Global Research Trends and Hotspots for Ferroptosis, Necroptosis, and Pyroptosis in Alzheimer’s Disease from the Past to 2023: A Combined Bibliometric Review
Abstract
Background:
Alzheimer’s disease (AD) is a genetically intricate neurodegenerative disorder. Studies on “Ferroptosis in AD”, “Pyroptosis in AD”, and “Necroptosis in AD” are becoming more prevalent and there is increasing evidence that they are closely related to AD. However, there has not yet been a thorough bibliometrics-based investigation on this subject.
Objective:
This study uses a bibliometric approach to visualize and analyze the literature within the field of three distinct types of cell death in AD and explores the current research hotspots and prospective research directions.
Methods:
We collected relevant articles from the Web of Science and used CiteSpace, VOS viewer, and Pajek to perform a visual analysis.
Results:
A total of 123, 95, and 84 articles were published in “Ferroptosis in AD”, “Pyroptosis in AD”, and “Necroptosis in AD”, respectively. Based on keywords analysis, we can observe that “oxidative stress” and “lipid peroxidation”, “cell death” and “activation”, and “Nlrp3 inflammasome” and “activation” were the three most prominent words in the field of “Ferroptosis in AD”, “Pyroptosis in AD”, and “Necroptosis in AD”, respectively. Focusing on the breakout words in the keyword analysis, we reviewed the mechanisms of ferroptosis, pyroptosis, and necroptosis in AD. By mapping the time zones of the keywords, we speculated on the evolutionary trends of ferroptosis, pyrotosis, and necroptosis in AD.
Conclusions:
Our findings can help researchers grasp the research status of three types of cell death in AD and determine new directions for future research as soon as possible.
INTRODUCTION
Alzheimer’s disease (AD) is the most prevalent complex irreversible neurological disorder. Originally described by the German Alois Alzheimer in 1907 [1], AD now affects one in ten adults over the age of 65, and its prevalence increases with age [2]. AD is mainly characterized by the formation of amyloid-β (Aβ) plaque deposition in senile plaques and intracellular neurofibrillary tangles (NFTs) formed by hyperphosphorylation of tau protein, and it is primarily explained by the amyloid theory, tau theory, and nerve and blood vessel theory [3, 4]. Amyloid-targeted therapy is the main treatment option for AD [5], but as amyloid is challenged as a therapeutic target, researchers are increasingly interested in other neurochemical changes in AD [6]. Recently, more and more studies have focus on “Ferroptosis in AD”, “Pyroptosis in AD”, and “Necroptosis in AD”, and the results revealed that those three distinct types of cell death are closely related to AD.
Ferroptosis is a morphologically, biochemically, and genetically distinct form of cell death from apoptosis and various forms of necrosis [7]. Ferroptosis is characterized by excessive accumulation of iron that induces the Fenton reaction, leading to a significant increase in reactive oxygen species (ROS) and lipid accumulation to lethal levels, inducing ferroptosis [8, 9]. Ferroptosis cells can be distinguished from other types of cell death by several changes in cell morphology, metabolism, and protein expression. For example, ferroptotic cells are characterized by reduced mitochondrial volume and increased membrane density, with normal-sized nuclei and chromatin condensation not present [10]. Multiple hallmarks of ferroptosis have been identified in AD, including increased accumulation of iron, elevated levels of ROS and lipid peroxides, and depleted levels of reduced glutathione and glutathione peroxidase 4 (GPX4) activity. Numerous studies have demonstrated that iron overload initiates a cascade of events, such as glial activation, and triggering the formation of Aβ plaques and tau tangles. These events subsequently promote plaque deposition and the formation of NFTs in the elderly, ultimately driving disease progression and accelerating cognitive decline [11, 12].
Pyroptosis is a type of programmed cell death characterized by necrosis and inflammation, which is triggered by inflammatory caspases [13]. Pyroptosis, as a biological process, is known for its ability to release cytoplasmic contents from dying host cells. This release of contents serves as a potent molecular signal, initiating inflammatory cascades that aid in the elimination of pathogens within the host. The process is as follows: the activation of inflammatory caspases specifically cleaves gasdermin D at the interdomain loop, thereby releasing the N-terminal pore-forming domain [14], cell membrane pores are formed, permeability increases, cells swell, capsules rupture, and eventually the contents of the cell are released, which activates a stronger inflammatory response [15]. However, it is important to note that excessive activation of pyroptosis can intensify the inflammatory response, thereby implying its involvement in the pathology of various diseases [16]. Another study pointed out that high levels of Interleukin-1 β (IL-1 β) induce tau hyperphosphorylation and aggravate AD pathogenesis [17]. Although, as a necrotizing, inflammatory type of programmed cell death induced by caspases, pyroptosis has been recognized for many years, the discovery of pyroptotic executive cells has attracted academic attention because of its role in triggering caspase-mediated cell death [18]. To initiate inflammation and defense, inflammasomes such as Nlrp3, Nlrp1b, Nlrc4, the HIN-200 family member AIM2, and the tripartite motif (TRIM) family member pyrin/TRIM20 meets and relies on caspase-1 activation to release mature IL-1 β and IL-18 and induce pyroptosis. Studies have proven that pyroptosis is relevant to the pathological mechanism of AD [19]. The inflammasome and proinflammatory cytokines in the pyroptosis activation pathway also have been proven to be related to the pathogenesis of AD, and the AD markers Aβ and tau proteins have been shown to be involved in the occurrence of pyroptosis [19].
