20°C freezer for 4 and 8 weeks abolished the decrease of the mean cystatin C ratio.
Fig. 5
Mean cystatin C ratio values of ten CSF sample pools with (white) or without (grey) proteinase inhibitors (PI), when stored at –20°C or –80°C for 4 and 8 weeks. The baseline samples (0 week) do not contain PI. Error bars are one standard deviation and statistically significant differences (p < 0.05) are denoted by *.
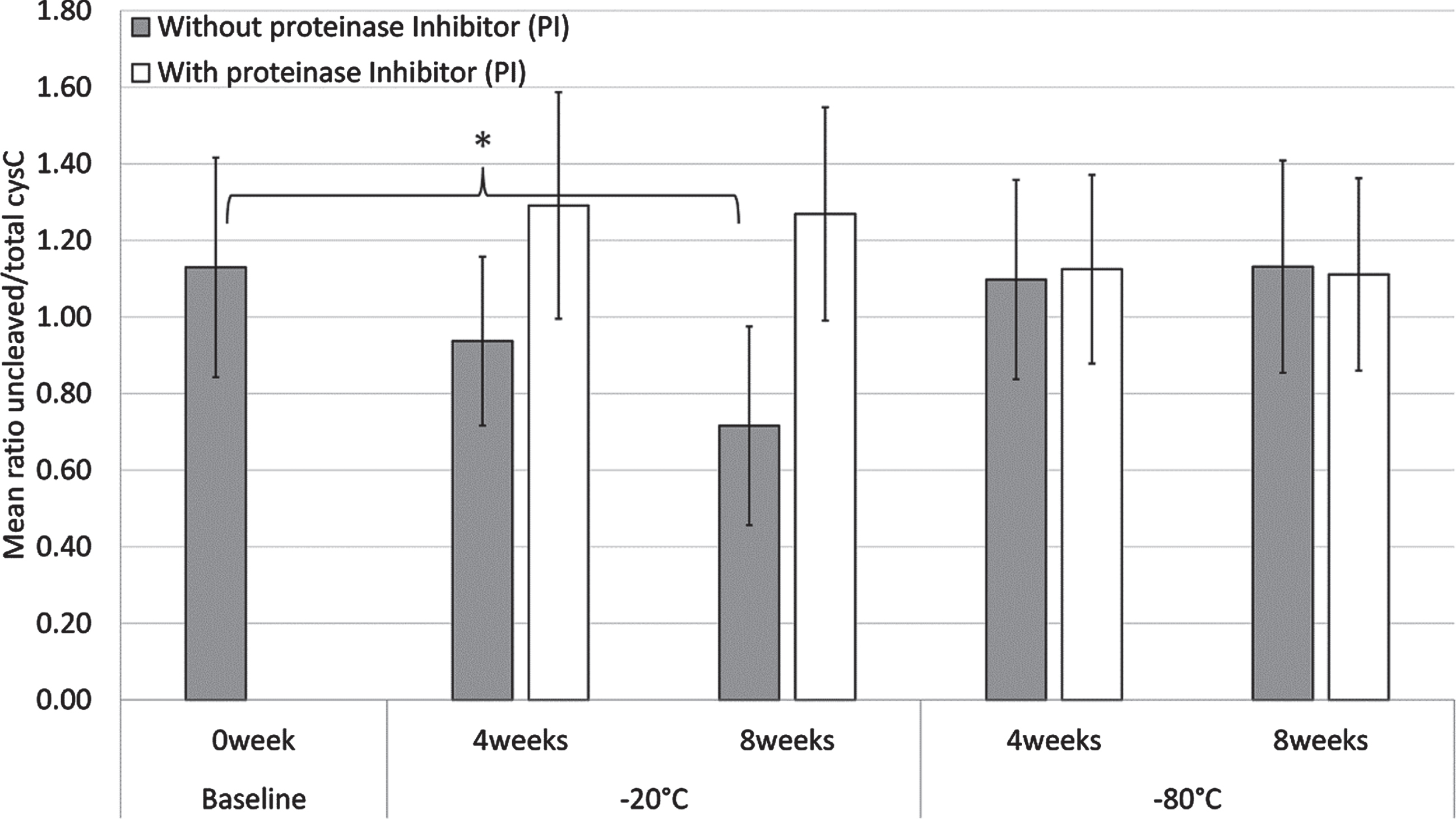
DISCUSSION
We developed an easily applicable tool for monitoring CSF sample quality using a novel double indirect sandwich ELISA. The assay, based on a monoclonal antibody that we produced, recognizes specifically the octapeptide-uncleaved form of cystatin C. Concerning the monoclonal antibody Cyst28, the sequences located in the N-terminal fragment do not correspond to the antibody binding site [22], thus allowing us to measure the truncation ratio of the protein. The intermediate reproducibility of this method is excellent with a CV of 10.9%for the cystatin C cleavage ratio values. The method is also accurate since the cystatin C concentration measurements overall are consistent with previously published concentration ranges. The concentration range of total cystatin C in the samples from the AD long-term storage cohort was 6.0–29.9 mg/L. These results are slightly higher than the range reported in literature [28, 29] but are in line with a study that reported a range of 2.7–18.5 mg/L by measuring cystatin C by ELISA in CSF from 15 patients with AD [30]. The difference in ranges of cystatin C levels across studies might relate to differences in study populations, as cystatin C shows a marked variation with age [31], and/or to the different assays that have been used in these studies.
