Evaluation of a DNA Aβ42 Vaccine in Aged NZW Rabbits: Antibody Kinetics and Immune Profile after Intradermal Immunization with Full-Length DNA Aβ42 Trimer
Abstract
A pathological hallmark of Alzheimer’s disease (AD) are amyloid plaques in the brain consisting of aggregated amyloid-β 42 peptide (Aβ42) derived from cellular amyloid-β protein precursor (AβPP). Based on successful experiments in mouse AD models, active immunization with Aβ42 peptide and passive immunizations with anti-Aβ42 antibodies were started in clinical trials. Active Aβ42 peptide immunization in humans had led to an inflammatory autoimmune response, and the trial was stopped. Passive immunizations had shown some effects in slowing AD pathology. Active DNA Aβ42 immunizations administered with the gene gun into the skin elicits a different immune response and is non-inflammatory. While in rodents, good responses had been found for this type of immunization, positive results in larger mammals are missing. We present here results from sixteen New Zealand White Rabbits, which underwent intradermal DNA Aβ42 immunization via gene gun. The humoral immune response was analyzed from blood throughout the study, and cellular immune responses were determined from spleens at the end of the study. A good anti-Aβ antibody response was found in the rabbit model. The T cell response after re-stimulation in cell culture showed no IFNγ producing cells when ELISPOT assays were analyzed from PBMC, but low numbers of IFNγ and IL-17 producing cells were found in ELISPOTS from spleens (both 5 immunizations). Brains from immunized rabbits showed no signs of encephalitis. Based on these results, DNA Aβ42 immunization is highly likely to be safe and effective to test in a possible clinical AD prevention trial in patients.
INTRODUCTION
Alzheimer’s disease (AD) is the sixth leading cause of death in the United States. With the projected increase in life expectance worldwide and no current treatment, the social and economic burden from this disease will potentiate in the next twenty years [1]. Immunization against Aβ1-42 peptide, a highly fibrillogenic end product, of the physiological turnover process of amyloid-β protein precursor (AβPP), which had shown success in AD mouse models with removal of amyloid plaques from brain, and benefits in learning and memory behavior tests, had failed in a stopped clinical trial, AN1792. 6% of patients developed a meningoencephalitis, which had been caused by an autoimmune and inflammatory T cell response. Despite the removal of amyloid from brain in the patients, the immunizations had not stopped the progression of dementia [2–6]. In another study, a small effect on the percentage of cognitive decline in positive antibody responders was reported [7]. One of the main conclusions from this trial was that the immunization against Aβ42 worked to remove amyloid from brain, but the treatment had been started too late, and did not reverse downstream pathologies. Biomarkers, such as Aβ deposition in brain, precede the clinical symptoms of AD by about twenty years, and therapy to treat AD must start before the symptoms are visible [8–10].
In the common late-onset form of AD, Aβ clearance from brain is significantly reduced in comparison to cognitively normal controls while rates of Aβ production are similar [11, 12], which supports the concept of immunotherapy with involvement of antibodies and cellular components such as microglia to facilitate the clearance processes. Ongoing clinical trials are concentrating on the use of passive immunization with injection of preformed anti-Aβ monoclonal antibodies to avoid a potentially inflammatory T cell response. With a positive outcome from three major prevention trials, a new focus will concentrate on active immunization again due to its higher applicability to large and diverse populations.
DNA immunization in the skin elicits a different type of immune response compared to the immunization with peptide. The full-length DNA Aβ1-42 immune response produces a non-inflammatory immune response with sufficient antibody production, and no inflammatory IFNγ and IL-17 cytokine secretion from accompanying T cell responses [13–17]. We have shown this previously in mouse models, and show here the results in a large animal model, the New Zealand White (NZW) Rabbit.
METHODS
Animals and immunizations
Sixteen four- to five-year-old NZW rabbits (ten females, six males, 3.5 to 5 kg) were purchased from Harlan (Indianapolis, IN). The rabbits were randomly separated in two groups, in which one group received a high dose immunization regimen receiving 16 μg DNA per immunization time point and the other group received a low dose regimen (8 μg DNA/immunization). The intradermal DNA immunizations with the two plasmid DNAs were performed into skin of the outer ear using the Helios gene gun (Bio-Rad, Hercules, CA). In brief, DNA coated gold particles were injected onto the skin of the shaved ears with a helium pressure of 400 psi for a total of five immunizations. The first three immunizations were done in biweekly intervals, followed by two vaccinations in monthly intervals. Two month following these five immunizations, nine of the rabbits were euthanized for pathological examination and cellular immune responses. One animal had died after the fourth immunization due to bacteremia secondary to a tooth root abscess. Immunization was continued in six of the rabbits for five additional time points (total of ten immunizations). Animal experimentation was performed in accord to the guide of the ARC at UT Southwestern Medical Center. Animal use for this study was approved by the UT Southwestern Medical Center IACUC (Institutional Animal Care and Use Committee).
The plasmid system used for DNA Aβ42 trimer immunization is illustrated in Fig. 1. A dual plasmid system, activator and responder plasmid is used for expression of the Aβ1-42 trimer (3 copies of Aβ1-42 in a row): The activator plasmid codes for the transcription factor Gal4, the responder plasmid codes for Aβ1-42 trimer. Gal4 transcription factor transcription is driven by a CMV promoter. The Gal4 protein binds as a homodimer to UAS sites present upstream of the promoter on the responder plasmid. Gal4 binding drives transcription of the Aβ1-42 trimer sequence containing also an Adenovirus E3 leader sequence and an endosomal targeting sequence derived from mouse MHC class II (H2-DM) (Fig. 1A). Expression of Aβ1-42 trimer from the plasmid was verified by analysis of mouse skin which was injected with the plasmids via gene gun. Protein lysates from mouse ear (24 h following DNA immunizations) were run on a 4–20% SDS PAGE and Aβ protein expression was detected with an anti-human Aβ42 antibody (Fig. 1B). UAS-monomer transfection (Mon, 1 copy of Aβ1-42) in mouse ear resulted in a unique band at about 10 kDa, UAS-trimer (Tri) transfection resulted in double fragments at 19 kDa, and dimerized bands of these doublets at about 40 kDa, respectively. A 19 kDa protein contains the leader sequence, three copies of Aβ1-42, and the endosomal targeting sequence (see Fig. 1A). The earlysate transfected with control DNA (Gal4/UAS-Luc) showed no protein bands detectable with the Aβ42 antibody (Luc).
Antibodies and peptides
As a standard antibody to determine the anti-Aβ IgG immune response a C-terminal Aβ specific rabbit monoclonal antibody recognizing Aβ peptides ending amino acid 42 was used (clone 1-11-3, Biolegend). Other anti-Aβ antibodies used in this study were the mouse moab 6E10 (Biolegend) and rabbit A11 (Life Technologies). As comparison to antisera generated in peptide immunized rabbits we purchased polyclonal rabbit antisera detecting Aβ1 - 15 or Aβ42 from GeneTex (Irvine, CA). A panel of secondary anti-rabbit IgG antibodies was used: anti-rabbit Ig, anti-rabbit IgG (Southern Biotech, Santa Cruz), anti-rabbit IgM (Southern Biotech), anti-rabbit IgA (Pierce), and three anti-rabbit IgG moabs (Southern Biotech, SIGMA). Aβ peptides used in this study had been purchased from rPeptide, AnaSpec, New England Peptide, Bachem, and American Peptide Company. Full-length Aβ1-42 peptides (rPeptide) were pretreated and fibrillized as described in Stine et al. [18].
Necropsy reports and histology
Nine of the rabbits underwent necropsy and histological evaluation of target tissues by a veterinarian pathologist.
Plasma and splenocyte collections
Blood was collected prior to the first, after the second, third, and fourth, and two, eight, and sixteen weeks past the fifth immunization. Antibody levels were determined from all blood samples. Blood chemistry and complete blood counts (CBC) were determined from samples prior to the first, after the fourth immunization, and from blood samples drawn eight weeks past the fifth immunization. Ten days following the final blood draw the rabbits were euthanized. Lymphocytes from blood were isolated by density separation centrifugation using Lympholyte® Cell Separation Media (Cedarlane Labs, Burlington, ON). Spleens and bone marrow were aseptically removed and processed for tissue culture. Tissue culture was performed as previouslydescribed [10].
Antibody ELISA
Aggregated Aβ1-42 and α-synuclein peptides were prepared as described previously [9]. Briefly, the peptide solution was prepared by adding 250 μl phosphate buffered saline (PBS pH 7.4) to 1 mg of lyophilized Aβ1-42 (counterions TFA, NaOH, or HCl) or α-synuclein, followed by an overnight incubation at 37°C.
Anti-Aβ antibodies in rabbit plasma are measured according to standard procedures. High binding 96-well plates were coated with human Aβ1-42 peptide (2 μg/ml) in 50 mM carbonate buffer pH 9.6 overnight at 4°C. Standard curves were included by adding serial dilutions of the rabbit anti-Aβ monoclonal antibody 1-11-3 in PBS/1.5% BSA. Plasma samples were diluted and added at concentrations ranging from 1:200 to 1:5000 to measure the antigen specific antibody concentrations. ELISAs were repeated three to four times and showed an inter-assay variability of 5–8%. Data from one ELISA werepresented.
Dot blot assays
2 μl of Aβ peptide solutions (0.5 μg/μl) were spotted in duplicates onto nitrocellulose filters and air dried. After blocking of the membranes in TBS-T containing 10% milk, further incubation with the respective antibodies (mouse- anti-human Aβ1 - 6 (6E10) and rabbit- anti-human Aβx - 42 (1-11-3) both 1:10,000, or oligomeric Aβ (A11, 1:2,000) was performed overnight at 4°C. Rabbit plasma was used at a dilution of 1:200. HRP labeled secondary antibodies (anti-mouse IgG and anti-rabbit IgG) were used in dilutions of 1:50,000. Antibody binding intensities were measured with an Opti4CN detection kit as recommended by the manufacturer(BioRad).
ELISA and ELISPOT assays
ELISA assays for antibody levels and titers in rabbit plasma were performed according to standard procedures. IgG, IgA, and IgM antibody isotypes were analyzed. Secondary isotype antibodies used had been cross adsorbed with rabbit IgG or IgM, respectively (Southern Biotech). The used polyclonal anti-rabbit IgA antibody was noted to show no cross-reactivity with other rabbit Ig isotypes (Pierce). As standard a monoclonal rabbit-anti-human Aβ42 IgG antibody (clone 1-11-3, Biolegend) was used. For testing of antibody avidity, a 90-min incubation step with the chaotropic salt NaCN in different molarities (5 M, 2 M, 1 M, 0.5 M, 0.25 M, 0.125 M, 0.006 M) was inserted in the ELISA protocol between the primary antibody binding and the detection step (NaCN ELISA). ELISPOT assays to determine frequencies of cytokine secreting cells were performed according to standard procedures (48 h), and as previously described using commercial available antibody sets for rabbit IFNγ, IL-17, and IL-4 (Kingfisher Biotech, Saint Paul, MN) [13, 15, 16]. For maximal T cell stimulation, Concanavalin A (Con A) was used at a concentration of 2.5 μg/ml.
B cell ELISPOT
MultiScreen-IP plates (Millipore, Billerica, MA) were washed with 70% ethanol, rinsed three times with phosphate-buffered saline (PBS), and then coated with 100 μl of Aβ42 (4 μg/ml) in PBS at 4°C overnight. Plates were washed six times with PBS and blocked with RPMI with 2% BSA for 2 h at room temperature. Rested splenocytes and bone marrow cells were plated in 200 μl of medium and incubated 48 h at 37°C. Plates were washed six times in PBS with 0.25% Tween 20 (Sigma) and incubated with 100 μl of 1:250 HRP conjugated goat-anti-rabbit IgG or goat-anti-rabbit IgA for 1 h at room temperature. The plates were again washed with PBS-T and a final wash in PBS, and developed with 100 μl of AEC substrate for 20 to 30 min at room temperature, and the reaction was stopped with deionized water. Spots were air dried and automatically counted with an ELISPOT reader.