Necroptosis is characterized by both apoptosis and necrosis. Receptor-interacting protein kinases (RIPKs) are critical mediators of necroptosis, among them the activation of three crucial proteins (RIPK1, RIPK3, and MLKL) in the brain of patients with AD is significantly increased [20, 21]. When cells become damaged by chemical, mechanical, or inflammatory stress, they undergo necrosis [22]. It is characterized by cell organelles and the cell itself swelling, which leads to cell rupture and damage to extracellular space [23]. Necroptosis is carried out by the effector proteins RIPK1 and RIPK3 and the executioner protein MLKL. When caspase-8 was inhibited, the necrotizing apoptotic cell death pathway was activated. A phosphorylated RIPK1 recruits and phosphorylates MLKL, while an oligomerized MLKL translocates to the plasma membrane, where it interacts with phospholipids to produce MIKL pores, leading to cell lysis, eventually leading to necrotic apoptosis [24]. Overall, necroptosis in the presence of inhibition of Caspase-8 activity responds to multiple signaling pathway activation, such as death receptors (TNFR, Fas, TRAIL-R) and pattern recognition receptors (such as TLR3 and TLR4) and IFN signaling [25].
Bibliometric analysis is a technique for reviewing and analyzing studies in a specific field of study during a specific period in both a qualitative and quantitative manner [26]. This approach concentrates on nations, organizations, publications, authors, and keywords, which are connected to the study in a certain topic. Additionally, it conducts performance analyses on authors, institutions, and countries, which provides readers with an objective view of the trends and boundaries in this area [27, 28]. Therefore, this study was conducted to point out evolutionary tendencies and emerging hotspots of “Ferroptosis in AD”, “Pyroptosis in AD”, and “Necroptosis in AD” as well as to offer direction for future research and scientific decision-making.
MATERIALS AND METHODS
Data sources and search strategies
The study’s chosen data source was the Web of Science, and to guarantee the completeness and accuracy of the data that was retrieved, the “citation index” was set to SCI-EXPANDED. It should be noted that the Web of Science has been regarded as the best database for bibliometric analysis and has been approved by most scholars as a high-quality digital literature resource database. Therefore, we conducted a literature search on the Web of Science Core Collection (WoSCC) database. Figure 1 depicts the retrieval procedure in detail and all retrieved records were downloaded in “plain text” format. We restricted the literature category to “article or review”, the language type to “English”, and set #1 Free words for Alzheimer’s disease (Supplementary Material), #2 for TS = Oxytosis OR TS = ferroptotic OR TS = ferroptosis, #3 for TS = pyroptotic OR TS = pyroptosome OR TS = pyroptosis, and #4 for TS = Necroptosis OR TS = necroptotic. Then we searched 3 times, respectively #1 and #2, #1 and #3, and #1 and #4. Finally, we retrieved 129 articles and 115 reviews from searches #1 and #2, 95 articles and 66 reviews from searches #1 and #3, and 96 articles and 51 reviews from searches #1 and #4. The next step we read the titles, abstracts, and even full texts of the papers to eliminate those unrelated to our subject. Finally, we use VOS viewer, CiteSpace, Pajek and other visualization software for visualization analysis. Then we extracted and analyzed data from the visualization to obtain relevant information results such as “Countries, Institutions and Journals”, “Cited and Co-cited Authors”, “Cited and Co-cited references”, and “Keywords”.
Fig. 1
Diagram of literature retrieval processes.
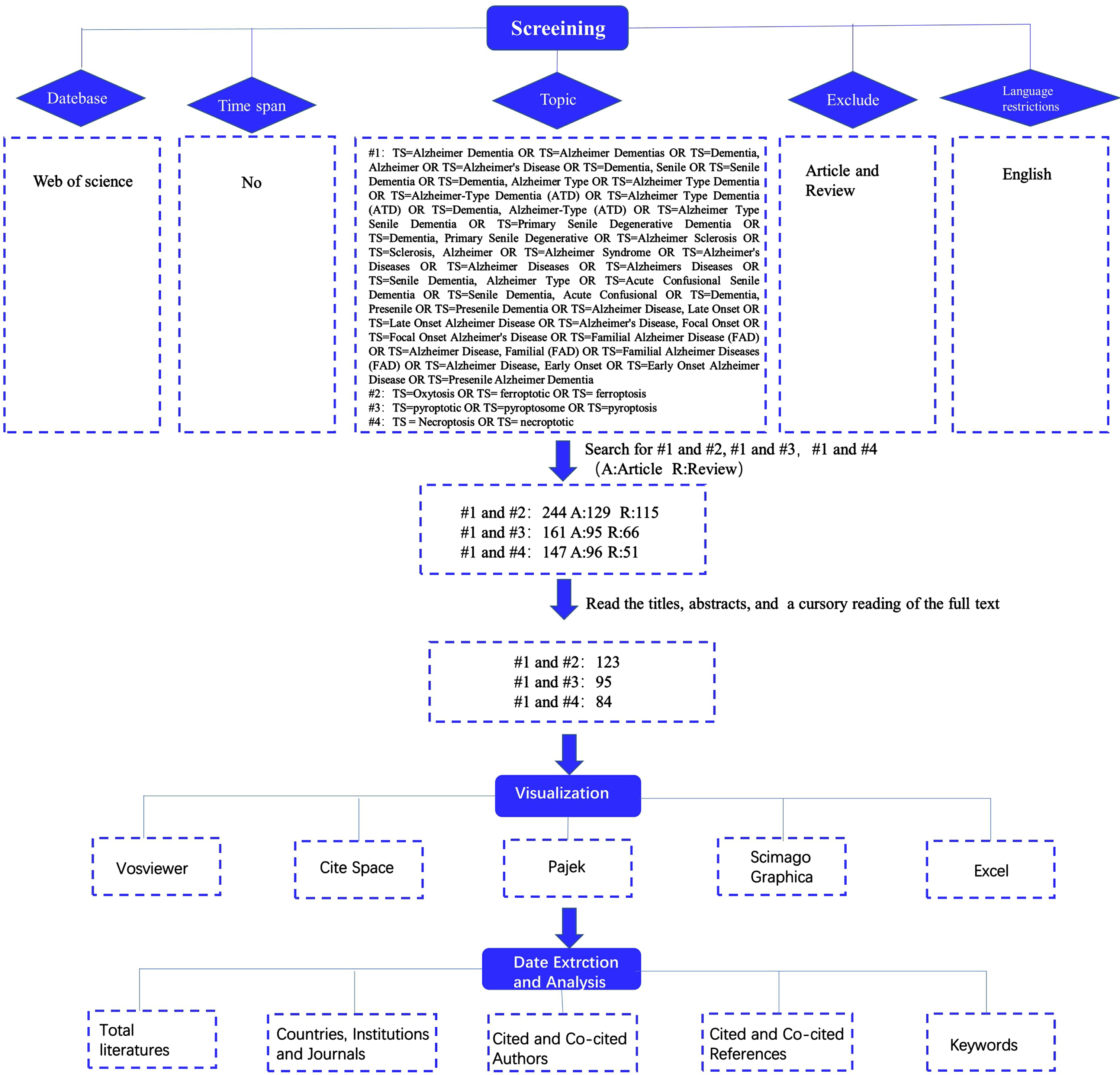
Statistical analysis
In this research, Microsoft Office Excel, VOS viewer (version 1.6.19), CiteSpace (version 6.2.R3), Pajek, and Scimago Graphica were used for visual analysis.
