Associations of Serum Liver Function with Cerebral Blood Flow in Patients with Alzheimer’s Disease
Abstract
Background:
Increasing evidence suggests that both amyloid-β metabolism disorders in the liver and cerebral hypoperfusion play an important role in the pathogenesis of Alzheimer’s disease (AD). However, the relevance of liver function alterations to cerebral blood flow (CBF) of patients with AD remains unclear.
Objective:
We aimed to investigate the associations between liver function changes and CBF of patients with AD.
Methods:
We recruited 17 patients with sporadic AD. In addition to physical and neurological examinations, detection of AD biomarkers in cerebrospinal fluid by enzyme-linked immunosorbent assay and CBF assessment by arterial spin labeling sequence of magnetic resonance image scans as well as measure of liver function markers in serum by routine laboratory testing were conducted. Neuropsychological tests were evaluated, including Mini-Mental State Examination and Montreal Cognitive Assessment. Linear and rank correlations were performed to test the associations of liver function alterations with regional CBF of AD.
Results:
We found that liver function markers, especially total protein, the ratio of albumin to globin, globin, alkaline phosphatase, and aspartate aminotransferase were significantly associated with regional CBF of AD patients.
Conclusions:
These findings demonstrated significant associations between perfusion in certain brain regions of AD and alterations of liver function markers, particularly proteins and liver enzymes, which might provide implications to the pathogenesis and treatment of AD.
INTRODUCTION
Alzheimer’s disease (AD) is the most common neurodegenerative disorder which typically manifests with memory, attention, executive, visuospatial, and perceptual impairments. At present, AD is officially listed as the sixth-leading and fifth-leading cause of death in the United States and China respectively, and the prevalence of AD will double by 2030 [1, 2]. Meanwhile, mild cognitive impairment (MCI) is a clinical transition stage between normal aging and dementia. The prevalence of MCI in adults aged 65 years and older is 10% to 20%, and approximately 5%–20% of MCI patients will convert to AD annually [3]. AD has become a great threat to human health [4]. The pathogenesis of AD focuses on some hypotheses including the amyloid-β (Aβ) cascades, hyperphosphorylation of tau protein, neuroinflammation, and so on [5]. Though anti-Aβ monoclonal antibodies including aducanumab and lecanemab had been approved by the Food and Drug Administration (FDA) of the USA [6, 7], it has been giving some expects for the treatment of AD, the long-term effects of aducanumab and lecanemab remain unclear.
However, increasing studies demonstrated that the liver plays essential roles in the pathogenesis of AD by affecting Aβ and lipids metabolism [8–10]. The liver was the major organ responsible for plasma clearance of Aβ40/42 [11–13]. The Aβ degradation capacity of the liver in AD patients decreased significantly due to the reduced expression of Aβ-degrading enzymes, including cathepsin D and insulin-degrading enzyme (IDE), in the liver of AD compared with ordinary people [14]. Withania somnifera could reverse AD pathology by enhancing low-density lipoprotein receptor-related protein (LRP) in the liver [15]. Our previous studies found that the liver weights of APP/PS-1 transgenic (Tg) mice, the animal model for AD, decreased significantly and liver cell necrosis and lymphocyte infiltration increased significantly compared to wild-type mice. Interestingly, parabiotic surgeries with wild-type mice could reverse the decreased liver weights and the increased liver cell necrosis and lymphocyte infiltration of Tg mice [16]. At the same time, lower levels of albumin and elevated aspartate aminotransferase (AST) to alanine aminotransferase (ALT) ratio were correlated with cognitive impairment in the Shenzhen aging-related disorder cohort in China [17]. In the atherosclerosis risk in communities (ARIC) study, a lower plasma level of ALT at mid-life was also associated with a greater long-term risk of dementia [18]. Moreover, in another cohort study, an elevated AST to ALT ratio and lower levels of ALT were not only related to poor cognitive performance and cerebrospinal fluid (CSF) biomarkers including lower CSF Aβ42 levels, higher CSF phosphorylated Tau-181, total-Tau levels, but also to the reduced brain glucose metabolism [19].
Moreover, the reduction of cerebral blood flow (CBF) is regarded as the early characteristic of AD [20, 21]. In healthy young adults, serum liver function markers were found to be associated with brain structure, function, and brain region perfusion by arterial spin labeling (ASL) sequence of magnetic resonance imaging (MRI) scans [22]. In the present study, we aimed to investigate whether there is an association between serum liver function alterations and CBF of AD by ASL technology.
MATERIALS AND METHODS
Participants
We recruited 17 sporadic AD patients from the clinical database in the Cognitive Impairment Ward of the Neurology Department, the Third Affiliated Hospital of Shenzhen University Medical College from 2019 to 2022. This study was approved by the Medical Research Ethics Committee of The Third Affiliated Hospital of Shenzhen University Medical College. All patients with main complaints of memory loss underwent clinical history, physical and neurological examinations, blood laboratory tests, MRI scans, and neuropsychological tests including Mini-Mental State Examination (MMSE) and Montreal Cognitive Assessment (MoCA) and CSF biomarkers of AD. The inclusion criteria for AD patients were: 1) diagnosed as sporadic AD according to the 2011 NIA-AA guidelines [23]; 2) aged between 55–80 years, right-handed, cognitive impairment manifested mainly by memory decline, MMSE≤23; 3) with good quality of ASL brain MRI scanned; 4) without other known brain diseases. Exclusion criteria were: 1) with other dementia including familial AD by gene tested, vascular dementia, dementia with Lewy’s bodies, Parkinson’s disease dementia, progressive supranuclear palsy, multiple system atrophy, mixed dementia, neurosyphilis, and so on; 2) with mild or severe liver diseases including fatty liver, virus hepatitis, hepatocirrhosis and so on; 3) with an acute illness or serious mental disease; 4) abuse of alcohol or psychoactive substances; 5) with additional diseases that may interfere with cognitive evaluation and the changes of liver function. According to the inclusion and exclusion criteria, 17 patients were retained in the current study.