Analysis of cell proliferation by CFSE dilution
Cells for labeling were re-suspended in PBS and immediately mixed with an equal volume of CFSE diluted in PBS (1:1). The cells were incubated for 5 min at room temperature with repeated mixing to obtain an even CFSE labeling of all cells. The labeling was stopped by adding of an equal volume of complete RPMI medium and removal of the solution from the cells by centrifugation. The cells were plated in round-bottom 96-well cell culture plates at concentrations of 1×106 cells per well and re-stimulated with Aβ42 peptide or ConA for six days. After harvesting cells were re-suspended in FACS buffer (PBS/1% BSA/ 0.1% NaN3) and stained with a mouse-anti-rabbit CD4 antibody (clone clone RTH1A, Kingfisher) followed by an APC conjugated rat anti-mouse IgG1 antibody (eBioscience). Fluorescence of the cells was measured using an Accuri C6 Flow Cytometer (Ann Arbor, MI) and analyzed with CFlow Plus and FCS Express Version 3 software programs.
AD mouse model brain immunohistochemistry
For plaque staining formalin fixed brain sections were treated with 70% formic acid for antigen retrieval. Non-specific staining was blocked by a 1-h incubation in 2% BSA/2% goat normal goat serum/0.3% Triton X100 in PBS at room temperature. Tissues were then incubated with primary antibodies (rabbit plasma, dilution 1:250) or control antibody (McSA1(Medimabs, Canada), 1:500) overnight at 4°C. Secondary antibody binding (goat-anti-rabbit IgG HRP, goat-anti-mouse IgG HRP) was detected with a 3,3’-diaminobenzidine (DAB) substrate kit. Sections were scanned using a NanoZoomer digital pathology system and analyzed with NDP viewer software (Hamamatzu, Japan).
Statistics
For statistics (unpaired t test with two tailed p values, Mann-Whitney t test, column statistics), we used GraphPad Prism version 6 for Windows (San Diego, CA, http://www.graphpad.com). p-values of≤0.05 were considered significant.
RESULTS
Blood work and general histology
Blood from the sixteen rabbits used in this study was tested before the vaccination and following the 4th vaccination for signs of inflammation (CBC), or signs of metabolic changes (blood chemistry). No changes were observed (data not shown). All nine rabbits undergoing necropsy were in good body condition with adequate body fat stores and no appreciable postmortem autolysis. No gross abnormalities were found. Two female rabbits had small cysts in their kidneys; these cysts in the renal cortex are not uncommon in aged rabbits. Two other female rabbits had developed uterine leiomyoma, which again is likely due to the age of these rabbits, and the fact that they were retired breeders. Three different HE stained brain regions were examined and showed no sign of lymphocyte infiltration or inflammation (Fig. 2). One female rabbit of the six remaining animals that received continued immunizations (10x) had a mammary adenocarcinoma, which was surgically removed. This animal was euthanized later due to the development of a tooth root abscess of the premolars. The pathology report for this rabbit showed metastasis of the adenocarcinoma to the uterus. The brain showed no sign of inflammation. Two male rabbits (both seven years old) died after this study was completed. One of them died 3 months after the last immunization. Necropsy and histological examinations showed no cause of death (undetermined). The brain of this rabbit showed no signs of inflammation. The second male rabbit was euthanized 4 months after the 10th immunization due to a soft tissue tumor (basal cell carcinoma) on one of his hind legs.
Four out of 12 analyzed rabbit brains showed positive staining with Prussian Blue (one to three cortical lesions, data not shown).
Antibody immune response in DNA Aβ42 immunized rabbits
Antibody levels and total antibody amounts were measured for anti-Aβ42 antibodies of the IgG, IgA, and IgM isotypes. In blood samples taken prior to the first immunizations, baseline levels of anti-Aβ42 antibody levels were found (< 20 μg/ml plasma). An analysis of the antibody titers found in the rabbits after three immunizations demonstrated the development of an anti-Aβ42 IgG antibody response. In Fig. 3, the representative titers for two rabbits which had received high dose DNA Aβ42 immunizations, and two rabbits which had received the low dose immunization are shown (Fig. 3A). Half maximal binding was determined for rabbit 828D as 1:25,000, 1:3,200 for rabbit 831D, 1:12,800 for rabbit 833D, and 1:1,600 and 1:5,000 for rabbit 858D. This second increase of antibody binding to Aβ1-42 at higher dilutions of the plasma samples is indicative of a second antibody epitope, which becomes more accessible at higher antibody dilutions due to antibody hinderance and steric competition at lower plasma dilutions. Similar findings were made in all of the sixteen rabbits. Further analyses to the epitope binding of the anti-Aβ42 antibodies produced in these four rabbits showed a strong binding to full-length Aβ1-42 peptide, and much lesser binding to a panel of shorter Aβ peptides (linear epitopes) spanning this peptide at this early time point (Fig. 3B). Shown are the binding pattern for the antibodies from the same rabbits for which the antibody titer analysis is depicted, but similar results were obtained for all sixteen animals. The binding to the linear epitopes at this early time point (3 immunizations) is low in rabbits 828D and 831D, much higher in rabbit 833D, and antibodies produced in rabbit 858D bound stronger to a mid-region Aβ peptide, Aβ10 - 26 and Aβ17 - 31, than to the typical B cell epitope of Aβ42, which is Aβ1 - 16 (Fig. 3B).
Antibody levels increased to mean antibody levels of 134.4±47.14 μg/ml plasma after 3 immunizations, and to 277.1±118.4 μg/ml plasma after 5 immunizations. No significant differences were found between the low dose (8 μg DNA/immunization) and high dose group (16 μg DNA/immunization) (Fig. 4A). Antibody levels declined slightly to 246.6±109.5 μg/ml (n = 15, p = 0.461) after a resting period of two month following the 5th immunization. The analyses of antibody producing plasma cells in spleen from the immunized rabbits confirmed that a good humoral immune response had been elicited in the rabbit model with the DNA vaccine. Anti-Aβ1-42 IgG antibody producing plasma cells were found in these cultures with mean values of 103.7 anti-Aβ42 IgG antibody forming units in splenocytes from nine rabbit analyzed (p > 0.0001 compared to controls with 14.4 antibody-forming cells AFC/106 cells (Fig. 4B). Splenocyte cell culture supernatant (48 h) contained an average of 0.2 μg anti-Aβ42 IgG antibody/ml medium confirming the presence of Aβ42 specific plasma cells in spleens of DNA Aβ42 immunized rabbits(Fig. 4C).
Antibody epitopes were determined for all sixteen rabbits following the 4th immunization. Anti-Aβ antibodies of the IgG (Fig. 4D), IgA (Fig. 4E), and IgM (Fig. 4F) isotypes bound to a wide panel of small Aβ peptides including N-truncated (Aβ3 - 42, Aβ4 - 17, Aβ5 - 17, Aβ6 - 17) and pyroglutamate modified Aβ peptides (3Pyr-Aβ3 - 42 and 11Pyr-Aβ11 - 42).
In the six rabbits, which received continued immunizations in monthly intervals, antibody levels did not increase further (Fig. 5). These six rabbits had anti-Aβ42 IgG antibody levels of 247.9±57.63 μg/ml plasma after five DNA immunizations, and an antibody level of 246.4±50.29 μg/ml after ten DNA Aβ42 immunizations. Following the stop of further vaccinations, the antibody levels declined to 162.7±32.5 μg/ml after a two-month period, and to 105±37.58 μg/ml after a four-month period (Fig. 5A). From these data, the antibody half-life was calculated as three months (Fig. 5B).
Linear anti-Aβ B-cell epitopes are distributed over the entire amino acid sequence Aβ1 - 40
For characterization of the linear anti-Aβ specific B-cell epitopes, we used a panel of shorter Aβ peptides spanning the Aβ1 - 40 sequence, Aβ1 - 16, Aβ6 - 20, Aβ10 - 26, Aβ17 - 31, Aβ22 - 35, and Aβ29 - 40, in a direct ELISA assay. Antibody epitopes were determined from antibodies of 3x, 5x, and 10x immunized rabbits, as well as from the antibodies following the two or four-month interval (plasma dilutions 1:400 (3x), Fig. 3B; plasma dilutions 1:1000 (5x, 10x), Fig. 5C-F). These data show a wide epitope binding, detecting epitopes throughout the smaller 11–16 amino acid long peptides spanning the entire Aβ1 - 40 sequence.
Antibody isotypes
The rabbit humoral immune response consists of four antibody isotypes: IgM, IgG, IgE, and IgA. While humans and mice have four or five IgG subclasses respectively, there is only one IgG subclass in the rabbit. We analyzed the binding of antibody isotypes, IgM, IgG, and IgA to full-length Aβ1-42, N-truncated (Aβ4 - 42, Aβ5 - 42) and Pyroglutamated Aβ peptides (3PyrAβ3 - 42 and 11PyrAβ11 - 42) in DNA Aβ42 immunized rabbits (Fig. 6A). Commercially available rabbit antisera against Aβ1 - 15 and Aβ1-42 showed similar binding of antibody isotypes indicative that the rabbit produces all three isotypes in an immune response (data not shown). The binding to Aβ4 - 42 or Aβ5 - 42 and 3PyrAβ3 - 42 contained mainly IgG and IgM antibodies and significantly less IgA antibodies (p values of 0.0317, 0.022, 0.022, Mann-Whitney). In Fig. 6B, the results for IgG antibody binding to Aβ1-42, Aβ4 - 42, Aβ5 - 42, and 3PyrAβ3 - 42 from six rabbits are shown. When the rabbit sera were tested against a different brain antigen, α-synuclein, they displayed the same binding pattern: strong binding of IgG and IgM antibody isotypes and significant less binding of antibodies with the IgA isotype. From the two commercially available antisera (anti-human Aβ1–15 and anti-human Aβ1-42), only the serum with Aβ1-42 specificity contained some IgG antibodies binding to Aβ4 - 42, Aβ5 - 42, 3PyrAβ3 - 42, and α-synuclein (Fig. 6B).
Since there is only one IgG antibody isotype in the rabbit and a lack of information about additional biological features of the antibodies (differences in complement binding or Fc receptor binding), it is not possible to classify the immune response based on isotyping data in this species.
Dot blot assay and avidity ELISA
For further specification of the antibody binding to Aβ, a dot blot assay was performed. Peptides were spotted on nitrocellulose membranes and probed with the commercially available antibodies 6E10, 1-11-3, and A11, or the different sera from rabbits immunized in this study. As shown in Fig. 7A, the 6E10 antibody (Aβ1 - 16) bound well to Aβ1-42 and Aβ1 - 40, while 1-11-3 bound to Aβ1-42, and not to Aβ1 - 40 consistent with the epitope specificity of this antibody in the C-terminal end of the Aβ1-42 peptide. A11 bound weakly to Aβ1-42/HFIP and Aβ1 - 40 indicative of some degree of oligomeric Aβ conformers in these preparations. Antibodies in the six rabbit plasma samples bound well to Aβ1-42 and Aβ1 - 40. Also, all six rabbit plasma samples bound to α-synuclein with the highest intensity found for rabbit 858D, and did not bind to amylin, another amyloid peptide.
