The Association Between Positive Amyloid-PET and Cognitive Decline Is Not Always Supportive of Alzheimer’s Disease: Suggestions from a Case Report
Abstract
Amyloid-β deposition is the pathological hallmark of both cerebral amyloid angiopathy and Alzheimer’s disease dementia, clinical conditions that can share cognitive decline and positive Amyloid-PET scan. A case is reported involving an 82-year-old Italian female who presented initially a memory deficit, later transient focal neurologic episodes, and finally two symptomatic lobar intracerebral hemorrhages. In light of these events, MRI and PET imaging findings, acquired before cerebral hemorrhages, are reconsidered and discussed, highlighting the utility of Amyloid-PET in supporting an in vivo diagnosis of cerebral amyloid angiopathy.
INTRODUCTION
Brain amyloid-β deposition is the pathological hallmark of two common diseases of the elderly: cerebral amyloid angiopathy (CAA) and Alzheimer’s disease (AD), conditions that in their early phase can share a clinical symptom, that is cognitive decline, and an imaging finding, that is Amyloid-PET (Amy-PET) tracer retention. Differential diagnosis is essential to manage proper drug prescription, avoiding anticoagulants and antiplatelet agents when CAA is suspected, and prescribing acetylcholinesterase Inhibitors in AD dementia. Comparing the pathology of these two conditions, sporadic CAA is caused by amyloid-β deposition in the walls of cortical and leptomeningeal small vessels, whereas in AD the deposition occurs in the cerebral cortex. The most widely recognized MRI markers of CAA are lobar intracerebral hemorrhage (ICH) (44%), cortical superficial siderosis (CSS) (49%), cerebral microbleeds (MBs) (52%), lacunar infarcts (30%), white matter hyperintensities with moderate or severe Fazekas score (53%), and enlarged perivascular spaces located in centrum semiovale and basal ganglia; subarachnoid hemorrhage can also be found, alone or with ICH [1]. The main structural MRI findings in early AD are atrophy in the hippocampus and the entorhinal cortex [2] which can also be quantified with visual scales [3]. Noteworthy, atrophy in this region may be missing in early-onset AD, where posterior cortical atrophy is often predominant [4]. Clinically, CAA may be associated with transient focal neurologic episodes (TFNE) (48%), called “amyloid spells” and with cognitive decline (50%), or with symptomatic ICH [1]. The identification of a specific pattern of neuropsychological impairment in CAA patients remains challenging as such lesions are multiple, heterogeneous, and variable both within and between patients, thus, multiple domains could be involved and psychiatric symptoms may also occur [5]. TFNEs were more often found in association with focal CSS [6] and are often misdiagnosed with recurrent transient ischemic attacks or focal seizures in CAA patients.
In early stages, AD occurs with typical “amnestic presentations” involving episodic memory with or without other deficits, and less frequently with atypical non-amnestic presentation, involving other cognitive domains.
According to the current diagnostic criteria for sporadic CAA, the Boston criteria version 2.0 [7], a “definite” diagnosis of CAA requires a full postmortem examination, whereas the in vivo diagnosis of “probable CAA with supporting pathology” requires the presence of clinical manifestations and of typical pathological tissue alterations detectable analyzing the evacuated hematoma or material from cortical biopsy. “Probable” and “Possible” CAA can be diagnosed using clinical findings and typical MRI markers, in the absence of other causes of hemorrhagic lesions. Since the pathology occurs beyond the age of 50, often patients present comorbidities, making difficult an in vivo diagnosis of CAA. In this regard, the localization of hemorrhagic lesions is essential to exclude hypertension-related ICH because the absence of any deep lesions on T2*weighted MRI is part of the diagnostic criteria of CAA [7]. Emerging evidence suggests the possible advantage of using Amy-PET to help distinguish between CAA–related ICH from hypertension-related ICH, given the capability of Amy-PET tracers in labeling vascular amyloid in patients with CAA-related ICH [8, 9]. Thus, since Amy-PET detects both CAA cerebrovascular and AD cortical amyloid, a negative scan might rule out CAA in the presence of ICH, whereas, when cognitive decline occurs as an isolated symptom, a positive scan does not permit the differentiation of CAA from incipient AD [10]. On the other side, according to the biological definition of AD, “A+”, as sustained by a positive Amy-PET, is sufficient to be classified in the “AD continuum” [11]. In brief, in the absence of ICHs, it is currently lacking an approach to differentiate with an Amy-PET between AD and CAA. Therefore, given the limitation due to a simple visual approach, a quantitative approach to Amy-PET, specifically implemented to differentiate between AD and CAA, has recently been proposed [10]. Regarding FDG-PET imaging, in CAA, a significant glucose hypometabolism in posterior cortical regions, including the visual cortex compared to healthy subjects [12], and a lower occipital/posterior cingulate standardized uptake value ratio (SUVR) compared to AD subjects [13] have been reported.
