Supplemental Retinal Carotenoids Enhance Memory in Healthy Individuals with Low Levels of Macular Pigment in A Randomized, Double-Blind, Placebo-Controlled Clinical Trial
Abstract
Background:
There is a biologically plausible rationale whereby the dietary carotenoids lutein (L), zeaxanthin (Z), and meso-zeaxanthin (MZ), which are collectively referred to as macular pigment (MP) in the central retina (macula), support the maintenance of cognition via their antioxidant and anti-inflammatory properties.
Objective:
To investigate the impact of supplemental L, Z, and MZ on memory, executive function, and verbal fluency among healthy individuals with low MP levels.
Methods:
In this double-blind, placebo-controlled, randomized clinical trial, subjects (n = 91; mean±SD age = 45.42±12.40; % male = 51.6) consumed a daily formulation of 10 mg L, 10 mg MZ, and 2 mg Z (n = 45) or placebo (n = 46) for 12 months. Cognitive domains assessed included verbal and visual learning, immediate and delayed memory, executive function, and verbal fluency. MP and serum carotenoid concentrations of L, Z, and MZ were also measured.
Results:
Following 12-month supplementation, individuals in the active group exhibited statistically significant improvements in memory when compared to the placebo group (paired associated learning [PAL] memory score [rANOVA, p = 0.009]; PAL errors [rANOVA, p = 0.017]). Furthermore, the observed reduction in the number of errors made in the PAL task among those in the intervention group was positively and significantly related to observed increases in MP volume (p = 0.005) and observed increases in serum concentrations of L (p = 0.009).
Conclusion:
This randomized, double-blind, placebo-controlled clinical trial demonstrates a memory-enhancing effect of daily supplementation with L, Z, and MZ in healthy subjects with low MP at baseline. The implications of these findings for intellectual performance throughout life, and for risk of cognitive decline in later life, warrant further study.
INTRODUCTION
Cognitive function encompasses a wide array of mental faculties, including memory, perception, language, decision-making, planning, and reasoning. Throughout a cognitively healthy life, some aspects of cognition are known to improve while other elements are known to deteriorate [1]. For example, some facets of cognition, such as vocabulary, improve with the passage of time, while others (e.g., processing speed and working memory [short-term maintenance and manipulation of information involving executive processes, such as reasoning and decision-making]) gradually decline with age [2]. It is known that the brain is not fully developed until the third decade of life [3], and that during this time its development can be influenced by many factors, such as a new career, parenthood, and lack of sleep. However, the observation that healthy educated adults in their 20 s and 30 s exhibit subclinical signs of general cognitive decline, albeit under test conditions, suggest that the onset of decline is earlier than previously thought [4]. Indeed, the findings of a recent study involving >3,000 participants suggests that cognitive decline begins as early as 24 years of age [5].
There is reason to believe that optimization of cognitive function throughout life may reduce the risk, or delay the onset, of cognitive decline in later life. Recently, it has been suggested that approximately 35% of dementia cases are attributable to a combination of nine risk factors [6]. These include: education (to a maximum of age 11-12 years), mid-life hypertension, mid-life obesity, hearing loss, late-life depression, diabetes, physical inactivity, smoking, and social isolation. Importantly, educational attainment is strongly correlated with socio-economic status, which in turn is associated with better health outcomes (e.g., physical activity [7], tobacco use [8], and healthier diets [9]. There is a growing body of evidence that good nutrition is important for optimal cognition [10] and maintenance of cognition [11] and is also associated with reduced risk of Alzheimer’s disease (AD), the most common form of dementia, in later life [12]. Accordingly, the role of carotenoids in human health has attracted the attention of researchers in recent years, as has the possibility that these dietary compounds may protect against cognitive decline [13].
Carotenoids are naturally occurring plant pigments ubiquitous throughout nature and synthesized de novo by photosynthetic organisms (plants, algae, cyanobacteria) and some non-photosynthetic organisms (but, importantly, not by mammals) [14]. Carotenoids are classed as carotenes (which are pure hydrocarbons and contain no oxygen) or xanthophylls (which are oxygen derivatives and more polar than carotenes). Due to their powerful antioxidant and anti-inflammatory properties, carotenoids are now known to play an important role in human health. Indeed, a higher consumption of carotenoids has been shown to be related to a lower risk of chronic diseases, including cardiovascular disease and some cancers [15].
Of the 750+ carotenoids found in nature, 40–50 are found in the human diet [16] and ∼18 in human blood [17], and yet only three carotenoids (lutein [L], zeaxanthin [Z], and meso-zeaxanthin [MZ]) accumulate in the central retina (macula), where they are collectively referred to as macular pigment (MP). L is the dominant carotenoid in the macula’s periphery, whereas Z and MZ are dominant in the mid-periphery and epicenter, respectively, of this tissue [18]. In other words, and with exquisite biological selectivity, the central retina captures L, Z, and MZ, where these dietary compounds have been shown to play a crucial role in optimizing vision in healthy subjects [19] and in retarding progression of age-related degenerative disorders of neural tissue (i.e., age-related macular degeneration [AMD]) [20]. While it appears that MP augmentation is best achieved with the inclusion of MZ [21], to date, no attempt has been made to identify this carotenoid in human brain tissue; however, L and Z have been identified in the hippocampus, cerebellum, and frontal, occipital, and temporal cortices [22–24]. Accordingly, given that the retina is a component of the central nervous system (CNS), and given that at least two of the three macular carotenoids are found in the brain [22, 23] in concentrations that correlate positively and significantly with their respective retinal concentrations [24], and since concentrations of these carotenoids relate positively to cognitive performance in cognitively impaired [25] and in cognitively intact individuals [26], the potential role of these dietary compounds in optimizing and maintaining cognition warrants study. Furthermore, given the antioxidant, anti-inflammatory, neurosupportive, and neuroprotective properties of these dietary compounds [27], and given that AMD and AD share risk factors (e.g., age, family history of disease [28, 29], tobacco use [30], poorer consumption of fruits and vegetables resulting in poorer antioxidant status [31, 32]) and also share disease-defining histopathological features (e.g., accumulation of amyloid-β [Aβ] in the respectively affected tissues [33]), the possibility that supplementation with these carotenoids could prevent or delay the onset (or even ameliorate the course) of cognitive decline cannot and should not be ignored.
Given the putative role of carotenoids in cognition, and that MP is a biomarker for brain concentrations of its constituent carotenoids [24] and correlates with cognitive performance [26], we conducted a randomized, double-blind, placebo-controlled clinical trial of supplementation with these compounds among subjects with low baseline MP levels.
MATERIALS AND METHODS
The design and methodology of this clinical trial (the Central Retinal Enrichment Supplementation Trial [CREST]) have been described in detail previously [34]. For the study reported herein, data from the “CREST Normal” sample were analyzed. In brief, “CREST Normal” was a parallel group, double-blind, placebo-controlled, block-randomized clinical trial, which investigated the impact of daily supplementation with a formulation containing all three macular carotenoids (in a L:MZ:Z [mg] ratio of 10 : 10 : 2) on visual function among individuals (n = 91; mean±SD age = 45.42±12.40; % male = 51.6) free of retinal disease with low MP levels at baseline. Low MP was defined as central MP (i.e., MP at 0.23° of eccentricity) ≤0.55 optical density units (ODU). Eligibility criteria included: low MP; 18 years or older; a best-corrected visual acuity of 6/6 or better; no more than five diopters spherical equivalence of refraction; no diabetes mellitus; no ocular pathology; and no previous consumption of supplements containing L and/or Z and/or MZ.
Prior to enrolment, written informed consent was obtained from all individuals. Ethical approval was granted by the Research Ethics Committee of the Waterford Institute of Technology, Waterford, Ireland, and the Ethics Committee of the European Research Council. CREST adhered to the tenets of the declaration of Helsinki, and followed the full code of ethics with respect to recruitment, testing and data protection (Trial registration number: ISRCTN68270512). Following enrolment, individuals were randomized in a ratio of 1 : 1 (with no stratification using block randomization [35]) to either the active (10 mg L; 10 mg MZ; 2 mg Z in a sunflower oil suspension) or placebo (sunflower oil) intervention group. Individuals were instructed to consume one capsule per day with a meal. Study visits occurred at baseline and at 3-, 6-, and 12-month intervals at a single site. This paper reports secondary outcome measures of the “CREST Normal” Trial, which examined the impact of carotenoid intervention on cognitive function in healthy individuals. Of note, the report on the primary outcome has already been published [19]. As outlined in Nolan et al. [19], of the 105 individuals originally enrolled into “CREST Normal”, 10 were excluded prior to statistical analyses as they exceeded the baseline threshold to meet the a priori-declared criteria of “low” MP (see above). Baseline cognition data were not collected for 4 individuals, thus leaving a sample size of 91 for this analysis and report.
Demographic, lifestyle, medical, ocular, and dietary assessment
Demographic, lifestyle, medical, ocular, and dietary information were documented via questionnaire. Weekly consumption of carotenoid-rich foods (eggs, broccoli, corn, and dark green leafy vegetables) was recorded using a dietary L/Z screener developed by Professor Elizabeth Johnson, Tufts University, USA. The L and Z values used in the screener were those reported by Perry et al. [36]. The dietary scores generated were weighted for frequency of intake of the food and for bioavailability of L and Z within these foods. Height and weight measurements were recorded to calculate body mass index (BMI) (kg/m2).