In an ELISA protocol using different molarities of the chaotropic salt NaCN to test for antibody binding avidities, the antigen binding specificities seen in the dot blot were confirmed (plasma dilutions 1:500, Fig. 7B). All rabbit plasma samples bound with high avidity to Aβ1-42 and the deletion mutant of Aβ1-42 lacking residue 22 (Δ22 Aβ1-42, not shown in the dot blot). Avidity of binding for the rabbit antibodies was compared to the control antibodies 6E10 and 1-11-3. High avidity to Δ22 Aβ1-42 was found for moab 11-3-1, the rabbit plasma antibodies bound to Δ22 Aβ1-42 with similar strength as moab 6E10. Highest Aβ1 - 40 binding was detected for the moab 6E10 with a substantial decrease of binding at NaCN concentration of 0.5 M. No binding was found for moab 1-11-3 consistent with the specificity of the antibody for Aβ peptides ending in residue Aβ42. The six rabbit plasma samples showed an intermediate avidity to Aβ1 - 40 with loss of binding at NaCN concentrations at 0.5 M, except plasma antibodies from 828D which showed loss of binding at 1 M NaCN. No binding to α-synuclein was found for moabs 6E10 and 1-11-3. The six rabbit plasma samples tested in this assay showed similar avidities in binding to α-synuclein with loss of binding at salt concentrations of 0.5 M NaCN. Plasma antibodies of 858D, which showed a strong signal in the dot blot assay (Fig. 6A), also showed the strongest binding to α-synuclein in the NaCN ELISA; binding was clearly detectable at concentrations up to 1 M NaCN (Fig. 7B).
Cross-reactivity of rabbit plasma samples with α-synuclein
The α-synuclein binding was further evaluated in a series of ELISA assays. Plasma samples from 15 DNA Aβ42 immunized rabbits were tested after the 5th immunization time point for binding to Aβ1-42, full-length α-synuclein (1–140), a deletion mutant α-synuclein/Δ61–95, α-synuclein 1–60, amylin, which is a 37 aa peptide, IAPP (Islet Amyloid Peptide), which is a 5 aa peptide, and SAP (Serum Amyloid P component), which is a 6 aa peptide, and a mutant Aβ1-42 peptide which lacks residue 22 (Δ22Aβ1-42), which has been described as highly oligomeric [19] (plasma dilution 1:500, Fig. 8A), High binding was found for Aβ1-42, Δ22Aβ1-42, and all three α-synucleins, low binding was found for amylin, SAP, and IAAP (p values (Mann-Whitney test) for the comparison of binding to Aβ1-42 and the latter three peptides was p < 0.0001 and 0.0007, respectively). From these 15 samples the data of the six rabbits, which received continued immunizations (ten times) were extracted and shown in Fig. 8B to allow a better comparison with the data showing the cross-reactivity following the 10th immunization (Fig. 8C).
Analyses of T cell responses in the immunized rabbits
IFNγ secretion was determined by an ELISPOT assay for all of the rabbits (n = 15) one week following the 5th immunization from peripheral blood lymphocytes. No IFNγ secreting cells were detected after Aβ42 peptide re-stimulation while high numbers of IFNγ spots were found in wells which had been stimulated with ConA (data not shown). Further T cell responses were determined from splenocytes in eight of the rabbits four months following the fifth DNA Aβ1-42 immunization by ELISPOT assays for IFNγ, IL-17, and IL-4 (Fig. 9) and a CFSE proliferation assay (Figs. 10 and 11).
The analyzed rabbits showed high variability in the cellular assays between the individual animals, which was expected since the cohort consisted of aged non-inbred breeders. The ELISPOT assays showed low numbers of IFNγ secreting cells but with significant differences in response to Aβ42 peptide re-stimulation in three of the eight rabbits (831D, 835D, and 855D). The IL-17 ELISPOT showed increased numbers of IL-17 secreting cells in all eight rabbits analyzed, and again for three of the rabbits significantly increased numbers of IL-17 secreting cells were present upon peptide re-stimulation (830D, 833D, and 836D). IFNγ and IL-17 producing cells were not overlapping as they were found in different animals. No significant differences between medium controls and Aβ42 peptide re-stimulated splenocyte cultures were found in regard to IL-4 secreting cells.
In the CFSE proliferation assay, high background levels with unspecific proliferation in medium control wells was found for rabbits 830D, 835D, and 855D. Two rabbits, 833D and 835D, showed significantly increased levels of CD4 T cell proliferation in response to the Aβ42 peptide re-stimulation in vitro, which increased from 17% in medium to 25% in Aβ42 peptide containing medium, or 8% to 22% in the second rabbit, respectively. The remaining three rabbits, 829D, 831D, and 836D showed no significant increase in T cell proliferation following Aβ42 peptide re-stimulation (Fig. 10). Representative histograms for the CFSE proliferation are shown in Fig. 11A-G. Figure 11A shows the gating (gate R1) on proliferating of CD4+ T cells following Aβ42 peptide re-stimulation of splenocytes in culture of rabbit 833D, which possessed 28.7% of proliferating CD4 T cells (Figs. 10, 11A, and 11B). Further analyses of these cells with the FCS Express Version 3 software program (shown in Fig. 11C, D), indicated that the clouds of cells seen in Fig. 10A are likely only noise, which do not form separated peaks of dividing cells, which is shown for the CD4 T cell populations following ConA stimulation in Fig. 11G. Only one clear peak for the undivided cells is seen (grey peak in Fig. 10C, D). In Fig. 11E-G, the same analyses were performed for a different rabbit, 831D, which showed no CD4 proliferation in Fig. 10. Also for this rabbit only one peak for the undivided cells can be found (Fig. 11E medium control, Fig. 11F Aβ42 peptide re-stimulation), but the histogram from the ConA stimulated splenocytes of this rabbit formed five clearly separated peaks of dividing cells (black) and one small peak of undivided cells (grey, Fig. 11G). Similar findings of background noise and no clearly dividing cell populations were made in the detailed proliferation analysis of the other six rabbits (data not shown). All rabbits showed high levels of CD4 T cell proliferation in response to ConA stimulation (positive control).
Anti-Aβ42 antibodies from immunized rabbits stained senile plaques in brain sections of 3xTg-AD mice
The staining of brain sections from a 3xTg-AD mouse (B6;129-Psen1tm1Mpm Tg(APPSwe,tauP301 L)1Lfa/Mmjax) with plasma from the rabbits, which had received DNA Aβ42 immunizations, showed clear staining in the typical areas in which amyloid plaques are present in this AD mouse model, frontal cortex and subiculum of the hippocampus (hippocampus shown in Fig. 12A). In the comparison to a commercial anti-Aβ42 antibody (McSA1, shown in Fig. 12B), we found a substantial overlap of detected amyloid plaques in the areas stained with both antibodies (indicated with blue and green asterisks). In addition to the overlapping areas, unique staining pattern can be identified for both of the antibodies as well. Brain sections from this 3xTg-AD mouse showed no staining with control plasma from a non-immunized rabbit (Fig. 12C). No staining was found in plaque free areas as shown for the cerebellum (Fig. 12D-F).
DISCUSSION
DNA Aβ42 trimer immunization with a two-plasmid system led to good antibody responses in the rabbit model. This is an important finding, because DNA immunization has been shown to be successful in rodents, but positive results in larger animals were missing, and had been a major drawback for developing these approaches further for clinical use. Furthermore, rabbits used for the DNA immunization were aged animals (≥5 years old), which is important in regard to analysis of the immune responses in large mammals showing age-associated immune deficiency and immunosenescence. Results described here, showing positive antibody responses but no T cell activation, were very similar to the findings we published for various mouse experiments (wild-type and AD mouse models) [13–17, 20, 21]. Brains from the immunized rabbits did not show any signs of inflammation. Thus, DNA Aβ42 immunization appears to be effective and safe in larger mammals, and the immune response is similar to the mouse models we analyzed previously.
The antibody levels produced in the sixteen rabbits reached mean values of 280 μg/ml plasma after five immunizations. Much lower antibody concentrations, 3.1–19.4 μg/ml plasma were found in a study in which rabbits received four immunizations with a DNA Aβ epitope vaccine containing three copies of Aβ1–11 [22]. This group developed an optimized vaccine with inclusion of more T helper epitopes into the DNA construct (3Aβ1–11-Thep), and in a second group of immunized rabbits receiving the optimized vaccine, mean antibody levels reached 40–80 μg/ml plasma. While the rabbits in our study produced IgG, IgM, and IgA antibodies, the rabbits in the epitope vaccine study produced only IgG antibodies, which is likely due to the lack of T cell help during the generation of an antigen specific immune response.
We have shown in different mouse models that DNA Aβ42 trimer immunization does not lead to continued T cell responses, and in particular does not lead to an inflammatory cellular immune response with IFNγ or IL-17 secreting cells [13–17]. T cell stimulation in the immunized rabbits in this study was analyzed on two levels: T cell proliferation upon antigen stimulation in culture and cytokine secretion. The results from these assays are divergent, which is likely due to the fact that these rabbits were all aged outbreed breeders (four to five years old) with underlying small and diverse medical problems. Three rabbits showed no specific CD4 proliferation upon Aβ42 peptide re-stimulation, which is consistent with our findings in the mouse. Three rabbits had similar levels of T cell proliferation in the medium controls and Aβ42 peptide containing wells, and two rabbits showed a significant increase in CD4 T cell proliferation upon Aβ42 peptide re-stimulation. A detailed analysis of the proliferating cells using the FCS express proliferation software package confirmed for all seven rabbits, which had CD4 T cell proliferation (8 to 16%, Fig. 9C) already in the medium control wells, that this proliferation appears indeed like to be unspecific proliferation (background noise), with no specific increase in wells to which Aβ42 peptide was added (Fig. 9A). Also, compared to levels of CD4 proliferation in Aβ42 peptide immunized mice, in which we find 50% CD4 proliferation [15], the proliferation levels (22%) are rather low. These low levels of proliferation can very well be due to Th2 or Treg proliferation, which we had also shown before in the mouse model [16]. Significantly increased numbers of IFNγ and IL-17 secreting cells were found in three of the eight rabbits, but for different animals (except one, 830D), and again non-overlapping with the rabbits which showed increased CD4 proliferation. Interestingly, rabbit 830D, which showed IFNγ and IL-17 secreting cells upon Aβ42 peptide re-stimulation was one of the rabbits, which had already high levels of CD4 T cell proliferation in the medium controls, and can thus be considered as nonspecific proliferation. Therefore, we conclude that IFNγ and IL-17 secretion in these animals was due to the age of the rabbits and possibly underlying medical complications, and was not due to the DNA Aβ42 immunization and the immune response thereof.
The humoral anti-Aβ42 immune response in the rabbit is a well-established research area. Already in the early publications, it was found that the antibodies produced after immunization with fibrillar Aβ42 peptide bound approximately 1000-fold more tightly to fibrillar Aβ1-42 than to the linear shorter Aβ peptides [23]. Following immunization of rabbits with a morphologically homogeneous population of Aβ42 fibrils, it was found that rabbits produced conformation dependent antibodies recognizing distinct assembly states of amyloids, such as prefibrillar oligomers and fibrils. One of the well characterized anti-oligomer antibodies, clone A11, was derived from one of these studies [24]. Large panels of monoclonal antibodies were described later and confirmed the variability of anti-Aβ antibodies generated in the rabbit detecting linear epitopes as well as several conformational epitopes [25, 26].
Antibodies in plasma from DNA Aβ42 immunized rabbits stained plaques in brain of 3xTg-AD mice. A common problem with polyclonal antisera is that they contain in addition to the specific antibodies large amounts of unspecific antibodies, which can result in high background noise. To reduce the background staining, we used a rather high dilution of the rabbit samples in exchange of losing probably some of the positive staining due to the low concentrations of specific antibody. Our analyses in staining of Aβ in brain of an AD mouse model, 3xTg-AD, with the polyclonal rabbit anti-Aβ42 antisera derived from the DNA Aβ42 immunizations, showed specific staining in areas in which amyloid plaques can be typically found in this mouse model (Fig. 12). The staining was not as strong as the control anti-Aβ42 moab McSA1, but this is also not surprising considering the reasons why polyclonal antibody staining can be problematic (see above in this paragraph). The staining was highly specific as no stain was found in plaque freeareas.