CASE REPORT
A 77-year-old woman, 5 years educated, was evaluated at the Neurology Unit of the Careggi University Hospital in Florence, because affected since 8 months by slow and progressive memory problems. No family history of dementia was referred, her father died as a consequence of a stroke. Since the age of 75, she was also affected by essential thrombocythemia (ET) treated with aspirin 100 mg and hydroxyurea 500 mg per day with stable platelet count in the years (550×109/L). Among investigations performed, neurological examination was unremarkable; neuropsychological examination confirmed memory and attention deficits (Mini-Mental State Examination (MMSE) score 25/30), a picture compatible with mild cognitive impairment (MCI); Apolipoprotein E genotype was ɛ2/3; a brain CT scan showed chronic microvascular ischemic disease; an FDG-PET scan was apparently within the normal range whereas an Amy-PET with Florbetapir, performed two years later, was positive (Fig. 1a), the last finding supporting a diagnosis of MCI due to AD [14]. Three years after the cognitive symptoms onset, the patient complained of stereotyped reversible recurrent episodes characterized by sudden paresthesia in the left upper limb and dysarthria lasting about 30 min. Cognitive decline was stable at this time (MMSE 25/30). A brain MRI on T2 FLAIR WI showed a small hyperintensity near the right precentral sulcus (Fig. 1b); on T2* weighted imaging (WI) an abnormal hypointense signal was evident (Fig. 1c) and it was indicative of hemosiderin deposition along with the right Rolandic fissure. Since the patient was affected by ET, a three-dimensional CT venography was also performed to exclude cerebral venous thrombosis. An FDG-PET scan carried out at the time of cognitive symptom onset has been not suggestive of a typical AD-like pattern (Fig. 1d). The stereotyped neurological manifestations were suspected for focal seizures and Carbamazepine was administered with only partial improvement. At the age of 82, the patient became suddenly symptomatic for aphasia and headache. An MRI scan revealed the occurrence of a lobar hemorrhage in the left temporo-polar region (Fig. 1e), siderosis in the left temporal sulcus (Fig. 1e), white matter hyperintensity (Fig. 1f), and CSS in the right superior frontal sulcus (Fig. 1g). The platelet count was stable (536 x 109/L) and, according to good clinical practice, aspirin administration was interrupted. MMSE score performed after hemorrhage resolution was 21/30, indicative of worsening in orientation and memory skills, and the clinical picture was characterized also by depression and psychomotor slowing at this time. Eight months later the patient became suddenly symptomatic for left hemiplegia and a CT scan revealed the presence of a large hemorrhage in the right fronto-parietal region, with small amount of subarachnoid hemorrhage (Fig. 1h, i). The platelet count was again stable, and the patient died after one month. Thus, a diagnosis of CAA was eventually taken into account. To support this hypothesis, a quantitative region-of-interest (ROI) analysis of Amy-PET imaging was performed using CortexID software (GE Healthcare, Waukesha, WI, USA), assuming the cerebellum (whole) as reference region, and it revealed a significant SUVR in the occipital cortices (SUVR/Z SCORE 1.6/6.5, 1.7/7.8 on the right and left, respectively) (Table 1 and Fig. 2).
Fig. 1
a) Florbetapir PET (age 79): amyloid presence in the brain. b) Brain MRI on T2 FLAIR WI (age 80): small hyperintensity in the right precentral lesion. c) Brain MRI on T2* WI (age 80): hemosiderin deposition along with the right Rolandic fissure. d) FDG-PET imaging (age 77): absence of AD-like pattern. e) Brain MRI on T2* WI (age 82): lobar hemorrhage in the left temporopolar region, cortical superficial siderosis in the left temporal sulcus. f) Brain MRI on T2 FLAIR WI (age 82): white matter hyperintensity. g) Brain MRI on T2*WI (age 82): cortical superficial siderosis in the right superior frontal sulcus. h, i) Brain CT (age 82): large hemorrhage on the right fronto-parietal region, small amount of subarachnoid hemorrhage. (Permission to share imaging was granted by the patient before death).