Using this novel double indirect sandwich ELISA, our study confirmed the hypothesis that the cystatin C protein is cleaved when CSF is stored at –20°C, but not at –80°C or in LN. Our results show that cleavage of human native cystatin C occurs short-term (up to 4 months) and continued to mid-term (up to one year) when CSF is stored at –20°C but not when CSF is stored at –80°C. The result of short term decrease in cystatin C ratio in CSF stored at –20°C is in agreement with several previous studies [15–18]. Our study replicated previous mass spectrometry findings using an ELISA, which is easier to implement. We suggest this ELISA assay can be used for quality control purposes.
Our mid-term stability study showed a statistically significant increase in the cystatin C ratio after 6 months of CSF storage at –80°C and starting from 4 weeks of CSF storage in LN. Similarly, in our long-term stability study, the broad –80°C storage time range (4.0–16.4 years) also showed an increase in the cystatin C ratio over storage time. It is possible that the fragmented protein degrades more rapidly with time than the uncleaved protein. As the cleavage state is measured by the ratio between the uncleaved protein concentration and the total concentration (encompassing both the uncleaved and cleaved forms), this can explain the increase of the ratio with long-term storage at –80°C or in LN. Furthermore, the monoclonal antibody measuring “total cystatin C” is asserted to react with a conformational epitope of cystatin C encompassing residues 54K-60V, 85F-91L, 92K-99F, and 101I-111T. If other proteolytic events, apart from the cleavage of the 8R-9L peptide bond, take place over long-term storage at –80°C or in LN, this may also explain the observed increase of the ratio.
Although it has been shown that cystatin C has high affinity to Aβ in a saturable and concentration-dependent manner [10, 32, 33], our approach assumed consistency of cystatin C CSF levels over the years in which the samples had been collected, similar to what we observed for the AD CSF biomarkers Aβ, tTau, and pTau employing the same design of analysis in a homogenous AD patient cohort [27]. Furthermore, the long –80°C storage time and high sample number, enabled detection of long-term storage effects, as discussed above.
