Cognitive Effects of Aerobic Exercise in Alzheimer’s Disease: A Pilot Randomized Controlled Trial
Abstract
Background:
Aerobic exercise has shown inconsistent cognitive effects in older adults with Alzheimer’s disease (AD) dementia.
Objective:
To examine the immediate and longitudinal effects of 6-month cycling on cognition in older adults with AD dementia.
Methods:
This randomized controlled trial randomized 96 participants (64 to cycling and 32 to stretching for six months) and followed them for another six months. The intervention was supervised, moderate-intensity cycling for 20–50 minutes, 3 times a week for six months. The control was light-intensity stretching. Cognition was assessed at baseline, 3, 6, 9, and 12 months using the AD Assessment Scale-Cognition (ADAS-Cog). Discrete cognitive domains were measured using the AD Uniform Data Set battery.
Results:
The participants were 77.4±6.8 years old with 15.6±2.9 years of education, and 55% were male. The 6-month change in ADAS-Cog was 1.0±4.6 (cycling) and 0.1±4.1 (stretching), which were both significantly less than the natural 3.2±6.3-point increase observed naturally with disease progression. The 12-month change was 2.4±5.2 (cycling) and 2.2±5.7 (control). ADAS-Cog did not differ between groups at 6 (p = 0.386) and 12 months (p = 0.856). There were no differences in the 12-month rate of change in ADAS-Cog (0.192 versus 0.197, p = 0.967), memory (–0.012 versus –0.019, p = 0.373), executive function (–0.020 versus –0.012, p = 0.383), attention (–0.035 versus –0.033, p = 0.908), or language (–0.028 versus –0.026, p = 0.756).
Conclusion:
Exercise may reduce decline in global cognition in older adults with mild-to-moderate AD dementia. Aerobic exercise did not show superior cognitive effects to stretching in our pilot trial, possibly due to the lack of power.
INTRODUCTION
Alzheimer’s disease (AD) affected 5.8 million Americans at a cost of $209 billion in 2019 and will reach an estimated 14 million Americans and $1.2 trillion in 2050 [1]. While disease-modifying drugs for AD are still lacking, aerobic exercise has been shown to favorably modify the accumulation, degradation, and removal of AD hallmark amyloid-β and hyperphosphorylated tau in animal models [2, 3]. Aerobic exercise also appears to impact AD non-hallmark pathology such as neuroinflammation, oxidative stress, and glucose hypometabolism [2, 3]. Despite these mechanistic supports, aerobic exercise has shown inconsistent cognitive effects in randomized controlled trials (RCTs) across the AD spectrum [4–12].
The inconsistent findings are attributable to meth-odological differences such as disease stage studied (mild cognitive impairment [MCI] versus AD dementia) and exercise modality (e.g., aerobic exercise only or combined exercises, walking or cycling) and dose variations (i.e., frequency, duration, and intensity) [4–7, 10–13]. Aerobic exercise has been shown to improve executive function and memory in individuals with MCI [8, 14]. In older adults with AD dementia, between-group differences in cognitive changes ranged from negligible [10, 11, 13] to moderate [4–7]. Even when between-group differences in cognition were not demonstrated, cognition appeared to stabilize in the intervention group, but continued to decline in the control group [10, 11]. Overall, meta-analyses of RCTs suggest that aerobic exercise has modest-to-moderate cognitive effects across the AD spectrum [15, 16]. Recent evidence further supports a potential dose-response relationship between aerobic exercise and cognition in MCI and AD dementia [10, 11].
To address these inconsistent findings, we conducted a pilot RCT to evaluate a rigorous 6-month, supervised aerobic exercise-only intervention relative to stretching control in older adults with AD dementia. The intervention was individualized moderate intensity cycling on recumbent stationary cycles to ensure proper dose delivery. We chose cycling above other modalities (e.g., walking) because cycling on recumbent cycles is non-weight-bearing and easy to do for a cohort with a high prevalence of knee and hip arthritis. Additionally, cycling eliminates the confounding factors of motor dysfunction and gait impairment that are both common among people with AD, can affect gait and walking stability, and limit aerobic exercise performance. Cycling on recumbent stationary cycles is powered by participants’ legs instead of electricity, which adds a safety feature because of the high prevalence of behavioral and psychological symptoms of dementia, preventing falls or other injuries related to electricity-powered exercise modes such as treadmills or elliptical machines. The objective of this pilot trial was to investigate the immediate and long-term effects of 6-month aerobic cycling on cognition in community-dwelling older adults with clinically defined probable mild-to-moderate AD dementia. Given the pilot nature, this trial was not powered to detect between-group differences. Instead, it was powered on the a priori hypothesis that cycling participants would have a smaller within-group increase in global cognition as measured by the AD Assessment Scale-Cognition (ADAS-Cog) at six months as compared to the natural, expected 3.2±6.3-point increase. The secondary hypothesis was that cycling participants would show a smaller increase in ADAS-Cog scores over 12 months compared to stretching participants. These hypotheses have been published previously [17]. In addition, we further examined the trajectory of changes in episodic memory, executive function, attention, processing speed, and language over 12 months between the two groups.
MATERIALS AND METHODS
This pilot RCT used a 2-parallel group design to randomize 96 participants to 6-month supervised cycling or stretching on a 2:1 allocation ratio. Participants were followed for another six months. Randomization was stratified by age (age 66–75, 76–85, and 85 + years) using random permuted blocks of 3 and 6. Allocation was concealed from all investigators and data collectors, except for statistician DV who generated the randomization sequence. The randomization sequence was concealed in opaque envelop and locked in the interventionist’s office. This trial was approved by the University of Minnesota Institutional Review Board (IRB: #1306M35661). Details of the trial protocol have been previously published (NCT01954550) [17].
Setting
In-person screening, data collections, and cycle-ergometer tests were performed on the university campus. Exercises were delivered in a YMCA gym or senior community. After the trial commencement, we added exercise sites in year 3. The non-blinded staff provided transportation for participants to attend study activities and supervised exercise delivery.
Participants
Community-dwelling older adults 66 + years old with AD dementia verified by providers and by clinician experts per 2011 diagnostic criteria [18] were eligible if their Mini-Mental State Examination (MMSE) and Clinical Dementia Rating (CDR) was 15–26 and 0.5–2, respectively, took AD drugs>1 month if prescribed, received provider clearance for exercise safety, and spoke English. Individuals were excluded who had a resting heart rate<50 or>100 beats per minute, neurologic disorders (e.g., non-AD dementia), psychiatric disorders (e.g., schizophrenia), alcohol or chemical dependency, exercise contraindications, new symptoms or diseases that had not been evaluated by providers, abnormal findings from the cycler-ergometer test or magnetic resonance imaging (MRI). Electrocardiograms from the cycle-ergometer test were independently read by the study physicians (LSC) and exercise physiologist (DS). MRI was processed and read for abnormality by the study radiologist and his team at Mayo Clinic. After the trial commencement, we removed investigator consensus diagnosis from inclusion criteria in year 2 to improve efficiency as few cases were disqualified. Instead, the principal investigator (FY) confirmed the accuracy of provider-verified AD dementia by reviewing the summary of each potential participant using data abstracted from medical records (if available) and collected during the screening (e.g., health history, diagnoses, medications, and scores on cognitive and functional instruments) and, if questionable, consulted with the study neuropsychologist (NWN) and geriatrician (MD).