CSF AD biomarkers assessment
A total of 17 patients underwent examination of CSF biomarkers of AD using enzyme-linked immunosorbent assay (ELISA) (OMeng Weiyi Medical Laboratory, Hangzhou, China). The positive results (+) of Aβ+ set to Aβ42 < 550 pg/ml or Aβ42/Aβ40 ≤ 0.1; and T+ as phosphorylated Tau-181 (p-Tau181) protein > 61 pg/ml and of N+ as total Tau (T-Tau) protein >452 pg/ml.
MRI data acquisition and cerebral blood flow analysis
The data of brain MRI from 17 AD patients were collected using 1.5T MRI scanner examination (TR: 4 s; TE: 16.36 ms; slice thickness: 3 mm; spacing: 3.28×3.28 mm; matrix: 64×63) in The Third Affiliated Hospital of Shenzhen University, including T1-weighted (T1W) Magnetization Prepared Rapid Gradient Echo (MPRAGE) sequence with TR = 2200 ms, TE = 2.67 ms, spatial resolution = 0.976×0.976×1 mm3, matrix size = 256×246. At last, 17 AD patients’ ASL MRIs were all ideal and processed and analyzed using Accubrain (Brainnow Medical Technology Ltd). By subtracting the label and control image, it could produce a perfusion-weighted image, which reflected the quantitative perfusion in each voxel. The perfusion image was relative to the CBF image. Meanwhile, the subject’s T1W MRI was segmented to get the automated anatomical atlas (AAL) labels [24]. The AAL labels were transformed to the individual’s CBF image space via a rigid registration. The absolute CBF values and standardized CBF values (normalized by the personal mean CBF value) in different regions, including left and right part of Temporal Cortex, Parietal Cortex, Occipital Cortex, Orbitofrontal Cortex, Frontal Cortex, Sensorimotor Cortex, Precuneus, Gyrus Rectus, Anterior Cingulate, Posterior Cingulate, Thalamus, and Cerebellum were calculated and analyzed.
Detection of serum liver function markers
Serum liver function markers by routine laboratory analyses were performed in 17 patients with AD. The liver function parameters including total protein (TP), albumin (ALB), globin (GLB), total bilirubin (TB), direct bilirubin (DB), indirect bilirubin (IB), alanine transaminase (ALT), aspartate aminotransferase (AST), γ-glutamyl transpeptidase (GGT), and alkaline phosphatase (ALP) were evaluated, and the ratio of albumin to globin (A/G) and the ratio of AST/ALT were calculated.
Statistical analysis
IBM SPSS Statistics 25.0 was used to perform the following statistical analyses. For demographic characteristics, continuous variables were presented as mean±standard deviation (SD), while categorical variables were expressed as ratios (%). Partial correlation analyses were performed to test the associations between serum liver markers (TP, ALB, GLB, A/G, TB, DB, IB, ALT, GGT, ALP, AST, and AST/ALT) and regional cerebral blood flow (rCBF) (bilateral temporal cortex, bilateral medial temporal cortex, bilateral lateral temporal cortex, bilateral parietal cortex, bilateral occipital cortex, bilateral orbitofrontal cortex, bilateral frontal cortex, bilateral sensorimotor cortex, bilateral precuneus gyrus, bilateral gyrus rectus, bilateral anterior cingulate gyrus, bilateral posterior cingulate gyrus, bilateral thalamus, and bilateral cerebellum). Linear correlation was performed when the variables of both groups conformed to a bivariate normal distribution, and rank correlation was performed when at least one of the groups had a skewed distribution or the whole distribution did not conform to a linear trend. The significance threshold was set at two-tailed p < 0.01 to balance the risk of type I and II errors. We did not perform corrections for multiple tests for the study aimed at generating some hypotheses for further study with larger samples.
RESULTS
Correlations between serum liver function markers and regional cerebral blood flow
The demographic information of the included subjects is shown in Table 1. Correlations between serum liver function markers and rCBF are illustrated in Table 2 and Fig. 1a and 1b. For absolute CBF: A/G exhibited a negative correlation with absolute CBF in the left precuneus gyrus (rs = –0.621, p = 0.008), ALP showed a positive correlation with absolute CBF in right thalamus (rs = 0.630, p = 0.007), and TP level was positively correlated with bilateral cerebellum (left and right: r = 0.630, p = 0.007). For standardized CBF: TP exhibited negative correlations with standardized CBF in left temporal cortex (r = –0.617, p = 0.008), left lateral temporal cortex (rs = –0.696, p = 0.002), and left gyrus rectus (r = –0.620, p = 0.008). GLB was negatively correlated with standardized CBF in the left temporal cortex (r = –0.640, p = 0.006), right medial temporal cortex (r = –0.814, p < 0.001), left lateral temporal cortex (rs = –0.664, p = 0.004), right orbitofrontal cortex (rs = –0.615, p = 0.009), bilateral gyrus rectus (left: r = –0.678, p = 0.003, right: rs = –0.664, p = 0.004), and positively in right parietal cortex (r = 0.660, p = 0.004). There were significant positive correlations between A/G and standardized CBF in the right medial temporal cortex (r = 0.765, p < 0.001), right orbitofrontal cortex (r = 0.662, p = 0.004), right gyrus rectus (rs = 0.647, p = 0.005), and negative correlation in the right parietal cortex (r = –0.662, p = 0.004). ALP showed a negative correlation with standardized CBF in the right anterior cingulate gyrus (rs = –0.681, p = 0.003) as well as AST exhibited highly significant negative correlations with standardized CBF in bilateral posterior cingulate gyrus (left: rs = –0.751, p = 0.001, right: rs = –0.800, p < 0.001). Our findings revealed significant associations between perfusion in certain brain regions and liver function markers, particularly proteins and liver enzymes. The overall relationship is presented in Fig. 2.