CiteSpace is a Java-based citation analysis application for scientometrics and data/information visualization. It is utilized for illustrating the distribution, patterns, and relationships of scientific knowledge [29]. It can be used to create author, literary, and other visual networks, compute betweenness centrality, perform burst detection, and identify changes in present research hotspots and developing trends [30]. It was applied to research cited articles and keywords in this study.
VOS viewer, a free computer program for constructing and viewing visual bibliometric charts based on web data, can comprehend scientific research’s dynamic evolution and structure systematically [31]. Pajek is a sophisticated tool for investigating the numerous complex nonlinear networks that are currently in existence. It is a huge complex network analysis tool. In our study, we use it to assist the VOS viewer in forming a more beautiful and clear visualization.
RESULTS
Annual publications and trends
The volume of publications over each period reflects the direction of the research in that area. According to our search strategy (Fig. 1), there were 125, 95, and 84 studies on “Ferroptosis in AD”, “Pyroptosis in AD”, and “Necroptosis in AD”, respectively, and the number of publications in these three fields increased year by year (Supplementary Figure 1). It is evident that attention in this area is gradually growing, which may be due to the aging of the global population, rising economic status, and advancements in research and technology. We can see from the figure that the slope of the annual cumulative publication volume of “Ferroptosis in AD”, “Pyroptosis in AD”, and “Necroptosis in AD” in 2021–2022 is the largest, that is the total number of papers in this period is significantly higher than that in other periods.
Analysis of authors and co-cited authors
A total of 649, 897, and 513 authors contributed to publications on “Ferroptosis in AD”, “Pyroptosis in AD”, and “Necroptosis in AD”, respectively. The top 10 authors for publications in the 3 fields are listed in Supplementary Table 1. Network visualization can show that each cluster provides information about the research field’s representative scientists and core strengths. In this context, author collaborations can be used to spot research cliques, and author analysis can reveal how researchers vary and agree on particular study topics. In our visualization of Fig. 2, we can see the visualization of authors related to “Ferroptosis in AD”, “Pyroptosis in AD”, and “Necroptosis in AD”, respectively. The size of the node represents the active degree of the author, and the shorter the distance of the node connection, the stronger the relationship and the closer the cooperation. We can see that some of the authors do not know one another, but some authors work closely together. As these three fields are emerging, there is not much difference in the number of articles that the authors have published, but we can observe that “Yuan, Junying”, “Lei, Peng”, and “Gordon, Richard” had the highest average citations in “Ferroptosis in AD”, “Pyroptosis in AD”, and “Necroptosis in AD”, respectively, indicating their influence is bigger in these three areas.
Fig. 2