Master’s-level staff in neuroscience (project manager) and exercise science (interventionist) were trained by the PI and used a multi-pronged recruitment approach and potential participants initiated their contact with the staff (published previously) [17]. Recruitment began in March 2014 and ended in March 2018. The trial ended when the last data collection was completed in October 2020. Based on assessment of the individual’s decision-making capacity, participants gave informed consent or assent with surrogate consent. Re-consent was conducted before each of the subsequent data collections. Decision-capacity was assessed to determine if self or surrogate consent was needed. The project manager provided a summary of a participant’s eligibility to the team (PI, exercise physiologist, and interventionist) to confirm eligibility and then enroll the participant. After completing baseline data collection, the participant was enrolled by the project manager. The interventionist then opened the randomization envelop sequentially within each stratum to reveal the participant’s group allocation.
The target sample size was 90, which was powered on the within-group, 6-month change in ADAS-Cog in the intervention group, in comparison to the mean 3.2-point increase from placebo groups in AD drug RCTs (N = 536; standard deviation [SD] = 6.25) [19]. Assuming 2-sided t-test at 0.05, 16% attrition at six months, and intention-to-treat, our target sample size had 80% power to detect a significant difference in ADAS-Cog [17]. After meeting our enrollment goal in year 4, we increased the sample size to 96 to allow individuals in the screening process to continue, which was approved by the Data Safety and Monitoring Board, NIA, and IRB.
Intervention
The cycling intervention was prescribed at 50–75% of heart rate reserve (HRR) or 9–15 on the 6–20 Borg Ratings of Perceived Exertion (RPE) scale for 20–50 min a session, three times a week for six months (72 sessions). An interventionist supervised each session which lasted 40–60 min after adding 5 min warm-up and 5 min cool-down. Sessions were delivered over 27 weeks to account for 3-month data collection, vacations, and illnesses. HR was continuously monitored with Precor HR monitor and the proper use of RPE was continuously reinforced. In week 1, participants started at 50–55% of HRR or RPE 9–11 for 20–30 min as tolerated. In week 2, participants maintained the same intensity as that in week 1, but the session duration was increased by five min to 30–40 min. In week 3, the session duration was held the same as that in week 2, but intensity was increased by 5% of HRR or 1-point on RPE to 55–60% of HRR or RPE 10–12. These alternative increases in session duration and intensity were continued until the targets of 70–75% HRR (or RPE 12–14) and 50 min were achieved, which typically occurred in week 10.
The control exercise was stretching and range-of-motion prescribed at the same frequency, session duration, and program duration as cycling, but at low intensity (<20% of HRR or<RPE 9). It included primarily seated movements and static stretches. The number of repetitions and durations for each stretch was gradually increased to match cycling session durations [17]. After the trial commencement, we increased interventionist-to-participants ratio from 1:2 and 2:3 to 1:3 in year 2 to efficiently and safely deliver sessions.
Main outcomes and measures
The primary outcome was global cognition as measured by the ADAS-Cog. Secondary outcomes (episodic memory, executive function, attention, processing speed, and language) were primarily measured using the AD Centers’ Uniform Data Set neuropsychological test battery [20]. Alternative forms of an instrument, if available, were used to reduce practice effects. Cognition was assessed prior to randomization and post-randomization at 3, 6, 9, and 12 months.
Global cognition was measured using the ADAS-Cog, which is used widely used in AD drug RCTs [21]. It assesses orientation, memory, recall, language, and praxis with a total score of 0–70 (higher score = worse function) with 0.65–0.99 interrater reliability and 0.51–1.0 test-retest reliability [21].
Episodic memory was evaluated with the Wechsler Memory Scale–Revised Logical Memory subtest [22] and Hopkins Verbal Learning Test–Revised [23]. Executive function was assessed with the Trail Making Test (TMT) Part B [24], Exit Interview-25 (EXIT-25) [25], and Executive Clock Drawing Task [26]. Attention was assessed with the Wechsler Adult Intelligence Scale–Revised (WAIS-R) Digit Span tests [27]. Processing speed was measured with the WAIS-Third Edition Digit Symbol test [22], Golden Stroop [28], and TMT Part A [24]. Language was assessed with the Controlled Oral Word Association test [29], Category Fluency test [30], and Boston Naming Test [29].
Covariates at baseline included dementia severity as measured by the MMSE. Premorbid intellect was measured using the Wechsler Test of Adult Reading [31]. Behavioral and psychological symptoms of dementia (BPSD) were measured by the Neuropsychiatric Inventory-Caregiver [32]. ADL was measured by the Disability Assessment for Dementia [33]. Body mass index was calculated from weight and height. Use of AD drug (yes/no), comorbidities (# of chronic conditions), and demographics (age, sex, race, education, marital status, and living arrangement) were collected using a questionnaire. Exercise adherence was objectively determined. During each exercise session, the interventionist documented participants’ heart rates and RPE every 5 min on the Session Report Form. Heart rates, RPE, and durations in each session were used to define adherence in three ways: 1) the percent of 72 prescribed sessions attended, 2) the percent of attended sessions that met both the session intensity and duration prescription, and 3) per-protocol adherence that was defined as attending >70% sessions and >70% of attended sessions meeting the session intensity and duration prescription.
Statistical analyses
ADAS-Cog raw scores were used. Composite z-scores were created for discrete cognitive domains, following the established procedures [34, 35]: 1) the directions of scores were reversed when lower raw scores are equivalent to better cognition (TMT Part A, TMT Part B, and EXIT-25), so that higher scores signify better cognition [34]; 2) raw scores were transformed to z-scores using the mean and SD of an instrument at baseline in the whole sample (n = 96): z-score = (raw score –baseline mean) / baseline SD; and 3) z-scores of all measures were averaged to create a domain-specific composite z-score.
Descriptive statistics were summarized as means (SD: standard deviation) for continuous variables or frequency (percentage) for categorical variables. Baseline differences between the groups were tested using Wilcoxon rank sum tests (continuous covariates) or Pearson chi-square tests (categorical covariates). The primary hypothesis was analyzed using 2-sided t-test of the mean change for each randomization group, in comparison to the 3.2-point increase expected with the natural disease course. The 6- and 12-month changes in cognition were compared between the two groups using two-sample t-tests.
The longitudinal trajectory of cognitive outcomes over 12-month (five data collection visits every three months) was assessed using mixed-effects models which include fixed effects for treatment group, (a function of) time, and their interaction and random participant-specific intercepts, and residuals with an AR(1) autoregressive correlation structure. This model allows one to estimate the average rates of change in outcomes within and between groups. All inferential analyses were conducted following the intention-to-treat principle [17].