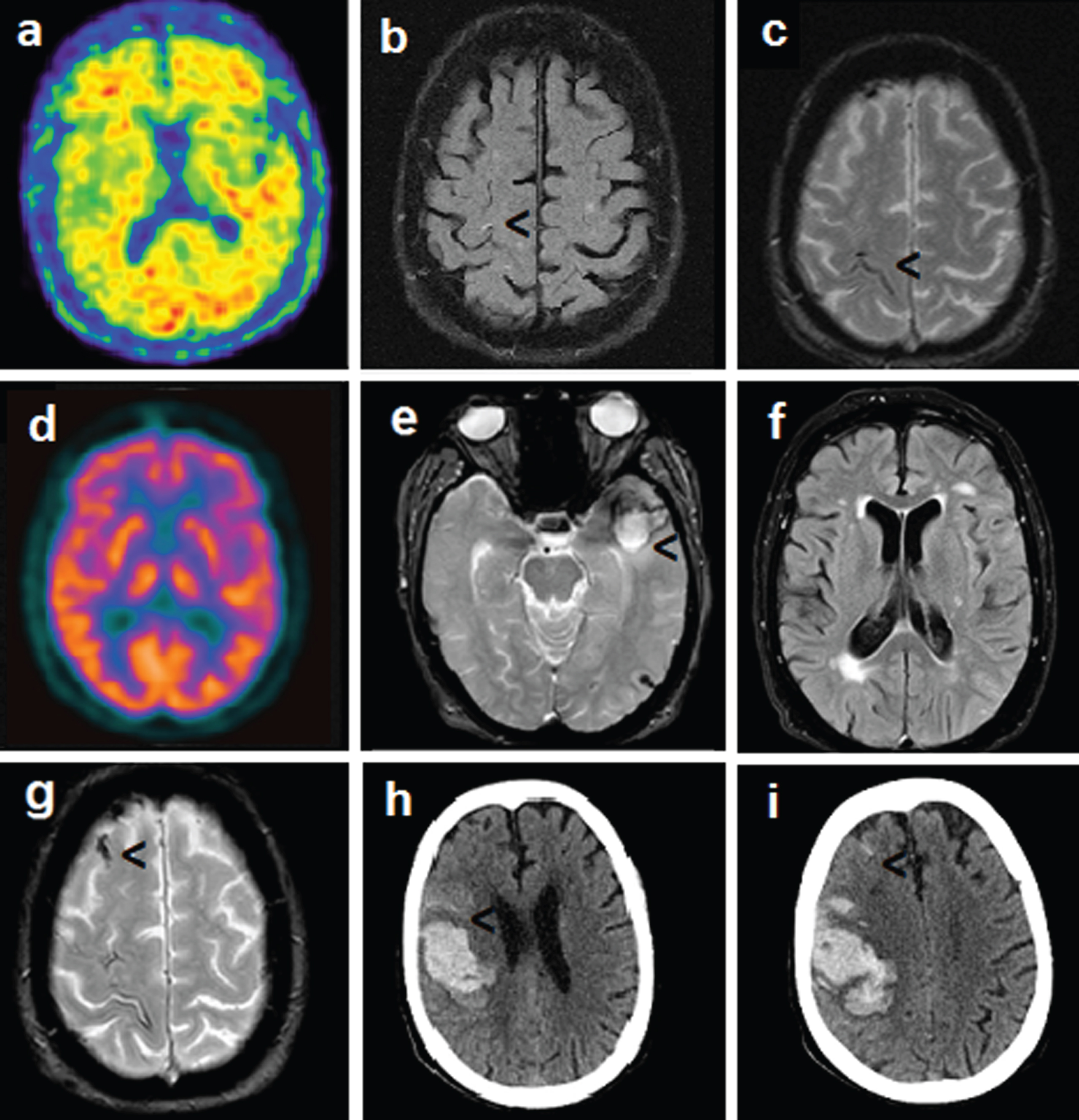
Table 1
Regional SUVRs obtained applying ROI analysis: higher Z-scores and SUVR for the occipital regions
REGION | SUVR | Z SCORE |
Composite | 1.45 | 5.11 |
Prefrontal R | 1.41 | 4.19 |
Prefrontal L | 1.40 | 4.13 |
Anterior Cingulate R | 1.24 | 1.78 |
Anterior Cingulate L | 1.22 | 0.96 |
Precuneus-Posterior Cingulate R | 1.56 | 5.24 |
Precuneus-Posterior Cingulate L | 1.69 | 5.95 |
Parietal R | 1.44 | 4.25 |
Parietal L | 1.42 | 4.73 |
Temporal Lateral R | 1.51 | 4.94 |
Temporal Lateral L | 1.48 | 5.06 |
Occipital R | 1.59 | 6.50 |
Occipital L | 1.71 | 7.78 |
Sensorimotor R | 1.40 | 3.58 |
Sensorimotor L | 1.46 | 4.51 |
Temporal Mesial R | 1.10 | 0.25 |
Temporal Mesial L | 1.20 | 1.72 |
Cerebellum Grey | 0.89 | 1.36 |
Cerebellum Whole | 1 | 0.00 |
ROI, region of interest; SUVR, standardized uptake value ratio.