We observed that the protein ratio was stable after seven freeze-thawing cycles. A strong adsorption on plastic tube walls and/or pipet tips however was detected for the mean total (39%) and the mean uncleaved (41%) cystatin C levels. Many published studies showed that various tube material adsorb CSF biomarkers to different degrees [34]. Aβ is affected to a greater degree than Cystatin C. Indeed, a previous study has found 63%adsorption of Aβ using the same polypropylene storage containers as in this study [35]. The hydrophobic nature of the Aβ peptide has been hypothesized as a cause. The cystatin C mature active form contains 120 amino acid residues and is a single non-glycosylated polypeptide chain [28]. Cystatin C is characterized by three main domains interacting with the target enzymes: the N-terminal disordered segment (1S-10V) and the two hairpin loops L1 (55Q-59G) and L2 (105P-108G) [36, 37]. These three regions of the protein contain many hydrophobic residues, which may cause adsorption to tube walls [38].
We hypothesized that the mechanism of the native cystatin C cleavage may be due either to a physical (cryogenic storage-related artefact) or an enzymatic mechanism. The percentage inhibition of trypsin after immediate addition of the protease inhibitor cocktail is 93%, as reported by the manufacturer. Decrease of the mean ratio was seen only for CSF without PI, stored at –20°C, suggesting that the cleavage is indeed protease-related. The freeze-thaw experiments show that the proteolytic cleavage is not due to the freeze thaw event. Furthermore, the stability studies at –20°C show that the proteolytic cleavage occurs continuously and cumulatively over the storage duration at –20°C. Surprisingly, this cleavage was not detected after one week at RT or at 4°C. An explanation is that the native protein configuration at –20°C (which may differ from the RT, 4°C, –80°C or LN structure) may lead to enzymatic proteolytic cleavage. The N-terminal part of the cystatin C polypeptide chain is displayed on the surface of the protein and therefore may be subject to proteolytic attack [38]. In an epitope assessment experimental approach, a trypsin enzymatic digestion of cystatin C was carried out and weak signals that could be associated with the octapeptide-cleaved protein were detected by mass spectrometry [22]. An octapeptide cleavage of cystatin C has also been demonstrated by matrix metalloproteinase 2 [39]. A limited number of protein studies demonstrate that enzymes can be active even at sub-zero temperatures [40–42]. The cleavage of cystatin C is therefore most probably an enzymatic proteolytic cleavage linked to the protein configuration at –20°C.
One strength of our study is that preanalytical processing factors were tightly controlled during all experiments. The same protocols were used throughout the whole analysis. A limitation of this study is that the analysis for RT or 4°C storage was stopped after one week. We do not know if the cystatin C cleavage occurs under these conditions after longer storage durations.
As a conclusion, the novel double indirect sandwich ELISA method described here can be used to measure concentrations of the total and uncleaved protein, using a recombinant protein for the calibration curve, and further to calculate the cystatin C truncation ratio. It is known that there is a variability in analytical results of AD biomarkers [43, 44] and preanalytical factors, such as CSF collection, storage, and adsorption to tube-walls, have been shown to contribute the highest to this variability [45]. Concerning storage preanalytical variability, the CSF AD biomarkers are stable in –80°C storage [27], whereas freezing at –20°C seems to affect concentrations of different CSF biomarkers [27, 46]. We present a simple and validated ELISA assay that enables retrospective characterization of CSF biospecimens without the use of mass spectrometry. In case of CSF sample collections with different cystatin C ratios, the cystatin C ratio could be used as a covariable in biomarker discovery and validation studies. Often researchers are unaware of the storage history of the CSF samples they use. We believe that researchers can apply this assay to their CSF collections to confirm the samples are fit-for-purpose for AD research and avoid bias linked to collections of different storage histories.
In summary, analysis of cystatin C with this assay can help qualify historical CSF sample collections of undocumented preanalytics, avoid bias linked to different storage conditions of CSF samples and assess the cystatin C integrity prior to quantification, structural and functional activity studies.
ACKNOWLEDGMENTS
We thank L. Reisser-Rubrecht from Bio-Rad laboratories for the generation of monoclonal antibodies. G. Hamot is acknowledged for his help during the optimization phase of the double indirect sandwich ELISA. We are grateful to J.P. Trezzi for providing human CSF samples as internal quality control. The technical assistance on Friedman Test of G. Le Coroller is gratefully acknowledged. We thank W. Mathieson for critical review of the manuscript.