Macular pigment measurement
MP was measured by dual-wavelength autofluorescence (AF) using the Spectralis HRA+OCT MultiColor (Heidelberg Engineering GmbH, Heidelberg, Germany). Pupillary dilation was performed prior to measurement and patient details were entered into the Heidelberg Eye Explorer (HEYEX version 1.7.1.0) software. Dual-wavelength AF in this device uses two excitation wavelengths, one that is well absorbed by MP (486 nm, blue), and one that is not (518 nm, green) [37]. The following acquisition parameters were used: high speed scan resolution, 2-s cyclic buffer size, internal fixation, 30-s movie and manual brightness control. Alignment, focus and illumination were first adjusted in infrared mode. Once the image was evenly illuminated, the laser mode was switched from infrared to blue plus green laser light AF. Using the HEYEX software, the movie images were aligned and averaged, and a MP density map was created. MP volume, calculated as MP average times the area under the curve out to 7° eccentricity, is reported here.
Serum carotenoid (L, Z, and MZ) analysis
Non-fasting blood samples were collected at each study visit by standard venipuncture techniques in 9 mL vacuette tubes (BD Vacutainer SST Serum Separation Tubes, Becton, Dickinson and Company, Plymouth, United Kingdom) containing a “Z Serum Sep Clot Activator”. Collection tubes were inverted at least five times to ensure thorough mixing of the clot activator. The blood samples were left to clot for 30 min at room temperature and then centrifuged at 725 g for 10 min in a GruppeGC12 centrifuge (Desaga Sarstedt, Hampshire, UK) to separate the serum from the whole blood. Following centrifugation, serum was transferred to light-resistant microtubes and stored at circa –80°C until the time of batch analysis. Serum carotenoid analysis was performed by high performance liquid chromatography (HPLC), using a method that has been described previously [38].
Cognitive assessment
Cognitive performance was assessed using the Cambridge Neuropsychological Test Automated Battery (CANTAB, Cambridge Cognition, Cambridge, UK) [39]. This computerized software program was performed on a touch-screen tablet PC and required a finger-operated response. CANTAB is one of the most widely employed cognition batteries and it has been shown to be sensitive in assessing cognitive performance for many population groups including healthy individuals [40], patients with neurodegenerative disorders such as AD [41], and individuals with psychiatric illnesses such as schizophrenia [42]. The CANTAB protocol [43] was followed in the administration of the test battery, which included the motor screening task (MOT) to assess comprehension, a modified version of verbal recognition memory task (VRM) and the paired associated learning task (PAL) to assess episodic memory, and an attention switching task (AST) to measure executive function [44]. An overview of the test battery is provided in Table 1. Phonemic fluency, the capacity to generate words beginning with specific letters, and semantic fluency, the capacity to generate words belonging to a particular category, were also assessed using the “FAS” and “Animal” tests, respectively. The FAS test required the individual to generate as many words as possible beginning with the letters F, A, and S, with a 1-min time limit per letter. The “Animal” tests required the individual to name as many animals as possible in 1 min [45]. A higher score was preferable for each of these short assessments.
Table 1
An overview of the tasks performed to assess cognition using CANTAB
Cognitive domain | Task | Description | Outcome measure | Desirable score |
Comprehension | MOT | The individual must touch the flashing cross shown in different locations on the screen. | Mean latency | Lower |
Mean total correct | Higher | |||
Mean total errors | Lower | |||
Executive function | AST | An arrow is displayed on one side of the screen (right or left) and pointed in one direction (also right or left). Each trial displays a cue at the top of the screen, which instructs the individual as to whether they must press the right or left hand button according to the “side on which the arrow appears” or the “direction in which the arrow is pointed”. Some trials display congruent stimuli (e.g., arrow on the right side of the screen pointing to the right), while other trials display incongruent stimuli, which require a higher cognitive ability (e.g., arrow on the right side of the screen pointing to the left). | Correct latency | Lower |
Percentage of correct trials | Higher | |||
Congruency cost | (see legend) | |||
Episodic memory (verbal stimuli) | VRM (modified version) | In the free recall format, the individual is presented with a sequence of 12 words. Immediately afterwards, the individual is asked to verbally recall as many words as possible from the list. This is repeated twice more. Following a 20-min delay, the individual is once again asked to verbally recall as many words as possible from the list presented to him/her earlier. | Immediate free recalls (trials 1–3) | Higher |
Total immediate free recall | Higher | |||
Intrusion errors (trials 1–3) | Lower | |||
Learning slope | Higher | |||
Delayed free recall | Higher | |||
Delayed intrusion errors | Lower | |||
Delayed recognition total | Higher | |||
Recognition false positives | Lower | |||
In the forced choice recall format, the individual is presented with a list of 24 words (one at a time), comprising the original 12 words and 12 distractor words. The individual is asked to select whether or not each word presented is part of the original list using yes/no prompts. | ||||
Episodic memory (visual stimuli) | PAL | Boxes are displayed on the screen and open one by one in a randomized order to reveal patterns hidden inside. The patterns are then displayed in the middle of the screen, one at a time, and the individual must touch the box where the pattern was originally located. If the individual makes an error, the patterns are re-presented to remind the individual of their locations. | Memory score | Higher |
Total errors | Lower | |||
Total errors stage 6 adjusted | Lower |
MOT, motor screening task; AST, attention switching task; VRM, verbal recognition memory; PAL, paired associated learning; Mean latency, the speed (ms) of response to the stimulus; Mean total correct, number of correct responses; Mean total errors, the distance between the center of the cross and the location touched; Correct latency, the speed (ms) of response to the stimulus; Percentage of correct trials, number of correct responses; Congruency cost, a positive score indicates that the individual is faster on congruent trials and a negative score indicates that the individual is faster on incongruent trials. Immediate free recalls (trials 1–3), total number of correct words recalled for trial 1,2 and 3; Total immediate free recall, the sum of trials 1–3; Intrusion errors (trials 1–3), total number of words recalled that did not appear in the list; Learning slope, trial 3 total minus trial 1 total, total score from trial 3 subtracted from trial 1; Delayed free recall, the total number of words correctly recalled after a 20-min delay; Delayed intrusion errors, total number of words recalled after a 20-min delay that did not appear in the list; Delayed recognition total, the total number of words correctly recognized; Recognition false positives, total number of times an individual mis-identified a distractor word as a correct word, memory score, number of patterns correctly located after the first trial, summed across the stages completed; Total errors, the adjusted score and includes an adjustment made for any stages not reached, allowing it to be comparable to all subjects even if the task was ended prematurely due to cognitive limitation; Total errors stage 6 adjusted, total errors made at the 6-pattern stage, adjusted for subjects who did not reach this stage.
Statistical analysis
The statistical package IBM SPSS version 22 was used, and the 5% significance level applied, for all analyses. We did not adjust for multiple tests. Results were expressed as means±standard deviations. Between-group differences were analyzed using Independent Samples t-tests or chi-square tests as appropriate. Repeated measures analyses of variance (rANOVA) was used to assess Time and/or Time-Group interaction effects between the active and placebo intervention groups for MP volume, serum concentrations of L, Z, and MZ and cognitive function variables. Time effects examine whether or not a response variable is different at the various time points of interest. Time-Group effects examine whether or not the time effect differs between the active and placebo intervention groups, and this is the key effect in these types of analyses, as it is testing the impact of the intervention. A general linear model was used to assess (for dependent variables serum L, Z, MZ, and MP volume) the potential impact of sex, smoking habits, and alcohol consumption on Time and Time-Group effects. Spearman’s rank correlation coefficient was used to investigate potential relationships between observed changes in cognitive scores and observed changes in MP volume and/or observed changes in serum concentrations of each of MP’s constituent carotenoids.
RESULTS
The baseline demographic, health and lifestyle, MP volume, serum carotenoid concentration, and cognition data are presented in Table 2. There were no statistically significant differences between active and placebo intervention groups for any of these baseline variables, with the exception of the semantic fluency score, which was significantly higher in the active intervention group (p = 0.001). No comprehension or sensorimotor difficulties were observed during the CANTAB assessment, as the MOT was successfully completed by all individuals. Therefore, subsequent cognitive assessments were deemed valid.