Fig. 1
Scatter plots of the correlations between serum liver function markers and regional cerebral blood flow of AD patients. (a) Absolute regional cerebral blood flow. (b) Standardized regional cerebral blood flow. CBF, cerebral blood flow; TP, total protein; GLB, globin; A/G, the ratio of albumin to globin; ALP, alkaline phosphatase; AST, aspartate aminotransferase.
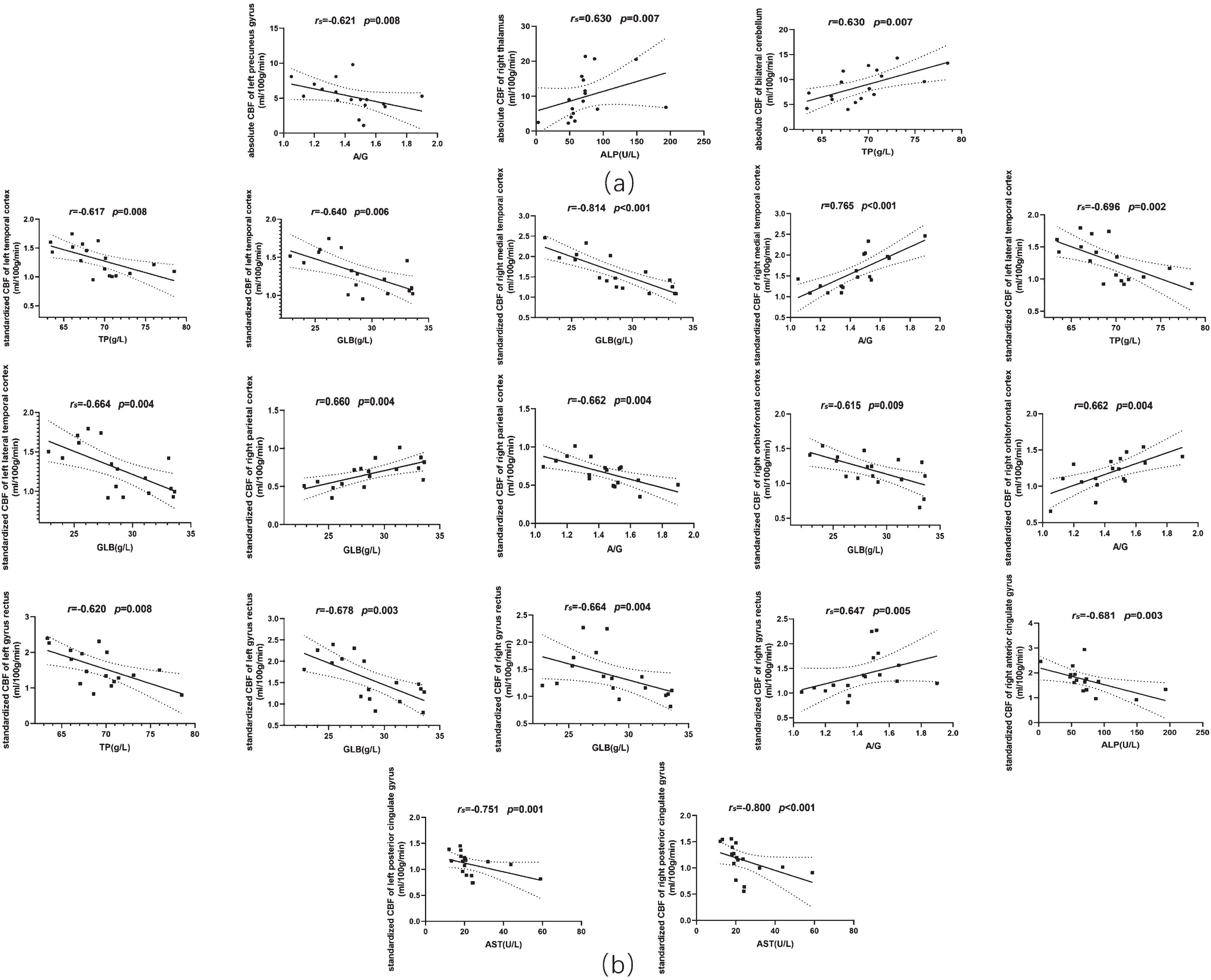
Fig. 2
Correlation heatmap to overview the associations between liver function markers and regional cerebral blood flow. (a) Absolute regional cerebral blood flow. (b) Standardized regional cerebral blood flow. TP, total protein; ALB, albumin; GLB, globin; A/G, the ratio of albumin to globin; ALT, alanine transaminase; GGT, γ-glutamyl transpeptidase; ALP, alkaline phosphatase; AST, aspartate aminotransferase; AST/ALT, the ratio of aspartate aminotransferase to alanine transaminase; LTC, left temporal cortex; RTC, right temporal cortex; LMTC, left medial temporal cortex; RLTC, right medial temporal cortex; LLTC, left lateral temporal cortex; RLTC, right lateral temporal cortex; LPC, left parietal cortex; RPC, right parietal cortex; LOC, left occipital cortex; ROC, right occipital cortex; LOFC, left orbitofrontal cortex; ROFC, right orbitofrontal cortex; LFC, left frontal cortex; RFC, right frontal cortex; LSC, left sensorimotor cortex; RSC, right sensorimotor cortex; LPG, left precuneus gyrus; RPG, right precuneus gyrus; LGR, left gyrus rectus; RGR, right gyrus rectus; LACG, left anterior cingulate gyrus; RACG, right anterior cingulate gyrus; LPCG, left posterior cingulate gyrus; RPCG, right posterior cingulate gyrus; LT, left thalamus; RT, right thalamus; LC, left cerebellum; RC, right cerebellum.
