Proteasome Activity in the Plasma as a Novel Biomarker in Mild Cognitive Impairment with Chronic Tinnitus
Abstract
Background:
Although the existence of proteasomes in human blood, termed circulating proteasomes (c-proteasomes), has been reported previously, their origin and pathophysiological functions remain largely unknown.
Objective:
Given that c-proteasome activity was significantly reduced in Alzheimer’s disease model mice and relatively high frequency of mild cognitive impairment (MCI) is accompanied by chronic tinnitus in aged patients, we examined whether c-proteasome activity in human plasma was associated with cognitive function in patients with chronic tinnitus.
Methods:
c-Proteasome activity in the plasma of tinnitus patients (N = 55) was measured with fluorogenic reporter substrate, suc-LLVY-AMC. To assess MCI, the Montreal Cognitive Assessment was conducted with a cut-off score of 22/23. All patients underwent audiological and psychoacoustic analyses. Levels of c-proteasomes, Aβ42, and Aβ40 were measured using ELISA, and their association with c-proteasome activity was evaluated.
Results:
The activity of circulating proteasomes was significantly lower in patients with chronic tinnitus and MCI (p = 0.042), whereas activities of other plasma enzymes showed little correlation. In addition, c-proteasome activity was negatively associated with the level of plasma Aβ and was directly dependent on its own concentration in the plasma of patients with chronic tinnitus.
Conclusion:
Our current work provides a new perspective for understanding the potential relationship between circulating proteasomes in the plasma and cognitive dysfunction, suggesting a novel, non-invasive biomarker in the context of MCI diagnosis.
INTRODUCTION
The ubiquitin-proteasome system degrades the majority of proteins in the cell, thereby regulating the half-lives of various regulatory proteins and removing misfolded and potentially toxic proteins [1]. The proteasome is the sole ATP-dependent protease in the eukaryotic cytoplasm and irreversibly and simultaneously hydrolyzes multiple peptide bonds of the target protein. The 26S proteasome consists of two functionally distinct protein compartments: the core complex (20S) and the regulatory complex (19S) [2]. The 20S proteasome contains six catalytic sites in its cylinder-shaped active site. The 19S proteasome performs diverse functions, including recognizing, unfolding, and translocating of substrates into the 20S proteasome. The 20S proteasome can degrade disordered proteins without requiring substrate ubiquitination and participates in cellular surveillance against oxidative and proteotoxic stress [3]. A significant proportion of proteasomes in the cell exist as both the 20S and 26S forms [4].
Although intracellular protein homeostasis (i.e., proteostasis) mechanisms have received the most attention in various pathophysiological contexts, many proteopathies, such as Alzheimer’s disease (AD), type II diabetes, and age-related macular degeneration, originate from accumulation of aggregation-prone proteins in the extracellular regions of the human body [5]. The recent identification of circulating chaperones and ubiquitin suggests the presence of an extracellular protein quality control system that antagonizes pathological processes in the blood and cerebrospinal fluid [6–8]. Indeed, there has been recent growing evidence that the interaction between amyloid-β (Aβ) peptide and ubiquitin has been hailed as a significant determinant for its clearance pathways [9]. Although several studies have reported the possible links between circulating proteasomes (c-proteasomes) in the plasma with various disease states, such as chronic obstructive pulmonary disorder [10] and head and neck squamous cell carcinoma [11], extracellular proteasomes have not been characterized in the context of neurodegenerative diseases.
Tinnitus is a common and potentially debilitating condition that is characterized by conscious auditory perception in the absence of an external stimulus [12]. Peripheral auditory deafferentation and maladaptive cortical plasticity have been suggested as etiological mechanisms for the development of tinnitus [13]. Recent studies have suggested a potential association between chronic tinnitus and cognitive deficits [14–17]. For example, the diagnostic criteria of mild cognitive impairment (MCI) were met with a relatively high prevalence in aged patients with chronic tinnitus, at approximately 17% [17]. Considering the substantial risk of patients with MCI advancing to dementia, particularly of the Alzheimer type [18, 19], there is a need for an effective biomarker predicting MCI or early AD for patients with chronic tinnitus and the aged.
Many causative proteins, including Aβ and tau, have been identified in the plasma of patients with AD and are proposed to be early-stage biomarkers [20–22]. Over the course of biochemically characterizing c-proteasomes, we observed that the enzymatic activity of c-proteasomes was significantly reduced in multiple lines of AD model mice, which led us to hypothesize that c-proteasome activity in the plasma might have prognostic significance potentially correlated with the status of cognitive deficits. The association of c-proteasome activity with cognitive deficits was recapitulated in patients with chronic tinnitus. In this study, we showed that the enzymatic activity of c-proteasomes positively correlated with its own levels but negatively correlated with Aβ40 levels in the plasma. Thus, these results provide for better understanding the potential relationship between c-proteasomes in the plasma and cognitive dysfunction and suggest a novel, non-invasive biomarker in the context of early MCI diagnosis.
MATERIALS AND METHODS
Patients
This study was approved by the Seoul National University Hospital Institutional Review Board and was conducted in accordance with the Declaration of Helsinki (IRB No. 10-2018-35). We prospectively recruited patients with chronic tinnitus, over 50 years old who were admitted to the Seoul National University Boramae Medical Centre between January 2010 and October 2018. Only patients in whom baseline cognitive test and blood sampling for c-proteasome activity measurement were performed were included. Additionally, samples exhibiting more than 33.3% difference in c-proteasome activity from the technical duplicates and positive for serology tests were further excluded from our analysis. Moreover, patients with 1) hearing loss >70 dB hearing level (dB HL) in at least one ear (calculated by averaging the pure tone thresholds at 0.5, 1, 2, and 4 kHz), 2) pulsatile tinnitus, Ménière’s disease, and otosclerosis, 3) any psychiatric or neurological disorders, history of drug or alcohol abuse, and chronic headache were further excluded from this study.
Audiological and psychoacoustic evaluations
At the initial visit, a structured history of the characteristics of tinnitus on the affected side and the psychoacoustic nature of tinnitus was obtained. All patients underwent psychoacoustic tests of tinnitus, such as tinnitus pitch matching, tinnitus loudness matching, and the minimum masking level test. In addition, the hearing thresholds for seven different octaves (0.25, 0.5, 1, 2, 3, 4, and 8 kHz) were evaluated using pure tone audiometry (PTA). The hearing threshold average was determined by calculating the four frequencies at 0.5, 1, 2, and 4 kHz and the hearing level was categorized as mild (20–40 dB), moderate (41–55 dB), moderate to severe (56–70 dB), severe (71–90 dB), or profound (>90 dB), based on the average of hearing thresholds. As described in our previous report [17], the hearing threshold corresponding to the tinnitus affected side was determined for analysis. We documented the mean hearing threshold in the dominant tinnitus side, provided that the patients had bilateral tinnitus. The perceived tinnitus handicap was measured using not only THI but also visual analogue scale scores in terms of tinnitus loudness, annoyance, awareness, and effect of life during daytime.
Neurocognitive evaluation
All patients underwent a battery of neurocognitive tests, including three questionnaires at the initial visit: Korean version of the patient health questionnaire-9 (PHQ-9), Korean version of the Lawton instrumental activities of daily living scale (IADL), and MoCA. Specifically, the MoCA test, which evaluates attention and concentration, executive functions, memory, language, visuoconstructional skills, conceptual thinking, calculation, and orientation, was conducted. MoCA is a rapid-screening, neurocognitive test for MCI, where it scores from 0 to 30. Based on the excellent sensitivity (89%) and good specificity (84%) of MoCA when applying the cut-off value of 22, we decided to define MCI as having an MoCA score below 23, in this study.