Authors’ disclosures available online (https://www.j-alz.com/manuscript-disclosures/21-0741).
REFERENCES
[1] | Tumani H , Huss A , Bachhuber F ((2017) ) The cerebrospinal fluid and barriers - anatomic and physiologic considerations. Handb Clin Neurol 146: , 21–32. |
[2] | Scheltens P , Blennow K , Breteler MM , de Strooper B , Frisoni GB , Salloway S , Van der Flier WM ((2016) ) Alzheimer’s disease. Lancet 388: , 505–517. |
[3] | Teunissen CE , Malekzadeh A , Leurs C , Bridel C , Killestein J ((2015) ) Body fluid biomarkers for multiple sclerosis–the long road to clinical application. Nat Rev Neurol 11: , 585–596. |
[4] | Betsou F , Bilbao R , Case J , Chuaqui R , Clements JA , De Souza Y , De Wilde A , Geiger J , Grizzle W , Guadagni F , Gunter E , Heil S , Kiehntopf M , Koppandi I , Lehmann S , Linsen L , Mackenzie-Dodds J , Aguilar Quesada R , Tebbakha R , Selander T , Shea K , Sobel M , Somiari S , Spyropoulos D , Stone M , Tybring G , Valyi-Nagy K , Wadhwa L (2018) Standard PREanalytical Code version 3.0. Biopreserv Biobank. |
[5] | Lippi G , Betsou F , Cadamuro J , Cornes M , Fleischhacker M , Fruekilde P , Neumaier M , Nybo M , Padoan A , Plebani M , Sciacovelli L , Vermeersch P , von Meyer A , Simundic AM ((2019) ) Preanalytical challenges - time for solutions. Clin Chem Lab Med 57: , 974–981. |
[6] | Willemse EA , Teunissen CE ((2015) ) Biobanking of cerebrospinal fluid for biomarker analysis in neurological diseases. Adv Exp Med Biol 864: , 79–93. |
[7] | Hok AHYS , Willemse EAJ , Teunissen CE , Del Campo M ((2019) ) Guidelines for CSF processing and biobanking: Impact on the identification and development of optimal CSF protein biomarkers. Methods Mol Biol 2044: , 27–50. |
[8] | Dubois B , Feldman HH , Jacova C , Hampel H , Molinuevo JL , Blennow K , DeKosky ST , Gauthier S , Selkoe D , Bateman R , Cappa S , Crutch S , Engelborghs S , Frisoni GB , Fox NC , Galasko D , Habert MO , Jicha GA , Nordberg A , Pasquier F , Rabinovici G , Robert P , Rowe C , Salloway S , Sarazin M , Epelbaum S , de Souza LC , Vellas B , Visser PJ , Schneider L , Stern Y , Scheltens P , Cummings JL ((2014) ) Advancing research diagnostic criteria for Alzheimer’s disease: The IWG-2 criteria. Lancet Neurol 13: , 614–629. |
[9] | Levy E , Sastre M , Kumar A , Gallo G , Piccardo P , Ghetti B , Tagliavini F ((2001) ) Codeposition of cystatin C with amyloid-beta protein in the brain of Alzheimer disease patients. J Neuropathol Exp Neurol 60: , 94–104. |
[10] | Sastre M , Calero M , Pawlik M , Mathews PM , Kumar A , Danilov V , Schmidt SD , Nixon RA , Frangione B , Levy E ((2004) ) Binding of cystatin C to Alzheimer’s amyloid beta inhibits in vitro amyloid fibril formation. Neurobiol Aging 25: , 1033–1043. |
[11] | Mueller-Steiner S , Zhou Y , Arai H , Roberson ED , Sun B , Chen J , Wang X , Yu G , Esposito L , Mucke L , Gan L ((2006) ) Antiamyloidogenic and neuroprotective functions of cathepsin B: Implications for Alzheimer’s disease. Neuron 51: , 703–714. |