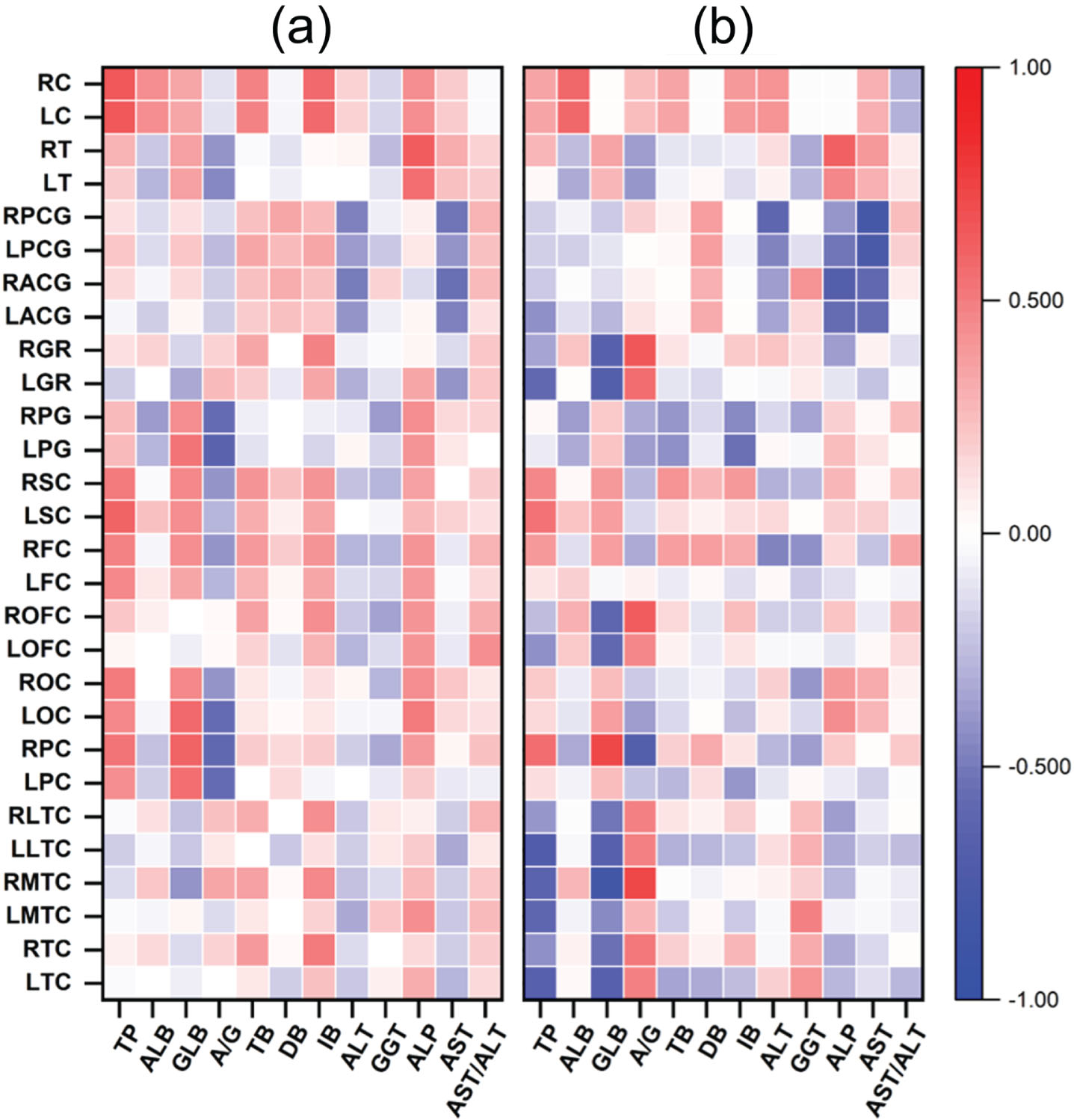
Table 1
Demographic and clinical characteristics of participants
Characteristics | Mean±SD/ratio |
Gender, n (%) | |
Males | 4 (23.5) |
Females | 13 (76.5) |
Age, y | 67.29±8.79 |
Education, y | 10.27±4.43 |
Hypertension, n (%) | 7 (41.2) |
Diabetes mellitus, n (%) | 5 (29.4) |
Hyperlipoidemia, n (%) | 10 (58.8) |
Heart disease, n (%) | 0 (0) |
Family history of dementia, n (%) | 3 (17.6) |
Neuropsychological scores | |
MMSE (/30) | 15.76±7.80 |
MoCA (/30) | 15.64±5.94 |
Table 2
Correlations between alterations of serum liver function markers and regional cerebral blood flow
Encephalic regions | Liver function markers | Pearsons, r | Spearmans, rs | p |
Absolute CBF of AD patients | ||||
Left precuneus gyrus | A/G | –0.621 | 0.008 | |
Right thalamus | ALP | 0.630 | 0.007 | |
Bilateral cerebellum | TP | 0.630 | 0.007 | |
Standardized CBF of AD patients | ||||
Left temporal cortex | TP | –0.617 | 0.008 | |
GLB | –0.640 | 0.006 | ||
Right medial temporal cortex | GLB | –0.814 | <0.001 | |
A/G | 0.765 | <0.001 | ||
Left lateral temporal cortex | TP | –0.696 | 0.002 | |
GLB | –0.664 | 0.004 | ||
Right parietal cortex | GLB | 0.660 | 0.004 | |
A/G | –0.662 | 0.004 | ||
Right orbitofrontal cortex | GLB | –0.615 | 0.009 | |
A/G | 0.662 | 0.004 | ||
Left gyrus rectus | TP | –0.620 | 0.008 | |
GLB | –0.678 | 0.003 | ||
Right gyrus rectus | GLB | –0.664 | 0.004 | |
A/G | 0.647 | 0.005 | ||
Right anterior cingulate gyrus | ALP | –0.681 | 0.003 | |
Left posterior cingulate gyrus | AST | –0.751 | 0.001 | |
Right posterior cingulate gyrus | AST | –0.800 | <0.001 |
All correlations between liver function markers and regional cerebral blood flow listed here satisfied p value < 0.01. TP, total protein; GLB, globin; A/G, the ratio of albumin to globin; ALP, alkaline phosphatase; AST, aspartate aminotransferase.