Fig. 2
Z-SCORE images are shown with a dedicated color table at the bottom of the figure (from 0 to 8), a threshold of 2 SD applied to the images; cerebellum whole as reference region in the ROI analysis performed with CortexID software; higher Z-score and standardized uptake value ratio (SUVR) for the occipital regions. a) right lateral view; b) left lateral view; c) right medial view; d) left medial view; e) anterior view; f) posterior view; g) superior view; h) inferior view.
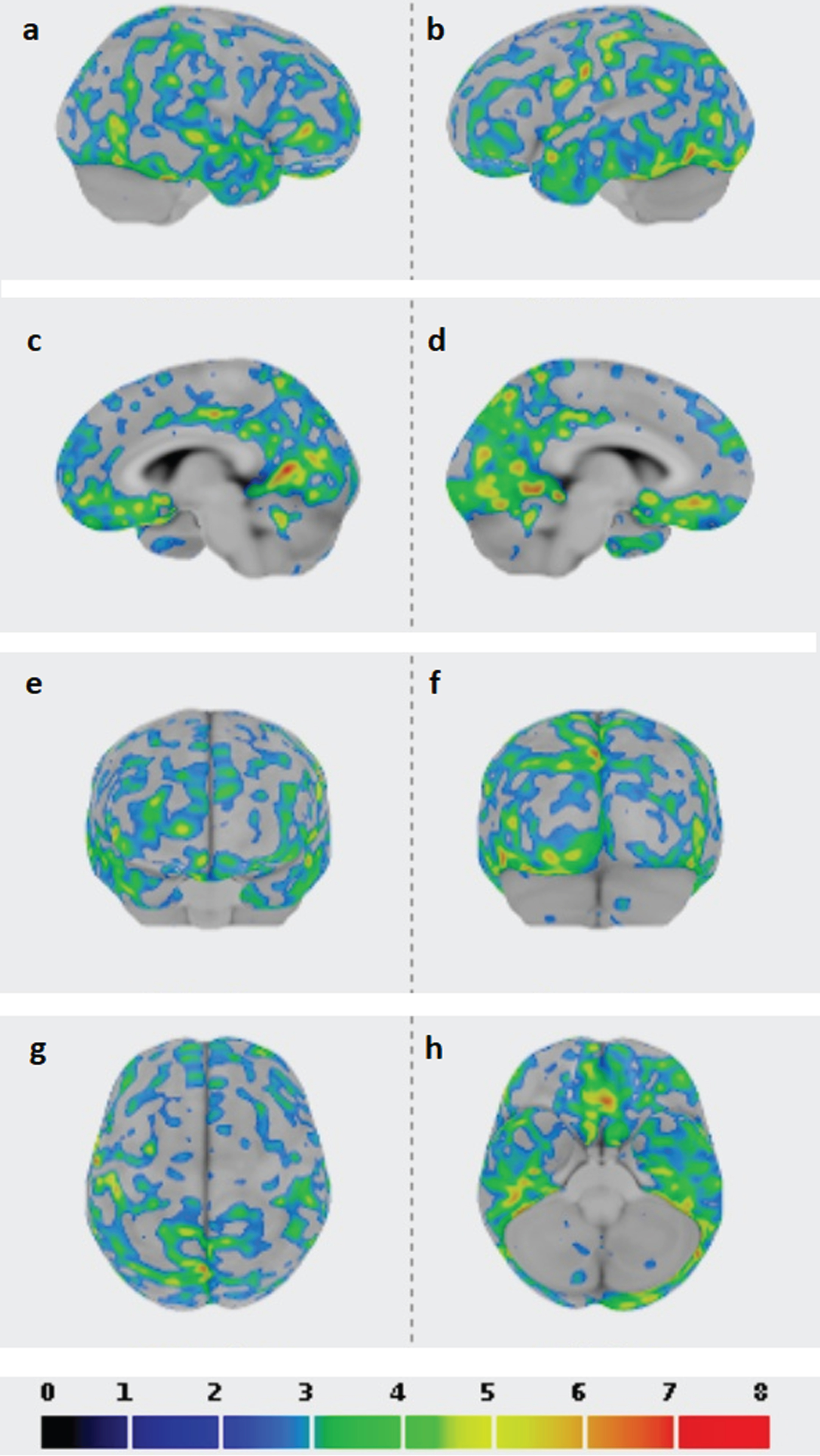
DISCUSSION
We illustrate a case characterized by multiple and progressive brain vascular lesions (first CSS and then recurrent lobar ICHs) in a patient affected by ET. Even in the presence of other factors that could enhance bleeding (ET diagnosis and aspirin use at the time of the first ICH), clinical symptoms and imaging findings were suggestive of CAA. Regarding clinical symptoms, cognitive decline occurred as the first manifestation of the disease. As suggested by Bolouis it is possible that patients first symptomatic for cognitive symptoms may have a more progressive silent expression of the disease compared to those symptomatic for TFNEs, the so-called focally active hemorrhagic phenotype [15]. Cognitive impairment is common in CAA, even in the absence of ICH, suggesting a key role for diffuse processes related to small vessel disease and/or neurodegeneration [16].