Mice
Generation of APP/PS1 was as previously described [23]. To generate APP/PS1/Tau-BiFC transgenic mouse, hemizygous APP/PS1 mice harboring APPswe and PSEN1M146V, were crossed with hemizygous TauP301L-BiFC mice that express the human TauP301L-BiFC (TauP301L-VC155 and TauP301L-VN173) [24]. Littermates that were negative for the APPswe, PSEN1M146V, and TauP301L-BiFC were used for wild-type control. Both APP/PS1 and TauP301L-BiFC mice were bred and maintained on a hybrid C57BL6/C3H background. Mice were housed on a 12:12 h light-dark cycle in pathogen-free facilities at the Korea Institute of Science and Technology. Animal protocols followed the principles and practices outlined in the approved guidelines by the Institutional Animal Care and Use Committee of the Korea Institute of Science and Technology (KIST-2019-032).
Genotyping of transgenic mice
Mice were genotyped by polymerase chain reaction (PCR) amplification of tail DNA. TauP301L-BiFC transgene was identified with the following sets of primers: PCR primers for TauP301L-VN173, forward 5′-CACATGAAGCAGCACGACTTCTTCA-3′ and reverse 5′-TGACTGTACCAGGTACAGG CACAGT-3′; for TauP301L-VC155, forward 5′-GAGAAGCGCGATCACATGGT-3′ and reverse 5′-AGGTACAGGCACAGTCCAAC-3′; for APPswe gene, forward 5′-AGGACTGACCACTCGACCAG-3′ and reverse 5′-CCGGGGTCTAGTTCTGCAT-3′; and for PSEN1M146V gene, forward 5′-AATAGAGAACGGCAGGAGCA-3′ and reverse 5′-GCCATGAGG GCACTAATCAT-3′.
Plasma preparation
Whole blood (5 mL) was separately collected and divided into two EDTA vacuum tubes, as technical duplicates. Each tube was subsequently processed entirely independently. Plasma was isolated by centrifugation at 1,600 rpm with a benchtop centrifuge for 10 min and aliquoted into 1.5 mL tubes in volumes of 0.5–1 mL and stored at –80°C until further analysis. Plasma showed more reliable proteasome activity and resistance against freeze-thaw cycles than the serum or whole blood. Hemolytic samples were discarded after visual or luminol inspection.
Native gel electrophoresis
Native gel analysis using purified proteasomes or cell lysates was performed as previously described [25]. Samples were resolved by native-PAGE using NuPAGE™ 3–8% Tris-Acetate Protein Gels (Thermo-Fisher) at 150 V for 3–4 h. Native gels were incubated in the in-gel activity assay buffer (20 mM Tris, 1 mM ATP, 5 mM MgCl2, and 100 μM suc-LLVY-AMC) for visualizing proteasomes. After the in-gel hydrolysis assay, proteins were transferred from the gel to polyvinylidene fluoride (PVDF) membranes for subsequent immunoblotting analysis.
Measurement of plasma Aβ levels
The levels of plasma Aβ42 and Aβ40 were quantified using the INNO-BIA plasma Aβ forms kit (Japan), largely with the manufacturer’s instructions. Briefly, the plasma samples were diluted 3-fold in the plasma diluent buffer. After washing the plate, 100μL of Aβ antibody-coated beads solution was transferred to the filter plate. Thereafter, 25μL of conjugate 1 working solution A and 75μL of samples, standards, blanks, controls were loaded. After overnight incubation at 4°C, the plate was washed again and 100μL of the detection solution was added to the wells of the plate. The plate was incubated at room temperature for 1 h and then washed again. Finally, the reading solution was added to each well. The plate was read using the Luminex xMAP technology (Bioplex 200 systems; Bio-Rad, USA).
Measurement of proteasome activity and levels
Hydrolysis of the fluorogenic peptide suc-LLVY-AMC (7-amino-4-methylcoumarin; Bachem) was kinetically measured to determine the proteolytic activity of the chymotrypsin-like sites on the proteasome, as previously described [26, 27]. Briefly, 20μL of human plasma and 250μM of suc-LLVY-AMC in 50 mM Tris-HCl (pH 7.5), 1 mg/mL BSA, 1 mM EDTA, 1 mM ATP, and 1 mM DTT were used. Samples exhibiting more than 33.3% difference in c-proteasome activity from the technical duplicates were excluded from further analysis. Circulating proteasome levels were calculated with standards of purified 20S proteasome activity [28] and measured with the proteasome ELISA kit (Enzo Life Sciences), as previously described [29].
Statistical analysis
All the analyses employed and illustrated used the GraphPad Prism (ver. 5.03, GraphPad Software). Results are presented as mean±standard deviation (SD). Statistical significance of difference between groups was determined by Student’s t-test, Welch’s t-test, Mann-Whitney U test, chi-square test, or Fisher’s exact test, as appropriate. According to normal distribution of data, Pearson and Spearman correlation analysis was employed. p-values of <0.05 were considered to indicate statistical significance.
RESULTS
Identifying c-proteasomes in the plasma as active 20S complexes
To characterize extracellular proteasomes in the plasma, we first tested the mouse plasma. Mouse plasma showed strong proteasome activity, as measured by the hydrolysis of the reporter substrate suc-LLVY-AMC, which is specific for the chymotrypsin-like peptidase activity of the 20S proteasome but is generally regarded to represent overall proteasome activity [30] (Fig. 1a). Carefully separated human plasma, using EDTA tubes, also exhibited robust c-proteasome activity (see below). Addition of the proteasome inhibitor MG132 effectively inhibited the assay (>85% inhibition; Fig. 1a), indicating that the activity mostly originated from the proteasomes in the plasma, and not from nonspecific blood proteases.
Fig. 1
Characterization of the circulating proteasome (c-proteasome) in mouse blood plasma. a) Proteasome activity from 12-week-old wild type male mouse plasma (1μL; collected using heparinized capillary tubes) was kinetically monitored by the hydrolysis of the fluorogenic reporter substrate (suc-LLVY-AMC; 12.5μM) in the presence or absence of the proteasome inhibitor, MG132 (10μM). Relative fluorescence units (RFU) were plotted as mean±SD of three independent experiments. b) Mouse plasma was analyzed with non-denaturing (native) polyacrylamide gel electrophoresis (PAGE). Purified human 26S proteasomes (Ptsm, 4μg), 1μL mouse plasma after removal of immunoglobulin G, and 20μg whole cell extracts from mouse embryonic fibroblasts were subjected to native PAGE, followed by in-gel suc-LLVY-AMC hydrolysis or by immunoblotting against the 20S subunit, PSMB6, to visualize the proteasomes. Sodium dodecyl sulfate (SDS, 0.02 %) was added to the gel to induce 20S activation (by opening the substrate entry gate). c) As in (a), except that the purified 19S complex was added to the suc-LLVY-AMC hydrolysis reaction in a dose-dependent manner. Elevated proteasome activity upon the addition of the 19S subunit indicates direct association of the 19S with the 20S complex in the plasma. d) Reduced c-proteasome activity was detected from different lines of 15-month-old AD model mice, which included double transgenic mice of mutant amyloid precursor protein and mutant presenilin-1 (APP/PS1), tau transgenics with bimolecular fluorescence complementation (tau-BiFC), and triple transgenics of APP, PS1, and tau-BiFC (3×TG). RFU values after 60 min reaction time were normalized with those obtained with 10μM MG132. Mean±SD (N = 9 per line). *p < 0.05 (two-tailed Student’s t-test). WT, wild-type.