All analyses were conducted using R Version 3.5.1 (R Foundation for Statistical Computing; Vienna, Austria) and two-sided tests with p < 0.05 indicating statistical significance. No adjustment for multiple testing was performed. Patterns of missing values were evaluated but were not imputed by last-observation-carried-forward to avoid biasing mean changes toward zero.
RESULTS
Potential participants were screened by phone (n = 301), in-person (n = 175), medical clearance (n = 132), and exercise testing (n = 117). Ninety-six met eligibility criteria and were enrolled: 64 randomized to cycling and 32 to stretching (Fig. 1). The attrition rate for the ADAS-Cog was 17.7% at 6 months (17.2% cycling versus 18.8% stretching) and 25% at 12 months (26.6% cycling versus 25% stretching; Fig. 2). Overall, 55% of the participants were male and 94% were non-Hispanic white with an average 15.6± 2.9 years of education (Table 1).
Fig. 1
Enrollment CONSORT Diagram for the FIT-AD Trial.
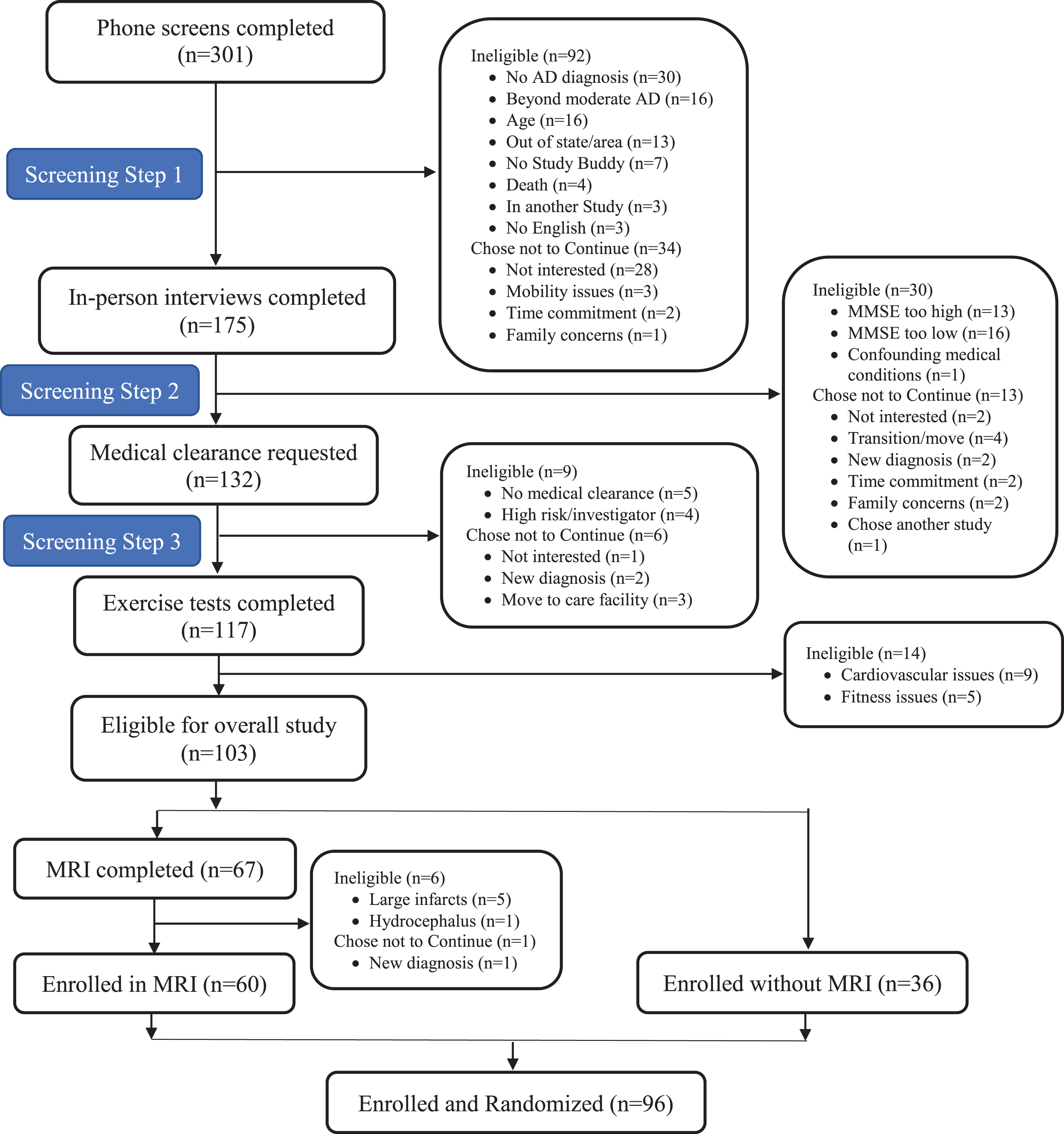
Fig. 2
CONSORT Diagram for the ADAS-Cog.
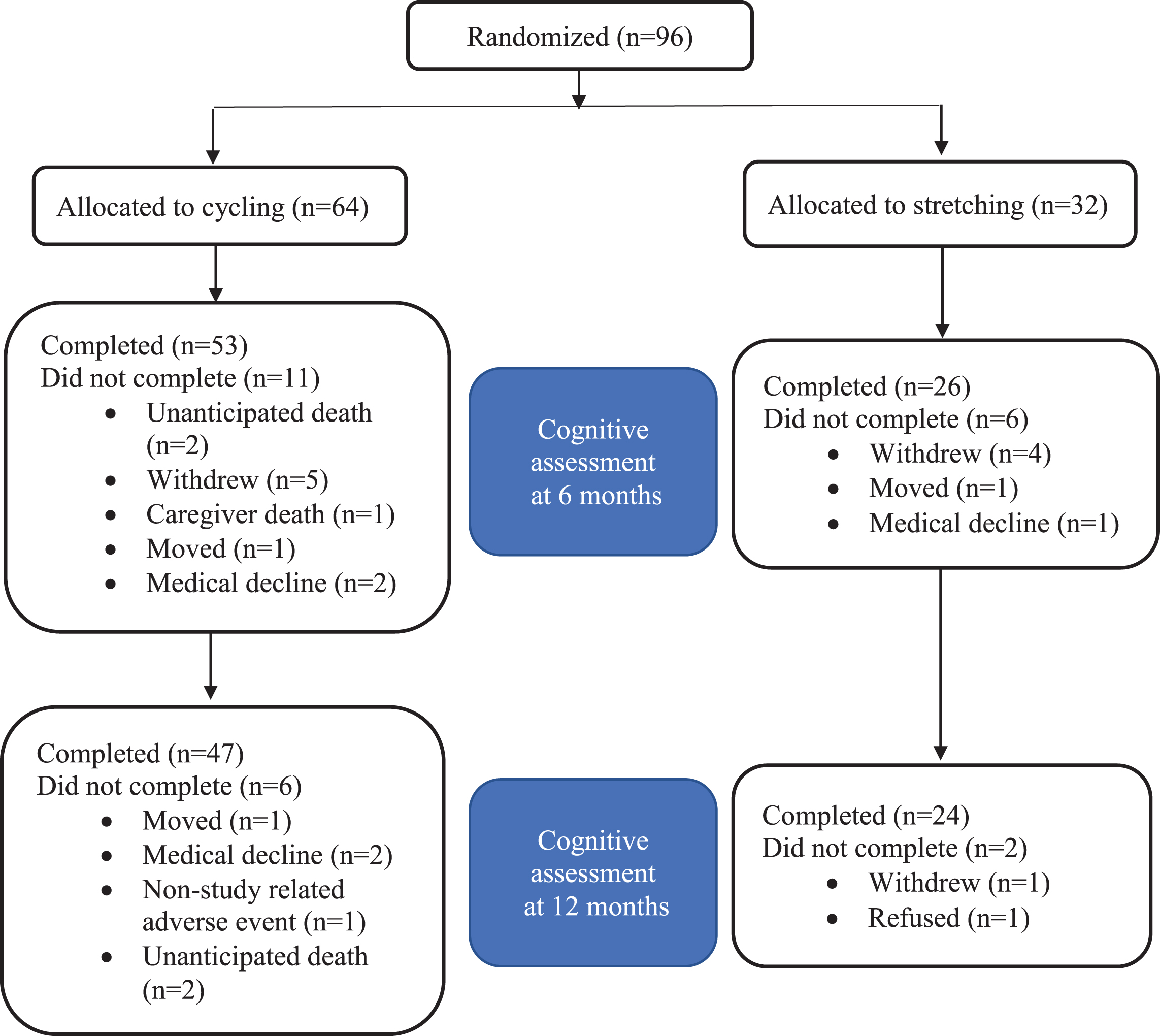
Table 1
Characteristics of the study sample at baseline (n = 96)
Overall (n = 96) Mean±SD or n (%) | Cycling (n = 64) Mean±SD or n (%) | Stretching (n = 32) Mean±SD or n (%) | p | |
Demographics | ||||
Age, y | 77.4±6.8 | 77.4±6.6 | 77.5±7.1 | 0.924 |
Sex | 0.772 | |||
Male | 53 (55) | 36 (56) | 17 (53) | |
Female | 43 (45) | 28 (44) | 15 (47) | |
Race/Ethnicity | 0.202 | |||
non-Hispanic White | 90 (94) | 62 (97) | 28 (88) | |
Hispanic White | 3 (3) | 1 (2) | 2 (6) | |
Black or African American | 3 (3) | 1 (2) | 2 (6) | |
Education, years completed | 15.6±2.9 | 15.5±2.8 | 15.8±3 | 0.636 |
Marital Status | 0.499 | |||
Married | 69 (72) | 46 (72) | 23 (72) | |
Divorced | 8 (8) | 6 (9) | 2 (6) | |
Widowed | 15 (16) | 9 (14) | 6 (19) | |
Live as married | 1 (1) | 0 (0) | 1 (3) | |
Never married | 3 (3) | 3 (5) | 0 (0) | |
Living arrangement | 0.353 | |||
Alone | 17 (18) | 13 (20) | 4 (13) | |
With spouse/partner only | 62 (65) | 41 (64) | 21 (66) | |
With spouse/partner &other | 5 (5) | 2 (3) | 3 (9) | |
With family | 11 (11) | 8 (13) | 3 (9) | |
Other | 1 (1) | 0 (0) | 1 (3) | |
Clinical Indicators | ||||
Dementia severity (MMSE) | 21.4±3.3 | 21±3.5 | 22.2±2.7 | 0.081 |