Concerning imaging findings, CSS is considered an MRI marker that is closely related to an increased risk of bleeding in CAA [1, 17], and it has been described in association with Amy-PET tracer retention, supporting the hypothesis that CSS reflects an amyloid etiology [18]. Moreover, the Apolipoprotein E ɛ2 allele (present in this case), has been recently described in association with CSS [18] and with the risk of bleeding in CAA [1, 19]. Differently, no relevant burden of MBs was detectable on MRI. However, the MB burden, known to be associated with the Apolipoprotein E ɛ4 allele, is less evident in cases with evident CSS [20]. Altogether, recurrent ICHs and the increment of CSS, as the amyloid tracer uptake at the Amy-PET, were imaging markers suggestive of an underlying CAA pathology, whereas the presence of normal blood flow at the three-dimensional CT venography scan ruled out the hypothesis of venous thrombosis. The result of the FDG-PET was not indicative of an AD-like pattern. Given the high sensitivity of FDG-PET to recognize AD [21], we used this visual finding to question a diagnosis of AD. Indeed, recent reports suggest the potential of FDG-PET itself to differentiate between CAA and AD [13], even if with lower strength compared to Amy-PET [22]. Nowadays a formal diagnosis of CAA is not accepted unless a brain biopsy is performed, making it hard to obtain an in vivo formal diagnosis. While the diagnostic role of MRI in the second version of Boston criteria is well established, that of Amy-PET is still not acknowledged. Amy-PET is a noninvasive imaging technique with the potential to provide a molecular signature of CAA adding specific biologic information in supporting CAA diagnosis. Amy-PET is largely used in the diagnostics of AD even if some limitations are acknowledged such as the ability to detect the presence of amyloid in the brain rather than specifically AD pathology. Moreover, the amyloid uptake may be influenced by multiple factors such as age, cerebral comorbidities, tracers characteristics and plaques conformation, highlighting that the association between positive Amy-PET and cognitive decline is not always supportive of AD [23]. The ability of Amy-PET to detect the presence of brain amyloid regardless of the underlying pathology, which is a limit in AD diagnostics, offers the opportunity to use Amy-PET in the diagnostics of other amyloid pathologies when this imaging technique is properly analyzed and interpreted. Quantitative and visual analysis has been demonstrated to be useful for distinguishing between CAA-related ICH versus hypertension-related ICH [8], while a major issue regarding the diagnostic usefulness of Amy-PET in suspected CAA is whether a significant difference exists in the regional distribution pattern of amyloid deposition, as it is found in hereditary CAA [24].
In this regard, an author compared the occipital/whole cortex uptake between AD and sporadic CAA cases in “late phase amyloid imaging” (using PIB as tracer and SUVR, pons as reference tissue), detecting a significantly higher occipital/whole cortex ratio in CAA than in AD cases [25].These findings have been confirmed later by other authors using again PIB as tracer and cerebellar cortex as the reference region [26], whereas Planton found only a trend for an increased occipital/global ratio in CAA-ICH patients compared to MCI-AD using Florbetapir as tracer and cerebellar cortex as the reference region [27]. The use of Amy-PET in the diagnostic of CAA is also supported by recent reviews on this topic [22, 28].