[12] | Wang C , Sun B , Zhou Y , Grubb A , Gan L ((2012) ) Cathepsin B degrades amyloid-β in mice expressing wild-type human amyloid precursor protein. J Biol Chem 287: , 39834–39841. |
[13] | Mason RW , Sol-Church K , Abrahamson M ((1998) ) Amino acid substitutions in the N-terminal segment of cystatin C create selective protein inhibitors of lysosomal cysteine proteinases. Biochem J 330: (Pt 2), 833–838. |
[14] | Hall A , Ekiel I , Mason RW , Kasprzykowski F , Grubb A , Abrahamson M ((1998) ) Structural basis for different inhibitory specificities of human cystatins C and D. Biochemistry 37: , 4071–4079. |
[15] | Carrette O , Burkhard PR , Hughes S , Hochstrasser DF , Sanchez JC ((2005) ) Truncated cystatin C in cerebrospiral fluid: Technical [corrected] artefact or biological process? Proteomics 5: , 3060–3065. |
[16] | Berven FS , Kroksveen AC , Berle M , Rajalahti T , Flikka K , Arneberg R , Myhr KM , Vedeler C , Kvalheim OM , Ulvik RJ ((2007) ) Pre-analytical influence on the low molecular weight cerebrospinal fluid proteome. Proteomics Clin Appl 1: , 699–711. |
[17] | Del Boccio P , Pieragostino D , Lugaresi A , Di Ioia M , Pavone B , Travaglini D , D’Aguanno S , Bernardini S , Sacchetta P , Federici G , Di Ilio C , Gambi D , Urbani A ((2007) ) Cleavage of cystatin C is not associated with multiple sclerosis. Ann Neurol 62: , 201–204; discussion 205. |
[18] | Jimenez CR , Koel-Simmelink M , Pham TV , van der Voort L , Teunissen CE ((2007) ) Endogeneous peptide profiling of cerebrospinal fluid by MALDI-TOF mass spectrometry: Optimization of magnetic bead-based peptide capture and analysis of preanalytical variables. Proteomics Clin Appl 1: , 1385–1392. |
[19] | Hansson SF , Simonsen AH , Zetterberg H , Andersen O , Haghighi S , Fagerberg I , Andréasson U , Westman-Brinkmalm A , Wallin A , Rüetschi U , Blennow K ((2007) ) Cystatin C in cerebrospinal fluid and multiple sclerosis. Ann Neurol 62: , 193–196; discussion 205. |
[20] | Irani DN , Anderson C , Gundry R , Cotter R , Moore S , Kerr DA , McArthur JC , Sacktor N , Pardo CA , Jones M , Calabresi PA , Nath A ((2006) ) Cleavage of cystatin C in the cerebrospinal fluid of patients with multiple sclerosis. Ann Neurol 59: , 237–247. |
[21] | Greco V , Pieragostino D , Piras C , Aebersold R , Wiltfang J , Caltagirone C , Bernardini S , Urbani A ((2014) ) Direct analytical sample quality assessment for biomarker investigation: Qualifying cerebrospinal fluid samples. Proteomics 14: , 1954–1962. |
[22] | Behrendt I , Pradzinska M , Spodzieja M , Kolodziejczyk AS , Rodziewicz-Motowidlo S , Szymanska A , Czaplewska P ((2016) ) Epitope location for two monoclonal antibodies against human cystatin C, representing opposite aggregation inhibitory properties. Amino Acids 48: , 1717–1729. |
[23] | Abraham JD , Prome S , Salvetat N , Rubrecht L , Cobo S , du Paty E , Galea P , Mathieu-Dupas E , Ranaldi S , Caillava C , Cremer GA , Rieunier F , Robert P , Molina F , Laune D , Checler F , Fareh J ((2013) ) Cerebrospinal Abeta11-x and 17-x levels as indicators of mild cognitive impairment and patients’ stratification in Alzheimer’s disease. Transl Psychiatry 3: , e281. |