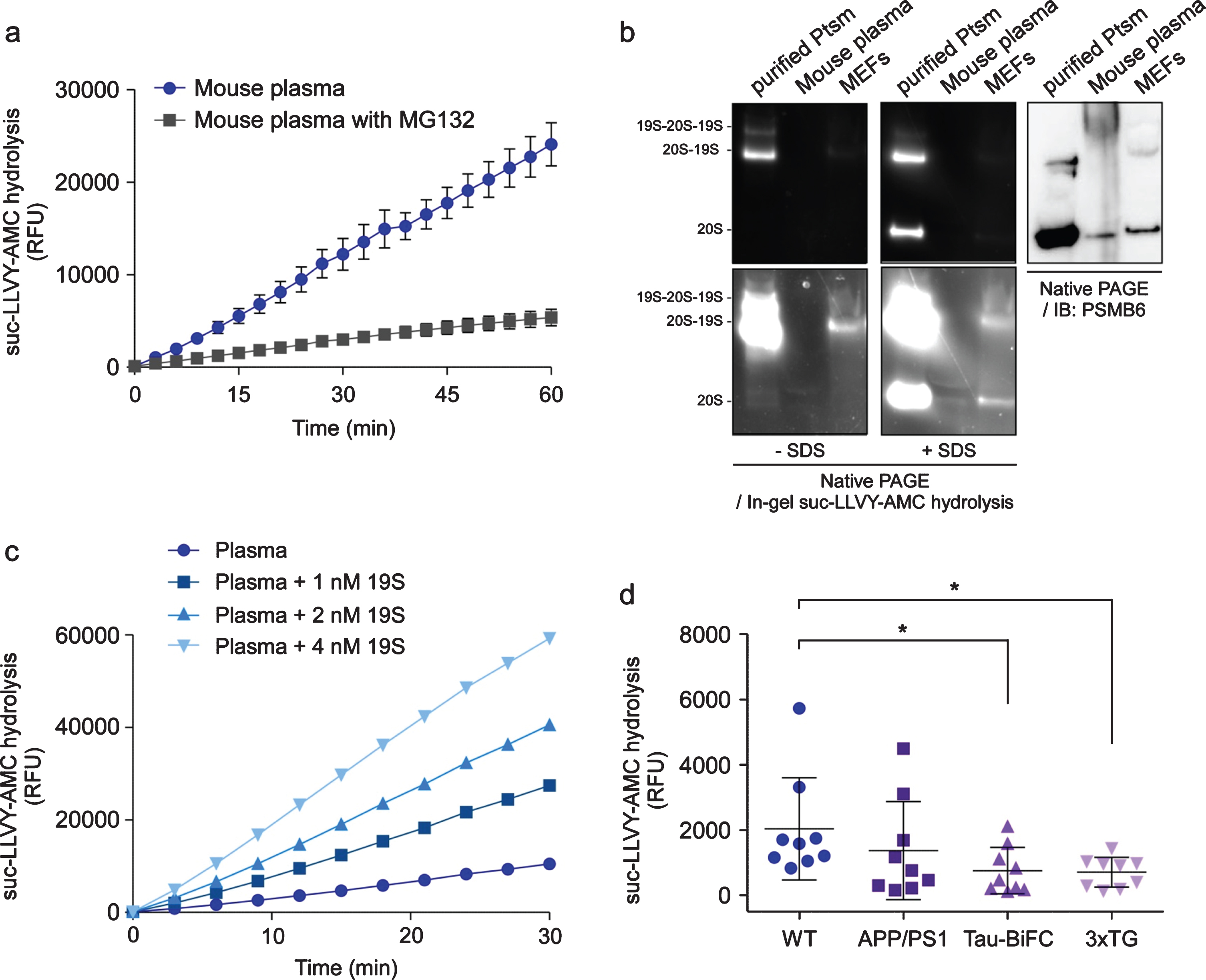
The structural integrity of the c-proteasome complex was analyzed using non-denaturing (native) polyacrylamide gel electrophoresis and the subsequent in-gel activity analysis. Purified human proteasomes and whole cell lysates from mouse embryonic fibroblasts showed signals from intact 26S particles, whereas no proteasome holoenzymes were detected in the mouse plasma (Fig. 1b). When sodium dodecyl sulfate was added to activate the 20S complex [31], the mouse plasma generated fluorescence signals from suc-LLVY-AMC hydrolysis by the 20S complex. This was also confirmed by immunoblotting analysis using an antibody against PSMB6, a 20S subunit (Fig. 1b). Consistent with this, c-proteasome activity from mouse plasma was significantly stimulated in the presence of purified 19S complex largely in a dose-dependent manner, which represents the de novo assembly of the 20S and 19S subunits, forming the highly functional 26S proteasome (Fig. 1c). Taken together, these results indicated that the 20S, and not the 26S, proteasomes exist as an intact complex, also implying that they might be a constitutive component of the blood protein pool and potentially reflect many aspects of physiological functions.
Reduced plasma proteasome activity in mouse AD models
We next assessed whether the activity of c-proteasome in the plasma was linked to the progression of AD using various mouse models. Transgenic mice with a hyperactivated Aβ cascade through overexpression of the mutant amyloid precursor protein (APP) and presenilin 1 (PS1) showed no significant difference from age-matched wild-type controls in c-proteasome activity (Fig. 1d). In contrast, in AD model mice overexpressing mutant tau, such as tau fused with bimolecular fluorescence complementation (tau-BiFC), and triple transgenic mice (APP, PS1, and tau-BiFC (3×TG)) [24], c-proteasome activity decreased compared to controls (Unpaired t-test, p = 0.040 and = 0.026, respectively; N = 9) (Fig. 1d). These AD model mice developed age-dependent synaptic dysfunction and neuronal loss as well as Aβ and tau deposition [32, 33]. Therefore, these data may suggest that reduced c-proteasome activity in the plasma might be predictive of early stage AD progression.
Significant association between c-proteasome activity and MCI in tinnitus patients
Given the relatively high prevalence of MCI among elderly patients with chronic tinnitus [17], it was hypothesized that c-proteasome activity measured in those patients might serve as an indicator of cognitive decline. Initially, 122 subjects with chronic tinnitus and over 50-year old were enrolled as per our inclusion criteria (Fig. 2). Eighteen patients were excluded due to incomplete data and positive serology test. Of the remaining 104 patients, 20 (19.23%) were disqualified because of unreliable c-proteasome activity, leaving only 84 patients with chronic tinnitus that were eligible for this study. Furthermore, 28 patients with severe or severe-to-profound sensorineural hearing loss and one patient with outlier value of c-proteasome (i.e., >2SD over average) were excluded to minimize the confounding factors associated with cognitive deficits; ultimately, 55 patients were included for analyses. When stratified to the presence and absence of MCI, 5 patients with chronic tinnitus had additional MCI (MCI group), while the remaining 50 patients with chronic tinnitus had no evidence of MCI (non-MCI group). To assess MCI, the Montreal cognitive assessment (MoCA) was conducted with a cut-off score of 22/23.
Fig. 2
Flow chart of patient selection.