Since in the present case, occipital SUVRs are at the highest level of significance (Table 1), our results are in line with the previous works of Johnson et al. [25] and Chang et al. [26] and encourage the use of Amy-PET together with MRI in the diagnosis of CAA [29], especially utilizing a quantitative data analysis. In this regard, even if CortexID has not been previously used in the context of Amy-PET in CAA research, it has the advantage of performing a fully automated spatial normalization applied to brain PET data without the need for same-patient MRI coregistration [13].
Recently, Farid et al. proposed a diagnostic stepwise algorithm of Amy-PET use in the clinical setting of suspected CAA based on a “late-phase Amy-PET” comparison to young controls; only in the case of a positive scan, the regional pattern is analyzed to establish whether the cortical uptake is higher in the occipital compared to the whole cortex. However, definitive results on the diagnostic value of regional uptake for differentiating CAA from AD, are still currently lacking [10]. Overall, even if amyloid distribution may differ between patients with CAA and with AD (i.e., highest retention in occipital regions in CAA), the spatial resolution of PET may affect the distinction between cortical and vascular amyloid deposition [30] and the exams is not always reported as positive in case of CAA, being the positivity referred to the presence of MBs and CSS [31].
Conclusions
Our results suggest that “hemosiderin-dedicated MRI sequences” as T2*WI gradient echo or susceptibility WI should be always performed and repeated in elderly patients with recurrent TFNS to rule out signs of previous bleeding as CSS, suggestive of CAA. According to our results, Amy-PET with quantitative analysis is a noninvasive imaging technique with the potential to provide support for the in vivo diagnosis before the occurrence of overt signs of tissue damage such as hemorrhage, especially in the presence of cognitive decline, when the differential diagnosis versus AD is mandatory. Further studies are needed to validate this method, involving cases with a diagnosis of CAA supported by pathology.
CRediT AUTHOR STATEMENT
Gemma Lombardi (Conceptualization; Funding acquisition; Methodology; Writing –original draft); Valentina Berti (Data curation; Investigation; Methodology; Software; Writing –review & editing; figures and tables preparation); Andrea Ginestroni (Methodology; Resources; Supervision; Writing –review & editing); Benedetta Nacmias (Funding acquisition; Resources; Writing –review & editing); Sandro Sorbi (Validation; Visualization; Writing –review & editing).
ACKNOWLEDGMENTS
The authors have no acknowledgments to report.
FUNDING
Work supported by #NEXTGENERATIONEU (NGEU) and funded by the Ministry of University and Research (MUR), National Recovery and Resilience Plan (NRRP), project MNESYS (PE0000006) –A Multiscale integrated approach to the study of the nervous system in health and disease (DN. 1553 11.10.2022)
CONFLICT OF INTEREST
The authors have no conflict of interest to report.
REFERENCES
[1] | Malhotra K , Theodorou A , Katsanos AH , Zompola C , Shoamanesh A , Boviatsis E , Paraskevas GP , Spilioti M , Cordonnier C , Werring DJ , Alexandrov AV , Tsivgoulis G ((2022) ) Prevalence of clinical andneuroimaging markers in cerebral amyloid angiopathy: A systematic review and meta-analysis. Stroke 53: , 1944–1953. |
[2] | Frisoni GB , Fox NC , Jack CR Jr , Scheltens P , Thompson PM ((2010) ) The clinical use of structural MRI in Alzheimer disease. Nat RevNeurol 6: , 67–77. |
[3] | Scheltens P , Launer LJ , Barkhof F , Weinstein HC , van Gool WA ((1995) ) Visual assessment of medial temporal lobe atrophy on magneticresonance imaging: Interobserver reliability. J Neurol 242: , 557–560. |