[24] | van der Flier WM , Pijnenburg YA , Prins N , Lemstra AW , Bouwman FH , Teunissen CE , van Berckel BN , Stam CJ , Barkhof F , Visser PJ , van Egmond E , Scheltens P ((2014) ) Optimizing patient care and research: The Amsterdam Dementia Cohort. J Alzheimers Dis 41: , 313–327. |
[25] | Mulder C , Verwey NA , van der Flier WM , Bouwman FH , Kok A , van Elk EJ , Scheltens P , Blankenstein MA ((2010) ) Amyloid-beta(1-42), total tau, and phosphorylated tau as cerebrospinal fluid biomarkers for the diagnosis of Alzheimer disease. Clin Chem 56: , 248–253. |
[26] | Andreasson U , Perret-Liaudet A , van Waalwijk van Doorn LJ , Blennow K , Chiasserini D , Engelborghs S , Fladby T , Genc S , Kruse N , Kuiperij HB , Kulic L , Lewczuk P , Mollenhauer B , Mroczko B , Parnetti L , Vanmechelen E , Verbeek MM , Winblad B , Zetterberg H , Koel-Simmelink M , Teunissen CE ((2015) ) A practical guide to immunoassay method validation. Front Neurol 6: , 179. |
[27] | Willemse EAJ , van Uffelen KWJ , van der Flier WM , Teunissen CE ((2017) ) Effect of long-term storage in biobanks on cerebrospinal fluid biomarker Abeta1-42, T-tau, and P-tau values. Alzheimers Dement (Amst) 8: , 45–50. |
[28] | Mussap M , Plebani M ((2004) ) Biochemistry and clinical role of human cystatin C. Crit Rev Clin Lab Sci 41: , 467–550. |
[29] | Yamamoto-Watanabe Y , Watanabe M , Jackson M , Akimoto H , Sugimoto K , Yasujima M , Wakasaya Y , Matsubara E , Kawarabayashi T , Harigaya Y , Lyndon AR , Shoji M ((2010) ) Quantification of cystatin C in cerebrospinal fluid from various neurological disorders and correlation with G73A polymorphism in CST3. Brain Res 1361: , 140–145. |
[30] | Mares J , Kanovsky P , Herzig R , Stejskal D , Vavrouskova J , Hlustik P , Vranova H , Burval S , Zapletalova J , Pidrman V , Obereigneru R , Suchy A , Vesely J , Podivinsky J , Urbanek K ((2009) ) The assessment of beta amyloid, tau protein and cystatin C in the cerebrospinal fluid: Laboratory markers of neurodegenerative diseases. Neurol Sci 30: , 1–7. |
[31] | Grubb A , Lofberg H ((1985) ) Human gamma-trace. Structure, function and clinical use of concentration measurements. Scand J Clin Lab Invest Suppl 177: , 7–13. |
[32] | Mi W , Pawlik M , Sastre M , Jung SS , Radvinsky DS , Klein AM , Sommer J , Schmidt SD , Nixon RA , Mathews PM , Levy E ((2007) ) Cystatin C inhibits amyloid-beta deposition in Alzheimer’s disease mouse models. Nat Genet 39: , 1440–1442. |
[33] | Gauthier S , Kaur G , Mi W , Tizon B , Levy E ((2011) ) Protective mechanisms by cystatin C in neurodegenerative diseases. Front Biosci (Schol Ed) 3: , 541–554. |
[34] | Hansson O , Mikulskis A , Fagan AM , Teunissen C , Zetterberg H , Vanderstichele H , Molinuevo JL , Shaw LM , Vandijck M , Verbeek MM , Savage M , Mattsson N , Lewczuk P , Batrla R , Rutz S , Dean RA , Blennow K ((2018) ) The impact of preanalytical variables on measuring cerebrospinal fluid biomarkers for Alzheimer’s disease diagnosis: A review. Alzheimers Dement 14: , 1313–1333. |