Table 2
Demographic, health and lifestyle, macular pigment, serum carotenoid, and cognition data of the active and placebo intervention groups
Variable | Active intervention (n = 45) | Placebo intervention (n = 46) | Sig. |
Demographic, health and lifestyle | |||
Age (y) | 44.38±11.57 | 46.43±13.21 | 0.432 |
BMI (kg/m2) | 27.34±4.72 | 26.22±4.67 | 0.280 |
Exercise (min/week) | 292±312 | 293±298 | 0.987 |
Diet (estimated L and Z intake) | 24±14 | 22±13 | 0.457 |
Serum L (μmol/l) | 0.248±0.155 | 0.247±0.126 | 0.971 |
Serum Z (μmol/l) | 0.051±0.038 | 0.052±0.039 | 0.916 |
Serum MZ (μmol/l) | 0.001±0.006 | 0.000±0.002 | 0.336 |
Sex (% male) | 48.9 | 54.3 | 0.602 |
Education (highest level %) | 0.998 | ||
Primary | 2.2 | 2.2 | |
Secondary | 20 | 19.6 | |
Higher (third level) | 77.8 | 78.3 | |
Smoking (%) | 0.750 | ||
Never | 46.7 | 45.7 | |
Past | 33.3 | 32.6 | |
Current | 17.8 | 21.7 | |
Alcohol frequency (%) | 0.227 | ||
Never | 4.5 | 2.2 | |
Special occasions | 6.8 | 19.6 | |
1-2 times/month | 25 | 21.7 | |
1-2 times/week | 63.6 | 52.2 | |
Every day | 0 | 4.3 | |
Macular pigment | |||
MP Volume | 4026±1301 | 3852±1563 | 0.564 |
Memory | |||
VRM Trial 1 immediate free recall | 8.31±1.87 | 8.41±1.80 | 0.791 |
VRM Trial 2 immediate free recall | 9.93±1.51 | 10.00±1.30 | 0.822 |
VRM Trial 3 immediate free recall | 10.47±2.57 | 10.61±1.14 | 0.734 |
VRM total immediate free recall | 28.71±4.77 | 29.02±3.65 | 0.728 |
VRM Trial 1 intrusion errors | 0.11±0.32 | 0.11±0.38 | 0.974 |
VRM Trial 2 intrusion errors | 0.07±0.25 | 0.02±0.15 | 0.301 |
VRM Trial 3 intrusion errors | 0.04±0.21 | 0.04±0.30 | 0.986 |
VRM learning slope | 2.16±2.57 | 2.17±1.37 | 0.966 |
VRM delayed free recall | 9.32±3.02 | 9.77±1.90 | 0.462 |
VRM delayed intrusion errors | 0.09±0.29 | 0±0 | 0.074 |
VRM delayed recognition total | 23.86±0.35 | 23.89±0.32 | 0.691 |
VRM recognition false positives | 0.02±0.15 | 0.07±0.25 | 0.334 |
PAL memory score | 19.23±4.93 | 20.44±4.46 | 0.231 |
PAL total errors | 21.50±28.20 | 17.22±16.93 | 0.382 |
PAL total errors stage 6 adjusted | 6.64±6.82 | 4.82±5.15 | 0.158 |
Executive function | |||
AST correct latency | 833.38±189.83 | 843.42±167.56 | 0.791 |
AST percent correct | 94.10±7.53 | 93.76±7.20 | 0.828 |
AST congruency cost | 98.53±97.66 | 122.67±87.56 | 0.220 |
Verbal fluency | |||
Phonemic fluency | 44.13±15.21 | 40.35±11.81 | 0.188 |
Semantic fluency | 23.76±6.70 | 19.85±4.02 | 0.001 |
Data displayed are mean±SD for numeric data and percentages for categorical data. BMI, body mass index; MP Volume, a volume of macular pigment calculated as macular pigment average times the area under the curve out to 8° eccentricity (measured using the Heidelberg Spectralis®); VRM, verbal recognition memory; PAL, paired associated learning; AST, attention switching task.
Change in outcome variables over time
MP and serum carotenoids
In Table 3, we report variables in this study for which we found a statistically significant Time or a Time-Group interaction effect. We found significant Time effects for 1) MP volume (baseline/exit 3982±1337/6540±2011); 2) serum concentrations of L (0.249±0.134/0.896±0.584μmol/l); and 3) serum concentrations of MZ (0.001±0.005/0.036±0.040μmol/l). We also found significant Time-Group interaction effects for these variables (i.e., each increased significantly in the active intervention group in comparison to the placebo group): MP volume (active/placebo groups at exit 4026±1758/3875±1927); serum L (active/placebo groups at exit 0.283±0.133/0.272±0.129μmol/l); and serum MZ (active/placebo groups at exit 0.036±0.040/0.000±0.000μmol/l) (rANOVA, p < 0.001, for all). Serum concentrations of Z did also increase in the active intervention group (baseline/exit 0.052±0.042/0.086±0.050μmol/l) and remained stable in the placebo group (0.058±0.037/0.063±0.054μmol/l), but the Time-Group interaction effect was borderline (rANOVA, p = 0.082).
Table 3
Repeated measures analysis of variance of macular pigment, serum carotenoid concentrations (L, Z, and MZ) and cognitive variables with a statistically significant time and/or time-group interaction effect for active and placebo intervention groups
Variable | Active intervention | Placebo intervention | ||||||||
n | Baseline | 12-months | % Δ | n | Baseline | 12-months | % Δ | Time | Time x Group | |
M±SD | M±SD | M±SD | M±SD | |||||||
MP volume | 38 | 3982±1337 | 6540±2011 | 64.24 | 31 | 4026±1758 | 3875±1927 | –3.75 | <0.001 | <0.001 |
Serum L | 35 | 0.249±0.134 | 0.896±0.584 | 259.84 | 29 | 0.283±0.133 | 0.272±0.129 | –3.89 | <0.001 | <0.001 |
Serum Z | 34 | 0.052±0.042 | 0.086±0.050 | 65.39 | 30 | 0.058±0.037 | 0.063±0.054 | 8.62 | 0.016 | 0.082 |
Serum MZ | 34 | 0.001±0.005 | 0.036±0.040 | 3500 | 30 | 0.000±0.000 | 0.000±0.000 | – | <0.001 | <0.001 |
VRM delayed intrusion errors | 26 | 0.12±0.33 | 0.04±0.20 | –66.67 | 24 | 0.000±0.000 | 0.21±0.51 | 0.21 | 0.309 | 0.030 |
PAL memory score | 35 | 18.91±4.96 | 20.77±4.57 | 9.84 | 31 | 21.26±3.52 | 20.32±4.57 | –4.42 | 0.376 | 0.009 |
PAL total errors stage 6 | 36 | 6.78±7.10 | 3.17±4.52 | –53.25 | 31 | 4.19±3.82 | 4.48±4.89 | 6.92 | 0.040 | 0.017 |
AST correct latency | 37 | 832±191.86 | 751.63±191.70 | –9.66 | 31 | 841.41±158.95 | 775.38±217.58 | –66.03 | <0.001 | 0.695 |
AST percent correct | 37 | 93.71±8.14 | 96.84±3.49 | 3.34 | 31 | 93.89±7.57 | 95.02±7.61 | 1.13 | 0.004 | 0.164 |
AST congruency cost | 37 | 98.46±103.10 | 94.43±70.01 | –4.09 | 31 | 128.10±99.07 | 72.74±86.65 | –55.36 | 0.019 | 0.041 |
Phonemic fluency | 36 | 44.44±15.49 | 50.11±15.55 | 12.76 | 30 | 40.23±11.84 | 45.93±10.91 | 14.17 | <0.001 | 0.986 |
Data displayed are mean±SD for numeric data and percentages for categorical data. % Δ, percentage change; MP Volume, a volume of macular pigment calculated as macular pigment average times the area under the curve out to 8° eccentricity (measured using the Heidelberg Spectralis®); Serums L, Z and MZ, serum carotenoid concentrations measured in μmol/l; VRM, verbal recognition memory; PAL, paired associated learning; AST, attention switching task.
Cognitive function variables
As seen in Table 3 and Fig. 1A and 1B (for examples), we found statistically significant Time-Group interaction effects for four cognitive variables, i.e., individuals consuming the active intervention demonstrated improvements in measures of cognitive function that were not seen in the placebo group. These were (baseline/exit score for active and placebo groups, respectively): VRM delayed intrusion errors (0.12±0.33/0.04±0.20 and 0.00±0.00/0.21±0.51); PAL memory score (18.91±4.96/20.77±4.57 and 21.26±3.52/20.32±4.57); PAL errors adjusted for stage 6 (6.78±7.10/3.17±4.52 and 4.19±3.82/4.48±4.49); AST congruency cost (98.46±103.10/94.43±70.01 and 128.10±99.07/72.74±86.65). Of note, improvements were mainly seen in tests assessing memory in this study, and the possible implications of these findings are discussed below. Of note, for three other cognitive tests (AST correct latency, AST percent correct and phonemic fluency) we found a statistically significant Time effect, but not a Time-Group effect, which suggests a learning effect in these measurements (i.e., the improvement did not differ between the groups and therefore was unlikely due to the intervention; rather, the individual probably performed better with the passage of time due to a learning effect).
Fig.1
(A) Box plots illustrating overall PAL memory score (A) and total PAL errors (adjusted for stage 6) (B) at baseline and 12-months by intervention group. 0-A, baseline for active intervention; 0, baseline; 12, 12-months; A, active intervention, P, placebo intervention; PAL, paired associated learning.