As shown in the dot blot analyses, Avidity ELISA (Fig. 8), and testing of cross-reactivity (Fig. 9), the rabbit plasma samples bound to fibrillized Aβ1-42 peptides, Aβ1 - 40 peptide, and bound strongly also to aggregated α-synuclein, a peptide, which has no sequence homology to Aβ1-42 peptide, and the two shorter α-synuclein peptides, Δ61–95 α-synuclein and 1–60 α-synuclein. The antibody response to α-synuclein is a new and potentially important finding as α-synuclein also accumulates in the brain of AD patients with some regularity, and thus the immune response may have additional potential to reduce α-synuclein levels as well [27]. It also might indicate that the antibodies generated after DNA Aβ42 trimer immunization contain at least in part antibodies which detect conformational epitopes or Aβ oligomers [28].
Conclusions
Positive results for antibody production after DNA gene gun immunization in large mammals has been missing, and the data presented here show that DNA Aβ42 immunization induced a good humoral immune response in aged NZW rabbits, which possess the same Aβ1-42 sequence as humans. The rabbit skin at the injection site showed no signs of inflammation or irritation in any of the vaccinated animals, and because the rabbit skin is an optimal target to test chemical agents for irritation, it is highly likely that human skin will show the same results following DNA gene gun vaccinations. Brains from the analyzed rabbits showed no sign of inflammation. The humoral immune response was polyclonal and multivalent; detecting N-terminal truncated Aβ peptides as well as several mid-region Aβ peptides very similar to our findings in the mouse [29]. Full-length DNA Aβ42 vaccination into the skin is likely to induce a good humoral immune response in humans as well.
ACKNOWLEDGMENTS
The authors would like to thank Angela Guillory and Brian Gutowsky for their expert animal care.
This study was supported by NIA/NIH P30AG12300-21, Zale Foundation, Rudman Foundation, McCune Foundation, AWARE, Presbyterian Village North, Freiberger, Losinger, and Denker Family Funds. We would also like to thank the UTSW Whole Brain Microscopy Facility (WBMF) in the Department of Neurology and Neurotherapeutics for assistance with slide scanning. WBMF is supported by the Texas Institute for Brain Injury and Repair (TIBIR).
Authors’ disclosures available online (http://j-alz.com/manuscript-disclosures/16-0947r1.
REFERENCES
[1] | Holtzman DM , Goate A , Kelly J , Sperling R ((2011) ) Mapping the road forward in Alzheimer’s disease. Sci Transl Med 3: , 114ps48. |
[2] | Schenk D , Barbour R , Dunn W , Gordon G , Grajeda H , Guido T , Hu K , Huang J , Johnson-Wood K , Khan K , Kholodenko D , Lee M , Liao Z , Lieberburg I , Motter R , Mutter L , Soriano F , Shopp G , Vasquez N , Vandevert C , Walker S , Wogulis M , Yednock T , Games D , Seubert P ((1999) ) Immunization with amyloid-beta attenuates Alzheimer-disease-like pathology in the PDAPP mouse. Nature 400: , 173–177. |
[3] | Morgan D , Diamond DM , Gottschall PE , Ugen KE , Dickey C , Hardy J , Duff K , Jantzen P , DiCarlo G , Wilcock D , Connor K , Hatcher J , Hope C , Gordon M , Arendash GW ((2000) ) A beta peptide vaccination prevents memory loss in an animal model of Alzheimer’s disease. Nature 408: , 982–985 |
[4] | Schenk D ((2002) ) Amyloid-beta immunotherapy for Alzheimer’s disease: The end of the beginning. Nat Rev Neurosci 3: , 824–828. |
[5] | Nicoll JA , Wilkinson D , Holmes C , Steart P , Markham H , Weller RO ((2003) ) Neuropathology of human Alzheimer disease after immunization with amyloid-beta peptide: A case report. Nat Med 9: , 448–452. |
[6] | Holmes C , Boche D , Wilkinson D , Yadegarfar G , Hopkins V , Bayer A , Jones RW , Bullock R , Love S , Neal JW , Zotova E , Nicoll JA ((2008) ) Long-term effects of Abeta42 immunization in Alzheimer’s disease: Follow-up of a randomized, placebo-controlled phase I trial. Lancet 372: , 216–223. |
[7] | Vellas B , Black R , Thal LJ , Fox NC , Daniels M , McLennan G , Tompkins C , Leibman C , Pomfret M , Grundman M ((2009) ) AN1792 (QS-21)-251 Study Team. Long-term follow-up of patients immunized with AN1792: Reduced functional decline in antibody responders. Curr Alzheimer Res 6: , 144–151. |
[8] | Rosenberg RN ((2011) ) Treat Alzheimer disease before it is symptomatic. Arch Neurol 68: , 1237–1238. |
[9] | Bateman RJ , Xiong C , Benzinger TL , Fagan AM , Goate A , Fox NC , Marcus DS , Cairns NJ , Xie X , Blazey TM , Holtzman DM , Santacruz A , Buckles V , Oliver A , Moulder K , Aisen PS , Ghetti B , Klunk WE , McDade E , Martins RN , Masters CL , Mayeux R , Ringman JM , Rossor MN , Schofield PR , Sperling RA , Salloway S , Morris JC ((2012) ) Dominantly Inherited Alzheimer Network. Clinical and biomarker changes in dominantly inherited Alzheimer’s disease. N Engl J Med 367: , 795–804. |
[10] | Miller G ((2012) ) News focus: Stopping Alzheimer’s before it starts. Science 337: , 790–792. |
[11] | Bateman RJ , Munsell LY , Morris JC , Swarm R , Yarasheski KE , Holtzman DM ((2006) ) Human amyloid-beta synthesis and clearance rates as measured in cerebrospinal fluid in vivo. Nat Med 12: , 856–861. |
[12] | Mawuenyega KG , Sigurdson W , Ovod V , Munsell L , Kasten T , Morris JC , Yarasheski KE , Bateman RJ ((2010) ) Decreased clearance of CNS beta-amyloid in Alzheimer’s disease. Science 330: , 1774. |
[13] | Lambracht-Washington D , Qu BX , Fu M , Eagar TN , Stüve O , Rosenberg RN ((2009) ) DNA beta-amyloid (1-42) trimer immunization for Alzheimer disease in a wild-type mouse model. JAMA 302: , 1796–1802. |
[14] | Qu BX , Lambracht-Washington D , Fu M , Eagar TN , Stüve O , Rosenberg RN ((2010) ) Analysis of three plasmid systems for use in DNA Aβ42 immunization as therapy for Alzheimer’s disease. Vaccine 28: , 5280–5287. |
[15] | Lambracht-Washington D , Qu BX , Fu M , Anderson LD Jr , Stüve O , Eagar TN , Rosenberg RN ((2011) ) DNA immunization against amyloid beta 42 has high potential as safe therapy for Alzheimer’s disease as it diminishes antigen-specific Th1 and Th17 cell proliferation. Cell Mol Neurobiol 31: , 867–874. |
[16] | Lambracht-Washington D , Rosenberg RN ((2015) ) Co-stimulation with TNF receptor superfamily 4/25 antibodies enhances in-vivo expansion of CD4+CD25+Foxp3+T cells (Tregs) in a mouse study for active DNA Aβ42 immunotherapy. J Neuroimmunol 278: , 90–99. |
[17] | Lambracht-Washington D , Rosenberg RN ((2015) ) A noninflammatory immune response in aged DNA Aβ42-immunized mice supports its safety for possible use as immunotherapy in AD patients. Neurobiol Aging 36: , 1274–1281. |
[18] | Stine WB , Dahlgren KN , Krafft GA , LaDu MJ ((2003) ) In vitro characterization of conditions for amyloid-beta peptide oligomerization and fibrillogenesis. J Biol Chem 278: , 11612–11622. |
[19] | Tomiyama T , Nagata T , Shimada H , Teraoka R , Fukushima A , Kanemitsu H , Takuma H , Kuwano R , Imagawa M , Ataka S , Wada Y , Yoshioka E , Nishizaki T , Watanabe Y , Mori H ((2008) ) A new amyloid beta variant favoring oligomerization in Alzheimer’s-type dementia. Ann Neurol 63: , 377–387. |
[20] | Qu B , Boyer PJ , Johnston SA , Hynan LS , Rosenberg RN ((2006) ) Aβ42 gene vaccination reduces brain amyloid plaque burden in transgenic mice. J Neurol Sci 244: , 151–158. |
[21] | Qu B-X , Xiang Q , Li L , Johnston SA , Hynan LS , Rosenberg RN ((2007) ) Aβ42 gene vaccine prevents Aβ42 deposition in brain of double transgenic mice. J Neurol Sci 260: , 204–213. |
[22] | Ghochikyan A , Davtyan H , Petrushina I , Hovakimyan A , Movsesyan N , Davtyan A , Kiyatkin A , Cribbs DH , Agadjanyan MG ((2003) ) Refinement of a DNA based Alzheimer’s disease epitope vaccine in rabbits. Hum Vaccin Immunother 9: , 1002–1010. |
[23] | Miller DL , Currie JR , Mehta PD , Potempska A , Hwang YW , Wegiel J ((2003) ) Humoral immune response to fibrillar beta-amyloid peptide. Biochemistry 42: , 11682–11692. |
[24] | Kayed R , Head E , Sarsoza F , Saing T , Cotman CW , Necula M , Margol L , Wu J , Breydo L , Thompson JL , Rasool S , Gurlo T , Butler P , Glabe CG ((2007) ) Fibril specific, conformation dependent antibodies recognize a generic epitope common to amyloid fibrils and fibrillar oligomers that is absent in prefibrillar oligomers. Mol Neurodegener 2: , 18. |
[25] | Hatami A , Albay R 3rd , Monjazeb S , Milton S , Glabe C ((2014) ) Monoclonal antibodies against Aβ42 fibrils distinguish multiple aggregation state polymorphisms in vitro and in Alzheimer disease brain. J Biol Chem 289: , 32131–32143. |
[26] | Kayed R , Canto I , Breydo L , Rasool S , Lukacsovich T , Wu J , Albay R 3rd , Pensalfini A , Yeung S , Head E , Marsh JL , Glabe C ((2010) ) Conformation dependent monoclonal antibodies distinguish different replicating strains or conformers of prefibrillar Aβ oligomers. Mol Neurodegener 5: , 57. |
[27] | Larson ME , Sherman MA , Greimel S , Kuskowski M , Schneider JA , Bennett DA , Lesné SE ((2012) ) Soluble α-synuclein is a novel modulator of Alzheimer’s disease pathophysiology. J Neurosci 32: , 10253–10266. |
[28] | Kayed R , Glabe CG ((2006) ) Conformation-dependent anti-amyloid oligomer antibodies. Methods Enzymol 413: , 326–344. |
[29] | Lambracht-Washington D , Rosenberg RN ((2015) ) DNA Aβ42 immunization generates a multivalent vaccine: Antibodies in plasma of active full-length DNA Aβ42 immunized mice show polyclonal Aβ42 peptide binding. Alzheimers Dement 11: (Suppl), P842. |
Figures and Tables
Fig.1
Schematic representation of the plasmid system (Gal4 activator, UAS/DNA Aβ42 trimer responder) and analysis of Aβ42 expression in mouse ear after gene gun transfection with Aβ42 monomer and Aβ42 trimer. A) Gal4 protein encoded by the activator plasmid binds as a homodimer to UAS sites present upstream of the promoter on the responder plasmid. Gal4 binding drives transcription of the Aβ42 trimer sequence, a peptide leader sequence, and an endosomal targeting sequence. B) Protein lysates from mouse ear were separated on 4–20% SDS PAGE and probed with an anti-human Aβ42 antibody. UAS-monomer transfection (Mon) in mouse ear resulted in a unique band (1), UAS-timer (Tri) transfection resulted in doublets at 19 kDa (2 + 3), and further dimerized bands 40 kDa (4 + 5), respectively. Control DNA (Gal4/UAS-Luc) mouse ear lysates showed no protein bands detectable with the Aβ42 antibody (Luc). (Modified and with permission from Vaccine, Qu et al., 2010 [14]).