DISCUSSION
In the present study, we have demonstrated for the first time that there were significant associations between the changes of serum liver function markers and CBF in some brain regions of sporadic AD patients evaluated by the ASL sequence of MRI (Table 2 and Fig. 2). The results were similar to the study of the previous cohort of healthy young adults, in which higher ALB was associated with increased gray matter volume in the parahippocampal gyrus and amygdala. Lower globulin and higher A/G ratio were associated with increased gray matter volume in the olfactory cortex and parahippocampal gyrus. Higher bilirubin was related to the increased regional homogeneity in the precuneus, middle cingulate gyrus, inferior parietal lobule, and supramarginal gyrus, and the decreased regional homogeneity in the caudate nucleus, additionally higher ALT was relevant with increased CBF in the superior frontal gyrus, while declined CBF in the middle occipital gyrus, angular gyrus, precuneus, and middle temporal gyrus [22]. It should be mentioned that there are inconsistent of the correlations between the same serum liver function marker and CBF in some brain regions in the current study, which might be due to the networks of brain and the compensatory mechanisms in AD patients [25, 26].
ASL MRI is an easy and non-invasive MRI technology to assess brain perfusion, therefore, it has been increasingly applied in research of neurodegenerative diseases. Several studies found that hypoperfusion existed in widespread brain regions, particularly in the posterior cortical areas of Parkinson’s disease patients via ASL MRI [27, 28]. Some studies have demonstrated hypo- and hyper-perfusion of specific brain regions in MCI and AD assessed by ASL MRI [29–31]. However, the mechanisms behind the associations in AD remain unclear. It has been reported that the liver may affect brain function through a complicated regulatory pathway, namely liver-to-brain axis regulation [9]. For example, stroke prognosis was negatively associated with elevated serum bilirubin, a direct agonist of the transient receptor potential melastatin channel to exacerbate ischemic brain damage [32]. Physical exercise not only counteracts environmental stress by hepatic biosynthesis of RNA N(6)-methyladenosine [33], and also could increase plasma concentrations of glycosylphosphatidylinositol (GPI)-specific phospholipase D1 (Gpld1), a GPI-degrading enzyme derived from liver, to ameliorate age-related cognitive impairments [34]. It had more predictive value for AD diagnosis employing the AD polygenic risk score in the liver (even without APOE) than in the heart, lung, kidney, and muscle etc. [35]. The liver was the earliest affected organ in APP/PS1 mice during amyloid pathology progression, followed by the kidney and heart [36].
Increasing studies demonstrated that alterations in liver function markers were associated with AD. ALT and AST levels decreased significantly in AD patients and had significant positive associations with cognitive performance. The ALP level and AST to ALT ratio were enhanced in AD patients markedly, and negatively associated with cognitive performance especially in male patients [18, 19, 37–39]. Moreover, another previous study found that GGT, a marker of oxidative stress, increased significantly in AD patients [40]. Plasma liver-type fatty acid-binding protein significantly elevated in AD patients and was associated with the CSF levels of Aβ, p-Tau181, and T-Tau of AD patients [41]. Albumin oxidation in CSF and plasma was increased in AD patients [42]. Higher indirect bilirubin (IBIL) concentrations, lower albumin concentrations, and a higher ratio of IBIL to albumin (IBIL/ALB) were observed in dementia patients including AD, dementia with Lewy’s bodies, and general paresis of insane [43]. Intravenous albumin infusion and plasma exchange with albumin replacement could significantly improve the cognitive function of AD and slow cognitive and functional decline [43, 44]. Though serum total bilirubin is not causally associated with AD assessed by a large-scale genome-wide association study, increased serum total bilirubin level in AD was regarded as a biomarker of AD severity [45].