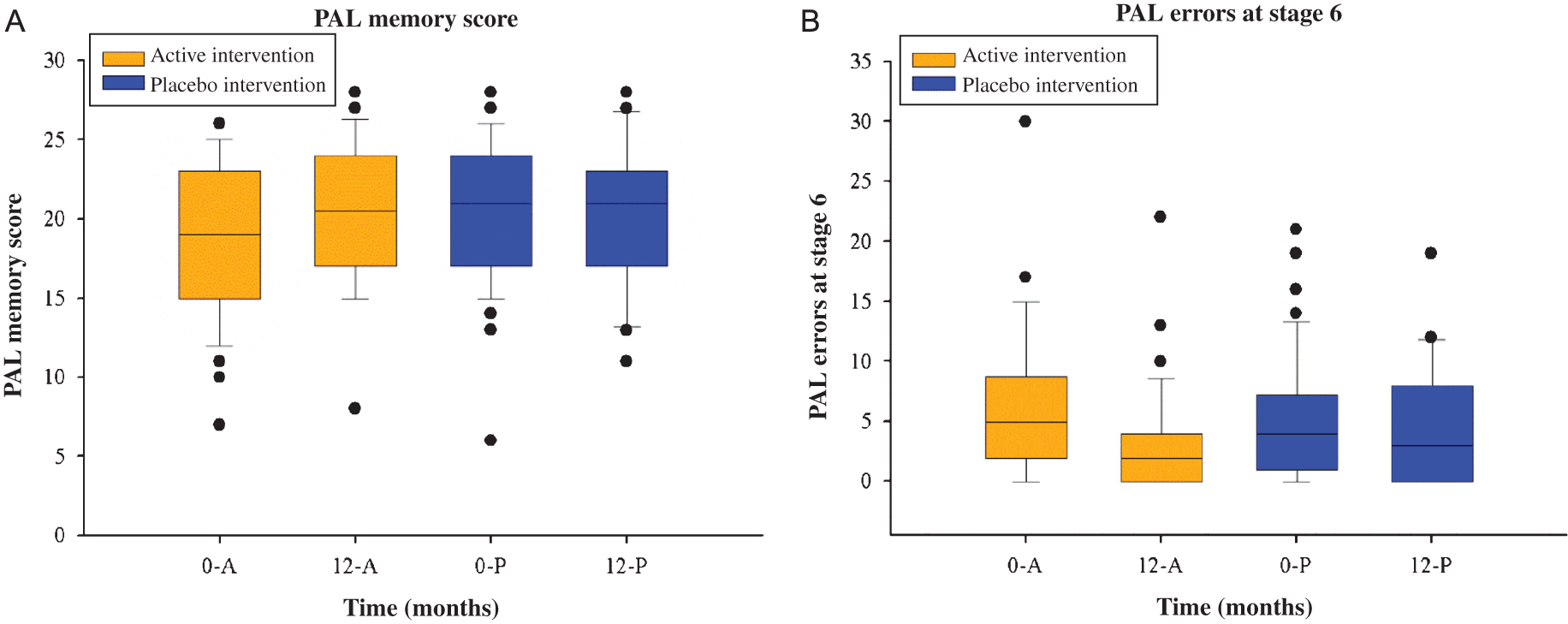
Effects of sex, smoking, and alcohol consumption
We also investigated, for statistically significant Time or Time-Group effects, possible interactions with other variables, namely sex, smoking habits, and alcohol consumption. The dependent variables in these analyses were serum L, Z, and MZ, and MP volume. No significant interactions were found (p > 0.05, for all). Thus, for example, changes in MP volume did not differ significantly between males and females, by smoking category, nor by alcohol consumption.
Relationship between change in MP/serum carotenoids and change in cognition
Spearman’s correlations were obtained in order to investigate whether observed changes in cognitive scores were related to observed changes in MP and/or observed changes in serum concentrations of L, and/or Z and/or MZ (See Table 4, and Fig. 2A and 2B for examples). Of interest, the observed reduction in total errors made at the 6-pattern stage of the PAL task was significantly related to the observed increases in MP volume (r = –0.342; p = 0.005; n = 67) and to the observed increases in serum concentrations of L (r = –0.346; p = 0.006; n = 61). Furthermore, the observed reduction in intrusion errors in the VRM delayed assessment was significantly related to the observed increases in MP volume (r = –0.306; p = 0.033; n = 49) and to the observed increases in serum concentrations of MZ (r = –0.304; p = 0.045; n = 44).
Table 4
Spearman’s correlation coefficients between observed change in MP and observed change in cognitive function variables
Observed change in MP | Observed change in VRM delayed intrusion and serum | Observed change in total PAL errors stage 6 errors | Observed change in PAL memory score | ||||||
R | p | n | R | p | n | R | p | n | |
MP volume | –0.306 | 0.033* | 49 | –0.342 | 0.005** | 67 | 0.219 | 0.078 | 66 |
Serum L | –0.189 | 0.220 | 44 | –0.346 | 0.006** | 61 | 0.159 | 0.226 | 60 |
Serum Z | 0.071 | 0.648 | 44 | –0.219 | 0.089 | 61 | 0.038 | 0.775 | 60 |
Serum MZ | –0.304 | 0.045* | 44 | –0.240 | 0.063 | 61 | 0.161 | 0.220 | 60 |
Observed change, exit visit data minus baseline visit data; MP volume (a volume of macular pigment calculated as macular pigment average times the area under the curve out to 8° eccentricity [measured using the Heidelberg Spectralis®]); Serums L, Z, and MZ, serum carotenoid concentrations measured in μmol/l; VRM, verbal recognition memory; PAL, paired associated learning; **Correlation is significant at the 0.01 level (2-tailed); *Correlation is significant at the 0.05 level (2-tailed).
Fig.2
(A) Scatter plot illustrating relationship between observed change in total PAL errors (adjusted for stage 6) and observed change in macular pigment (MP) volume (A) and in serum concentrations of lutein (B). Observed change, exit visit data minus baseline visit data; PAL, paired associated learning; MP volume (a volume of macular pigment calculated as macular pigment average times the area under the curve out to 8° eccentricity [measured using the Heidelberg Spectralis®]).
![(A) Scatter plot illustrating relationship between observed change in total PAL errors (adjusted for stage 6) and observed change in macular pigment (MP) volume (A) and in serum concentrations of lutein (B). Observed change, exit visit data minus baseline visit data; PAL, paired associated learning; MP volume (a volume of macular pigment calculated as macular pigment average times the area under the curve out to 8° eccentricity [measured using the Heidelberg Spectralis®]).](https://content.iospress.com:443/media/jad/2018/61-3/jad-61-3-jad170713/jad-61-jad170713-g002.jpg)
DISCUSSION
Given that MP levels correlate with concentrations of L and Z in brain tissue, and that retinal and serum concentrations of these carotenoids relate positively to cognitive function, this randomized, double-blind, placebo-controlled clinical trial was undertaken to examine the impact of supplemental L, Z, and MZ on memory, executive function and verbal fluency in healthy individuals.
Following carotenoid supplementation (in a L:MZ:Z [mg] ratio of 10 : 10 : 2) for a period of 12 months, individuals exhibited significant improvements in episodic memory. These findings are consistent with the literature, where positive associations between MP levels and cognitive function have been demonstrated [44, 46, 47]. With specific reference to memory assessments, a population-based, cross-sectional study (n = 4453) [26] showed that lower MP was associated with reduced likelihood of success on one prospective memory task (i.e., remembering to perform or recall a planned action or intention at some future point in time), but did not observe any significant associations between MP levels and immediate or delayed memory. The present study assessed episodic memory using the PAL task and a modified version of the VRM task. No significant improvements were observed for immediate memory, although individuals consuming the active intervention did commit significantly fewer intrusion errors during the delayed memory assessment (VRM delayed free recall) than subjects receiving placebo, but this latter finding should be interpreted with full appreciation that baseline error rates were low in each group (thus calling into question the clinical meaningfulness of this statistically significant finding). Individuals consuming the active intervention did, however, exhibit improvements in total PAL memory score, and a reduction in the number of errors made during this task. Interestingly, the PAL task has been shown to be an effective test of hippocampal connectivity and to be sensitive to subtle impairments (and fluctuations of impairment) in pre-symptomatic and mild stages of cognitive impairment [48]. The PAL 6-pattern error score, in particular, has been shown to discriminate between AD patients and non-demented (depressed and healthy) individuals, and to predict cognitive decline in sub-groups of memory-impaired patients, prompting investigators to postulate that the PAL may be particularly sensitive to entorhinal/hippocampal damage that characterizes AD because of its inherent need to combine object-based and contextual information [49]. Additionally, the PAL task has real-world, holistic relevance, having been shown to correlate positively with self-reported every day memory failures and with independent completion of activities of daily living [48].
In our study, observed increases in MP volume and in serum concentrations of L were significantly related to the observed reductions in total errors made at the 6-pattern stage of the PAL task, while observed increases in MP volume and in serum concentrations of MZ were significantly related to observed reductions in intrusion errors during the VRM delayed task. In a recent study by Feeney et al. [50], plasma concentrations of L and Z were each independently and positively related to measures of global cognition, memory and executive function in a cross-sectional analysis of a population-based cohort of 4,076 subjects. Additionally, serum concentrations of Z were noted to be significantly and positively related to processing speed in the same population [50]. A smaller scale cross-sectional study (n = 589) reported positive associations between cognitive function and plasma levels of Z [51]. Other data derived from population-based [22] and cross-sectional studies [52] (n = 43 to 220) have also reported positive associations between cognitive function and serum levels of L and Z.