Body Mass Index | 27.7±5 | 27.8±4.7 | 27.4±5.6 | 0.755 |
AD medication | 0.310 | |||
Any AD medication | 53 (55) | 33 (52) | 20 (63) | |
Not used | 43 (45) | 31 (48) | 12 (38) | |
# comorbidities | 3.2±1.8 | 3.5±1.8 | 2.6±1.7 | 0.018* |
NPI-Q symptom presence | 3.8±2.1 | 4.2±2 | 2.9±2.1 | 0.010* |
NPI-Q severity | 5.9±4.2 | 6.6±4.2 | 4.4±3.8 | 0.012* |
NPI-Q caregiver distress | 8.2±6.1 | 9.2±5.8 | 6.2±6.4 | 0.030* |
Hostile mood | 14.2±4.2 | 14.9±4.5 | 12.9±3.1 | 0.017* |
Content mood | 19±3.6 | 18.5±3.4 | 20±3.8 | 0.075 |
ADL (DAD percent score) | 74.86±16.48 | 73.86±15.43 | 76.87±18.52 | 0.438 |
Premorbid intellect (WTAR) | 34.2±10.8 | 34.8±10.6 | 33.0±11.4 | 0.457 |
Cognitive Outcomes | ||||
Global cognition (ADAS-Cog) | 18.8±7.1 | 19.3±7.4 | 17.8±6.5 | 0.350 |
Episodic Memory | ||||
LM immediate recall | 8.5±6.3 | 8.2±5.8 | 9.2±7.3 | 0.524 |
LM delayed recall | 3.2±5.2 | 3±4.3 | 3.6±6.7 | 0.685 |
HVLT-R immediate recall | 11.1±5.2 | 11.4±5.3 | 10.7±5.1 | 0.538 |
HVLT-R delayed recall | 0.4±1.4 | 0.3±1.3 | 0.5±1.7 | 0.697 |
Executive function | ||||
TMT Part B | 217.5±83.8 | 217.5±86.8 | 217.3±78.8 | 0.991 |
EXIT-25 | 13.5±6 | 13.2±6.2 | 14.2±5.5 | 0.416 |
Executive Clock Drawing test | 8.3±3.8 | 8.3±3.8 | 8.3±3.9 | 1.000 |
Attention | ||||
Digit Span Forward & Backward | 12.2±3.5 | 12.3±3.6 | 12.1±3.2 | 0.701 |
Processing speed | ||||
Wechsler Digit Symbol | 32.8±16.7 | 33.3±17.3 | 31.8±15.6 | 0.687 |
TMT Part A | 83.9±60.8 | 84.6±64.3 | 82.5±54.2 | 0.868 |
Language | ||||
COWAT | 28.1±11.7 | 28.6±11.7 | 27.1±11.8 | 0.558 |
Category Fluency | 20.6±9.3 | 20.7±9.9 | 20.6±8.1 | 0.961 |
Boston Naming Test | 40.8±13.8 | 41.4±14.2 | 39.7±13.3 | 0.575 |
ADAS-Cog, Alzheimer’s Disease Assessment Scale-Cognition; BPSD, Behavioral and Psychological Symptoms of Dementia; COWAT, Controlled Oral Word Association Test; DAD, Disability in Alzheimer’s Disease; EXIT-25, Executive Interview-25; HVLT-R, Hopkins Verbal Learning Test-Revised; LM, Logical Memory; MMSE, Mini-Mental State Examination; NPI-Q, Neuropsychiatric Inventory-Questionnaire; TMT, Trail Making Test; WTAR, Wechsler Test of Adult Reading. The p-values for continuous outcomes are from two-sample t tests; the p-values for categorical variables are from Fisher exact tests. *p < 0.05.