[4] | Lehmann M , Koedam EL , Barnes J , Bartlett JW , Ryan NS , Pijnenburg YA , Barkhof F , Wattjes MP , Scheltens P , Fox NC ((2012) ) Posterior cerebralatrophy in the absence of medial temporal lobe atrophy inpathologically confirmed Alzheimer’s disease. Neurobiol Aging 33: , 627e1-627.e12. |
[5] | Planton M , Raposo N , Albucher JF , Pariente J ((2017) ) Cerebral amyloidangiopathy-related cognitive impairment: The search for a specificneuropsychological pattern. Rev Neurol (Paris) 173: , 562–565. |
[6] | Lummel N , Wollenweber FA , Demaerel P , Bochmann K , Malik R , Opherk C , Linn J ((2015) ) Clinical spectrum, underlying etiologies andradiological characteristics of cortical superficial siderosis. J Neurol 262: , 1455–1462. |
[7] | Charidimou A , Boulouis G , Frosch MP , Baron JC , Pasi M , Albucher JF , Banerjee G , Barbato C , Bonneville F , Brandner S , Calviere L , Caparros F , Casolla B , Cordonnier C , Delisle MB , Deramecourt V , Dichgans M , Gokcal E , Herms J , Hernandez-Guillamon M , Jäger HR , Jaunmuktane Z , Linn J , Martinez-Ramirez S , Martínez-Sáez E , Mawrin C , Montaner J , Moulin S , Olivot JM , Piazza F , Puy L , Raposo N , Rodrigues MA , Roeber S , Romero JR , Samarasekera N , Schneider JA , Schreiber S , Schreiber F , Schwall C , Smith C , Szalardy L , Varlet P , Viguier A , Wardlaw JM , Warren A , Wollenweber FA , Zedde M , van Buchem MA , Gurol ME , Viswanathan A , Al-Shahi Salman R , Smith EE , Werring DJ , Greenberg SM ((2022) ) The Boston criteria version 2.0 for cerebralamyloid angiopathy: A multicentre, retrospective, MRI-neuropathologydiagnostic accuracy study. Lancet Neurol 21: , 714–725. |
[8] | Gurol ME , Becker JA , Fotiadis P , Riley G , Schwab K , Johnson KA , Greenberg SM ((2016) ) Florbetapir-PET to diagnose cerebral amyloidangiopathy: A prospective study. Neurology 87: , 2043–2049. |
[9] | Charidimou A , Farid K , Baron JC ((2017) ) Amyloid-PET in sporadiccerebral amyloid angiopathy: A diagnostic accuracy meta-analysis. Neurology 89: , 1490–1498. |
[10] | Farid K , Charidimou A , Baron JC ((2017) ) Amyloid positron emissiontomography in sporadic cerebral amyloid angiopathy: A systematiccritical update. Neuroimage Clin 15: , 247–263. |
[11] | Jack CR Jr , Bennett DA , Blennow K , Carrillo MC , Dunn B , Haeberlein SB , Holtzman DM , Jagust W , Jessen F , Karlawish J , Liu E , Molinuevo JL , Montine T , Phelps C , Rankin KP , Rowe CC , Scheltens P , Siemers E , Snyder HM , Sperling R ;Contributors ((2018) ) NIA-AA ResearchFramework: Toward a biological definition of Alzheimer’s disease. Alzheimers Dement 14: , 535–562. |
[12] | Bergeret S , Queneau M , Rodallec M , Landeau B , Chetelat G , Hong YT , Dumurgier J , Hugon J , Paquet C , Farid K , Baron JC ((2021) ) Brainglucose metabolism in cerebral amyloid angiopathy: An FDG-PET study. Stroke 52: , 1478–1482. |
[13] | Bergeret S , Queneau M , Rodallec M , Curis E , Dumurgier J , Hugon J , Paquet C , Farid K , Baron JC ((2021) ) [18 F]FDG PET may differentiatecerebral amyloid angiopathy from Alzheimer’s disease. Eur JNeurol 28: , 1511–1519. |
[14] | Albert MS , DeKosky ST , Dickson D , Dubois B , Feldman HH , Fox NC , Gamst A , Holtzman DM , Jagust WJ , Petersen RC , Snyder PJ , Carrillo MC , Thies B , Phelps CH ((2011) ) The diagnosis of mild cognitiveimpairment due to Alzheimer’s disease: Recommendations from theNational Institute on Aging-Alzheimer’s Association workgroups ondiagnostic guidelines for Alzheimer’s disease. AlzheimersDement 7: , 270–279. |
[15] | Boulouis G , Charidimou A , Jessel MJ , Xiong L , Roongpiboonsopit D , Fotiadis P , Pasi M , Ayres A , Merrill ME , Schwab KM , Rosand J , Gurol ME , Greenberg SM , Viswanathan A ((2017) ) Small vessel disease burdenin cerebral amyloid angiopathy without symptomatic hemorrhage. Neurology 88: , 878–884. |