In order to understand the potential implications of our findings it is important to discuss how they relate to episodic memory. Episodic memory refers to the ability to learn, store and retrieve information about experiences that occurred at a particular time and place, e.g., remembering where you parked your car in a multi-story car park [53]. Despite marked inter-individual variability, episodic memory is subject to age-related decline, which accelerates after age 60, as a result of changes in the relevant neurocircuitry, including neocortical regions, medial temporal lobe (MTL) regions, the hippocampus and adjacent cortical areas [54]. Reduced neuroplasticity, as a result of aging, causes a reduction in white and gray matter volume [55], as well as alterations in hippocampus-cortex connectivity, prefrontal-MTL connectivity (involved in encoding), and precunes (located in the medial parietal cortex)-MTL connectivity (involved in memory retrieval), which collectively and interactively contribute to impairments in episodic memory [54, 56]. This age-related decline in episodic memory is subject to provocation by several risk factors, including (but not limited to): fewer years of formal education; low socio-economic status; isolated environment and marital status; genetic background; low physical activity level; smoking; cardiovascular disease; and depressive symptoms [28, 57]. It should also be appreciated that poor episodic memory in cognitively normal adults is associated with increased risk for AD [58], and that age-related decline in episodic memory and AD share many of the aforementioned antecedents.
Optimization of episodic memory can improve social inclusion and cohesiveness, where individuals maintain their capacity to participate in, and continue to create memories of, life events, e.g., attending a family function [59], for subsequent retrieval. Additionally, optimal episodic memory is important for an individual’s level of risk awareness by using the memory of a previous experience to develop protective behaviors in future situations, e.g., giving your money to strangers or leaving the front door open [59]. Although an improvement in episodic memory favorably alters the risk profile for AD, it cannot be asserted that an improvement in episodic memory (as a result of, say, supplementation) necessarily negates the longstanding and pre-existing risk for going on to develop AD thereafter. Nevertheless, it is reasonable to hypothesize that the observed improvements in episodic memory reflect favorable changes in physiological functionality, structural integrity and synaptic activity of brain regions involved in memory, and that these favorable changes are attributable to the supplemental carotenoids as these compounds have been shown to be neurosupportive and neuroprotective in these respects (see below), and that this observed and desirable effect may have some long-term benefits [60, 61].
As noted, episodic memory is supported by a large network of brain areas including neocortical regions (prefrontal, parietal and temporal cortices) and components of the MTL, including parahippocampal regions and the hippocampus [62]. To date, L and Z have been identified in the hippocampus, cerebellum and frontal, occipital and temporal cortices [22–24]. The emerging view that the macular carotenoids are neuroprotective is premised upon their chemical composition (i.e., amount of conjugated double bonds and the presence of polar hydroxyl groups) and their localization within the lipid bilayer of the cell membrane, thus bestowing antioxidant and anti-inflammatory activity upon these compounds at loci where they are needed [18, 63], and thereby potentially mitigating processes involved in neurodegeneration. It has also been suggested that L can facilitate the transfer of compounds (e.g., molecules or nutrients) from one cell to another via gap junctions (i.e., intercellular membrane structures comprised of proteins known as connexions). Gap junction channels contribute to sharpened neuronal activity, which have been proposed to underlie cognitive processes such as memory, perception, and learning [64]. Other mechanisms whereby L and Z may play a neuroprotective and/or neurosupportive role have been proposed, and it has been suggested that these xanthophylls can enhance the structural integrity of membranes [61] and positively impact neural efficiency [47, 52]. This is not the first study to report improved cognition following supplementation with the macular carotenoids, and examples of what previous investigators have found in this respect include: improved verbal learning [52], verbal reasoning [61], verbal fluency and memory [65], as well as greater processing speed [47] and reduced reaction time [66].
While it was anticipated that the global prevalence of dementia will continue to rise [28, 67] (due to increases in average life expectancy), a number of population-based epidemiological studies have recently reported that the age-specific incidence and that the anticipated prevalence of dementia are actually declining in high-income countries [68–73]. While it is unclear whether or not this trend is set to continue (given that some studies report mixed results [74, 75]), this unexpected and observed decline merits comment as it seems to dispel the received wisdom that we are all predestined by an admixture of age and genetic background to ultimately develop dementia. Although the factors offsetting the anticipated rise in prevalence of dementia have yet to be identified, and in spite of the suggestion that rising levels of educational attainment and improved control of vascular risk factors may be playing a role in the welcome observation [70, 71], there is a consensus that a single variable is unlikely to account for the finding.
Over the last century or so, educational attainment has improved with each succeeding generation, reflected in the observation that the proportion of U.S. adults aged 65+ years with a high school diploma (12 years of education) increased from 53% in 1990 to 72% in 2003, and that the proportion with a college degree increased from 11% to 17% during the same period [76]. Wealth levels have also increased during the same period of time, reflected in a U.S. median household net worth of $108,000 and at $180,000 in 1989 and 2009, respectively, for individuals aged 65+ years [77]. Interestingly, higher educational attainment and higher income levels have been consistently associated with better cognitive function status [78, 79]. Moreover, education has been consistently associated with a reduced risk of dementia, and this observation has been putatively attributed to the beneficial impact of education on cognitive development, i.e., the cognitive reserve hypothesis [80], the implications for and consequences of associated greater wealth [81], ongoing mental stimulation, e.g., more challenging occupations [82] and a consequentially enhanced ability to navigate health care systems [83]. Importantly, education (a determinant of socio-economic status), is linearly associated with better health indicators including higher rates of physical activity [7], lower rates of tobacco use [8] and healthier diets [9, 84], and greater consumption of organic foods [85], all of which (while not denying the importance of hereditary factors) are believed to play a role in minimizing dementia risk [6]. Interestingly, a recent population-based study of 4,280 individuals identified education as an independent predictor of MP [9], and therefore to brain concentrations of its constituent carotenoids [24], thus representing a hitherto overlooked explanation whereby education protects against dementia risk in later life (see below).
But is it really plausible that nutrition is so important for cognition and its maintenance in later life, that the risk and clinical course of an age-related condition, such as AD, could be favorably modified by simple nutritional intervention? First, we here emphasize that neither the incidence nor the prevalence of dementia has turned out to be a mere function of age, as discussed above. Second, it is important to understand that the adult human nervous system, including the brain, is composed entirely of post-mitotic cells (i.e., cells which cannot divide and therefore cannot replace themselves), and is therefore more vulnerable to cumulative oxidative stress (as such cells must endure a lifetime of free radical injury because they are not replaced [86], thereby rendering a healthy neurocognitive system particularly dependent upon antioxidants that can and do cross the blood-brain barrier, e.g., vitamin C, L, Z) [87]. In other words, there is a sound rationale to postulate that the interaction of the aging brain and lifelong nutrition (rather than merely the aging brain, and notwithstanding other and acknowledged risk factors, such as genetic background) represents a determinant of whether and/or when a person goes on to develop dementia.
One of the most profound changes to everyday life in the last 100 years is the widespread availability of affordable foods, made possible by the advent of intensive farming. Over the last 70 years, the nutrient content of intensively farmed fruits and vegetables has plummeted [88, 89], a finding that is relevant to this study and to the current epidemic of AD. The catastrophic meltdown of the nutrient value of foodstuffs has occurred as a result of a process known as devolution, whereby genetic traits that are no longer required are lost or are not expressed, and this is attributable to genetic drift and/or gene-environment interaction (epigenetics) [90, 91]. A simple and dramatic example of devolution is pigmentation loss (due to differential gene expression of the Mc1r gene) in subterranean, deep-dwelling varieties of cavefish (where, because of darkness, no pigmentation is required), when compared with surface-dwelling varieties (where pigmentation is required) of the precise same species [92]. Indeed, this phenomenon is seen across animal and plant species, where non-essential traits are lost (through genetic drift or through epigenetic non-expression) in an attempt at energy conservation [93]. If evolution is an arms race, devolution is downsizing. Equally, the nutrient (as opposed to calorie) content of a given vegetable (e.g., vitamin C, L, Z) affords that vegetable protection against environmental stresses (e.g., oxidative stress) and is not there for the benefit of the herbivore/omnivore that consumes the vegetable (e.g., rabbit, humans, etc.), and these nutrients are surplus to need where fertilizers, insecticides and other growth facilitators are being employed, and devolution ensues with the result that the nutrient content of the vegetable depletes [94]. And given that a generation of crops is sewn and harvested, at least, annually, a period of 70 years allows for meaningful (d)evolution-driven phenotypic change (equivalent to ∼1,750 years of human [d]evolution, as a human generation is traditionally deemed to be 25 years; interestingly, significant changes in human height are observed in a mere fraction of such a time period [95]).