Visualization of authors related to “Ferroptosis in AD” (A), “Pyroptosis in AD” (B), and “Necroptosis in AD” (C).
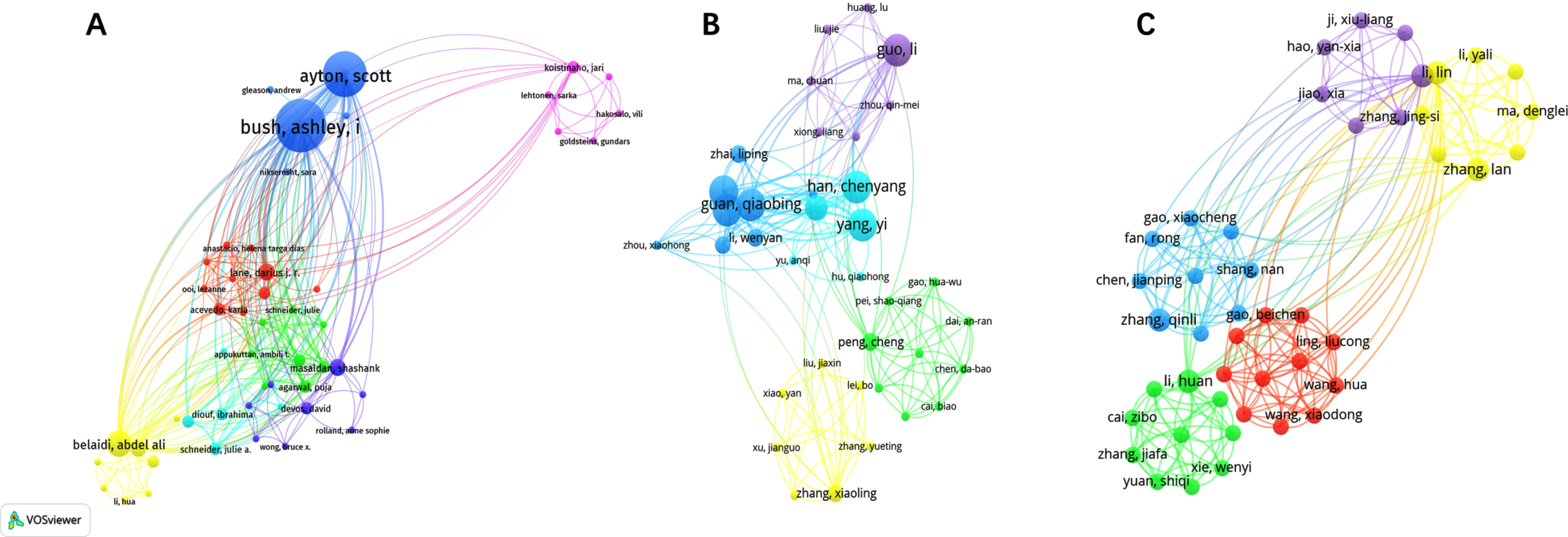
Analysis of cited and co-cited references
Supplementary Table 2 shows the top 10 cited references as shown in the figure. The most cited article in the field of “Ferroptosis in AD” is “Ablation of ferroptosis regulator GPX4 in forebrain neurons promotes cognitive impairment and neurodegeneration”, which indicates that forebrain neurons are vulnerable to ferroptosis, suggesting that AD may involve ferroptosis as a neurodegenerative process, giving us hope for treating neurodegenerative diseases such as AD [32]. The most cited article in the field of “Pyroptosis in AD” is “NLRP3 inflammasome activation drives tau pathology”. This shows how NLRP3 inflammasome activation causes tau pathology and supports the amyloid cascade hypothesis in AD [33]. The article with the most citations in the discipline of “Necroptosis in AD” is “Necroptosis activation in Alzheimer’s disease”, which reveals a new cell death pathway in AD patient’s brains: necroptosis, a novel form of programmed cell death, leads to the death of neurons, resulting in the worsening of AD, cognitive decline, and brain atrophy. This study offers new hope for treating brain cell death and opens a new window for AD research [34].
The clusters in the three domains are enumerated in Supplementary Table 3. Figure 3A-C shows the citation reference map of “Ferroptosis in AD”, “Pyroptosis in AD”, and “Necroptosis in AD”, respectively (nodes in the figure represent cited references, and lines between nodes represent co-citation relationships). The various colors in the nodes represent different years, and the larger the node area, the greater the number of citations. Purple rings represent centrality, and nodes with high centrality are considered key points in the literature. Figure 3D-F shows the Cluster view plot of cited references for “Ferroptosis in AD”, “Pyroptosis in AD”, and “Necroptosis in AD” (The various colors in the cluster represent different years, and the larger the cluster area, the greater the number of citations. Nodes in the map represent cited references). Cluster analysis categorized the majority of terms related to “Ferroptosis in AD” into 11 primary groups (Supplementary Table 3) and the first three clusters in the field were “selective ferroptosis vulnerability “, “age-associated neurodegenerative diseases”, and “iron exposure”. Of all clusters, “selective ferroptosis vulnerability” (cluster #0) had the most articles with 39. The major citing article of the cluster is “Ferroptosis as a mechanism of neurodegeneration in Alzheimer’s disease”. This review describes the increasing molecular and clinical evidence implicating ferroptosis in AD pathogenesis and suggests that iron and ferroptosis may be therapeutic targets for AD [35].
Fig. 3
Visual analysis of most commonly cited references. Visualization of co-cited references related to “Ferroptosis in AD” (A), “Pyroptosis in AD” (B), and “Necroptosis in AD” (C). A-C) Nodes are sized according to the number of citations. Cluster analysis of co-cited references related to “Ferroptosis in AD” (D), “Pyroptosis in AD” (E), and “Necroptosis in AD” (F).