However, our study has several limitations, which should be considered when interpreting the results. First, the sample size is a little small and there is a lack of age-matched healthy control subjects. Second, we did not study the mechanism of the association between changes in liver function markers and CBF of AD. Third, this study could not demonstrate whether the association between changes in serum liver function and regional CBF of AD is unique to AD. Therefore, it is very valuable to study the association between the changes in serum liver function markers and regional CBF of AD in the future. Fourth, 1.5T MRI scanners have certain technical limitations for ASL imaging including lower signal-to-noise ratio, sensitivity to labeling efficiency, lower spatial resolution, higher B0 and B1 in homogeneity, and higher vulnerability to magnetic susceptibility artifacts.
In conclusion, our results evidenced a clear correlation between perfusion in certain brain regions of AD and the alteration of serum liver function markers, especially proteins and liver enzymes. However, the mechanisms behind the associations are still unclear. Moreover, without no healthy subjects as a control group, it could not be certain whether similar relationships could be found in healthy people. Although this study has many shortcomings, it may provide a basis for exploring further the role of the liver in the pathogenesis of AD and finding a novel target for treatment in AD.
AUTHOR CONTRIBUTIONS
Feiqi Zhu (Conceptualization; Methodology; Formal analysis; Visualization; Investigation; Writing – Original Draft; Writing – Review & Editing); Hao Wang (Formal analysis; Investigation; Writing – Original Draft; Writing – Review & Editing); Lin Shi (Formal analysis); Yishan Luo (Formal analysis); Shimei Luo (Investigation); Taikun Lu (Formal analysis); Kangding Liu (Formal analysis); Chunyan Xu (Investigation); Guozhen Qiu (Investigation); Qiwen Guo (Investigation); Chunchun Chen (Investigation).
ACKNOWLEDGMENTS
We would like to thank the Sanming Project of Medicine in Shenzhen and the Key Project of the Shenzhen Science and Technology Commission for supporting this work.
FUNDING
This work was supported by the Sanming Project of Medicine in Shenzhen (SZSM201801014) and the Key Project of the Shenzhen Science and Technology Commission (JCYJ20200109143431341).
CONFLICT OF INTEREST
The authors have no conflict of interest to report.
DATA AVAILABILITY
The raw data supporting the findings and conclusions of this article will be made available by the authors, without any reservations and restrictions.
REFERENCES
[1] | Ren R , Qi J , Lin S , Liu X , Yin P , Wang Z , Tang R , Wang J , Huang Q , Li J , Xie X , Hu Y , Cui S , Zhu Y , Yu X , Wang P , Zhu Y , Wang Y , Huang Y , Hu Y , Wang Y , Li C , Zhou M , Wang G ((2022) ) The China Alzheimer Report 2022. Gen Psychiatr 35: , e100751. |
[2] | ((2023) ) 2023 Alzheimer’s disease facts and figures. Alzheimers Dement 19: , 1598–1695. |
[3] | Langa KM , Levine DA ((2014) ) The diagnosis and management of mild cognitive impairment: A clinical review. JAMA 312: , 2551–2561. |
[4] | Jia J , Wei C , Chen S , Li F , Tang Y , Qin W , Zhao L , Jin H , Xu H , Wang F , Zhou A , Zuo X , Wu L , Han Y , Han Y , Huang L , Wang Q , Li D , Chu C , Shi L , Gong M , Du Y , Zhang J , Zhang J , Zhou C , Lv J , Lv Y , Xie H , Ji Y , Li F , Yu E , Luo B , Wang Y , Yang S , Qu Q , Guo Q , Liang F , Zhang J , Tan L , Shen L , Zhang K , Zhang J , Peng D , Tang M , Lv P , Fang B , Chu L , Jia L , Gauthier S ((2018) ) The cost of Alzheimer’s disease in China and re-estimation of costs worldwide. Alzheimers Dement 14: , 483–491. |
[5] | Long JM , Holtzman DM ((2019) ) Alzheimer disease: An update on pathobiology and treatment strategies. Cell 179: , 312–339. |
[6] | Dhillon S ((2021) ) Aducanumab: First approval. Drugs 81: , 1437–1443. |
[7] | van Dyck CH , Swanson CJ , Aisen P , Bateman RJ , Chen C , Gee M , Kanekiyo M , Li D , Reyderman L , Cohen S , Froelich L , Katayama S , Sabbagh M , Vellas B , Watson D , Dhadda S , Irizarry M , Kramer LD , Iwatsubo T ((2023) ) Lecanemab in early Alzheimer’s disease. N Engl J Med 388: , 9–21. |
[8] | Bassendine MF , Taylor-Robinson SD , Fertleman M , Khan M , Neely D ((2020) ) Is Alzheimer’s disease a liver disease of the brain? J Alzheimers Dis 75: , 1–14. |
[9] | Huang Z , Lin HWK , Zhang Q , Zong X ((2022) ) Targeting Alzheimer’s disease: The critical crosstalk between the liver and brain. Nutrients 14: , 4298. |
[10] | Jakhmola-Mani R , Islam A , Katare DP ((2021) ) Liver-brain axis in sporadic Alzheimer’s disease: Role of ten signature genes in a mouse model. CNS Neurol Disord Drug Targets 20: , 871–885. |