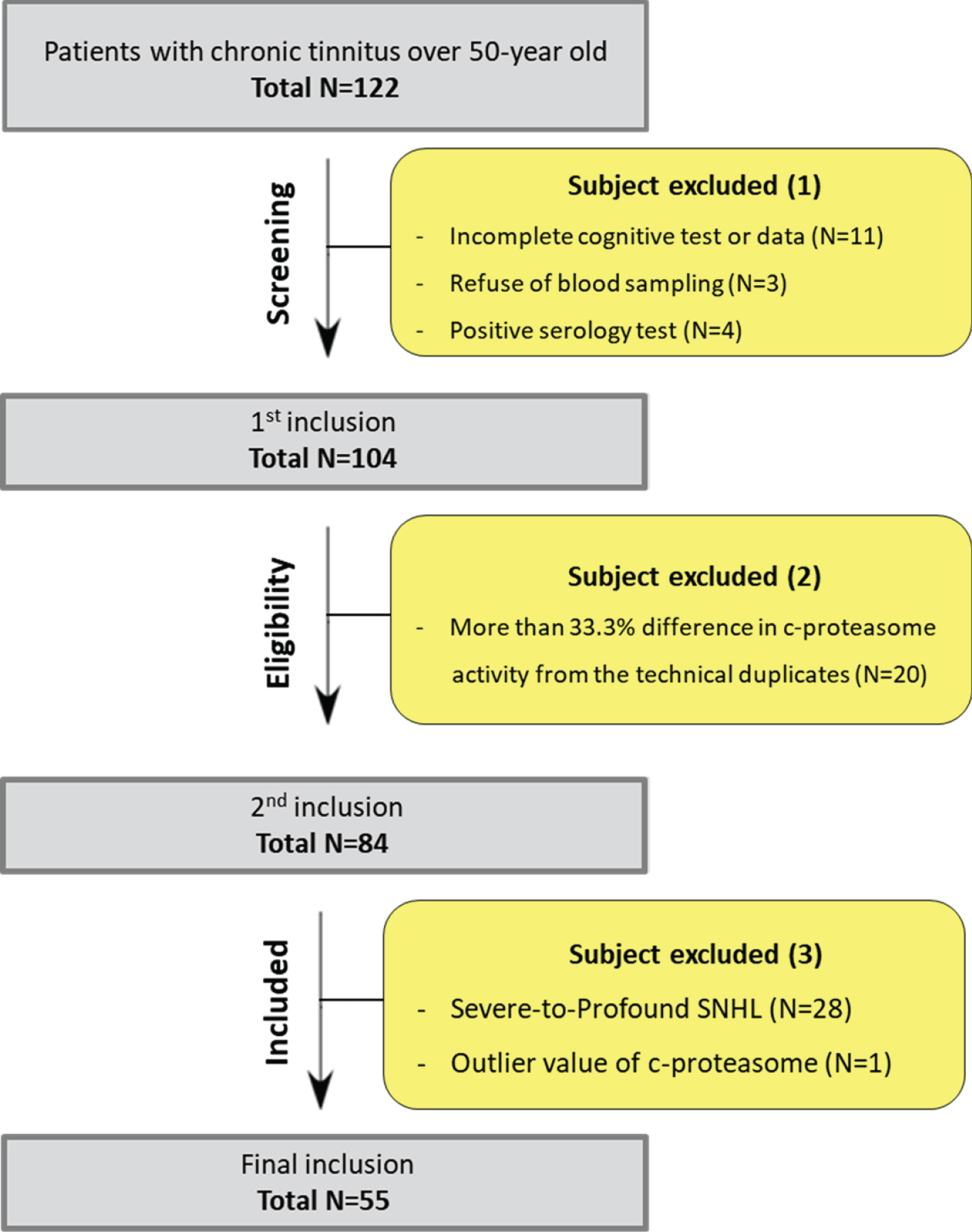
There were no significant differences in age, tinnitus duration, and mean hearing threshold between the two groups (Fig. 3a). Analysis using other neurocognition scales, such as the patient health questionnaire-9 (PHQ) and instrumental activities of daily living (IADL) also showed comparable results. As shown in Fig. 3b, the degree of tinnitus severity, except for visual analogue scale annoyance, also did not differ between the groups. Similarly, the tinnitus handicap inventory (THI) score appeared mildly higher (without statistical significance) in the MCI group than that in the non-MCI group (Fig. 3b). Nonetheless, the correlation between tinnitus severity, based upon the THI and MoCA scores, was replicated (data not shown), as in our previous report [17]. As anticipated, the MCI group exhibited significantly lower scores in multiple subdomains of MoCA, including education, visuospatial execution, attention, and delayed recall areas, compared with the non-MCI group (Fig. 3b). Overall, these data indicated that most variables were controlled to compare the plasma c-proteasome activity between the two groups.
Fig. 3
Reduced c-proteasome activity in patients with chronic tinnitus and mild cognitive impairment (MCI). a, b) Difference in demographics (a) and clinical characteristics (b) between the MCI (Montreal cognitive assessment (MoCA) score, ≤22) and non-MCI (MoCA score, >23) groups from patients with chronic (more than 6 months) tinnitus. None of them reached a statistically significant difference, except for the visual analogue scale (VAS) annoyance. PHQ, patient health questionnaire-9; IADL, instrumental activities of daily living (IADL); THI, tinnitus handicap index. c) Comparison of MoCA scores at each domain between two groups. d) Comparison of c-proteasome activity between the MCI and non-MCI groups. Data indicate means±SD. *p < 0.05 (total N = 55, Welch’s t-test). e, f) Statistically negligible association between activities from c-proteasome in the plasma and glutamic pyruvic transaminase (GPT/ALT; e) or glutamic oxaloacetic transaminase (GOT/AST; f) (p values from Spearman’s correlation analysis = 0.410 and 0.189, respectively). Orange circles indicate samples from patients with MCI. Solid and dashed lines represent the regression line and 95% confidence intervals, respectively.