In other words, there is a biologically plausible hypothesis, underpinned by (albeit circumstantial) empirical evidence, that reconciles the observed increase in prevalence of AD over the last thirty years or so with the declining prevalence of AD in association with high-income in recent years. This hypothesis implicates declining nutrient content of commonly ingested foods over the last thirty years or so among lower socio-economic groups, is reflected in parallel finding in MP (a biomarker of CNS nutrition) and is consistent with the recently articulated notion that one third of cases of dementia worldwide could be averted by modification of lifestyle [96]. However, it is important to note that the current study was conducted in normal healthy subjects (and not in patients with AD). Therefore, the aforementioned links between nutrition and AD, while supported by our study, cannot be directly addressed by our work.
It is important to note that the results of this study are not necessarily generalizable to the overall population, given that CREST was a single-site study and a large proportion of the individuals enrolled into the study were recruited from the same geographical area. Another limitation of this study rests on the lack of a cognitive screening assessment prior to enrollment, thereby precluding the opportunity to apply inclusion/exclusion criteria on the basis of cognition. In an attempt to address this issue, baseline verbal fluency (phonemic and semantic) [97], and CANTAB data were compared with available normative data (PAL only) obtained from Cambridge Cognition (http://www.cambridgecognition.com). All individuals scored within the normal ranges for semantic, phonemic, and PAL variables, suggesting that they were free from cognitive impairment at baseline. Additionally (and as noted previously), all individuals successfully completed the MOT assessment, indicating that there were no comprehension or sensorimotor difficulties. It is also important to note that other nutrients related to cognitive health, e.g., omega-3 fatty acids, choline or vitamin B were not assessed in this study, but merit investigation in further studies. A major strength of this study rests on its design. CREST was a double-blind, randomized clinical trial, where neither the subjects nor researchers knew which individuals were consuming the active supplement and which subjects were consuming the placebo, and where allocation to the active treatment or placebo was randomized. In addition, CREST provided a unique opportunity to test whether observable, supplementation-induced changes in MP relate to observable changes in cognition in healthy adults with low MP (and therefore, putatively low brain concentrations of L and Z).
In conclusion, we have shown improvements in episodic memory among healthy individuals with low MP following supplementation for 12 months with a formulation containing the carotenoids L, Z, and MZ. These findings are in keeping with emerging and previous research, which has consistently shown a positive relationship between measures of MP and cognitive function. Given that MP levels correlate with L and Z concentrations in brain tissue, and that concentrations of these carotenoids relate positively to cognitive function, it is reasonable to hypothesize that these compounds assist in optimizing the neurocognitive environment. The implications of these findings for cognition in health and disease warrants further exploration.
ACKNOWLEDGMENTS
The CREST study was funded by the European Research Council; reference number 281096. We thank the CREST participants, and we also acknowledge Cambridge Cognition, UK, for guidance with respect to the assessment of cognitive function. We also thank the CREST Data Safety and Monitoring Committee (Professor James Loughman, Vision Scientist (Chairperson), Dr. Ailbhe Whyte, Medical Ophthalmologist, Dr. Michael Harrison Research Ethics Committee member, Frank Leonard, MSc, Statistician) for their support and guidance. We thank the Whitfield Clinic Pharmacy, Waterford, Ireland, for their support with randomization and intervention (Catherine Kelly and Lisa O’Brien). We would also like to thank Professor Elizabeth Johnson from Tuffs University, USA, for permission to use the dietary L/Z screener for estimating dietary intake of lutein and zeaxanthin in this study.
Authors’ disclosures available online (https://www.j-alz.com/manuscript-disclosures/17-0713r2).
REFERENCES
[1] | Hedden T , Garbrieli JDE ((2004) ) Insights into the ageing mind: A view from cognitive neuroscience. Nat Rev Neurosci 5: , 87–96. |
[2] | Harada CN , Natelson Love MC , Triebel KL ((2013) ) Normal cognitive aging. Clin Geriatr Med 29: , 737–752. |
[3] | Johnson SB , Blum RW , Giedd JN ((2009) ) Adolescent maturity and the brain: Theromise and pitfalls of neuroscience research in adolescent health policy. J Adolesc Health 45: , 216–221. |
[4] | Salthouse TA ((2009) ) When does age-related cognitive decline begin? Neurobiol Aging 30: , 507–514. |
[5] | Thompson JJ , Blair MR , Henrey AJ ((2014) ) Over the hill at 24: Persistent age-related cognitive-motor decline in reaction times in an ecologically valid video game task begins in early adulthood. PLoS One 9: , e94215. |
[6] | Livingston G , Sommerlad A , Orgeta V , Costafreda SG , Huntley J , Ames D , Ballard C , Banerjee S , Burns A , Cohen-Mansfield J , Cooper C , Fox N , Gitlin LN , Howard R , Kales HC , Larson EB , Ritchie K , Rockwood K , Sampson EL , Samus Q , Schneider LS , Selbaek G , Teri L , Mukadam N ((2017) ) Dementia prevention, intervention, and care. Lancet. http://dx.doi.org/10.1016/S0140-6736 (17)31756-31757 |
[7] | Talaei M , Rabiei K , Talaei Z , Amiri N , Zolfaghari B , Kabiri P , Sarrafzadegan N ((2013) ) Physical activity, sex, and socioeconomic status: A population based study. ARYA Atherscler 9: , 51–60. |
[8] | Hiscock R , Bauld L , Amos A , Fidler JA , Munafo M ((2012) ) Socioeconomic status and smoking: A review. Ann N Y Acad Sci 1248: , 107–123. |
[9] | Nolan JM , Feeney J , Kenny RA , Cronin H , O’Regan C , Savva GM , Loughman J , Finucane C , Connolly E , Meagher K , Beatty S ((2012) ) Education is positively associated with macular pigment: The Irish Longitudinal Study on Ageing (TILDA). Invest Ophthalmol Vis Sci 53: , 7855–7861. |
[10] | Valls-Pedret C , Sala-Vila A , Serra-Mir M , Corella D , de la Torre R , Martinez-Gonzalez MA , Martinez-Lapiscina EH , Fito M , Perez-Heras A , Salas-Salvado J , Estruch R , Ros E ((2015) ) Mediterranean diet and age-related cognitive decline: A randomized clinical trial. JAMA Intern Med 175: , 1094–1103. |
[11] | Pelletier A , Barul C , Feart C , Helmer C , Bernard C , Periot O , Dilharreguy B , Dartigues JF , Allard M , Barberger-Gateau P , Catheline G , Samieri C ((2015) ) Mediterranean diet and preserved brain structural connectivity in older subjects. Alzheimers Dement 11: , 1023–1031. |
[12] | Otaegui-Arrazola A , Amiano P , Elbusto A , Urdaneta E , Martinez-Lage P ((2014) ) Diet, cognition, and Alzheimer’s disease: Food for thought. Eur J Nutr 53: , 1–23. |
[13] | Guest J , Grant R ((2016) ) Carotenoids and Neurobiological Health. Adv Neurobiol 12: , 199–228. |
[14] | Alcaino J , Baeza M , Cifuentes V ((2016) ) Carotenoid distribution in nature. Subcell Biochem 79: , 3–33. |
[15] | Fiedor J , Burda K ((2014) ) Potential role of carotenoids as antioxidants in human health and disease. Nutrients 6: , 466–488. |
[16] | Khachik F ((2006) ) Distrubution and metabolism of dietary carotenoids in humans as a criterion for development of nutritional supplements. Pure Appl Chem 78: , 1551–1557. |
[17] | Khachik F , Beecher GR , Goli MB , Lusby WR , Smith JC Jr ((1992) ) Separation and identification of carotenoids and their oxidation products in the extracts of human plasma. Anal Chem 64: , 2111–2122. |
[18] | Landrum JT , Bone RA ((2001) ) Lutein, zeaxanthin, and the macular pigment. Arch Biochem Biophys 385: , 28–40. |
[19] | Nolan JM , Power R , Stringham J , Dennison J , Stack J , Kelly D , Moran R , Akuffo KO , Corcoran L , Beatty S ((2016) ) Enrichment of macular pigment enhances contrast sensitivity in subjects free of retinal disease: Central retinal enrichment supplementation trials – report 1. Invest Ophthalmol Vis Sci 57: , 3429–3439. |