[11] | Ghiso J , Shayo M , Calero M , Ng D , Tomidokoro Y , Gandy S , Rostagno A , Frangione B ((2004) ) Systemic catabolism of Alzheimer’s Abeta40 and Abeta42. J Biol Chem 279: , 45897–45908. |
[12] | Xiang Y , Bu XL , Liu YH , Zhu C , Shen LL , Jiao SS , Zhu XY , Giunta B , Tan J , Song WH , Zhou HD , Zhou XF , Wang YJ ((2015) ) Physiological amyloid-beta clearance in the periphery and its therapeutic potential for Alzheimer’s disease. Acta Neuropathol 130: , 487–499. |
[13] | Cheng Y , He CY , Tian DY , Chen SH , Ren JR , Sun HL , Xu MY , Tan CR , Fan DY , Jian JM , Sun PY , Zeng GH , Shen YY , Shi AY , Jin WS , Bu XL , Liu HM , Xu YM , Wang J , Wang YJ ((2023) ) Physiological β-amyloid clearance by the liver and its therapeutic potential for Alzheimer’s disease. Acta Neuropathol 145: , 717–731. |
[14] | Maarouf CL , Walker JE , Sue LI , Dugger BN , Beach TG , Serrano GE ((2018) ) Impaired hepatic amyloid-beta degradation in Alzheimer’s disease. PLoS One 13: , e0203659. |
[15] | Sehgal N , Gupta A , Valli RK , Joshi SD , Mills JT , Hamel E , Khanna P , Jain SC , Thakur SS , Ravindranath V ((2012) ) Withania somnifera reverses Alzheimer’s disease pathology by enhancing low-density lipoprotein receptor-related protein in liver. Proc Natl Acad Sci U S A 109: , 3510–3515. |
[16] | Wu K , Li C , Liu K , Zhu F ((2022) ) The effects of high plasma levels of Aβ42 on the liver of Alzheimer’s disease mice model. China J Alzheimers Dis Relat Disord 5: , 38–41. |
[17] | Wu K , Xu C , Qiu G , Guo Q , Chen C , Liu W , Liu J , Liu K , Zhu F ((2022) ) Association of lower liver function with cognitive impairment in the Shenzhen ageing-related disorder cohort in China. Front Aging Neurosci 14: , 1012219. |
[18] | Lu Y , Pike JR , Selvin E , Mosley T , Palta P , Sharrett AR , Thomas A , Loehr L , Sidney Barritt A , Hoogeveen RC , Heiss G ((2021) ) Low liver enzymes and risk of dementia: The Atherosclerosis Risk in Communities (ARIC) Study. J Alzheimers Dis 79: , 1775–1784. |
[19] | Nho K , Kueider-Paisley A , Ahmad S , MahmoudianDehkordi S , Arnold M , Risacher SL , Louie G , Blach C , Baillie R , Han X , Kastenmüller G , Trojanowski JQ , Shaw LM , Weiner MW , Doraiswamy PM , van Duijn C , Saykin AJ , Kaddurah-Daouk R ((2019) ) Association of altered liver enzymes with Alzheimer disease diagnosis, cognition, neuroimaging measures, and cerebrospinal fluid biomarkers. JAMA Netw Open 2: , e197978. |
[20] | Nortley R , Korte N , Izquierdo P , Hirunpattarasilp C , Mishra A , Jaunmuktane Z , Kyrargyri V , Pfeiffer T , Khennouf L , Madry C , Gong H , Richard-Loendt A , Huang W , Saito T , Saido TC , Brandner S , Sethi H , Attwell D ((2019) ) Amyloid β oligomers constrict human capillaries in Alzheimer’s disease via signaling to pericytes. Science 365: , eaav9518. |
[21] | Korte N , Nortley R , Attwell D ((2020) ) Cerebral blood flow decrease as an early pathological mechanism in Alzheimer’s disease. Acta Neuropathol 140: , 793–810. |
[22] | Chen J , Liu S , Wang C , Zhang C , Cai H , Zhang M , Si L , Zhang S , Xu Y , Zhu J , Yu Y ((2021) ) Associations of serum liver function markers with brain structure, function, and perfusion in healthy young adults. Front Neurol 12: , 606094. |
[23] | Jack CR Jr. , Bennett DA , Blennow K , Carrillo MC , Dunn B , Haeberlein SB , Holtzman DM , Jagust W , Jessen F , Karlawish J , Liu E , Molinuevo JL , Montine T , Phelps C , Rankin KP , Rowe CC , Scheltens P , Siemers E , Snyder HM , Sperling R ((2018) ) NIA-AA Research Framework: Toward a biological definition of Alzheimer’s disease. Alzheimers Dement 14: , 535–562. |
[24] | Tzourio-Mazoyer N , Landeau B , Papathanassiou D , Crivello F , Etard O , Delcroix N , Mazoyer B , Joliot M ((2002) ) Automated anatomical labeling of activations in SPM using a macroscopic anatomical parcellation of the MNI MRI single-subject brain. Neuroimage 15: , 273–289. |
[25] | Clément F , Belleville S ((2010) ) Compensation and disease severity on the memory-related activations in mild cognitive impairment. Biol Psychiatry 68: , 894–902. |
[26] | Ding B , Ling HW , Zhang Y , Huang J , Zhang H , Wang T , Yan FH ((2014) ) Pattern of cerebral hyperperfusion in Alzheimer’s disease and amnestic mild cognitive impairment using voxel-based analysis of 3D arterial spin-labeling imaging: Initial experience. Clin Interv Aging 9: , 493–500. |
[27] | Syrimi ZJ , Vojtisek L , Eliasova I , Viskova J , Svatkova A , Vanicek J , Rektorova I ((2017) ) Arterial spin labelling detects posterior cortical hypoperfusion in non-demented patients with Parkinson’s disease. J Neural Transm (Vienna) 124: , 551–557. |