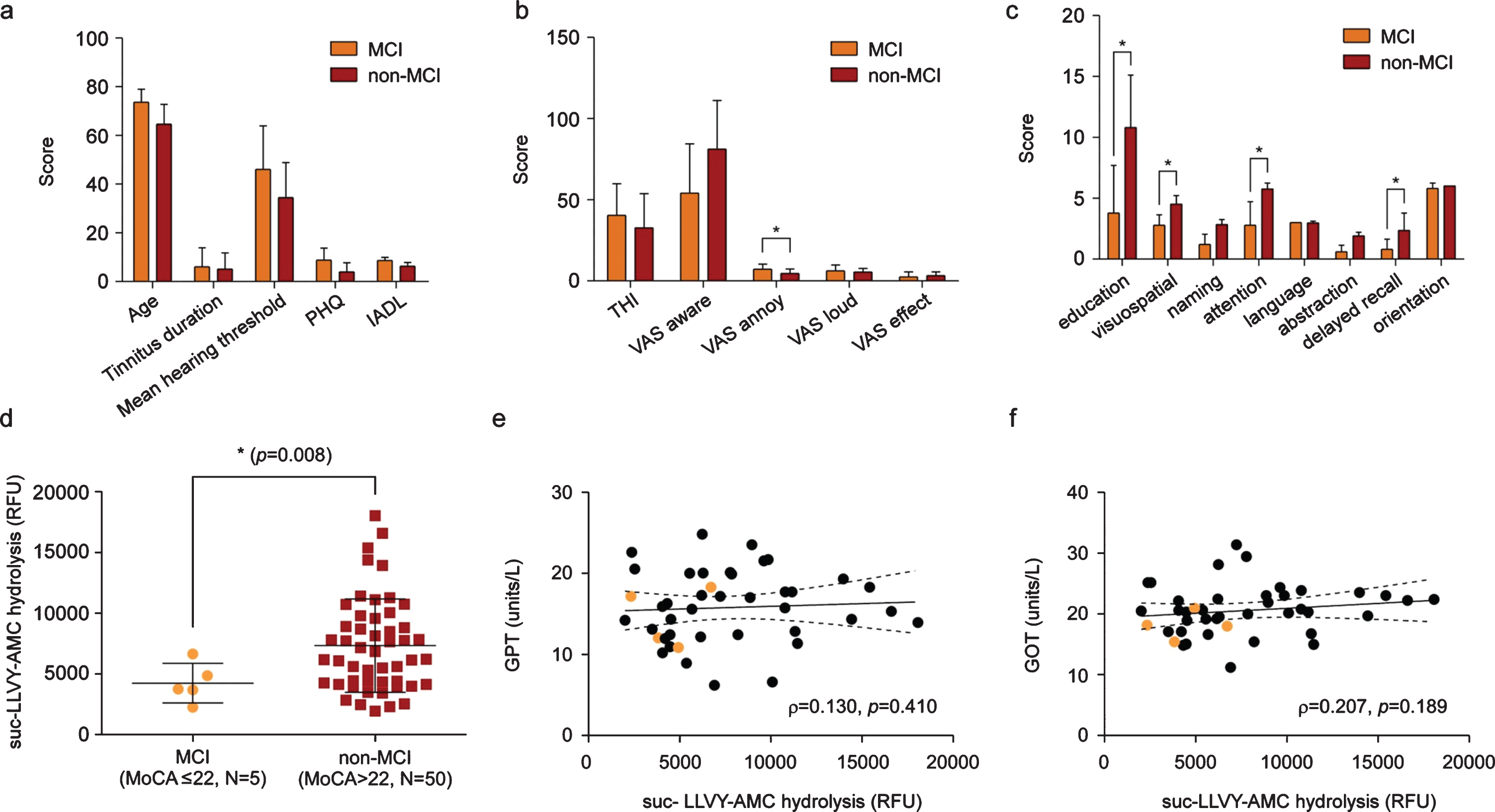
Using fluorogenic reporter substrates we employed in the previous assay, we measured c-proteasome activity from patients and found a significantly reduced plasma c-proteasome activity in patients in the MCI group (N = 5), compared with patients in the non-MCI group (N = 50, p = 0.008 by t-test with Welch’s correction; Fig. 3d). No significant correlation was detected between c-proteasome and glutamic pyruvic transaminase (Fig. 3e) or glutamic oxaloacetic transaminase (Fig. 3f) activities, suggesting that c-proteasome activity in the plasma may be specifically linked to cognitive decline in patients with chronic tinnitus. Indeed, upon fluorogenic reporter substrates, the Control group consisting of healthy subjects had significantly higher plasma c-proteasome activity, as compared with the MCI (p = 0.009 by one-way ANOVA and post-hoc Tukey’s test) and non-MCI groups (p = 0.04 by one-way ANOVA and post-hoc Tukey’s test) (Supplementary Figure 1).
Moreover, these results implied that the changes of c-proteasome activity in the plasma might originate from active extracellular secretion [29, 34], but not from passive release from ruptured cells. MCI, especially in its amnestic form, is known to convert into AD dementia in 10–15% of the cases, requiring prompt diagnosis of cognitive deficits and eventual intervention to delay progression of AD. In this regard, our findings posed many interesting questions with implications for the diagnosis of MCI and its relationship to cognitive deterioration over time.
Significant correlation between c-proteasome activity with its plasma level
We initially predicted that reduced c-proteasome activity would originate from the “clogging” [35] of the active sites of the proteasome by excess loading of AD-related, non-degradable proteins. However, we found that c-proteasome levels, measured using enzyme-linked immunosorbent assay, dynamically varied and strongly correlated with plasma c-proteasome activity (Spearman’s correlation, N = 55, ρ= 0.366, and p = 0.006; Fig. 4a). These results reflected that the number of proteasomes in the extracellular space was directly responsible for the observed activity change in patients with MCI, instead of being mediated by other inhibitory mechanisms. The plasma proteasome concentrations presented here were similar to the results from other studies, ranging from 0.4–2.4μg/mL (equivalent to ∼0.5–3 nM of the 20S complexes) [29, 34, 36–38].
Fig. 4
Significant association between c-proteasome activity and the levels of Aβ40 or c-proteasomes in the plasma. a) Spearman’s correlation analysis revealed a strong positive correlation between c-proteasome activity and proteasome level in the plasma from patients with chronic tinnitus (N = 55, ρ= 0.366, p = 0.006). b) Significant negative correlation between Aβ40 concentration and c-proteasome activity in the plasma (Spearman’s correlation: total N = 55, ρ= –0.266, p = 0.049). c) Negative correlation between Aβ40 and c-proteasome levels in the plasma from patients with chronic tinnitus (N = 55, ρ= –0.380, p = 0.004). The orange circles indicate samples from patients with MCI. Solid and dashed lines represent the regression line and 95% confidence intervals, respectively.
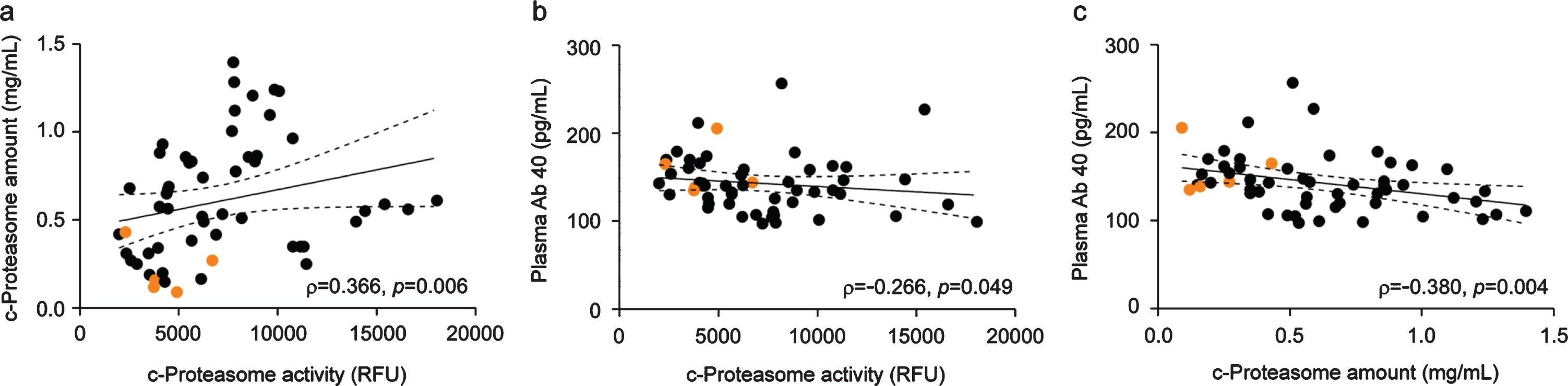
Unlike the clear association between Aβ levels in the cerebrospinal fluid and AD diagnosis, the association between Aβ levels in the plasma and the diagnosis of MCI and AD is disputable. Using the plasma of patients with chronic tinnitus (N = 55), we only found a modest, but not statistically significant, association of Aβ40 or Aβ42 levels with MoCA scores (Supplementary Figure 2A). However, we observed that plasma Aβ40 levels negatively correlated with c-proteasome activity and levels (p = 0.049 and 0.004, respectively, by Spearman correlation analysis; Fig. 4b, c). This result fits well with recent reports suggesting that Aβ is a proteasome substrate and that its degradation may be enhanced by specific molecules acting on gating 20S mechanisms [39]. Aβ42 levels also exhibited significant negative correlation with c-proteasomes activity (p = 0.026), but only little correlated with c-proteasome levels, possibly due to its lower concentration than Aβ40 in the plasma [40, 41] (Supplementary Figures 2B, C). Instead, Aβ42/Aβ40 ratios significantly correlated with c-proteasome amounts in human plasma (p = 0.033; Supplementary Figure 2C-ii). It is notable that ∼20-fold more proteasomes exist in the plasma than Aβ peptides (∼0.7 nM versus ∼0.04 nM from our study). Although a study with a larger cohort population is required to confirm these results, our data collectively suggest that c-proteasome activity from blood plasma, which is significantly correlated with the MCI status and Aβ40 levels, may have prognostic relevance as a biomarker of cognitive decline.