[20] | Chew EY , Clemons TE , Sangiovanni JP , Danis RP , Ferris 3rd FL , Elman MJ , Antoszyk AN , Ruby AJ , Orth D , Bressler SB , Fish GE , Hubbard GB , Klein ML , Chandra SR , Blodi BA , Domalpally A , Friberg T , Wong WT , Rosenfeld PJ , Agron E , Toth CA , Bernstein PS , Sperduto RD ((2014) ) Secondary analyses of the effects of lutein/zeaxanthin on age-related macular degeneration progression: AREDS2 report No. 3. JAMA Ophthalmol 132: , 142–149. |
[21] | Akuffo KO , Nolan JM , Howard AN , Moran R , Stack J , Klein R , Klein BE , Meuer SM , Sabour-Pickett S , Thurnham DI , Beatty S ((2015) ) Sustained supplementation and monitored response with differing carotenoid formulations in early age-related macular degeneration. Eye (Lond) 29: , 902–912. |
[22] | Johnson EJ , Vishwanathan R , Johnson MA , Hausman DB , Davey A , Scott TM , Green RC , Miller LS , Gearing M , Woodard J , Nelson PT , Chung HY , Schalch W , Wittwer J , Poon LW ((2013) ) Relationship between serum and brain carotenoids, alpha-tocopherol, and retinol concentrations and cognitive performance in the oldest old from the Georgia Centenarian Study. J Aging Res 2013: , 951786. |
[23] | Craft NE , Haitema TB , Garnett KM , Fitch KA , Dorey CK ((2004) ) Carotenoid, tocopherol, and retinol concentrations in elderly human brain. J Nutr Health Aging 8: , 156–162. |
[24] | Vishwanathan R , Schalch W , Johnson EJ ((2016) ) Macular pigment carotenoids in the retina and occipital cortex are related in humans. Nutr Neurosci 19: , 95–101. |
[25] | Renzi LM , Dengler MJ , Puente A , Miller LS , Hammond BR Jr ((2014) ) Relationships between macular pigment optical density and cognitive function in unimpaired and mildly cognitively impaired older adults. Neurobiol Aging 35: , 1695–1699. |
[26] | Feeney J , Finucane C , Savva GM , Cronin H , Beatty S , Nolan JM , Kenny RA ((2013) ) Low macular pigment optical density is associated with lower cognitive performance in a large, population-based sample of older adults. Neurobiol Aging 34: , 2449–2456. |
[27] | Crichton GE , Bryan J , Murphy KJ ((2013) ) Dietary antioxidants, cognitive function and dementia–a systematic review. Plant Foods Hum Nutr 68: , 279–292. |
[28] | Alzheimer’s Association ((2016) ) 2016 Alzheimer’s disease facts and figures. Alzheimers Dement 12: , 459–509. |
[29] | Shahid H , Khan JC , Cipriani V , Sepp T , Matharu BK , Bunce C , Harding SP , Clayton DG , Moore AT , Yates JR ((2012) ) Age-related macular degeneration: The importance of family history as a risk factor. Br J Ophthalmol 96: , 427–431. |
[30] | Yu SS , Tang X , Ho YS , Chang RC , Chiu K ((2016) ) Links between the brain and retina: The effects of cigarette smoking-induced age-related changes in Alzheimer’s disease and macular degeneration. Front Neurol 7: , 119. |
[31] | Schleicher M , Weikel K , Garber C , Taylor A ((2013) ) Diminishing risk for age-related macular degeneration with nutrition: A current view. Nutrients 5: , 2405–2456. |
[32] | Trichopoulou A , Kyrozis A , Rossi M , Katsoulis M , Trichopoulos D , La Vecchia C , Lagiou P ((2015) ) Mediterranean diet and cognitive decline over time in an elderly Mediterranean population. Eur J Nutr 54: , 1311–1321. |
[33] | Ohno-Matsui K ((2011) ) Parallel findings in age-related macular degeneration and Alzheimer’s disease. Prog Retin Eye Res 30: , 217–238. |
[34] | Akuffo KO , Beatty S , Stack J , Dennison J , O’Regan S , Meagher KA , Peto T , Nolan J ((2014) ) Central Retinal Enrichment Supplementation Trials (CREST): Design and methodology of the CREST randomized controlled trials. Ophthalmic Epidemiol 21: , 111–123. |
[35] | Friedman L , Furberg C , Demets D ((2010) ) Fundamentals of Clinical Trials, Springer, New York. |
[36] | Perry A , Rasmussen H , Johnson EJ ((2009) ) Xanthophyll (lutein, zeaxanthin) content in fruits, vegetables and corn and egg products. J Food Compost Anal 22: , 9–15. |
[37] | Trieschmann M , Heimes B , Hense HW , Pauleikhoff D ((2006) ) Macular pigment optical density measurement in autofluorescence imaging: Comparison of one- and two-wavelength methods. Graefes Arch Clin Exp Ophthalmol 244: , 1565–1574. |
[38] | Meagher KA , Thurnham DI , Beatty S , Howard AN , Connolly E , Cummins W , Nolan JM ((2013) ) Serum response to supplemental macular carotenoids in subjects with and without age-related macular degeneration. Br J Nutr 110: , 289–300. |
[39] | Zygouris S , Tsolaki M ((2015) ) Computerized cognitive testing for older adults: A review. Am J Alzheimers Dis Other Demen 30: , 13–28. |
[40] | Hayat SA , Luben R , Moore S , Dalzell N , Bhaniani A , Anuj S , Matthews FE , Wareham N , Khaw KT , Brayne C ((2014) ) Cognitive function in a general population of men and women: A cross sectional study in the European Investigation of Cancer-Norfolk cohort (EPIC-Norfolk). BMC Geriatr 14: , 142. |
[41] | Nolan JM , Loskutova E , Howard A , Mulcahy R , Moran R , Stack J , Bolger M , Coen RF , Dennison J , Akuffo KO , Owens N , Power R , Thurnham D , Beatty S ((2015) ) The impact of supplemental macular carotenoids in Alzheimer’s disease: A randomized clinical trial. J Alzheimers Dis 44: , 1157–1169. |
[42] | Kim HS , An YM , Kwon JS , Shin MS ((2014) ) A preliminary validity study of the cambridge neuropsychological test automated battery for the assessment of executive function in schizophrenia and bipolar disorder. Psychiatry Investig 11: , 394–401. |
[43] | Cognition Limited ((2012) ) CANTAB Eclipse Test Administration Guide, Cambridge Cognition Limited, Cambridge, UK. |
[44] | Kelly D , Coen RF , Akuffo KO , Beatty S , Dennison J , Moran R , Stack J , Howard AN , Mulcahy R , Nolan JM ((2015) ) Cognitive function and its relationship with macular pigment optical density and serum concentrations of its constituent carotenoids. J Alzheimers Dis 48: , 261–277. |
[45] | Shao Z , Janse E , Visser K , Meyer AS ((2014) ) What do verbal fluency tasks measure? Predictors of verbal fluency performance in older adults. Front Psychol 5: , 772–782. |
[46] | Vishwanathan R , Iannaccone A , Scott TM , Kritchevsky SB , Jennings BJ , Carboni G , Forma G , Satterfield S , Harris T , Johnson KC , Schalch W , Renzi LM , Rosano C , Johnson EJ ((2014) ) Macular pigment optical density is related to cognitive function in older people. Age Ageing 43: , 271–275. |
[47] | Bovier ER , Hammond BR ((2015) ) A randomized placebo-controlled study on the effects of lutein and zeaxanthin on visual processing speed in young healthy subjects. Arch Biochem Biophys 572: , 54–57. |
[48] | Barnett JH , Blackwell AD , Sahakian BJ , Robbins TW ((2016) ) The Paired Associates Learning (PAL) Test: 30 tears of CANTAB translational neuroscience from laboratory to bedside in dementia research. Curr Top Behav Neurosci 28: , 449–474. |
[49] | Swainson R , Hodges JR , Galton CJ , Semple J , Michael A , Dunn BD , Iddon JL , Robbins TW , Sahakian BJ ((2001) ) Early detection and differential diagnosis of Alzheimer’s disease and depression with neuropsychological tasks. Dement Geriatr Cogn Disord 12: , 265–280. |
[50] | Feeney J , O’Leary N , Moran R , O’Halloran AM , Nolan JM , Beatty S , Young IS , Kenny RA ((2017) ) Plasma lutein and zeaxanthin areassociated with better cognitive function across multiple domainsin a large population-based sample of older adults: The IrishLongitudinal Study on Ageing. J Gerontol A Biol Sci Med Sci 72: , 1431–1436. |
[51] | Akbaraly NT , Faure H , Gourlet V , Favier A , Berr C ((2007) ) Plasma carotenoid levels and cognitive performance in an elderly population: Results of the EVA Study. J Gerontol A Biol Sci Med Sci 62: , 308–316. |
[52] | Lindbergh CA , Mewborn CM , Hammond BR , Renzi-Hammond LM , Curran-Celentano JM , Miller LS ((2017) ) Relationship of lutein and zeaxanthin levels to neurocognitive functioning: An fMRI study of older adults. J Int Neuropsychol Soc 23: , 11–22. |
[53] | Hasselmo ME ((2011) ) How We Remember: Brain Mechanisms of Episodic Memory, MIT Press, USA. |
[54] | Nyberg L ((2017) ) Functional brain imaging of episodic memory decline in ageing. J Intern Med 281: , 65–74. |
[55] | Peters R ((2006) ) Ageing and the brain. Postgrad Med J 82: , 84–88. |