A priori hypothesis on the 6-month effect of cycling on ADAS-Cog
Our primary hypothesis that cycling participants will have a smaller within-group increase in ADAS-Cog at six months (1.0±4.6) than the natural, expected 3.2± 6.3-point increase was supported (p = 0.001).
Secondary hypothesis on the 12-month change in global cognition
Our secondary hypothesis that cycling participants would show a smaller increase in the ADAS-Cog over 12 months as compared to stretching participants was not supported. The 12-month change in ADAS-Cog was 2.4±5.2 for cycling and 2.2±5.7 for stretching. There were no significant differences in ADAS-Cog at 6 and 12 months between groups (Table 2).
Table 2
Adjusted 6- and 12-month changes in the ADAS-Cog and composite scores
Cycling | Stretching | Between-group difference | |||
Measure | Mean±SEM | p | Mean±SEM | p | p |
6-month Changes | |||||
ADAS-Cog | 1.0±4.6 | 0.1±4.1 | 0.386 | ||
Composites | |||||
Memory | –0.017±0.017 | 0.320 | –0.044±0.016 | 0.012* | 0.447 |
Executive function | –0.016±0.010 | 0.126 | –0.016±0.018 | 0.380 | 0.849 |
Attention | –0.042±0.017 | 0.015* | –0.024±0.019 | 0.208 | 0.539 |
Processing speed | –0.037±0.009 | 0.000* | –0.040±0.014 | 0.009* | 0.778 |
Language | –0.038±0.010 | 0.000* | –0.039±0.012 | 0.004* | 0.925 |
Global cognition | –0.031±0.007 | 0.000* | –0.033±0.009 | 0.001* | 0.844 |
12-month Changes | |||||
ADAS-Cog | 2.4±5.2 | 2.2±5.7 | 0.856 | ||
Cognitive composites | |||||
Memory | –0.012±0.005 | 0.024* | –0.023±0.007 | 0.003* | 0.274 |
Executive function | –0.013±0.005 | 0.015* | –0.010±0.008 | 0.218 | 0.864 |
Attention | –0.033±0.010 | 0.001* | –0.036±0.011 | 0.005* | 0.900 |
Processing speed | –0.030±0.006 | 0.000* | –0.026±0.008 | 0.003* | 0.763 |
Language | –0.028±0.005 | 0.000* | –0.027±0.007 | 0.001* | 0.925 |
Global cognition | –0.024±0.004 | 0.000* | –0.025±0.005 | 0.000* | 0.830 |
The p-values for cycling and stretching are from testing the effect of time on the longitudinal outcome using mixed-effects models, which adjusts for covariates and participant-specific random intercepts. The p-values for between-group difference are from testing the interactive effect of group and time on the longitudinal outcomes using mixed-effects, which includes fixed effects for treatment group, time, and their interaction, covariates, and participant-specific random intercepts.
Rates of changes in cognition over 12 months
The rate of changes in ADAS-Cog over 12 months was smaller for the cycling group than that of the control (0.192 versus 0.200), but their differences were not statistically significant (Table 3). During the 6-month intervention period, cycling participants experienced significant cognitive declines in attention, processing speed, and language, but the declines in memory and executive function were not significant (Table 2). Their 12-month declines in all cognitive domains were statistically significant. In contrast, control participants manifested significant declines in memory, processing speed, and language, but not in executive function and attention over the 6-month intervention period. Their cognition declined over 12 months except for executive function (Table 2). The rates of changes over 12 months in discrete cognitive domains did not differ statistically between groups (Table 3).
Table 3
Rates of changes in ADAS-Cog and cognitive composites
Cycling | Stretching | Between-group difference | |||
Measure | Mean±SEM | p | Mean±SEM | p | p |
ADAS-Cog | 0.192±0.050 | 0.000* | 0.200±0.081 | 0.016* | 0.975 |
Composites | |||||
Memory | –0.013±0.006 | 0.040* | –0.019±0.007 | 0.007* | 0.365 |
Executive function | –0.019±0.005 | 0.000* | –0.010±0.009 | 0.251 | 0.562 |
Attention | –0.035±0.007 | 0.000* | –0.033±0.011 | 0.003* | 0.915 |
Processing speed | –0.033±0.006 | 0.000* | –0.028±0.008 | 0.000* | 0.660 |
Language | –0.029±0.005 | 0.000* | –0.026±0.005 | 0.000* | 0.758 |
Global cognition | –0.027±0.004 | 0.000* | –0.024±0.005 | 0.000* | 0.843 |
The p-values for cycling and stretching are from testing the effect of time on the longitudinal outcome using mixed-effects models, which adjusts for covariates and participant-specific random intercepts with residuals modeled with AR(1) correlation structure. The p-values for between-group difference are from testing the interactive effect of group and time on the longitudinal outcomes using mixed-effects, which includes fixed effects for treatment group, time, and their interaction, covariates, and participant-specific random intercepts with AR(1)-autocorrelated residuals.
Exercise adherence and adverse events
There were no differences between the groups in the percent of attended exercise sessions, the percent of attended sessions that met the intensity and prescription, or adherence per protocol (>70% sessions attended and >70% sessions attended meeting the intensity prescription). Exercise adherence did not affect outcomes. Seven study-related adverse events occurred: chest pain during an exercise session (n = 1); car accidents during travel to the research site (n = 2); disorientations (n = 2); altercation between participants (n = 1); and a fall (n = 1). All participants were medically cleared to continue the study.
DISCUSSION
Our findings support a priori hypothesis that intervention participants had a smaller 6-month increase in ADAS-Cog than the expected decline associated with the natural disease progression. The 6- and 12-month changes in ADAS-Cog did not differ between the cycling and stretching groups. The 12-month rates of changes in global cognition and discrete cognitive domains did not reach statistical significance between groups.
Our primary finding indicates that a 6-month aerobic exercise intervention significantly reduced the decline in global cognition in comparison to AD dementia’s natural course of decline. This finding is consistent with the results from other RCTs which have demonstrated that aerobic exercise improved or stabilized global cognition over time among older adults with MCI or dementia [36–38]. We benchmarked the intervention effect on ADAS-Cog decline to the 6-month natural course of decline in AD which has well been established in older adults with mild-to-moderate AD dementia [19]. In addition, ADAS-Cog has been the most frequently used measure in drug RCTs [19] and allows the comparison of the effect sizes from many different interventions from aerobic exercise to novel AD therapeutics.