[35] | Kofanova OA , Mommaerts K , Betsou F ((2015) ) Tube polypropylene: A neglected critical parameter for protein adsorption during biospecimen storage. Biopreserv Biobank 13: , 296–298. |
[36] | Wallin H , Abrahamson M , Ekstrom U ((2013) ) Cystatin C properties crucial for uptake and inhibition of intracellular target enzymes. J Biol Chem 288: , 17019–17029. |
[37] | Abrahamson M , Mason RW , Hansson H , Buttle DJ , Grubb A , Ohlsson K ((1991) ) Human cystatin C. role of the N-terminal segment in the inhibition of human cysteine proteinases and in its inactivation by leucocyte elastase. Biochem J 273: (Pt 3), 621–626. |
[38] | Ekiel I , Abrahamson M , Fulton DB , Lindahl P , Storer AC , Levadoux W , Lafrance M , Labelle S , Pomerleau Y , Groleau D , LeSauteur L , Gehring K ((1997) ) NMR structural studies of human cystatin C dimers and monomers. J Mol Biol 271: , 266–277. |
[39] | Dean RA , Butler GS , Hamma-Kourbali Y , Delbé J , Brigstock DR , Courty J , Overall CM ((2007) ) Identification of candidate angiogenic inhibitors processed by matrix metalloproteinase 2 (MMP-2) in cell-based proteomic screens: Disruption of vascular endothelial growth factor (VEGF)/heparin affin regulatory peptide (pleiotrophin) and VEGF/Connective tissue growth factor angiogenic inhibitory complexes by MMP-2 proteolysis. Mol Cell Biol 27: , 8454–8465. |
[40] | Rasmussen BF , Stock AM , Ringe D , Petsko GA ((1992) ) Crystalline ribonuclease A loses function below the dynamical transition at 220K. Nature 357: , 423–424. |
[41] | Tilton RF Jr. , Dewan JC , Petsko GA ((1992) ) Effects of temperature on protein structure and dynamics: X-ray crystallographic studies of the protein ribonuclease-A at nine different temperatures from 98 to 320K. Biochemistry 31: , 2469–2481. |
[42] | More N , Daniel RM , Petach HH ((1995) ) The effect of low temperatures on enzyme activity. Biochem J 305: (Pt 1), 17–20. |
[43] | Mattsson N , Andreasson U , Persson S , Carrillo MC , Collins S , Chalbot S , Cutler N , Dufour-Rainfray D , Fagan AM , Heegaard NH , Robin Hsiung GY , Hyman B , Iqbal K , Kaeser SA , Lachno DR , Lleó A , Lewczuk P , Molinuevo JL , Parchi P , Regeniter A , Rissman RA , Rosenmann H , Sancesario G , Schröder J , Shaw LM , Teunissen CE , Trojanowski JQ , Vanderstichele H , Vandijck M , Verbeek MM , Zetterberg H , Blennow K ((2013) ) CSF biomarker variability in the Alzheimer’s Association quality control program. Alzheimers Dement 9: , 251–261. |
[44] | Vos SJ , Visser PJ , Verhey F , Aalten P , Knol D , Ramakers I , Scheltens P , Rikkert MG , Verbeek MM , Teunissen CE ((2014) ) Variability of CSF Alzheimer’s disease biomarkers: Implications for clinical practice. PLoS One 9: , e100784. |
[45] | Plebani M ((2006) ) Errors in clinical laboratories or errors in laboratory medicine? Clin Chem Lab Med 44: , 750–759. |
[46] | Le Bastard N , De Deyn PP , Engelborghs S ((2015) ) Importance and impact of preanalytical variables on Alzheimer disease biomarker concentrations in cerebrospinal fluid. Clin Chem 61: , 734–743. |