DISCUSSION
To the best of our knowledge, the present study is the first to explore the role of c-proteasome activity and its concentration in determining the presence of MCI among patients with chronic tinnitus. Notably, the findings presented herein replicate those of mouse AD models overexpressing mutant tau, demonstrating that c-proteasome activity from patients with chronic tinnitus and MCI was significantly reduced. What merited our attention was the fact that c-proteasome activity in patients with chronic tinnitus was largely dependent on its concentration in blood plasma. Given this, we speculate that cellular proteasomes can be actively released via unconventional secretory mechanisms [42], and that these proteasomes, like the 20S complex, may play a critical role in the selective degradation of misfolded or aggregation-prone proteins in the extracellular space. As proteasome concentration in the cell is relatively high (∼150 nM in the cytoplasm and ∼900 nM in the nucleus [43], which is at least >200-fold higher than the c-proteasome concentration measured in this study), a dynamic alteration of c-proteasome concentration is readily achievable by activating these exporting system. Considering the size of 20S proteasomes as a cargo molecule, they are expected to be packaged into membrane-bound organelles, such as autophagosomes, lysosomes, or exosomes, for unconventional extracellular secretion [42].
Although it is known that pathologic Aβ and tau in the peripheral blood mainly originate from the brain through the disrupted blood-brain barrier, the origin of c-proteasomes remains unclear. Reduced c-proteasome level and activity in patients with MCI are possibly related with defective proteasome secretion via membrane-less vesicles when cells are under chronic proteostatic stress. Alternatively, in light of the fact that intracellular proteasome activity was significantly reduced in postmortem human brains affected with AD and other neurodegenerative diseases [44, 45], it might be possible that the secretion mechanism is intact but only inactivated proteasomes as a result of disrupted blood-brain barrier might be secreted. The origin of c-proteasomes remains to be determined. Reduced c-proteasome level and activity in patients with MCI are possibly related the intact, fully-functional complex to individual substrates. Therefore, changes in c-proteasomes activity may function as a surrogate marker that allows to predict the progression of pathologic protein accumulation inside the cell.
It is worth noting that tinnitus mostly stems from hearing loss. As the human brain works in a Bayesian manner to minimize uncertainty for external stimuli, the deafferented brain due to hearing loss attempts to obtain or “fill in” the missing auditory information. This results in the perception of auditory phantoms (i.e., tinnitus) [12]. Recent studies have shown that AD mice also manifest hearing loss, suggesting that the AD pathology could occur in the auditory neural pathway [46–48]. Specifically, APP/PS1 AD mice had an early onset of hearing loss, which was detectable as early as 2 months in the high-frequencies [48]. Given this, AD mice may exhibit the auditory phenotype with tinnitus, similar to that in our prospectively recruited chronic tinnitus cohort. However, we could detect a significant correlation between c-proteasome activity between wild-type and AD model mice only after they became 12 months old (Fig. 1d). Therefore, there is a discrepancy in terms of the manifestation state of c-proteasome activity reduction between mouse and human. Whether this originates from the intrinsic limitation of transgenic AD models or other mechanisms such as c-proteasome release and clearance is to be determined.
With a growing body of evidence indicating a potential association between tinnitus and cognitive deficits, chronic tinnitus, which typically results from auditory deafferentation, might be implicated by the dysfunctional protein quality mechanism in the circulatory system. Interestingly, a recent animal study showed that proteasome inhibitor increases auditory hair cell and neuronal damages, which is mediated by the extent of peroxisome dysfunction [49]. Since peroxisome dynamics acts as an antioxidant defense mechanism against oxidative stress, such as noise exposure, the reduced proteasome activity as evidenced by MCI group may be more sensitive to peroxisome dysfunction, which, in turn, results in auditory hair cell and neuronal damages even in an environment with ambient background noise [50]. These findings are in line with several clinical reports where hearing loss and tinnitus are often, if not always, manifested among patients treated with the proteasome inhibitor bortezomib [51]. Although the specific origin and secretion mechanism that accounts for the reduced c-proteasome activity in the MCI group remain elusive, a damaged ubiquitin-proteasome system due to disrupted proteostasis may contribute to auditory phenotypes, such as hearing loss and tinnitus.
There are several limitations that should be addressed in the follow-up clinical studies. First, we enrolled tinnitus subjects with normal hearing to moderate hearing loss. Although we aimed to minimize the confounding effect of hearing loss, mild or moderate hearing loss has been shown to associate with cognitive impairment, leading to dementia [52, 53]. Second, the present study was designed to be a cross-sectional study consisting of a relatively small number of cases. The small number of cases in the MCI group may cause a bias in obtaining significant statistical power. To validate the exact role of c-proteasome activity for predicting cognitive deterioration, a prospective study from a large population is currently in progress. Lastly, the specific origin of c-proteasomes in the plasma remains to be determined. Given the reciprocal relationship between tinnitus and cognition in terms of auditory selective attention and working memory [54], it would be desirable to include a control group consisting of patients with MCI who do not show audiological phenotypes.
Nevertheless, our results pave the way for understanding the potential relationship between c-proteasome activity and MCI, and potentially early-stage AD. A simple measurement of c-proteasome activity, as a non-invasive blood-based biomarker, may provide an important tool in the diagnosis of cognitive dysfunction and selection of subjects requiring its prevention or treatment among patients with chronic tinnitus. Considering the importance of proteasomes in protein quality control, it will be of great interest to understand their ability in the extracellular region to promote the clearance of aggregation-prone proteins and their pathophysiological roles.
ACKNOWLEDGMENTS
This work was supported by grants from the National Research Foundation (2016M3C7A1913895, 2019R1A2B5B02069530, and 2020R1A5A1019023 to M.J.L., 2016M3C7A1913845 to Y.K.K., 2019R1A6A3A01094785 to W.H.C., and 2019R1A2C1005987 to J.H.L.), the National Research Council of Science & Technology (CRC-15-04-KIST to Y.K.K) the Convergence Research Grant through Seoul Municipal Boramae Medical Center (02-2018-5 to Y.H.K.), the Basic-Clinical Collaborative Research grant through Seoul National University College of Medicine (800-20190257 to Y.H.K. and 800-20190258 to M.J.L.), and the Creative-Pioneering Researchers Program through Seoul National University (to M.J.L.).
Authors’ disclosures available online (https://www.j-alz.com/manuscript-disclosures/20-0728r2).