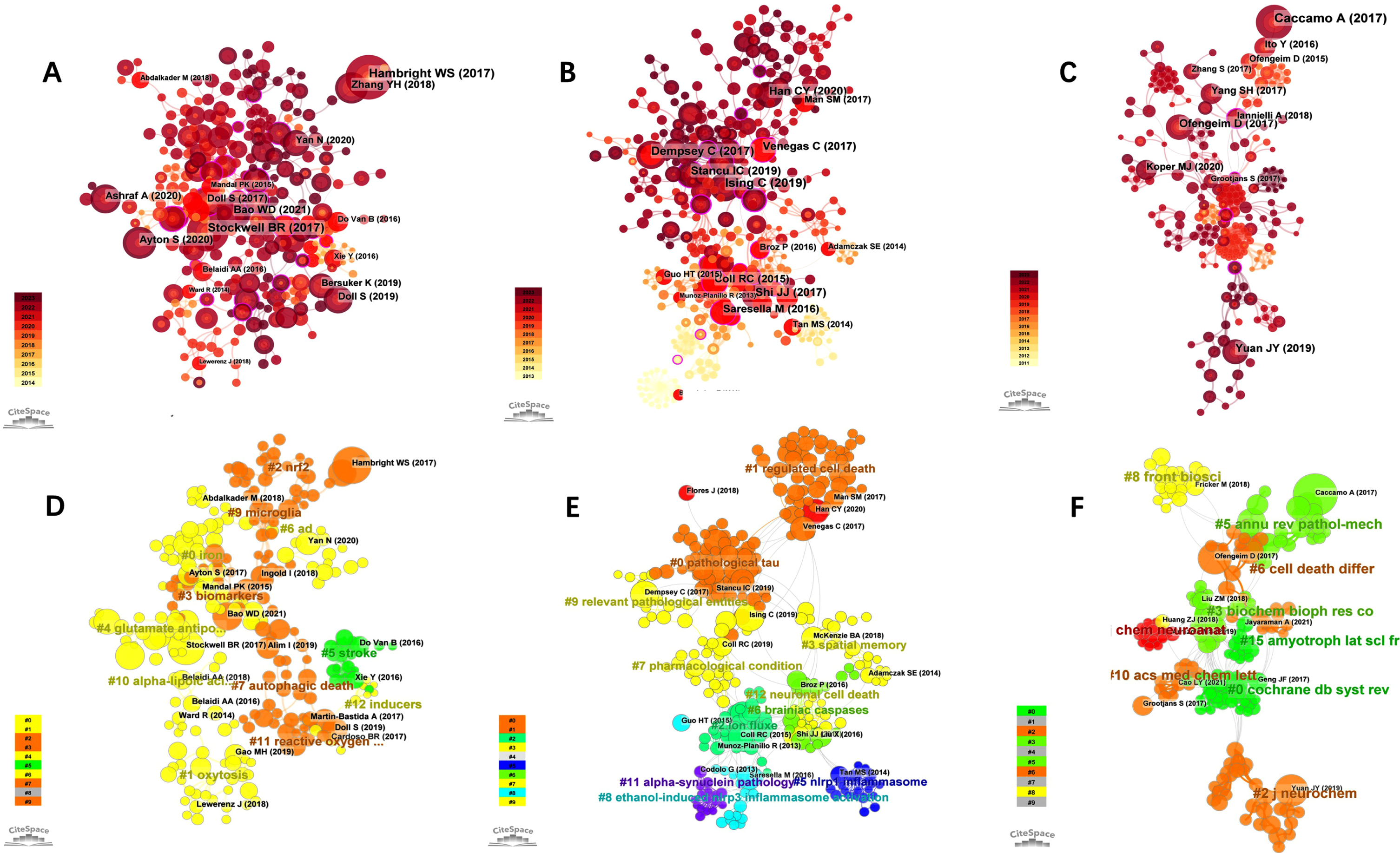
Cluster analysis categorized the majority of terms related to “Pyroptosis in AD” into 11 main categories (Supplementary Table 3), and the first three clusters in this area were “pathological tau”, “regulated cell death”. and “ion flux”. Of all the clusters, “pathological tau” (cluster #0) had the most articles with 59 articles, and the most cited article in this cluster is “Pyroptosis, and its Role in Central Nervous System Disease”, which describes the characteristics, molecular regulatory mechanisms, and therapeutic targets of pyroptosis, as well as their role in various forms of neurological disease, as well as promising new therapeutic strategies against pyroptosis [36].
Cluster analysis categorized the majority of terms related to “Necroptosis in AD” into 9 main categories (Supplementary Table 3) and the top three clusters in the field were “ripk1-mediated neuroinflammation”, “Coeloglossum viride var”. and “molecular mechanism”. Of all clusters, “ripk1-mediated neuroinflammation” (cluster #0) had the most articles with 35 articles. The major citing article of the cluster is “Necroptosis and RIPK1-mediated neuroinflammation in CNS diseases”. This paper reviews necroptosis in neurological illnesses and suggests that inhibiting RIPK1 may help suppress numerous cell death pathways, reduce neuroinflammation, and treat AD.
The profile value (S) reflects the cluster’s mean contour value. In general, if S > 0.5 and S > 0.7, the clustering is deemed correct and convincing, respectively. In this study, all the clusters we chose above were > 0.7 indicating that the clusters were considered credible.
Analysis of keywords
Figure 4A-C shows the Keyword plots of “Ferroptosis in AD”, “Pyroptosis in AD”, and “Necroptosis in AD” (nodes in the plots represent keywords). Line shows the co-occurrence relation between nodes. The various colors in the nodes represent different years, and the larger the node area, the higher the frequency. Purple rings represent centrality, and nodes with high centrality are considered key points in the literature. According to CiteSpace’s visual keyword analysis (Fig. 4A-C) and the top ten keywords with the highest frequency (Supplementary Table 4), in the field of “Ferroptosis in AD” we can observe that “Alzheimer’s disease”, “oxidative stress”, and “lipid peroxidation” were the three most prominent words; alpha lipoic acid (0.4), activation (0.34), and cerebrospinal fluid (0.32) showed highest intermediary centrality. In the field of “Necroptosis in AD”, “Alzheimer’s disease”, “nlrp3 inflammasome”, and “activation” were the three most prominent words; brain (0.43), caspase 1 activation (0.35), and gasdermin-d (0.25) showed highest intermediary centrality. In the field of “Pyroptosis in AD”, “Alzheimer’s disease”, “cell death”, and “activation” were the three most prominent words; double cell death (0.45), Alzheimer’s disease (0.35), and amyloid precursor protein (0.34) showed highest intermediary centrality.
Fig. 4
Visualization of keywords in “Ferroptosis in AD” (A), “Pyroptosis in AD” (B), and “Necroptosis in AD” (C). Burst keyword analysis in “Ferroptosis in AD” (D), “Pyroptosis in AD” (E), and “Necroptosis in AD” (F).