![Schematic representation of the plasmid system (Gal4 activator, UAS/DNA Aβ42 trimer responder) and analysis of Aβ42 expression in mouse ear after gene gun transfection with Aβ42 monomer and Aβ42 trimer. A) Gal4 protein encoded by the activator plasmid binds as a homodimer to UAS sites present upstream of the promoter on the responder plasmid. Gal4 binding drives transcription of the Aβ42 trimer sequence, a peptide leader sequence, and an endosomal targeting sequence. B) Protein lysates from mouse ear were separated on 4–20% SDS PAGE and probed with an anti-human Aβ42 antibody. UAS-monomer transfection (Mon) in mouse ear resulted in a unique band (1), UAS-timer (Tri) transfection resulted in doublets at 19 kDa (2 + 3), and further dimerized bands 40 kDa (4 + 5), respectively. Control DNA (Gal4/UAS-Luc) mouse ear lysates showed no protein bands detectable with the Aβ42 antibody (Luc). (Modified and with permission from Vaccine, Qu et al., 2010 [14]).](https://content.iospress.com:443/media/jad/2017/57-1/jad-57-1-jad160947/jad-57-jad160947-g001.jpg)
Fig.2
Hematoxylin and eosin stain (H&E) of rabbit brain tissue. Shown is the characteristic histology for cortex and hippocampus found in one low dose rabbit (830D) and one high dose rabbit (835D). Scale bar indicates 500 μm.
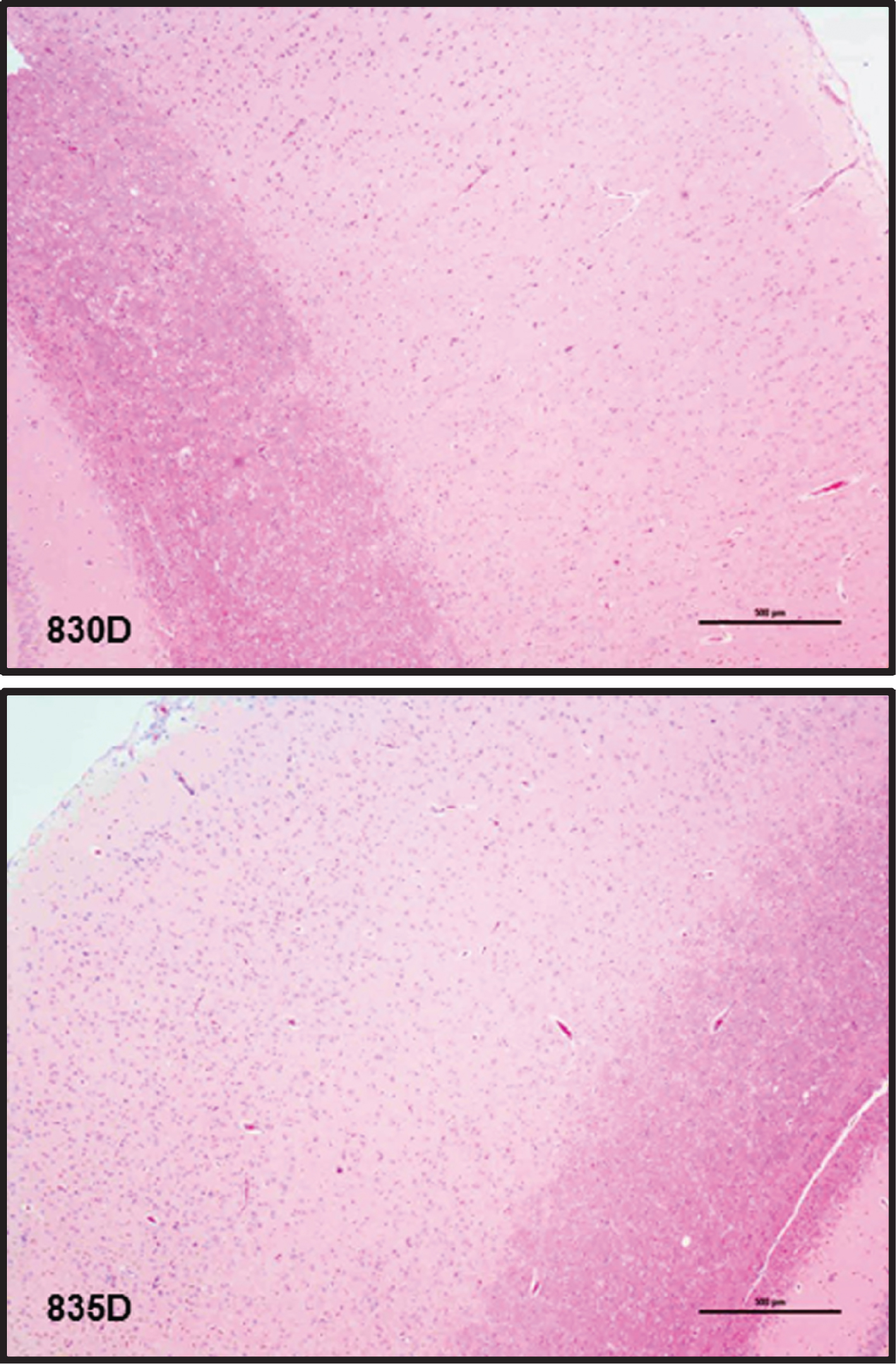
Fig.3
Anti-Aβ42 antibody titers in NZW rabbits after three DNA Aβ42 immunizations. A) Serial dilutions of rabbit plasma were analyzed for binding to Aβ1-42 peptide in a standard ELISA assay. Half maximal binding is indicated with an asterisk. Titers from two rabbits, which received the low dose immunizations, and two rabbits, which received the high dose immunizations are shown. B) Epitope binding of these plasma samples, two low dose and two high dose rabbits, is shown against a panel of short Aβ peptides. Plasma dilutions in this assay were 1:400.
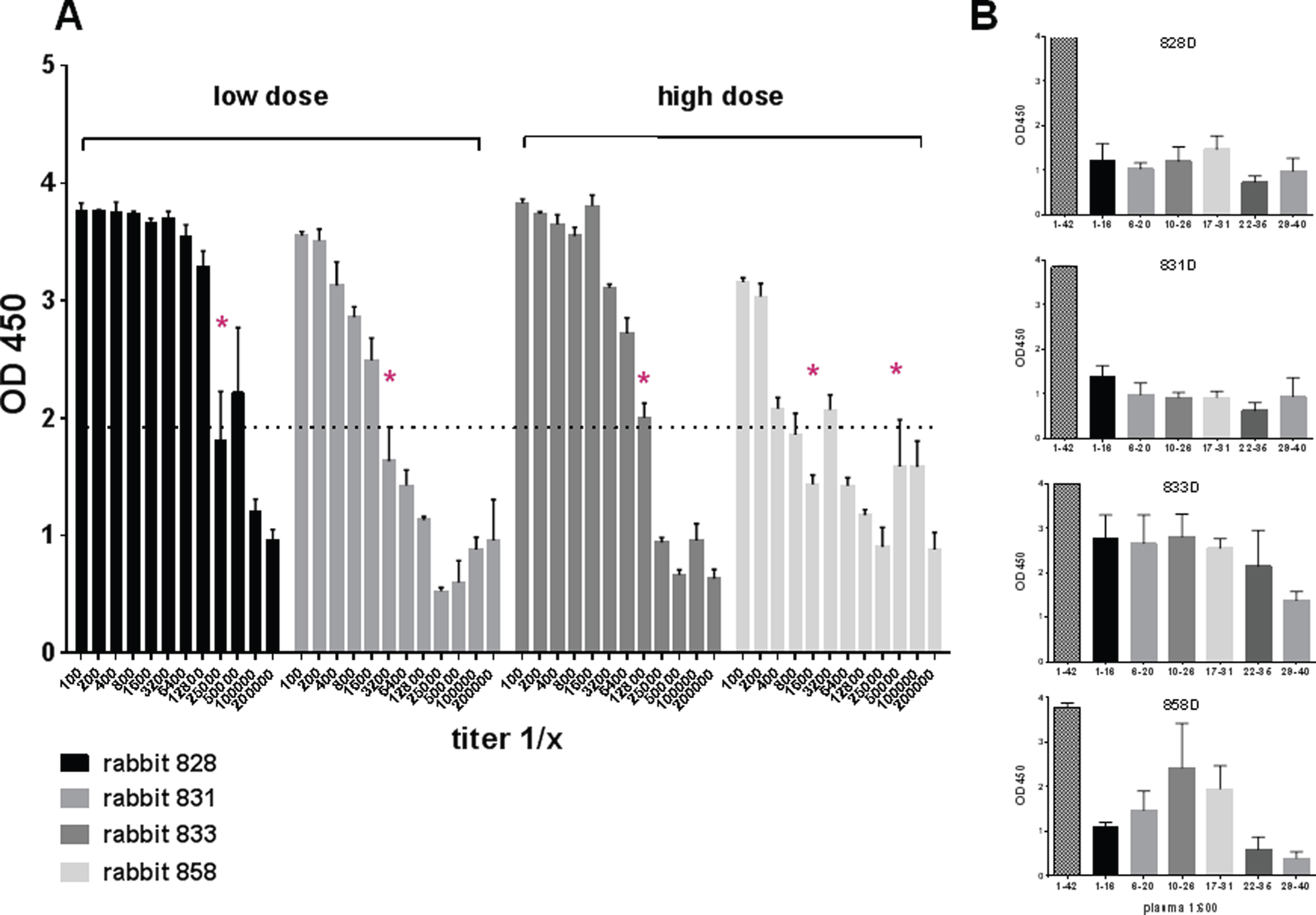
Fig.4
Antibody production in NZW rabbits following different DNA Aβ42 immunization time points. A) Anti-Aβ42 antibody levels in plasma are shown prior to the immunizations, and for 2x, 3x, and 5x immunized animals. The light grey bars show antibody levels in low dose rabbits, the dark grey bars show the levels in high dose rabbits. In B, the number of antibody forming units/cells (AFC) in spleens from DNA Aβ42 immunized rabbits as measured in a B cell ELISPOT assay is shown. C) The corresponding levels of anti-Aβ42 IgG antibodies produced in splenocyte cell cultures are shown. D-F) Binding of the anti-Aβ IgG (D), IgA (E), and IgM (F) antibodies of all sixteen rabbits after the 4th immunization to full-length Aβ1-42 and a panel of shorter Aβ peptides. Plasma dilutions in these assays were 1:400. ****, p-value of < 0.0001.