[16] | Chan E , Bonifacio GB , Harrison C , Banerjee G , Best JG , Sacks B , Harding N , Del Rocio Hidalgo Mas M , Jäger HR , Cipolotti L , Werring DJ ((2023) ) Domain-specific neuropsychological investigationof CAA with and without intracerebral haemorrhage. J Neurol 270: , 6124–6132. |
[17] | Charidimou A , Linn J , Vernooij MW , Opherk C , Akoudad S , Baron JC , Greenberg SM , Jäger HR , Werring DJ ((2015) ) Cortical superficialsiderosis: Detection and clinical significance in cerebral amyloidangiopathy and related conditions. Brain 138: (Pt 8), 2126–2139. |
[18] | Na HK , Park JH , Kim JH , Kim HJ , Kim ST , Werring DJ , Seo SW , Na DL ((2015) ) Cortical superficial siderosis: A marker of vascular amyloidin patients with cognitive impairment. Neurology 84: , 849–855. |
[19] | Jang H , Chun MY , Kim HJ , Na DL , Seo SW ((2023) ) The effects of imagingmarkers on clinical trajectory in cerebral amyloid angiopathy: Alongitudinal study in a memory clinic. Alzheimers Res Ther 15: , 14. |
[20] | Shoamanesh A , Martinez-Ramirez S , Oliveira-Filho J , Reijmer Y , Falcone GJ , Ayres A , Schwab K , Goldstein JN , Rosand J , Gurol ME , Viswanathan A , Greenberg SM ((2014) ) Interrelationship of superficialsiderosis and microbleeds in cerebral amyloid angiopathy. Neurology 83: , 1838–1843. |
[21] | Mosconi L , McHugh PF ((2011) ) FDG- and amyloid-PET in Alzheimer’sdisease: Is the whole greater than the sum of the parts? Q JNucl Med Mol Imaging 55: , 250–264. |
[22] | Dondi F , Bertoli M , Lucchini S , Cerudelli E , Albano D , Bertagna F ((2022) ) PET imaging for the evaluation of cerebral amyloidangiopathy: A systematic review. Clin Transl Imaging 10: , 391–401. |
[23] | Lombardi G , Pupi A , Bessi V , Polito C , Padiglioni S , Ferrari C , Lucidi G , Berti V , De Cristofaro MT , Piaceri I , Bagnoli S , Nacmias B , Sorbi S ((2020) ) Challenges in Alzheimer’s disease diagnosticwork-up: Amyloid biomarker incongruences. J Alzheimers Dis 77: , 203–217. |
[24] | Greenberg SM , Grabowski T , Gurol ME , Skehan ME , Nandigam RN , Becker JA , Garcia-Alloza M , Prada C , Frosch MP , Rosand J , Viswanathan A , Smith EE , Johnson KA ((2008) ) Detection of isolated cerebrovascularbeta-amyloid with Pittsburgh compound B. Ann Neurol 64: , 587–591. |
[25] | Johnson KA , Gregas M , Becker JA , Kinnecom C , Salat DH , Moran EK , Smith EE , Rosand J , Rentz DM , Klunk WE , Mathis CA , Price JC , Dekosky ST , Fischman AJ , Greenberg SM ((2007) ) Imaging of amyloid burden anddistribution in cerebral amyloid angiopathy. Ann Neurol 62: , 229–234. |
[26] | Chang Y , Liu J , Wang L , Li X , Wang Z , Lin M , Jin W , Zhu M , Xu B ((2021) ) diagnostic utility of integrated 11C-Pittsburgh compound Bpositron emission tomography/magnetic resonance for cerebral amyloidangiopathy: A pilot study. Front Aging Neurosci 13: , 721780. |
[27] | Planton M , Saint-Aubert L , Raposo N , Payoux P , Salabert AS , Albucher JF , Olivot JM , Péran P , Pariente J ((2020) ) Florbetapir regionaldistribution in cerebral amyloid angiopathy and Alzheimer’s disease:A PET study. J Alzheimers Dis 73: , 1607–1614. |
[28] | Charidimou A , Farid K , Tsai HH , Tsai LK , Yen RF , Baron JC ((2018) ) Amyloid-PET burden and regional distribution in cerebral amyloidangiopathy: A systematic review and meta-analysis of biomarkerperformance. J Neurol Neurosurg Psychiatry 89: , 410–417. |
[29] | Szidonya L , Nickerson JP ((2023) ) Cerebral amyloid angiopathy. Radiol Clin North Am 61: , 551–562. |
[30] | Raposo N , Sonnen JA ((2017) ) Amyloid-PET in cerebral amyloidangiopathy: Detecting vascular amyloid deposits, not just blood. Neurology 89: , 1437–1438. |
[31] | Jang H , Jang YK , Kim HJ , Werring DJ , Lee JS , Choe YS , Park S , Lee J , Kim KW , Kim Y , Cho SH , Kim SE , Kim SJ , Charidimou A , Na DL , Seo SW ((2019) ) Clinical significance of amyloid β positivity inpatients with probable cerebral amyloid angiopathy markers. EurJ Nucl Med Mol Imaging 46: , 1287–1298. |