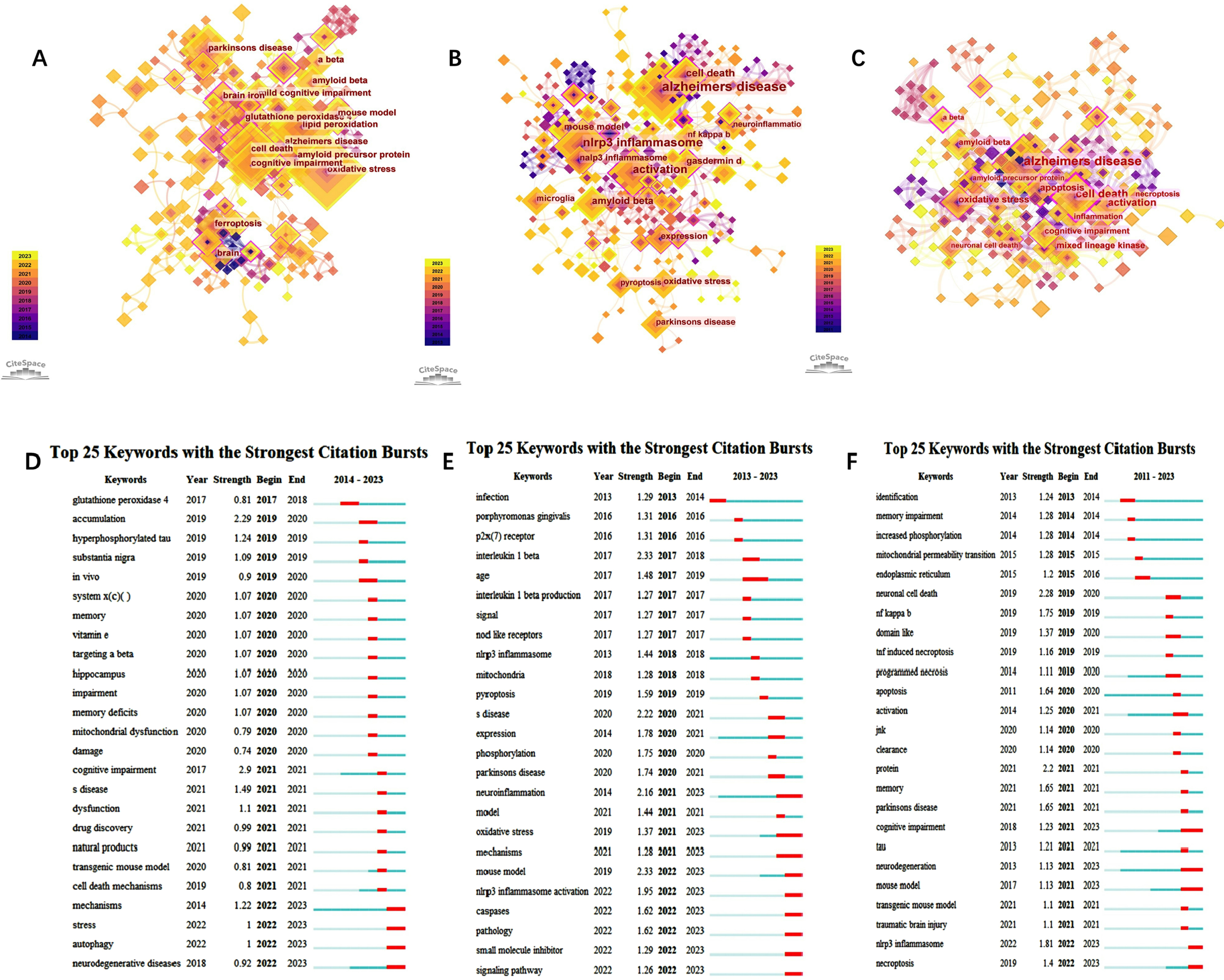
Burst keyword analysis may track a topic’s development, foretell its future course, and uncover possible flashpoints. We collected all burst keywords in the three domains (Fig. 4D-F). Figure 4D-F shows the Burst plots of keywords from “Ferroptosis in AD”, “Pyroptosis in AD”, and “Necroptosis in AD” (red bars indicate that the keyword is frequently cited and green bars indicate that the keyword is infrequently cited).
In the domain of “Ferroptosis in AD”, the focus of the keywords was primarily between 2020 and 2021. The strongest keywords are “accumulation”, “mechanisms” stress”, “autophagy”, and “neurodegenerative”. Furthermore, “mechanisms”, “stress”, “autophagy”, and “neurodegenerative” had not only been followed for the longest time, and so far they are still the burst words. This shows that scientists are currently concentrating their efforts on understanding the mechanisms underlying AD.
In the field of “Pyroptosis in AD”, the year with the most Burst keywords was 2022. The stronger keywords are “infection”, “porphyry monas gingivalis”, “p2x (7)receptor”, “interleukin 1 beta”, “age”; “neuroinflammation”, “age”, “oxidative stress”, “mechanisms” had followed for the longest time; the latest bursts were concentrated in “neuroinflammation”, “mouse model”, “oxidative stress”, “mechanisms” caspases” “pathology”, “small molecule inhibitor” “nlrp3 inflammasome activation”, and “signaling pathway”. All of these pointed to the possibility that the current hotspots may be centered on the question of how pyroptosis causes AD.
In the field of “Necroptosis in AD”, the year with the most Burst keywords was 2021. The stronger keywords are “neuronal cell death”, “protein”, “nlrp3 inflammasome”, “nf kappa b”, and “memory” indicating that “cognitive impairment”, “neurodegeneration”, and “mouse model” followed for the longest time, and so far they are still the Burst words. Except for the three above, the latest bursts were concentrated in “nlrp3 inflammasome” and “necroptosis”.
The biggest role of the keyword time zone map is to help beginners in a certain field quickly understand the development of a certain field’s pulse and predict the direction of development. Figure 5A-C shows the time zone view of the keywords (The nodes in the figure represent the keywords. Lines between nodes represent co-occurrence relationships. Various colors in the nodes represent different years; the larger the node area, the higher the frequency. Purple rings represent centrality; nodes with high centrality are considered key points in the literature). For example, our research in Fig. 5A in 2023 mainly focuses on neurons, transition metals, behavior brain insulin resistance, calcium homeostasis, astrocytes, cell, and alternative activation. In Fig. 5B, in 2023 research mainly focuses on inflammatory caspases, association, gsdmd, autoinhibition, cognitive dysfunction, functional enrichment analysis, and accumulation. In Fig. 5C, in 2023 research focuses on mitochondrial dysfunction, programmed cell death, amyloid degrading enzymes, gene expression, extracellular tau, and hippocampal.
Fig. 5
The keyword time zone map in “Ferroptosis in AD”, “Pyroptosis in AD”, and “Necroptosis in AD”.