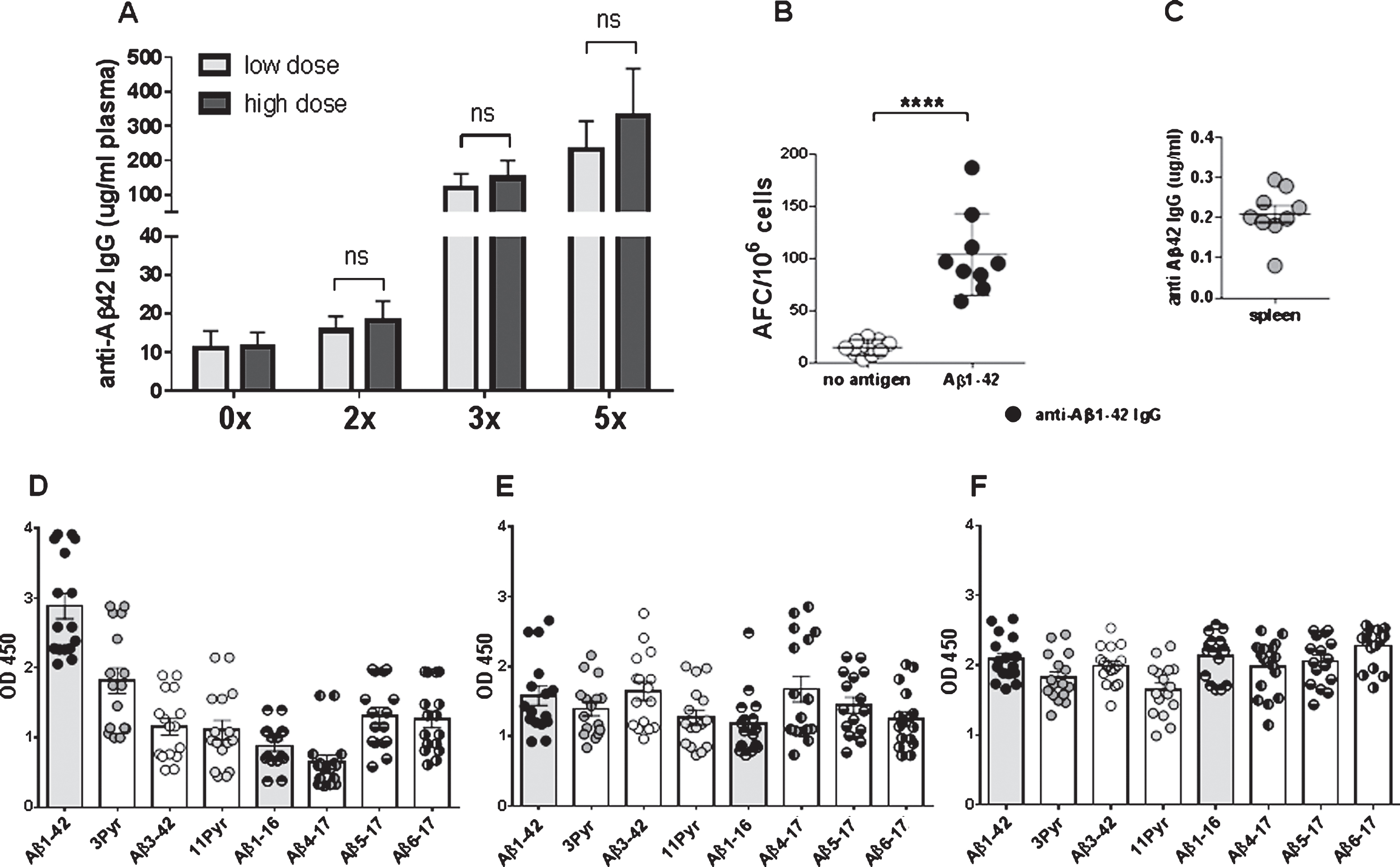
Fig.5
Antibody production in NZW rabbits following continued DNA Aβ42 immunization time points (up to 10x), determination of antibody half-life, and Aβ antibody epitope distribution. A) The plasma antibody levels from six rabbits following the 5th, and the 10th immunization are shown, as well as the respective antibody levels following a two or four month interval without further vaccination (resting). B) The antibody half-life was determined as three months. C-F) Epitope binding for antibodies from six rabbits was determined for the four time points. G) The amino acid sequence of Aβ peptides used for the epitope analyses is shown. Plasma dilutions used in these analyses (A-G) was 1:1000.
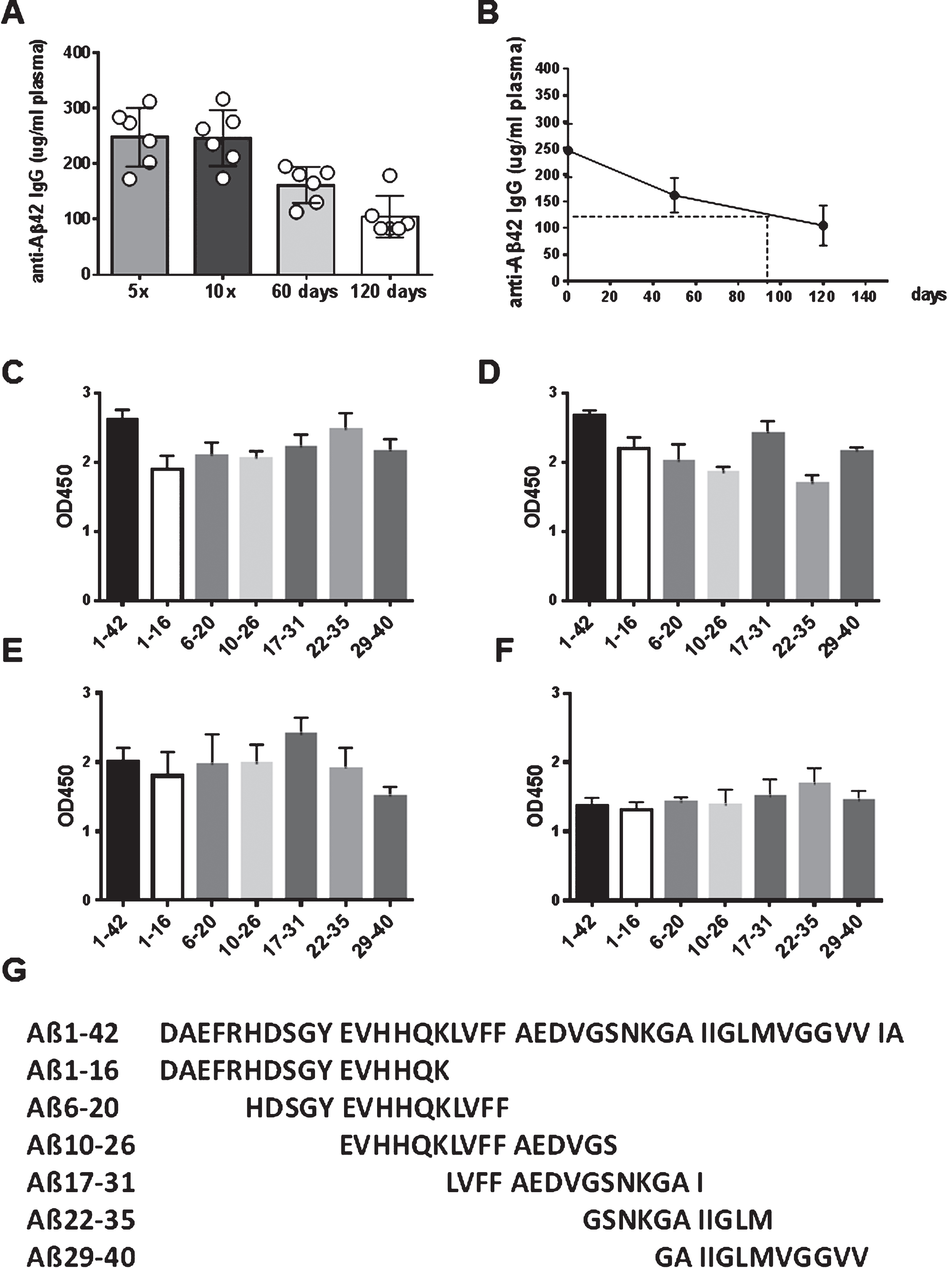
Fig.6
Antibody isotypes and epitopes of anti-Aβ antibodies produced in the rabbit. A) The humoral immune response generated anti-Aβ IgG, - IgM, and - IgA antibodies in the immunized rabbits. Rabbit plasma samples had been used in a 1:1500 dilution, the commercial available rabbit antisera had been used in a 1:2000 dilution. B) Antibodies in plasma from DNA Aβ42 trimer immunized rabbits reacted with several N-truncated and N-modified Aβ42 peptides, as well as α-synuclein. The two commercial available antisera from peptide immunized rabbits reacted strong with Aβ1-42, and much weaker with the N-truncated or Pyroglutamated Aβ42 peptides, and α-synuclein. In fact, only the antiserum generated against Aβ1-42 showed some weak reactivity with these peptides, the antiserum generated against Aβ1–15 did not.
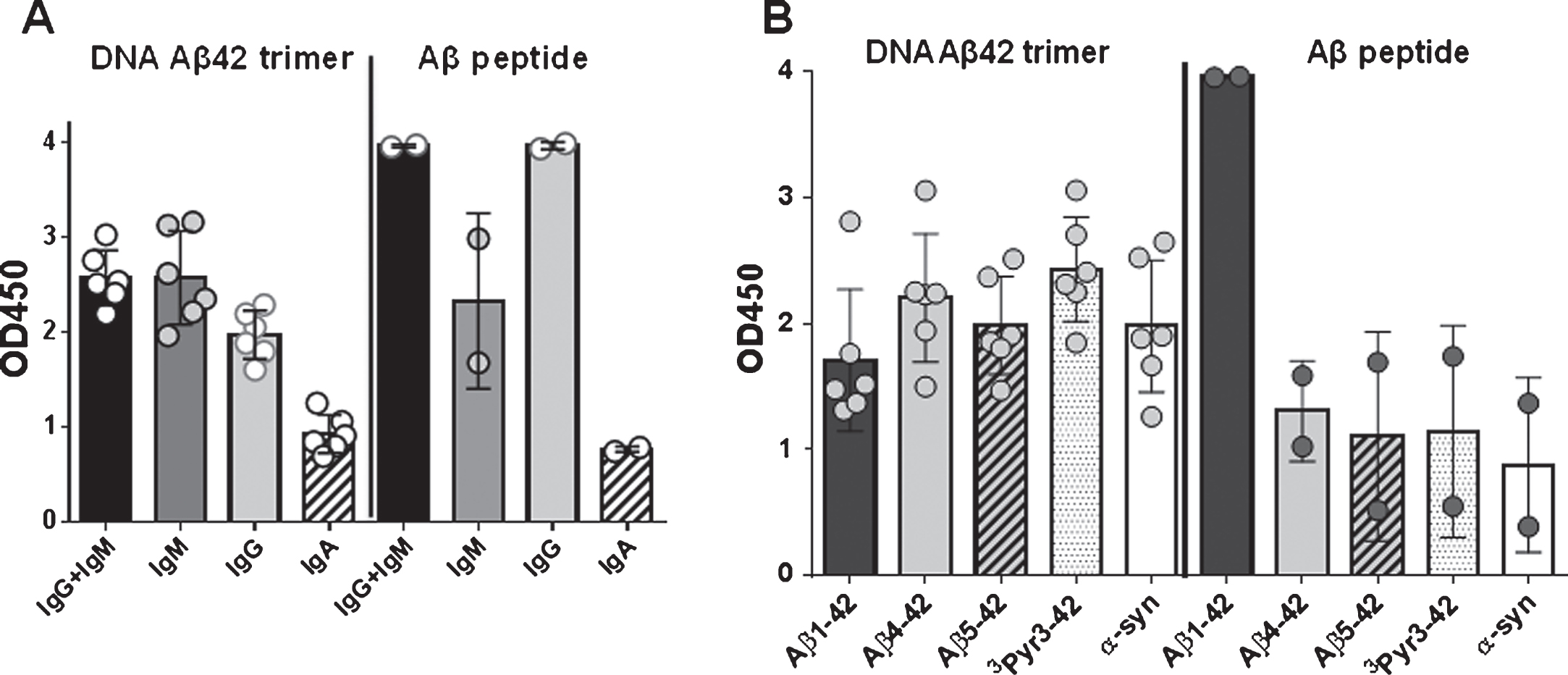
Fig.7
Comparison of the antibody binding of plasma from six rabbits with the commercial anti-Aβ42 antibodies, 6E10 and 1-11-3, and the anti-oligomer antibody A11 in a dot-blot assay (A) and NaCN Affinity ELISA (B). A) Nitrocellulose filters contained duplicate spots of the peptides indicated on the bottom of the filters (1–4). Filters were incubated with the different rabbit plasma samples or monoclonal antibodies as noted above of each of the membranes. Rabbit plasma was used in a 1:200 dilution, the moabs 6E10 and 1-11-3 had been used in a 1:5.000 dilution, A11 was used in a 1:2.000 dilution. B) Avidity of the antibody binding was studied using the chaotrophic salt NaCN in different concentrations. A comparison of binding of the rabbit plasma samples and moabs 6E10 and 1-11-3 to Aβ1-42, Δ22Aβ1-42, Aβ1 - 40, and α-synuclein is shown. Rabbit plasma samples (0.2 mg/ml) were used in a dilution 1:500; 6E10 and 1-11-3 (1 mg/ml) were used in a dilution 1:5.000. Samples were tested in triplicates, mean values are shown.