SUPPLEMENTARY MATERIAL
[1] The supplementary material is available in the electronic version of this article: https://dx.doi.org/10.3233/JAD-200728.
REFERENCES
[1] | Glickman MH , Ciechanover A ((2002) ) The ubiquitin-proteasome proteolytic pathway: Destruction for the sake of construction. Physiol Rev 82: , 373–428. |
[2] | Finley D ((2009) ) Recognition and processing of ubiquitin-protein conjugates by the proteasome. Annu Rev Biochem 78: , 477–513. |
[3] | Taggart CC , Lowe GJ , Greene CM , Mulgrew AT , O’Neill SJ , Levine RL , McElvaney NG ((2001) ) Cathepsin B, L, and S cleave and inactivate secretory leucoprotease inhibitor. J Biol Chem 276: , 33345–33352. |
[4] | Smith DM , Kafri G , Cheng Y , Ng D , Walz T , Goldberg AL ((2005) ) ATP binding to PAN or the 26S ATPases causes association with the 20S proteasome, gate opening, and translocation of unfolded proteins. Mol Cell 20: , 687–698. |
[5] | Wyatt AR , Yerbury JJ , Poon S , Wilson MR ((2009) ) Therapeutic targets in extracellular protein deposition diseases. Curr Med Chem 16: , 2855–2866. |
[6] | Mueller O , Anlasik T , Wiedemann J , Thomassen J , Wohlschlaeger J , Hagel V , Keyvani K , Schwieger I , Dahlmann B , Sure U , Sixt SU ((2012) ) Circulating extracellular proteasome in the cerebrospinal fluid: A study on concentration and proteolytic activity. J Mol Neurosci 46: , 509–515. |
[7] | Ma W , Kantarjian H , O’Brien S , Jilani I , Zhang X , Estrov Z , Ferrajoli A , Keating M , Giles F , Albitar M ((2008) ) Enzymatic activity of circulating proteasomes correlates with clinical behavior in patients with chronic lymphocytic leukemia. Cancer 112: , 1306–1312. |
[8] | Jakob C , Egerer K , Liebisch P , Turkmen S , Zavrski I , Kuckelkorn U , Heider U , Kaiser M , Fleissner C , Sterz J , Kleeberg L , Feist E , Burmester GR , Kloetzel PM , Sezer O ((2007) ) Circulating proteasome levels are an independent prognostic factor for survival in multiple myeloma. Blood 109: , 2100–2105. |
[9] | Bellia F , Lanza V , Garcia-Vinuales S , Ahmed I , Pietropaolo A , Iacobucci C , Malgieri G , D’Abrosca G , Fattorusso R , Nicoletti V ((2019) ) Ubiquitin binds the amyloid β peptide and interferes with its clearance pathways. Chem Sci 10: , 2732–2742. |
[10] | Lee K-Y , Chen T-T , Chiang L-L , Chuang H-C , Feng P-H , Liu W-T , Chen K-Y , Ho S-C ((2017) ) Proteasome activity related with the daily physical activity of COPD patients. Int J Chron Obstruct Pulmon Dis 12: , 1519–1525. |
[11] | Kakurina G , Cheremisina O , Choinzonov E , Kondakova I ((2017) ) Circulating proteasomes in the pathogenesis of head and neck squamous cell carcinoma. Bull Exp Biol Med 163: , 92–94. |
[12] | Lee SY , Nam DW , Koo JW , De Ridder D , Vanneste S , Song JJ ((2017) ) No auditory experience, no tinnitus: Lessons from subjects with congenital- and acquired single-sided deafness. Hear Res 354: , 9–15. |
[13] | Langguth B , Kreuzer PM , Kleinjung T , De Ridder D ((2013) ) Tinnitus: Causes and clinical management. Lancet Neurol 12: , 920–930. |
[14] | Trevis KJ , McLachlan NM , Wilson SJ ((2016) ) Cognitive mechanisms in chronic tinnitus: Psychological markers of a failure to switch attention. Front Psychol 7: , 1262. |
[15] | Zarenoe R , Hallgren M , Andersson G , Ledin T ((2017) ) Working memory, sleep, and hearing problems in patients with tinnitus and hearing loss fitted with hearing aids. J Am Acad Audiol 28: , 141–151. |
[16] | Rossiter S , Stevens C , Walker G ((2006) ) Tinnitus and its effect on working memory and attention. J Speech Lang Hear Res 49: , 150–160. |
[17] | Lee SY , Lee JY , Han SY , Seo Y , Shim YJ , Kim YH ((2020) ) Neurocognition of aged patients with chronic tinnitus: Focus on mild cognitive impairment. Clin Exp Otorhinolaryngol 13: , 8–14. |
[18] | Langa KM , Levine DA ((2014) ) The diagnosis and management of mild cognitive impairment: A clinical review. JAMA 312: , 2551–2561. |
[19] | Levey A , Lah J , Goldstein F , Steenland K , Bliwise D ((2006) ) Mild cognitive impairment: An opportunity to identify patients at high risk for progression to Alzheimer’s disease. Clin Ther 28: , 991–1001. |
[20] | Kim Y , Yoo YK , Kim HY , Roh JH , Kim J , Baek S , Lee JC , Kim HJ , Chae MS , Jeong D , Park D , Lee S , Jang H , Kim K , Lee JH , Byun BH , Park SY , Ha JH , Lee KC , Cho WW , Kim JS , Koh JY , Lim SM , Hwang KS ((2019) ) Comparative analyses of plasma amyloid-beta levels in heterogeneous and monomerized states by interdigitated microelectrode sensor system.eaav. Sci Adv 5: , 1388. |
[21] | Nation DA , Sweeney MD , Montagne A , Sagare AP , D’Orazio LM , Pachicano M , Sepehrband F , Nelson AR , Buennagel DP , Harrington MG , Benzinger TLS , Fagan AM , Ringman JM , Schneider LS , Morris JC , Chui HC , Law M , Toga AW , Zlokovic BV ((2019) ) Blood-brain barrier breakdown is an early biomarker of human cognitive dysfunction. Nat Med 25: , 270–276. |
[22] | Park JC , Han SH , Yi D , Byun MS , Lee JH , Jang S , Ko K , Jeon SY , Lee YS , Kim YK , Lee DY , Mook-Jung I ((2019) ) Plasma tau/amyloid-beta1-42 ratio predicts brain tau deposition and neurodegeneration in Alzheimer’s disease. Brain 142: , 771–786. |
[23] | Esquerda-Canals G , Montoliu-Gaya L , Guell-Bosch J , Villegas S ((2017) ) Mouse models of Alzheimer’s disease. J Alzheimers Dis 57: , 1171–1183. |
[24] | Shin S , Kim D , Song JY , Jeong H , Hyeon SJ , Kowall NW , Ryu H , Pae AN , Lim S , Kim YK ((2020) ) Visualization of soluble tau oligomers in TauP301L-BiFC transgenic mice demonstrates the progression of tauopathy. Prog Neurobiol 187: , 101782. |
[25] | Kim E , Park S , Lee JH , Mun JY , Choi WH , Yun Y , Lee J , Kim JH , Kang MJ , Lee MJ ((2018) ) Dual function of USP14 deubiquitinase in cellular proteasomal activity and autophagic flux. Cell Rep 24: , 732–743. |
[26] | Shin SK , Kim JH , Lee JH , Son YH , Lee MW , Kim HJ , Noh SA , Kim KP , Kim IG , Lee MJ ((2017) ) Docosahexaenoic acid-mediated protein aggregates may reduce proteasome activity and delay myotube degradation during muscle atrophy in vitro. Exp Mol Med 49: , e287. |