[56] | Gallagher M , Koh MT ((2011) ) Episodic memory on the path to Alzheimer’s disease. Curr Opin Neurobiol 21: , 929–934. |
[57] | Olaya B , Bobak M , Haro JM , Demakakos P ((2017) ) Trajectories of verbal episodic memory in middle-aged and older adults: Evidence from the English Longitudinal Study of Ageing. J Am Geriatr Soc 65: , 1274–1281. |
[58] | Blacker D , Lee H , Muzikansky A , Martin EC , Tanzi R , McArdle JJ , Moss M , Albert M ((2007) ) Neuropsychological measures in normal individuals that predict subsequent cognitive decline. Arch Neurol 64: , 862–871. |
[59] | Allen TA , Fortin NJ ((2013) ) The evolution of episodic memory. Proc Natl Acad Sci U S A 110: (Suppl 2), 10379–10386. |
[60] | Zhang LX , Cooney RV , Bertram JS ((1991) ) Carotenoids enhance gap junctional communication and inhibit lipid peroxidation in C3H/10T1/2 cells: Relationship to their cancer chemopreventive action. Carcinogenesis 12: , 2109–2114. |
[61] | Zamroziewicz MK , Paul EJ , Zwilling CE , Johnson EJ , Kuchan MJ , Cohen NJ , Barbey AK ((2016) ) Parahippocampal cortex mediates the relationship between lutein and crystallized intelligence in health, older adults. Front Ageing Neurosci 8: , 297. |
[62] | Dickerson BC , Eichenbaum H ((2010) ) The episodic memory system: Neurocircuitry and disorders. Neuropsychopharmacology 35: , 86–104. |
[63] | Bian Q , Gao S , Zhou J , Qin J , Taylor A , Johnson EJ , Tang G , Sparrow JR , Gierhart D , Shang F ((2012) ) Lutein and zeaxanthin supplementation reduces photooxidative damage and modulates the expression of inflammation-related genes in retinal pigment epithelial cells. Free Radic Biol Med 53: , 1298–1307. |
[64] | Sohl G , Maxeiner S , Willecke K ((2005) ) Expression and functions of neuronal gap junctions. Nat Rev Neurosci 6: , 191–200. |
[65] | Johnson EJ , McDonald K , Caldarella SM , Chung HY , Troen AM , Snodderly DM ((2008) ) Cognitive findings of an exploratory trial of docosahexaenoic acid and lutein supplementation in older women. Nutr Neurosci 11: , 75–83. |
[66] | Renzi LM , Bovier ER , Hammond BR Jr ((2013) ) A role for the macular carotenoids in visual motor response. Nutr Neurosci 16: , 262–268. |
[67] | Prince M , Comas-Herrera A , Knapp M , Guerchet M , Karagiannidou M ((2016) ) World Alzheimer Report 2016. Improving Healthcare for People Living with Dementia. Coverage, Quality and Costs Now and Into the Future., Alzheimer’s Disease International, London, UK. |
[68] | Matthews FE , Arthur A , Barnes LE , Bond J , Jagger C , Robinson L , Brayne C ((2013) ) A two-decade comparison of prevalence of dementia in individuals aged 65 years and older from three geographical areas of England: Results of the Cognitive Function and Ageing Study I and II. Lancet 382: , 1405–1412. |
[69] | Langa KM , Larson EB , Crimmins EM , Faul JD , Levine DA , Kabeto MU , Weir DR ((2017) ) A comparison of the prevalence of dementia in the United States in 2000 and 2012. JAMA Intern Med 177: , 51–58. |
[70] | Satizabal C , Beiser AS , Seshadri S ((2016) ) Incidence of dementia over three decades in the Framingham Heart Study. N Engl J Med 375: , 93–94. |
[71] | Manton KC , Gu XL , Ukraintseva SV ((2005) ) Declining prevalence of dementia in the U.S. elderly population. Adv Gerontol 16: , 30–37. |
[72] | Dodge HH , Zhu J , Lee CW , Chang CC , Ganguli M ((2014) ) Cohort effectsin age-associated cognitive trajectories. J Gerontol A BiolSci Med Sci 69: , 687–694. |
[73] | Qiu C , von Strauss E , Backman L , Winblad B , Fratiglioni L ((2013) ) Twenty-year changes in dementia occurrence suggest decreasing incidence in central Stockholm, Sweden. Neurology 80: , 1888–1894. |
[74] | Prince M , Ali GC , Guerchet M , Prina AM , Albanese E , Wu YT ((2016) ) Recent global trends in the prevalence and incidence of dementia, and survival with dementia. Alzheimers Res Ther 8: , 23. |
[75] | Wu YT , Beiser AS , Breteler MMB , Fratiglioni L , Helmer C , Hendrie HC , Honda H , Ikram MA , Langa KM , Lobo A , Matthews FE , Ohara T , Peres K , Qiu C , Seshadri S , Sjolund BM , Skoog I , Brayne C ((2017) ) The changing prevalence and incidence of dementia over time – current evidence. Nat Rev Neurol 13: , 327–339. |
[76] | Federal Interagency Forum of Aging-Related Statistics, Educational attainment of the population age 65 and over, https://agingstats.gov/docs/PastReports/2004/OA2004.pdf |
[77] | Federal Interagency Forum of Aging-Related Statistics, Household net worth of head of household, https://agingstats.gov/docs/PastReports/2004/OA2004.pdf |
[78] | Goveas JS , Rapp SR , Hogan PE , Driscoll I , Tindle HA , Smith JC , Kesler SR , Zaslavsky O , Rossom RC , Ockene JK , Yaffe K , Manson JE , Resnick SM , Espeland MA ((2016) ) Predictors of optimal cognitive aging in 80+ women: The Women’s Health Initiative Memory Study. J Gerontol A Biol Sci Med Sci 71: (Suppl 1), S62–71. |
[79] | Miu J , Negin J , Salinas-Rodriguez A , Manrique-Espinoza B , Sosa-Ortiz AL , Cumming R , Kowal P ((2016) ) Factors associated with cognitive function in older adults in Mexico. Global Health Action 9: , 30747. |
[80] | Stern Y ((2012) ) Cognitive reserve in ageing and Alzheimer’s disease. Lancet Neurol 11: , 1006–1012. |
[81] | Cagney KA , Lauderdale DS ((2002) ) Education, wealth, and cognitive function in later life. J Gerontol B Psychol Sci Soc Sci 57: , P163–172. |
[82] | Smart EL ((2015) ) Occupational complexity and lifetime cognitive abilities. Neurology 84: , 1721. |
[83] | Zimmerman EB , Woolf SH , Haley A ((2015) ) Understanding therelationship between education and health: A review of the evidence and an examination of community perspectives. In Population Health: Behavioural and Social Sciences Insights, Kaplan RM, Spittel ML, David DH, eds. Agency for Healthcare Research and Quality and Office of Behavioural and Social Sciences Research, National Institute of Health, Rockville, MD. |
[84] | Bonaccio M , Di Castelnuovo A , Pounis G , Costanzo S , Persichillo M , Cerletti C , Donati MB , de Gaetano G , Iacoviello L ((2017) ) High adherence to the Mediterranean diet is associated with cardiovascular protection in higher but not in lower socioeconomic groups: Prospective findings from the Moli-sani study. Int J Epidemiol 46: , 1478–1487. |
[85] | Curl CL , Beresford SA , Hajat A , Kaufman JD , Moore K , Nettleton JA , Diez-Roux AV ((2013) ) Associations of organic produce consumption with socioeconomic status and the local food environment: Multi-Ethnic Study of Atherosclerosis (MESA). PLoS One 8: , e69778. |
[86] | Tokarz P , Kaarniranta K , Blasiak J ((2016) ) Role of the cell cycle re-initiation in DNA damage response of post-mitotic cells and its implication in the pathogenesis of neurodegenerative diseases. Rejuvenation Res 19: , 131–139. |
[87] | Niedzielska E , Smaga I , Gawlik M , Moniczewski A , Stankowicz P , Pera J , Filip M ((2016) ) Oxidative stress in neurodegenerative diseases. Mol Neurobiol 53: , 4094–4125. |
[88] | Davis DR , Epp MD , Riordan HD ((2004) ) Changes in USDA foodcomposition data for 43 garden crops, 1950 to 1999. J Am CollNutr 23: , 669–682. |
[89] | White PJ , Broadley MR ((2005) ) Historical variation in the mineral composition of edible horticultural products. J Hortic Sci Biotechnol 80: , 660–667. |
[90] | Ottman R ((1996) ) Gene-environment interaction: Definitions and study designs. Prev Med 25: , 764–770. |
[91] | Masel J ((2011) ) Genetic drift. Curr Biol 21: , R837–838. |
[92] | Gross JB , Borowsky R , Tabin CJ ((2009) ) A novel role for Mc1r in the parallel evolution of depigmentation in independent populations of the cavefish Astyanax mexicanus. PLoS Genet 5: , e1000326. |
[93] | Dawkins R ((1989) ) The Selfish Gene, Oxford University Press, Oxford. |
[94] | Thomas D ((2003) ) A study on the mineral depletion of the foods available to us as a nation over the period 1940 to 1991. Nutr Health 17: , 85–115. |
[95] | Styne DM , McHenry H ((1993) ) The evolution of stature in humans. Horm Res 39: (Suppl 3), 3–6. |
[96] | Barnes DE , Yaffe K ((2011) ) The projected effect of risk factor reduction on Alzheimer’s disease prevalence. Lancet Neurol 10: , 819–828. |
[97] | Tombaugh TN , Kozak J , Rees L ((1999) ) Normative data stratified by age and education for two measures of verbal fluency: FAS and animal naming. Arch Clin Neuropsychol 14: , 167–177. |