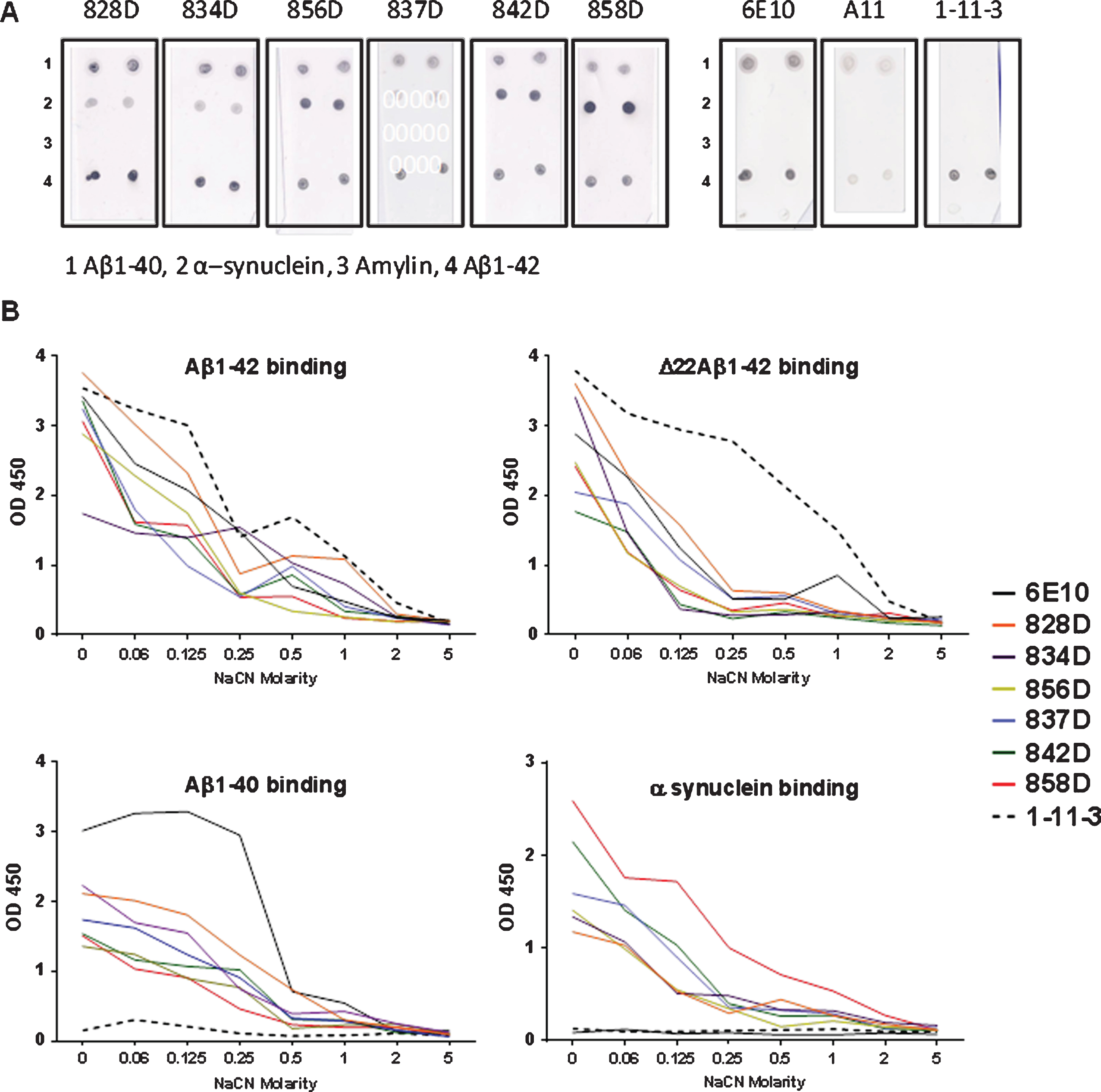
Fig.8
Cross-reactivity of antibodies in plasma of DNA Aβ42 immunized rabbits with α-synuclein. A) The plasma samples from fifteen rabbits from the 5th immunization time point were tested for binding to a variety of amyloid [amylin, islet amyloid protein (IAPP), serum amyloid P component (SAP)] and α-synuclein peptides (1–140, Δ61–95, and 1–60). Plasma was diluted 1:500 for these assays. No significant differences were found for the comparison of binding to Aβ1-42 and the α-synuclein peptides, whereas significantly less binding was found for amylin, IAPP, and SAP (Mann-Whitney p < 0.0001). B) The six rabbits which received continued immunizations were extracted from the 15 samples in A and shown again in B for a better comparison to C. Levels of significance dropped due to the smaller sample size, but were still highly significant in the comparison of binding to Aβ1-42 with binding to amylin or SAP (Mann-Whitney p = 0.0043 and 0.0173). C) Cross-reactivity of plasma antibodies in six rabbits, which had received a total of ten DNA Aβ42 immunizations, to α-synuclein was confirmed, as well as no binding to amylin, SAP, and IAPP. *, **, ***, and **** indicate p-values of < 0.05, < 0.005, < 0.0005, and < 0.0001, respectively.
![Cross-reactivity of antibodies in plasma of DNA Aβ42 immunized rabbits with α-synuclein. A) The plasma samples from fifteen rabbits from the 5th immunization time point were tested for binding to a variety of amyloid [amylin, islet amyloid protein (IAPP), serum amyloid P component (SAP)] and α-synuclein peptides (1–140, Δ61–95, and 1–60). Plasma was diluted 1:500 for these assays. No significant differences were found for the comparison of binding to Aβ1-42 and the α-synuclein peptides, whereas significantly less binding was found for amylin, IAPP, and SAP (Mann-Whitney p < 0.0001). B) The six rabbits which received continued immunizations were extracted from the 15 samples in A and shown again in B for a better comparison to C. Levels of significance dropped due to the smaller sample size, but were still highly significant in the comparison of binding to Aβ1-42 with binding to amylin or SAP (Mann-Whitney p = 0.0043 and 0.0173). C) Cross-reactivity of plasma antibodies in six rabbits, which had received a total of ten DNA Aβ42 immunizations, to α-synuclein was confirmed, as well as no binding to amylin, SAP, and IAPP. *, **, ***, and **** indicate p-values of < 0.05, < 0.005, < 0.0005, and < 0.0001, respectively.](https://content.iospress.com:443/media/jad/2017/57-1/jad-57-1-jad160947/jad-57-jad160947-g008.jpg)
Fig.9
ELISPOT analyzes for Aβ42 peptide re-stimulated splenocyte cultures of DNA Aβ42 immunized rabbits. A) The number of IL-17 secreting cells is shown following 48 h in cell culture. B) The number of IFNγ secreting cells. C) The number of IL-4 secreting cells per 106 splenocytes. *p < 0.05, **p < 0.005, ****p < 0.0001.
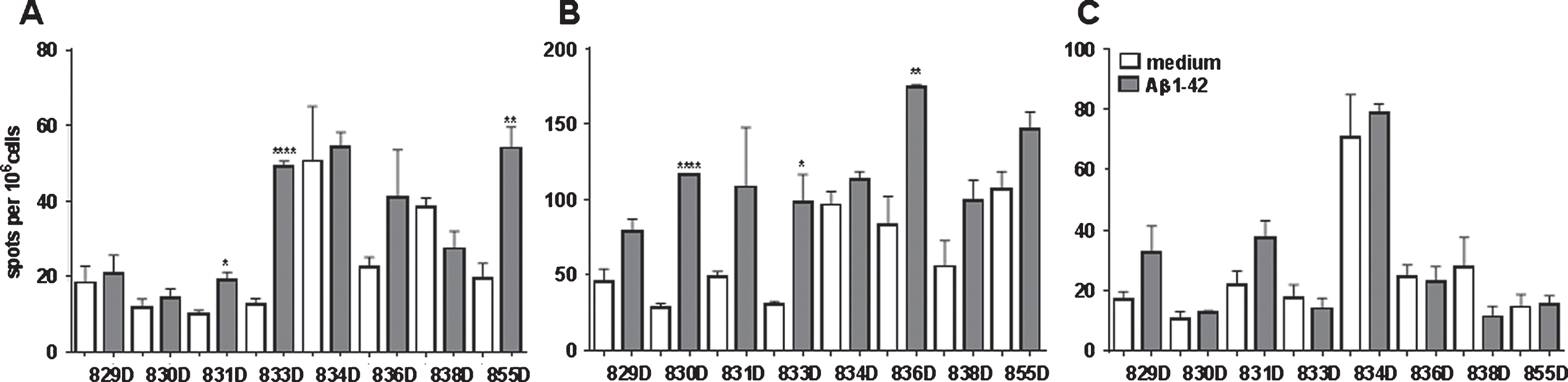
Fig.10
CD4 T cell proliferation in Aβ42 peptide re-stimulated splenocyte cultures. CD4 proliferation was analyzed using a CFSE dilution assay. Events were gated on CD4 positive cells, and percentages directly taken from the flow cytometer histograms for medium controls and Aβ42 peptide re-stimulated cultures. All samples were run in triplicates. ** and **** indicate p-values of < 0.05 and < 0.0001, respectively.