[28] | Melzer TR , Watts R , MacAskill MR , Pearson JF , Rüeger S , Pitcher TL , Livingston L , Graham C , Keenan R , Shankaranarayanan A , Alsop DC , Dalrymple-Alford JC , Anderson TJ ((2011) ) Arterial spin labelling reveals an abnormal cerebral perfusion pattern in Parkinson’s disease. Brain 134: , 845–855. |
[29] | Wierenga CE , Hays CC , Zlatar ZZ ((2014) ) Cerebral blood flow measured by arterial spin labeling MRI as a preclinical marker of Alzheimer’s disease. J Alzheimers Dis 42 Suppl 4: , S411–419. |
[30] | Camargo A , Wang Z ((2021) ) Longitudinal cerebral blood flow changes in normal aging and the Alzheimer’s disease continuum identified by arterial spin labeling MRI. J Alzheimers Dis 81: , 1727–1735. |
[31] | Camargo A , Wang Z ((2023) ) Hypo- and hyper-perfusion in MCI and AD identified by different ASL MRI sequences. Brain Imaging Behav 17: , 306–319. |
[32] | Liu HW , Gong LN , Lai K , Yu XF , Liu ZQ , Li MX , Yin XL , Liang M , Shi HS , Jiang LH , Yang W , Shi HB , Wang LY , Yin SK ((2023) ) Bilirubin gates the TRPM2 channel as a direct agonist to exacerbate ischemic brain damage. Neuron 111: , 1609–1625.e1606. |
[33] | Yan L , Wei JA , Yang F , Wang M , Wang S , Cheng T , Liu X , Jia Y , So KF , Zhang L ((2022) ) Physical exercise prevented stress-induced anxiety via improving brain RNA methylation. Adv Sci (Weinh) 9: , e2105731. |
[34] | Horowitz AM , Fan X , Bieri G , Smith LK , Sanchez-Diaz CI , Schroer AB , Gontier G , Casaletto KB , Kramer JH , Williams KE , Villeda SA ((2020) ) Blood factors transfer beneficial effects of exercise on neurogenesis and cognition to the aged brain. Science 369: , 167–173. |
[35] | Panyard DJ , Deming YK , Darst BF , Van Hulle CA , Zetterberg H , Blennow K , Kollmorgen G , Suridjan I , Carlsson CM , Johnson SC , Asthana S , Engelman CD , Lu Q ((2023) ) Liver-specific polygenic risk score is associated with Alzheimer’s disease diagnosis. J Alzheimers Dis 92: , 395–409. |
[36] | Zheng H , Cai A , Shu Q , Niu Y , Xu P , Li C , Lin L , Gao H ((2019) ) Tissue-specific metabolomics analysis identifies the liver as a major organ of metabolic disorders in amyloid precursor protein/presenilin 1 mice of Alzheimer’s disease. J Proteome Res 18: , 1218–1227. |
[37] | Han SW , Park YH , Jang ES , Nho K , Kim S ((2022) ) Implications of liver enzymes in the pathogenesis of Alzheimer’s disease. J Alzheimers Dis 88: , 1371–1376. |
[38] | Elinav E , Ackerman Z , Maaravi Y , Ben-Dov IZ , Ein-Mor E , Stessman J ((2006) ) Low alanine aminotransferase activity in older people is associated with greater long-term mortality. J Am Geriatr Soc 54: , 1719–1724. |
[39] | Ferri E , Rossi PD , Scichilone M , Lucchi TA , Arosio B ((2022) ) Liver enzymes in a cohort of community-dwelling older persons: Focus on sex contribution. Nutrients 14: , 4973. |
[40] | Yavuz BB , Yavuz B , Halil M , Cankurtaran M , Ulger Z , Cankurtaran ES , Aytemir K , Ariogul S ((2008) ) Serum elevated gamma glutamyltransferase levels may be a marker for oxidative stress in Alzheimer’s disease. Int Psychogeriatr 20: , 815–823. |
[41] | Cheng Y , Jian JM , He CY , Ren JR , Xu MY , Jin WS , Tan CR , Zeng GH , Shen YY , Chen DW , Li HY , Yi X , Zhang Y , Zeng F , Wang YJ ((2022) ) The correlations of plasma liver-type fatty acid-binding protein with amyloid-β and tau levels in patients with Alzheimer’s disease. J Alzheimers Dis 88: , 375–383. |
[42] | Costa M , Horrillo R , Ortiz AM , Pérez A , Mestre A , Ruiz A , Boada M , Grancha S ((2018) ) Increased albumin oxidation in cerebrospinal fluid and plasma from Alzheimer’s disease patients. J Alzheimers Dis 63: , 1395–1404. |
[43] | Zhong X , Liao Y , Chen X , Mai N , Ouyang C , Chen B , Zhang M , Peng Q , Liang W , Zhang W , Wu Z , Huang X , Li C , Chen H , Lao W , Zhang CE , Wang X , Ning Y , Liu J ((2020) ) Abnormal serum bilirubin/albumin concentrations in dementia patients with Aβ deposition and the benefit of intravenous albumin infusion for Alzheimer’s disease treatment. Front Neurosci 14: , 859. |
[44] | Boada M , López OL , Olazarán J , Núñez L , Pfeffer M , Paricio M , Lorites J , Piñol-Ripoll G , Gámez JE , Anaya F , Kiprov D , Lima J , Grifols C , Torres M , Costa M , Bozzo J , Szczepiorkowski ZM , Hendrix S , Páez A ((2020) ) A randomized, controlled clinical trial of plasma exchange with albumin replacement for Alzheimer’s disease: Primary results of the AMBAR Study. Alzheimers Dement 16: , 1412–1425. |
[45] | Wang H , Wu S , Wang L , Gou X , Guo X , Liu Z , Li P ((2022) ) Association between serum total bilirubin and Alzheimer’s disease: A bidirectional Mendelian randomization study. Arch Gerontol Geriatr 103: , 104786. |