We did not find a significant between-group difference in global cognition at either six or 12 months. Our findings are consistent those from recent RCTs using similar aerobic exercise doses in older adults with MCI [36] and early AD dementia [10, 11]. For example, the Danish ADEX trial showed that 16 weeks of aerobic exercise on treadmill, cycling, or cross-trainer at 70–80% of age-predicted maximal heart rate (= 220-age) did not produce significant group differences in executive function as measured by the Symbol Digit Modalities Test in participants with mild AD dementia [11]. A U.S. RCT also reported a lack of group differences in memory and executive function from 26 weeks of moderate-intensity aerobic exercise on in older adults with MCI or mild AD dementia [10]. Another trial that evaluated a combined aerobic and strength training exercise program in dementia in the UK, the largest exercise trial conducted to date, reported a negative intervention effect on ADAS-Cog [12]. However, the lack of statistically significant between-group differences in cognition should not necessarily be interpreted as though aerobic exercise was not effective. The Danish ADEX trial, for example, did find a 2.5-point difference, albeit not statistically significant, between the groups in favor of the intervention group [11]. The UK trial included all dementia subtypes with 36% of the sample having AD dementia and it is unclear if the delivered aerobic-exercise reached the recommended 150-minute moderate-intensity weekly dose for general health [12]. When comparing participants who achieved high dose (high attendance and high intensity) of the intervention versus low dose in the ADEX trial, the between-group difference in executive function was significant [39]. A U.S. trial further found that changes in aerobic fitness and memory were positively correlated [10].
The lack of between-group differences in cognitive effect in our trial was anticipated since our trial was not powered to detect group differences. The lack of power may be a reason why the recent RCTs were unable to detect significant between-group differences in cognitive outcomes [10, 11]. Currently, only a few exercise RCTs in AD have used the ADAS-Cog [11, 40, 41]. In an Australian study (n = 40), four months of aerobic exercise decreased ADAS-Cog scores by 4.9±1.1 points from baseline 22.7±9.7 in comparison to an increase of 2.1±1.4 points from baseline 26.6±16.6 in the control group (p = 0.001) [41]. In the Denmark ADEX Trial (n = 200), only ADAS-Cog immediate and delayed recall trials were used as secondary measures and showed neither within- nor between-group differences [11]. The pooled placebo groups in drug trials showed a baseline score of 28.4±10.6 (n = 536) [19]. In contrast, our participants scored 19.3±7.4 versus 17.8±6.5 (intervention versus control) at baseline, suggesting that their relatively better cognition at baseline may have left little room for improvement above and beyond measurement errors, contributing further to challenges identifying between-group differences in ADAS-Cog changes. Lastly, our intervention participants had more chronic conditions, BPSD, and caregiver distress as well as worse mood than the controls at baseline than controls (Table 1). These differences may have affected dose adherence, attenuated the benefits of cycling, and limited our ability to detect between-group differences.
We have learned several important lessons for designing future trials. First, a non-exercise control may be more appropriate to reduce Hawthorne and social interaction effects such as a waitlist, usual care, or non-exercise group. When designing the FIT-AD trial, we specifically matched the intervention and control exercises on frequency and duration, which inadvertently and substantially increased social interactions in the control group during implementation. Participants perceived stretching as boring and the interventionists socialized with stretching participants extensively to prevent non-adherence and dropout. In contrast, the physical demand of cycling limited social interaction. At least one previous trial showed that exercise and social interactions affected cognition similarly in nursing home residents with dementia [42]. Second, strategies are needed to reduce cross-contamination from the control group. Some family members and participants had reached out to us, expressing their strong dissatisfaction about being in the control group. Approximately a third of our control participants improved their aerobic fitness over the 6-month intervention period, re-affirming our perception that some stretching participants participated in aerobic exercise outside of the study. Third, makeup sessions need to be offered to all participants including those who achieved the per-protocol dose. We only offered makeup sessions to participants who fell below 70% attendance. Subsequently, we implemented this strategy in our ACT Trial [43] and improved session adherence to >80% with a number of participants achieving 100%. Fourth, the intervention duration should not be restricted to calendar months. While calendar months made it easy to track data collections, they prohibited our participants from receiving an actual 6-month duration of exercise because extended absences from vacations and medical clearance were frequent. The 6-month duration is considered to be the minimal dose necessary to induce cognitive changes [44]. Greater intensity and longer duration are needed in future trials. For example, post-hoc analysis showed that the average intensity achieved by our participants was about 54% of HRR, which was below the prescription.
The strengths of our RCT include a rigorous design and implementation following clinical trial guidelines, interventionist-supervised exercise delivery to allow an accurate measurement of delivered doses, relatively high attendance, matched social interventions between groups, and the use of validated outcome measures. In addition, examining cognitive outcomes from the perspective of lack of decline instead of improvement is a unique strength. When AD pathology reaches certain thresholds to warrant the diagnosis of AD dementia, ongoing cognitive decline is likely irreversible [45]. This conclusion has led trials to re-focus on preclinical AD and MCI when AD pathology is less severe [46]. Despite the known irreversible, declining trajectory of cognition in AD dementia, clinical trials and meta-analyses continue to focus on cognitive improvement as a favorable outcome [15, 16]. Our findings better align with the direction of future AD dementia trials by focusing on the more realistic outcome of reducing the rate of cognitive decline.
We acknowledge several weaknesses in our study. One weakness is the lack of power to detect group differences in outcomes. Other weaknesses include enrollment of participants with mild or moderate stages of AD dementia which reduced sample homogeneity and may have restricted our ability to identify significant effects, a selection bias where mild and moderate stages of AD dementia were unequally represented, and a lack of a validated measure of comorbidity. This trial is also limited by not using biomarkers as the primary outcome. The ADAS-Cog was the most widely used endpoint in AD clinical trials, particularly in drug trials. It is increasingly used in exercise trials, albeit limited, which allows the benchmarking of exercise effects to those of novel drug therapeutics. There are still a limited number of aerobic-exercise trials in older adults with AD dementia using the ADAS-Cog. In contrast, biomarker endpoints may be more sensitive to intervention effects. Once the methodological (consistent processing and analytical methods and cut points) [45] and cost issues are resolved, biomarkers could potentially serve as endpoints in future exercise trials.
Conclusions and relevance
Our trial showed that aerobic exercise reduced global cognition decline. The lack of between-group differences in cognition over time is likely explained by the lack of power, a sicker intervention group than control, and under-recognized Hawthorne and social interaction effects. Our findings support the clinical relevance of promoting aerobic exercise in individuals with AD dementia. Analyses of our baseline data showed that our participants had substantially lower aerobic fitness in comparison to age- and gender-matched peers [47] and have an average of 3.5 comorbidities, and both issues are amenable to aerobic exercise. Although we did not measure quality of life, participants and family caregivers had consistently and overwhelmingly expressed the positive impact of exercise on their lives. Studies in AD have also shown that aerobic exercise improved physical function and behavioral and psychological symptoms of dementia [11, 39]. In addition, aerobic exercise has a low profile of adverse events in older adults with AD dementia as demonstrated by our trial. Hence, regardless of its effect on cognition, the current collective evidence supports the use of aerobic exercise as an adjuvant therapy for AD.
ACKNOWLEDGMENTS
The research study reported in this publication was supported by the National Institute on Aging of the National Institutes of Health (R01AG043392-01A1). The CTSI and Center for Magnetic Resonance Resources were supported by the National Institutes of Health National Center for Advancing Translational Sciences of the National Institutes of Health Award Number UL1TR000114 and the National Institute of Biomedical Imaging and Bioengineering Award Number P41 EB1058941, respectively. The content is solely the responsibility of the authors and does not necessarily represent the official views of the National Institutes of Health.