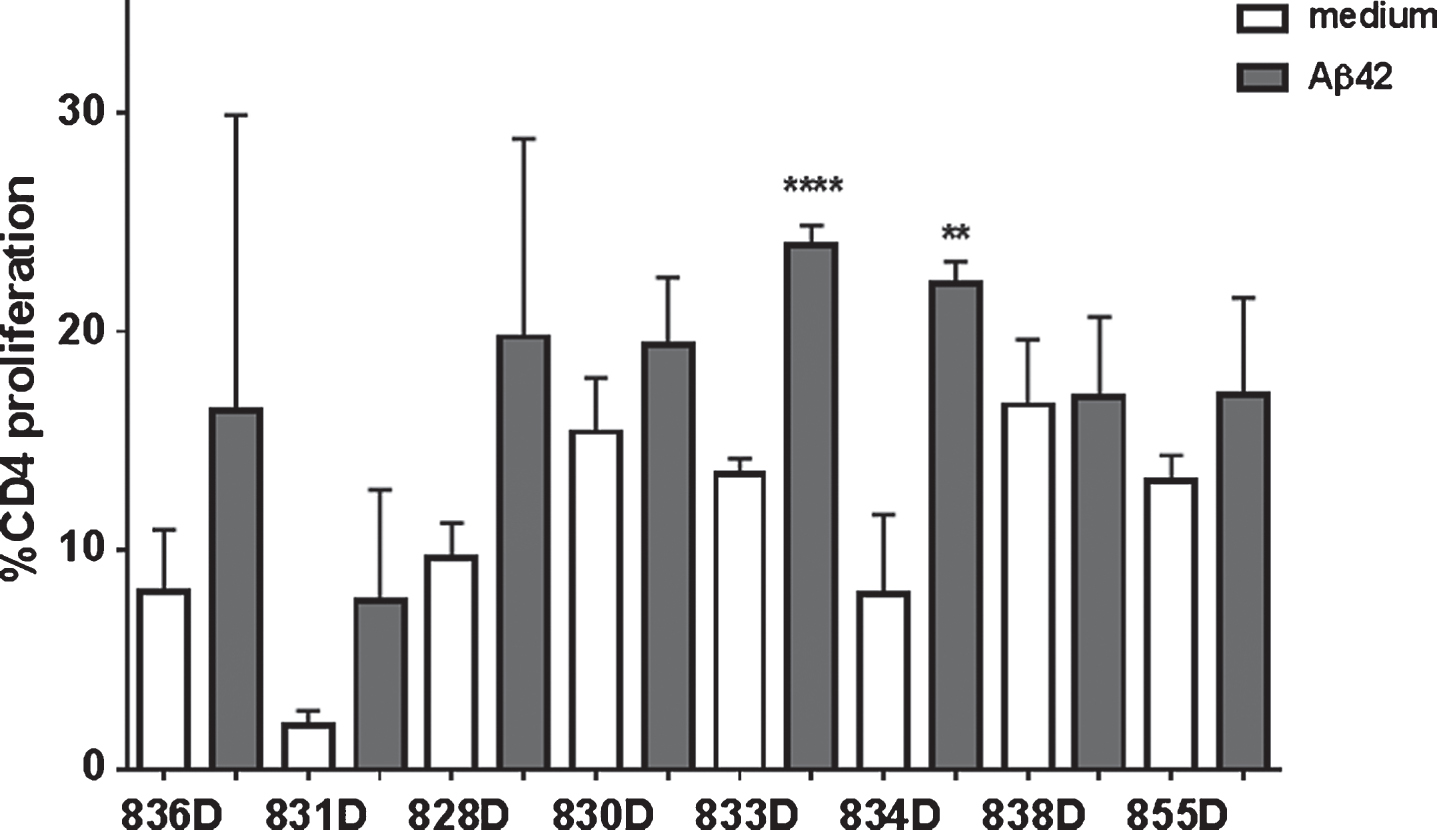
Fig.11
Analyses of the CD4 proliferation in rabbits 833D (A to D) and 831D (E to G). A) A characteristic dot histogram for the analysis of proliferating cells is shown. Gates were set around the CD4+ T cells (R1). B) Histogram and marker show the percentage of proliferating cells. C, D) These cells were further analyzed using the FCS express proliferation software. E-G) The same analyses for CD4+ T cells of a different rabbit in medium (E), Aβ42 peptide containing medium (F), and after ConA stimulation (G).
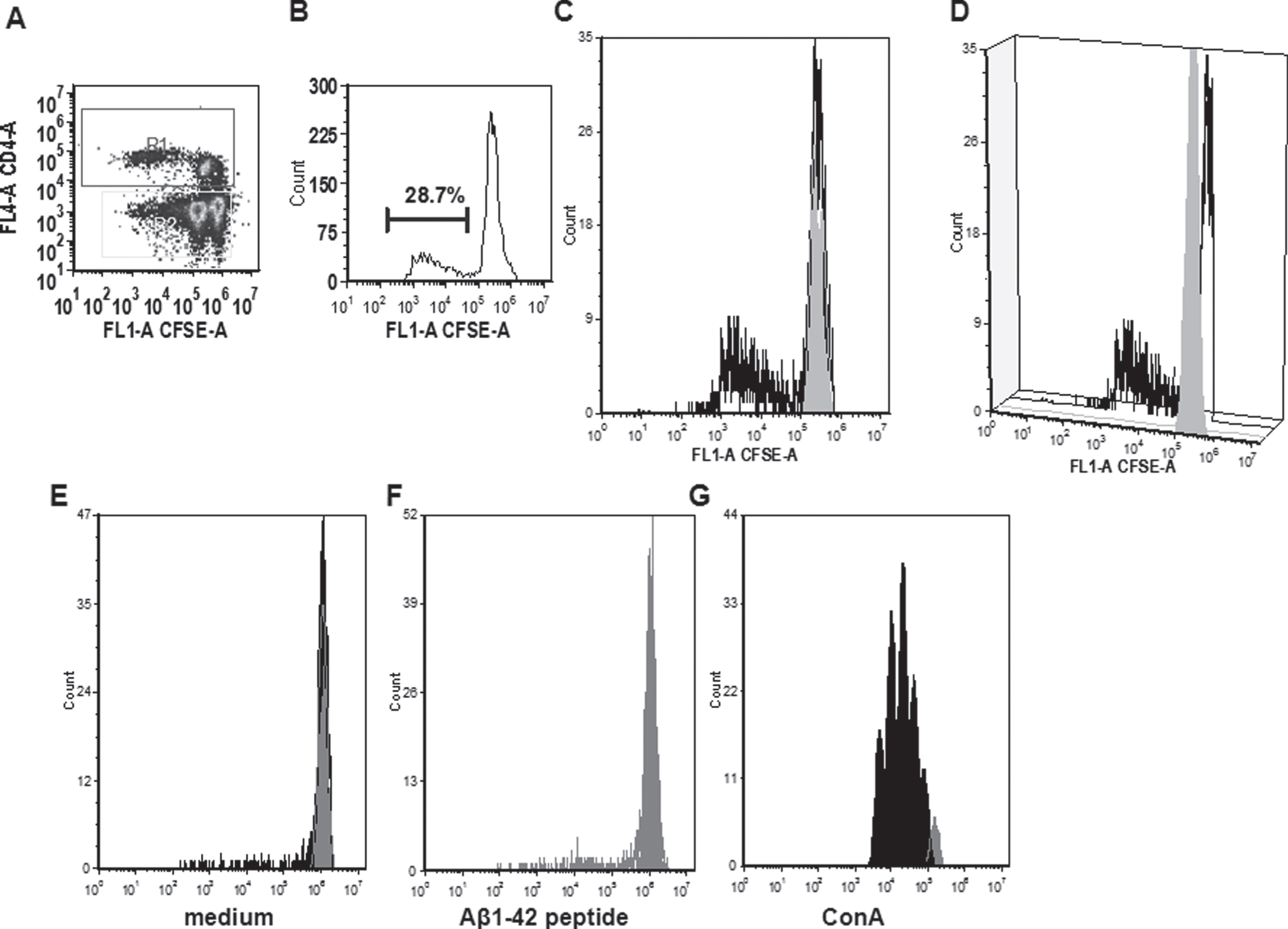
Fig.12
Antibodies from DNA Aβ42 immunized rabbits stain amyloid plaques in brain of 3xTg-AD mice (24 months old mouse). A) Aβ staining in hippocampus area with plasma from rabbit 828D (brown), B) control staining in parallel section with commercial anti-Aβ42 antibody (McSA1, brown) and NeuN (red), C) control staining of brain section from this 3xTg-AD mouse with control serum from a non-immunized rabbit, D–F) show no staining with rabbit antiserum and commercial antibody in plaque free cerebellum. In all sections, a 10x magnification is shown (Hamamatzu Nanozoomer). Asterisks indicate plaques stained with rabbit plasma (in A, blue in the color version online and black in the print version) and commercial control antibody (in B, green in the color version online and black in the print version).
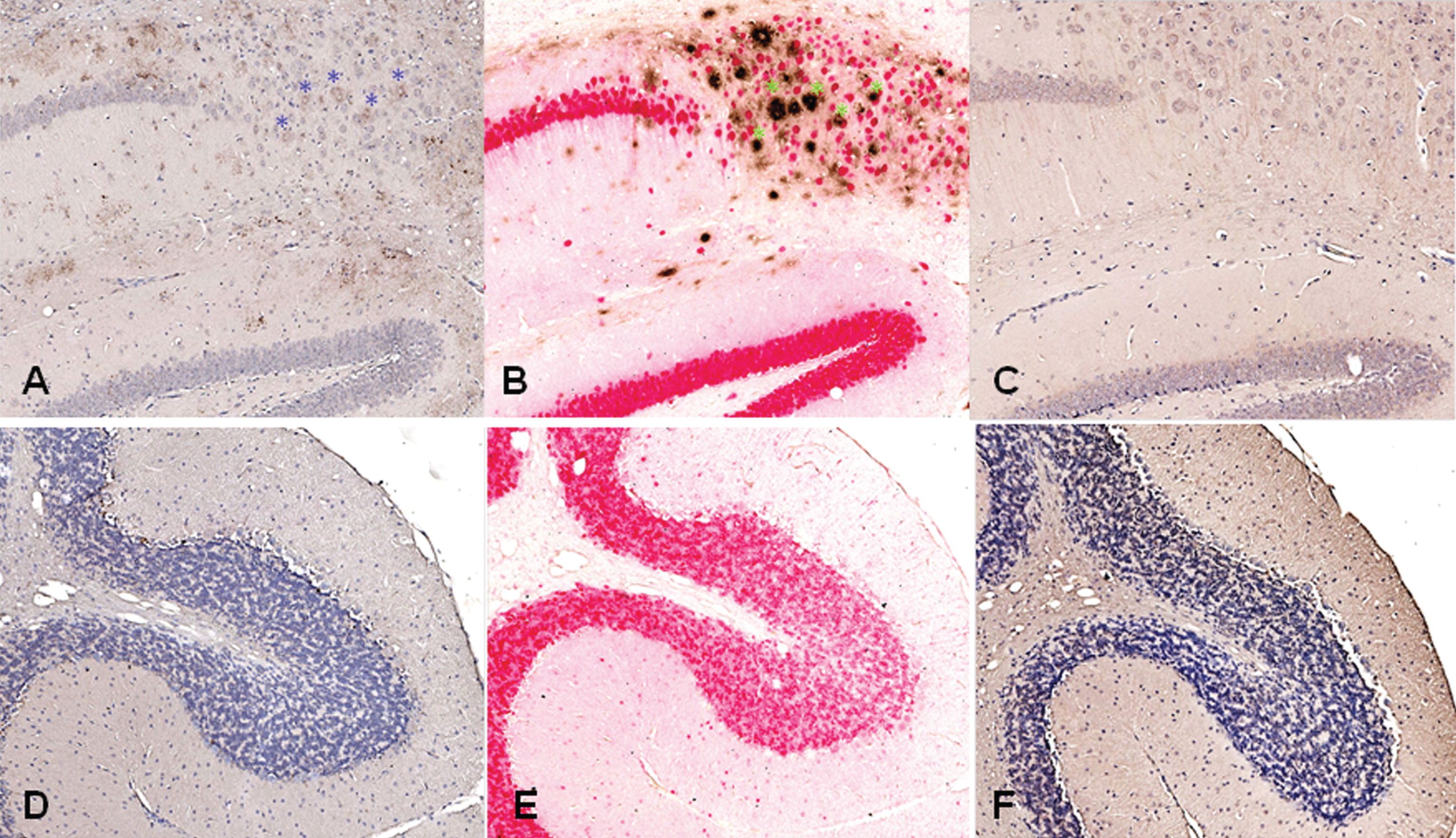