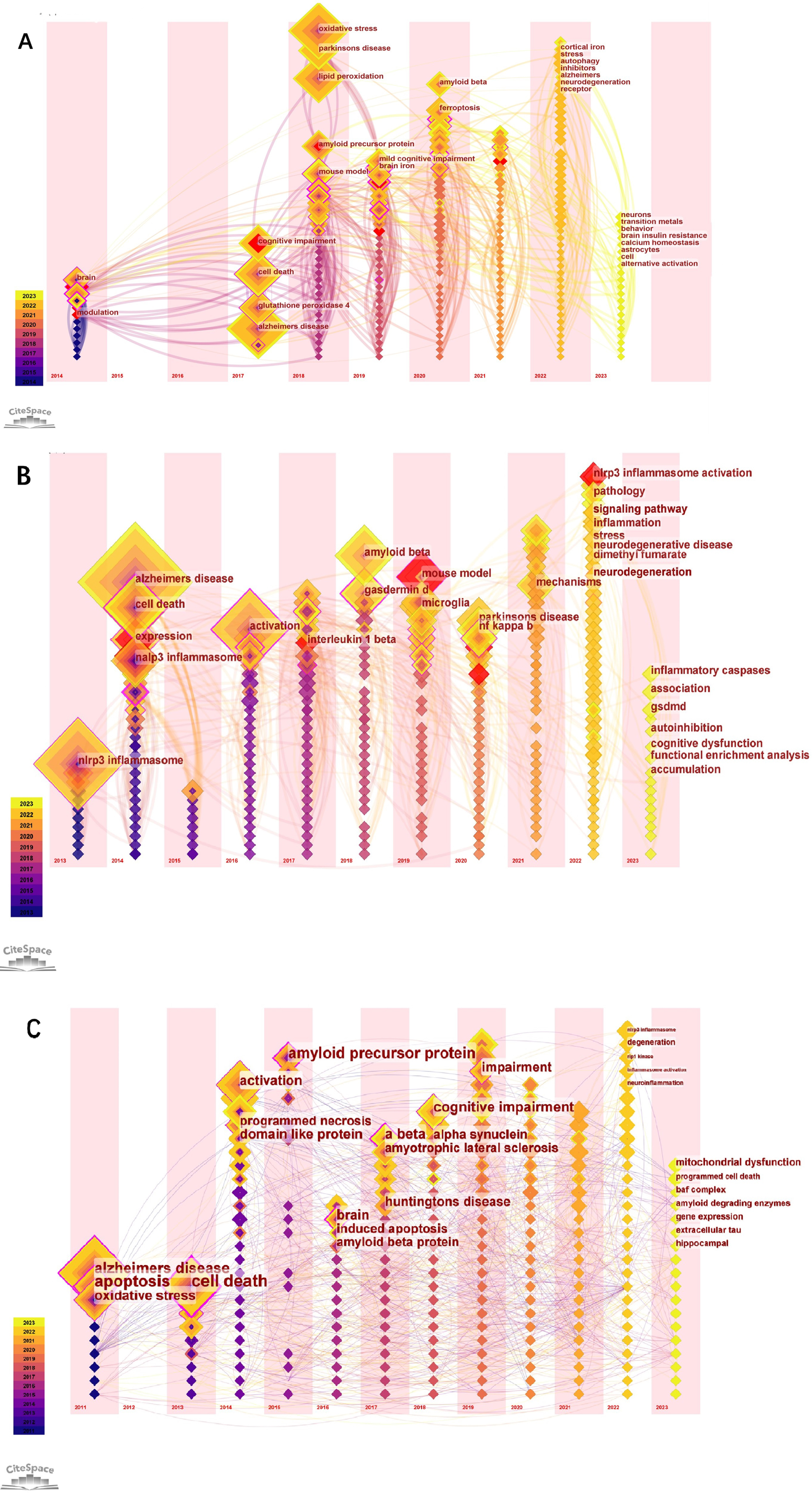
Analysis of countries/regions and analysis of institutions
There were 123 articles published on “Ferroptosis in AD” by a total of 174 organizations from 23 countries. 95 manuscripts were published in the field of “Pyroptosis in AD” by 280 organizations from 37 countries. 163 organizations from 25 countries contributed 84 publications to the field of “Necroptosis in AD”. The top ten productive countries and institutions in these three domains are in Supplementary Table 5. China and the United States were the top two countries for publishing in all three areas, with Australia having a higher average number of publications in all three areas. Additionally, China, the United States, and Australia were all cited a lot, which indicates that they have made a substantial contribution and influence in this area. The size of the circle in Supplementary Figure 2A-C indicates the number of papers published, and the thick line in the middle line of Supplementary Figure 2D-F indicates the close degree of cooperation between the two countries. Supplementary Figure 2 clearly shows a collaborative network of publications and relationships in each country, and it is worth noting that there is a lot of active collaboration between different countries. For example, China and the United States have active cooperation in three areas.
Analysis of journals
A total of 74, 89, and 69 journals distributed publications on “Ferroptosis in AD”, “Pyroptosis in AD”, and “Necroptosis in AD” respectively. Supplementary Table 6 shows the top 10 most productive journals in the three fields. Supplementary Figure 3A-C shows the visualization of journals, Supplementary Figure 3D-F shows the visualization of the years of literature published, and Supplementary Figure 3G-I shows the thermodynamic chart of journals. Acta Neuropathologica Communications (IF7.1), Frontiers in Aging Neuroscience (IF8.3), and Frontiers in Immunology (IF7.3) ranked first in “Ferroptosis in AD”, “Pyroptosis in AD”, and “Necroptosis in AD” respectively. Obviously, the average publication of the three journals, Cell Death and Differentiation, Redox Biology, and Cell Death & Disease were the highest in the three leading rates of “Ferroptosis in AD”, “Pyroptosis in AD”, and “Necroptosis in AD”, respectively, indicating that they had the greatest influence. Supplementary Figure 4 shows the journal double maps. Journal double maps shows the citation relationship between journals and co-cited journals and the fields in which they are mainly concentrated. The left side is the citing journal group, and the right side is the cited journal group. The orange path is the main citation path, which represents the research published in Molecular/Biology/Genetics that was mainly cited by the literature in Molecular/Biology/Immunology.