We thank everyone who responded to our recruitment, the study participants and their family caregivers for their participations, and our community partners for helping us recruit and carry out our study. We’re indebted to our passionate and respectful staff (Susan Greimel and Kaitlin Kelly) in rigorously implementing all aspects of the study, and our students who helped with the day-to-day operations.
Authors’ disclosures available online (https://www.j-alz.com/manuscript-disclosures/20-1100r2).
SUPPLEMENTARY MATERIAL
[1] The supplementary material is available in the electronic version of this article: https://dx.doi.org/10.3233/JAD-201100.
REFERENCES
[1] | Alzheimer’s Association ((2020) ) 2020 Alzheimer’s disease facts and figures. Alzheimers Dement 16: , 391–460. |
[2] | McGurran H , Glenn JM , Madero EN , Bott NT ((2019) ) Prevention and treatment of Alzheimer’s disease: Biological mechanisms of exercise. J Alzheimers Dis 69: , 311–338. |
[3] | da Costa Daniele TM , deBruin PFC , de Matos RS , de Bruin GS , Maia Chaves C Junior , de Bruin VMS ((2020) ) Exercise effects on brain and behavior in healthy mice, Alzheimer’s disease and Parkinson’s disease model-a systematic review and meta-analysis. Behav Brain Res 383: , 112488. |
[4] | Arcoverde C , Deslandes A , Moraes H , Almeida C , de Araujo NB , Vasques PE , Silveira H , Laks J ((2014) ) Treadmill training as an augmentation treatment for Alzheimer’s disease: A pilot randomized controlled study. Arq Neuropsiquiatr 72: , 190–196. |
[5] | Bossers WJR , van der Woude LH , Boersma F , Hortobágyi T , Scherder EJ , van Heuvelen MJ ((2015) ) A 9-week aerobic and strength training program improves cognitive and motor function in patients with dementia: A randomized, controlled trial. Am J Geriatr Psychiatry 23: , 1106–1116. |
[6] | Cancela JM , Ayán C , Varela S , Seijo M ((2016) ) Effects of a long-term aerobic exercise intervention on institutionalized patients with dementia. J Sci Med Sport 19: , 293–298. |
[7] | Holthoff VA , Marschner K , Scharf M , Steding J , Meyer S , Koch R , Donix M ((2015) ) Effects of physical activity training in patients with Alzheimer’s dementia: Results of a pilot RCT study. PloS One 10: , e0121478. |
[8] | Law LL , Barnett F , Yau MK , Gray MA ((2014) ) Effects of functional tasks exercise on older adults with cognitive impairment at risk of Alzheimer’s disease: A randomised controlled trial. Age Ageing 43: , 813–820. |
[9] | Öhman H , Savikko N , Strandberg T , Kautiainen H , Raivio M , Laakkonen ML , Tilvis R , Pitkälä KH ((2016) ) Effects of exercise on functional performance and fall rate in subjects with mild or advanced Alzheimer’s disease: Secondary analyses of a randomized controlled study. Dement Geriatr Cogn Disord 41: , 233–241. |
[10] | Morris JK , Vidoni ED , Johnson DK , Van Sciver A , Mahnken JD , Honea RA , Wilkins HM , Brooks WM , Billinger SA , Swerdlow RH , Burns JM ((2017) ) Aerobic exercise for Alzheimer’s disease: A randomized controlled pilot trial. PLoS One 12: , e0170547. |
[11] | Hoffmann K , Sobol NA , Frederiksen KS , Beyer N , Vogel A , Vestergaard K , Braendgaard H , Gottrup H , Lolk A , Wermuth L , Jacobsen S , Laugesen LP , Gergelyffy RG , Høgh P , Bjerregaard E , Andersen BB , Siersma V , Johannsen P , Cotman CW , Waldemar G , Hasselbalch SG ((2016) ) Moderate-to-high intensity physical exercise in patients with Alzheimer’s disease: A randomized controlled trial. J Alzheimers Dis 50: , 443–453. |
[12] | Lamb SE , Mistry D , Alleyne S , Atherton N , Brown D , Copsey B , Dosanjh S , Finnegan S , Fordham B , Griffiths F , Hennings S , Khan I , Khan K , Lall R , Lyle S , Nichols V , Petrou S , Zeh P , Sheehan B ((2018) ) Aerobic and strength training exercise programme for cognitive impairment in people with mild to moderate dementia: The DAPA RCT. Health Technol Assess 22: , 1–202. |
[13] | Toots A , Littbrand H , Boström G , Hörnsten C , Holmberg H , Lundin-Olsson L , Lindelöf N , Nordström P , Gustafson Y , Rosendahl E ((2017) ) Effects of exercise on cognitive function in older people with dementia: A randomized controlled trial. J Alzheimers Dis 60: , 323–332. |
[14] | Baker LD , Frank LL , Foster-Schubert K , Green PS , Wilkinson CW , McTiernan A , Plymate SR , Fishel MA , Watson GS , Cholerton BA , Duncan GE , Mehta PD , Craft S ((2010) ) Effects of aerobic exercise on mild cognitive impairment: A controlled trial. Arch Neurol 67: , 71–79. |
[15] | Panza GA , Taylor BA , MacDonald HV , Johnson BT , Zaleski AL , Livingston J , Thompson PD , Pescatello LS ((2018) ) Can exercise improve cognitive symptoms of Alzheimer’s disease? A meta-analysis. J Am Geriatr Soc 66: , 487–495. |
[16] | Colcombe S , Kramer AF ((2003) ) Fitness effects on the cognitive function of older adults: A meta-analytic study. Psychol Sci 14: , 125–130. |
[17] | Yu F , Bronas UG , Konety S , Nelson NW , Dysken M , Jack C Jr , Wyman JF , Vock D , Smith G ((2014) ) Effects of aerobic exercise on cognition and hippocampal volume in Alzheimer’s disease: Study protocol of a randomized controlled trial (The FIT-AD trial). Trials 15: , 394. |
[18] | McKhann GM , Knopman DS , Chertkow H , Hyman BT , Jack CR Jr , Kawas CH , Klunk WE , Koroshetz WJ , Manly JJ , Mayeux R , Mohs RC , Morris JC , Rossor MN , Scheltens P , Carillo MC , Thies B , Weintraub S , Phelps CH ((2011) ) The diagnosis of dementia due to Alzheimer’s disease: Recommendations from the National Institute on Aging and the Alzheimer’s Association workgroup. Alzheimers Dement 7: , 263–269. |
[19] | Doraiswamy PM , Kaiser L , Bieber F , Garman RL ((2001) ) The Alzheimer’s Disease Assessment Scale: Evaluation of psychometric properties and patterns of cognitive decline in multicenter clinical trials of mild to moderate Alzheimer’s disease. Alzheimer Dis Assoc Disord 15: , 174–183. |