[27] | Kim JH , Kim A , Yun Y , Park S , Lee JH , Lee YS , Lee MJ ((2020) ) Reduced chronic restraint stress in mice overexpressing hyperactive proteasomes in the forebrain. Mol Brain 13: , 4. |
[28] | Lee JH , Shin SK , Jiang Y , Choi WH , Hong C , Kim DE , Lee MJ ((2015) ) Facilitated tau degradation by USP14 aptamers via enhanced proteasome activity. Sci Rep 5: , 10757. |
[29] | Zoeger A , Blau M , Egerer K , Feist E , Dahlmann B ((2006) ) Circulating proteasomes are functional and have a subtype pattern distinct from 20S proteasomes in major blood cells. Clin Chem 52: , 2079–2086. |
[30] | Choi WH , de Poot SA , Lee JH , Kim JH , Han DH , Kim YK , Finley D , Lee MJ ((2016) ) Open-gate mutants of the mammalian proteasome show enhanced ubiquitin-conjugate degradation. Nat Commun 7: , 10963. |
[31] | Elsasser S , Schmidt M , Finley D ((2005) ) Characterization of the proteasome using native gel electrophoresis. Methods Enzymol 398: , 353–363. |
[32] | Oddo S , Caccamo A , Shepherd JD , Murphy MP , Golde TE , Kayed R , Metherate R , Mattson MP , Akbari Y , LaFerla FM ((2003) ) Triple-transgenic model of Alzheimer’s disease with plaques and tangles: Intracellular Abeta and synaptic dysfunction. Neuron 39: , 409–421. |
[33] | Gengler S , Hamilton A , Holscher C ((2010) ) Synaptic plasticity in the hippocampus of a APP/PS1 mouse model of Alzheimer’s disease is impaired in old but not young mice. PLoS One 5: , e9764. |
[34] | Wada M , Kosaka M , Saito S , Sano T , Tanaka K , Ichihara A ((1993) ) Serum concentration and localization in tumor cells of proteasomes in patients with hematologic malignancy and their pathophysiologic significance. J Lab Clin Med 121: , 215–223. |
[35] | Thibaudeau TA , Anderson RT , Smith DM ((2018) ) A common mechanism of proteasome impairment by neurodegenerative disease-associated oligomers. Nat Commun 9: , 1097. |
[36] | Dutaud D , Aubry L , Henry L , Levieux D , Hendil KB , Kuehn L , Bureau JP , Ouali A ((2002) ) Development and evaluation of a sandwich ELISA for quantification of the 20S proteasome in human plasma. J Immunol Methods 260: , 183–193. |
[37] | Fukasawa H , Kaneko M , Niwa H , Matsuyama T , Yasuda H , Kumagai H , Furuya R ((2015) ) Circulating 20S proteasome is independently associated with abdominal muscle mass in hemodialysis patients. PLoS One 10: , e0121352. |
[38] | Egerer K , Kuckelkorn U , Rudolph PE , Ruckert JC , Dorner T , Burmester GR , Kloetzel PM , Feist E ((2002) ) Circulating proteasomes are markers of cell damage and immunologic activity in autoimmune diseases. J Rheumatol 29: , 2045–2052. |
[39] | Santoro AM , Lanza V , Bellia F , Sbardella D , Tundo GR , Cannizzo A , Grasso G , Arizzi M , Nicoletti VG , Alcaro S ((2020) ) Pyrazolones activate the proteasome by gating mechanisms and protect neuronal cells from β-amyloid toxicity. ChemMedChem 15: , 302–316. |
[40] | Park JC , Han SH , Cho HJ , Byun MS , Yi D , Choe YM , Kang S , Jung ES , Won SJ , Kim EH , Kim YK , Lee DY , Mook-Jung I ((2017) ) Chemically treated plasma Abeta is a potential blood-based biomarker for screening cerebral amyloid deposition. Alzheimers Res Ther 9: , 20. |
[41] | Chen TB , Lai YH , Ke TL , Chen JP , Lee YJ , Lin SY , Lin PC , Wang PN , Cheng IH ((2019) ) Changes in plasma amyloid and tau in a longitudinal study of normal aging, mild cognitive impairment, and Alzheimer’s disease. Dement Geriatr Cogn Disord 48: , 180–195. |
[42] | Kim J , Gee HY , Lee MG ((2018) ) Unconventional protein secretion - new insights into the pathogenesis and therapeutic targets of human diseases. J Cell Sci 131: , jcs213686. |
[43] | Pack CG , Yukii H , Toh-e A , Kudo T , Tsuchiya H , Kaiho A , Sakata E , Murata S , Yokosawa H , Sako Y , Baumeister W , Tanaka K , Saeki Y ((2014) ) Quantitative live-cell imaging reveals spatio-temporal dynamics and cytoplasmic assembly of the 26S proteasome. Nat Commun 5: , 3396. |
[44] | Keller JN , Hanni KB , Markesbery WR ((2000) ) Impaired proteasome function in Alzheimer’s disease. J Neurochem 75: , 436–439. |
[45] | Lopez Salon M , Morelli L , Castano EM , Soto EF , Pasquini JM ((2000) ) Defective ubiquitination of cerebral proteins in Alzheimer’s disease. J Neurosci Res 62: , 302–310. |
[46] | Wang S-E , Wu C-H ((2015) ) Physiological and histological evaluations of the cochlea between 3xTg-AD mouse model of Alzheimer’s diseases and R6/2 mouse model of Huntington’s diseases. Chin J Physiol 58: , 359–366. |
[47] | O’Leary TP , Shin S , Fertan E , Dingle RN , Almuklass A , Gunn RK , Yu Z , Wang J , Brown RE ((2017) ) Reduced acoustic startle response and peripheral hearing loss in the 5xFAD mouse model of Alzheimer’s disease. Genes Brain Behav 16: , 554–563. |
[48] | Liu Y , Fang S , Liu LM , Zhu Y , Li CR , Chen K , Zhao HB ((2020) ) Hearing loss is an early biomarker in APP/PS1 Alzheimer’s disease mice. Neurosci Lett 717: , 134705. |
[49] | Lee JN , Kim SG , Lim JY , Kim SJ , Choe SK , Park R ((2015) ) Proteasome inhibitors induce auditory hair cell death through peroxisome dysfunction. Biochem Biophys Res Commun 456: , 269–274. |
[50] | Mardones P , Hetz C ((2015) ) Peroxisomes get loud: A redox antidote to hearing loss. Cell 163: , 790–791. |
[51] | Argyriou AA , Iconomou G , Kalofonos HP ((2008) ) Bortezomib-induced peripheral neuropathy in multiple myeloma: A comprehensive review of the literature. Blood 112: , 1593–1599. |
[52] | Thomson RS , Auduong P , Miller AT , Gurgel RK ((2017) ) Hearing loss as a risk factor for dementia: A systematic review. Laryngoscope Investig Otolaryngol 2: , 69–79. |
[53] | van Boxtel MP , van Beijsterveldt CE , Houx PJ , Anteunis LJ , Metsemakers JF , Jolles J ((2000) ) Mild hearing impairment can reduce verbal memory performance in a healthy adult population. J Clin Exp Neuropsychol 22: , 147–154. |
[54] | Tegg-Quinn S , Bennett RJ , Eikelboom RH , Baguley DM ((2016) ) The impact of tinnitus upon cognition in adults: A systematic review. Int J Audiol 55: , 533–540. |