[20] | Weintraub S , Salmon D , Mercaldo N , Ferris S , Graff-Radford NR , Chui H , Cummings J , DeCarli C , Foster NL , Galasko D , Peskind E , Dietrich W , Beekly DL , Kukull WA , Morris JC ((2009) ) The Alzheimer’s Disease Centers’ Uniform Data Set (UDS): The neuropsychologic test battery. Alzheimer Dis Assoc Disord 23: , 91–101. |
[21] | Rosen WG , Mohs RC , Davis KL ((1984) ) A new rating scale for Alzheimer’s disease. Am J Psychiatry 141: , 1356–1364. |
[22] | Wechsler D (1997) Wechsler Adult Intelligence Scale–Third Edition (WAIS-III), The Psychological Corporation, Odessa, FL. |
[23] | Brandt J , Benedict RHB (2001) Hopkins Verbal Learning Test - Revised, Psychological Assessment Resources, Odessa, FL. |
[24] | Reitan RM , Wolfson D ((2004) ) The Trail Making Test as an initial screening procedure for neuropsychological impairment in older children. Arch Clin Neuropsychol 19: , 281–288. |
[25] | Royall DR , Mahurin RK , Gray KF ((1992) ) Bedside assessment of executive cognitive impairment: The executive interview. J Am Geriatr Soc 40: , 1221–1226. |
[26] | Royall DR , Cordes JA , Polk M ((1998) ) CLOX: An executive clock drawing task. J Neurol Neurosurg Psychiatry 64: , 588–594. |
[27] | Wechsler D , Coalson D , Raiford S ((2009) ) Weschler Adult Intelligence Test: Fourth edition technical and interpretive manual, Pearson, San Antonio, TX. |
[28] | Golden CJ , Freshwater SM (2002) Stroop Color and Word Test: Revised Examiner’s Manual, Stoelting Co., Wood-dale, IL. |
[29] | Ivnik RJ , Malec JF , Smith GE , Tangalos EG , Petersen RC ((1996) ) Neuropsychological tests’ norms above age 55: COWAT, BNT, MAE Token, WRAT-R Reading, AMNART, STROOP, TMT, and JLO. Clin Neuropsychol 10: , 262–278. |
[30] | Lucas JA , Ivnik RJ , Smith GE , Bohac DL , Tangalos EG , Graff-Radford NR , Petersen RC ((1998) ) Mayo’s older Americans normative studies: Category fluency norms. J Clin Exp Neuropsychol 20: , 194–200. |
[31] | WAIS-III Technical Manual, The Psychological Corporation, San Antonio, TX. |
[32] | Cummings JL ((1997) ) The Neuropsychiatric Inventory: Assessing psychopathology in dementia patients. Neurology 48: , S10–S16. |
[33] | Gélinas I , Gauthier L , McIntyre M , Gauthier S ((1999) ) Development of a functional measure for persons with Alzheimer’s disease: The disability assessment for dementia. Am J Occup Ther 53: , 471–481. |
[34] | Cosentino SA , Stern Y , Sokolov E , Scarmeas N , Manly JJ , Tang MX , Schupf N , Mayeux RP ((2010) ) Plasma β-amyloid and cognitive decline. Arch Neurol 67: , 1485–1490. |
[35] | Nandipati S , Luo X , Schimming C , Grossman HT , Sano M ((2012) ) Cognition in non-demented diabetic older adults. Curr Aging Sci 5: , 131–135. |
[36] | Tarumi T , Rossetti H , Thomas BP , Harris T , Tseng BY , Turner M , Wang C , German Z , Martin-Cook K , Stowe AM , Womack KB , Mathews D , Kerwin DR , Hynan L , Diaz-Arrastia R , Lu H , Cullum CM , Zhang R ((2019) ) Exercise training in amnestic mild cognitive impairment: A one-year randomized controlled trial. J Alzheimers Dis 71: , 421–433. |
[37] | Song D , Yu DSF ((2019) ) Effects of a moderate-intensity aerobic exercise programme on the cognitive function and quality of life of community-dwelling elderly people with mild cognitive impairment: A randomised controlled trial. Int J Nurs Stud 93: , 97–105. |
[38] | Sampaio A , Marques EA , Mota J , Carvalho J ((2019) ) Effects of a multicomponent exercise program in institutionalized elders with Alzheimer’s disease. Dementia (London) 18: , 417–431. |
[39] | Sobol NA , Dall CH , Høgh P , Hoffmann K , Frederiksen KS , Vogel A , Siersma V , Waldemar G , Hasselbalch SG , Beyer N ((2018) ) Change in fitness and the relation to change in cognition and neuropsychiatric symptoms after aerobic exercise in patients with mild Alzheimer’s disease. J Alzheimers Dis 65: , 137–145. |
[40] | Yang SY , Shan CL , Qing H , Wang W , Zhu Y , Yin MM , Machado S , Yuan TF , Wu T ((2015) ) The effects of aerobic exercise on cognitive function of Alzheimer’s disease patients. CNS Neurol Disord Drug Targets 14: , 1292–1297. |
[41] | Vreugdenhil A , Cannell J , Davies A , Razay G ((2012) ) A community-based exercise programme to improve functional ability in people with Alzheimer’s disease: A randomized controlled trial. Scand J Caring Sci 26: , 12–19. |
[42] | de Souto Barreto P , Cesari M , Denormandie P , Armaingaud D , Vellas B , Rolland Y ((2017) ) Exercise or social intervention for nursing home residents with dementia: A pilot randomized, controlled trial. J Am Geriatr Soc 65: , E123–E129. |
[43] | Yu F , Lin FV , Salisbury DL , Shah KN , Chow L , Vock D , Nelson NW , Porsteinsson AP , Jack C Jr ((2018) ) Efficacy and mechanisms of combined aerobic exercise and cognitive training in mild cognitive impairment: Study protocol of the ACT trial. Trials 19: , 700. |
[44] | Erickson KI , Kramer AF ((2009) ) Aerobic exercise effects on cognitive and neural plasticity in older adults. Br J Sports Med 43: , 22–24. |
[45] | Jack CR Jr , Bennett DA , Blennow K , Carrillo MC , Dunn B , Haeberlein SB , Holtzman DM , Jagust W , Jessen F , Karlawish J , Liu E , Molinuevo JL , Montine T , Phelps C , Rankin KP , Rowe CC , Scheltens P , Siemers E , Snyder HM , Sperling R ; Contributors ((2018) ) NIA-AA Research Framework: Toward a biological definition of Alzheimer’s disease. Alzheimers Dement 14: , 535–562. |
[46] | Porsteinsson AP , Clark ED ((2020) ) Editorial: The Trial-Ready Cohort for Preclinical/Prodromal Alzheimer’s Disease (TRC-PAD) - A fundamental ally in AD prevention research. J Prev Alzheimers Dis 7: , 206–207. |
[47] | Salisbury D , Yu F ((2020) ) Establishing reference cardiorespiratory fitness parameters in Alzheimer’s disease. Sports Med Int Open 4: , E1–E7. |