DISCUSSION
General overview
In this study, we objectively analyzed the literature in the fields of “Ferroptosis in AD”, “Pyroptosis in AD”, and “Necroptosis in AD” using bibliometric techniques. We discovered that these three fields are constantly changing, especially “Ferroptosis in AD,” which is anticipated to become a new AD hypothesis. Analysis based on keywords, in the field of “Ferroptosis in AD” we can observe that “oxidative stress” and “lipid peroxidation” were the two most prominent words. In the field of “Pyroptosis in AD”, “cell death” and “activation” were the two most prominent words and in the field of “Necroptosis in AD”, “nlrp3 inflammasome” and “activation” were the two most prominent words. The central content and hotspots of a particular field are revealed by co-cited articles and literature cluster analysis. Highly cited articles can indicate central research, whereas high-intensity outbreaks can indicate the emergence of academic hotspots [37]. In this study, “Cell Death and Differentiation” had the highest impact factors in the area of “Necroptosis in AD” and “Ferroptosis in AD”, “Journal of Neuroinflammation” had the highest impact factor in the field of “Pyroptosis in AD”, and “Cell Death and Differentiation”, “Redox Biology” and “Cell Death & Disease” had the highest average citations in the above three fields, respectively, indicating that their published articles were of high quality and had strong persuasive power and influence. While among the top 10 institutions, Harvard Medical School, Sichuan University, and University of Queensland are international leaders in the fields of “Ferroptosis in AD”, “Pyroptosis in AD”, and “Necroptosis in AD” respectively. In addition, we can observe that the institutions in these three fields have contacts and exchanges with each other. If the institutions can have more cooperation and communication in the future, it will be conducive to the development of related fields.
Mechanisms of ferroptosis, pyroptosis, and necroptosis in AD
Ferroptosis and AD
Iron in the brain is controlled by a precise and tightly controlled mechanism, and when it is dysregulated, both iron overload and deficiency are harmful to the brain [38]. The most typical histopathological features of AD are the deposition of extracellular Aβ in senile plaques and intracellular NFTs formed by hyperphosphorylation of tau protein. Many studies have shown that brain iron dyshomeostasis is tightly associated with Aβ plaques and NFTs. Iron can enhance β-secretase activity by reducing Furin [39, 40], thereby favoring β-secretase activity [41] and enhancing the amyloid-producing pathway [42]. Iron also directly binds to Aβ in the amino acid residues of His6, His13, and His14, thus strengthening the neurotoxicity of Aβ [43, 44]. Additionally, tau hyperphosphorylation and aggregation impair the surface transport of AβPP, which leads to toxic neuronal iron accumulation and aggravation of NFT, leading to a vicious cycle that eventually leads to AD [45, 46]. Neuroinflammatory response also are the typical changes of AD pathogenesis. The inflammatory response releases cytokines and pro-inflammatory substances, which in turn promote the accumulation of iron ions in nerve cells. Excess iron ions can trigger further worsening of the inflammatory response, forming a vicious cycle that leads to cell death. The pathogenesis of AD is iron promoting the production of free radicals and oxidative stress. In general, oxidative stress levels are higher in the brains of patients with AD, which leads to increased iron release and accumulation in nerve cells. Excessive iron ions will react with oxygen free radicals and produce harmful oxidative substances that damage cellular lipids, proteins, and DNA, eventually leading to cell death, and this ferroptosis-induced neuronal loss in AD has attracted increasing attention [47, 48].
According to the mechanism of “Ferroptosis in AD”, many pharmacological modulators of the ferroptosis pathway have been identified, targeting proteins involved in iron homeostasis and autophagy; the production and detoxification of lipid peroxides, and cyst(e)/glutathione metabolism [49]. Ferroptosis can be prevented with iron chelators, antioxidants, and ferroptosis blockers. Although various molecules have been found to have the potential to alleviate iron homeostasis and prevent ferroptosis, finding a way for these potentially useful drugs, such as iron chelators and antioxidants, to efficiently move the blood-brain barrier across the brain to exert their effects while avoiding systemic side effects will be the greatest challenge for future pharmacological studies of AD treatment based on the underlying “ferroptosis hypothesis” [50].
Pyroptosis and AD
Aβ plaque deposition and NFTs can activate neuroinflammation, and neuroinflammation can both promote and accelerate the occurrence and development of AD, so the neuro-inflammatory response plays an important role in the occurrence and development of AD. When the central nervous system is damaged or inflamed which releases cytokines and proinflammatory substances such as IL-1β, it induces the proliferation and recruitment of neuroinflammation-related cells, microglia and astrocytes, to the corresponding sites, mediating the occurrence of neuroinflammation. At present, there are two relatively established pyroptosis pathways, namely, the classical pathway, which is dependent on caspase-1 [51], and the non-classical pathway, which is dependent on caspase-4/5/11, and by recognizing danger signals from pathogens and host sources, inflammasomes initiate inflammation, participate in pathogen removal, and complete the adaptive immune response [52]. The inflammasome mainly consists of receptor protein, apoptosis-associated speck-like protein (ASC), and pro-caspase-1. The inflammasome is associated with the occurrence and development of AD. NLRP1 is the first member of the discovered NOD-like receptor (NLR) family, it induces downstream inflammatory cascade through caspase-1 activation, IL-1β and IL-18 maturation, and neuronal death, which further contributes to the development of AD [53]. NLRP3 is a relatively common inflammasome and has been extensively studied, which is considered a potential inflammatory marker. On the one hand, the NLRP3 inflammasome has been shown to significantly cause age-related cognitive decline and can be effectively activated by Aβ plaques. On the other hand, the NLRP3 inflammasome also promotes hyperphosphorylation of tau proteins, causing them to accumulate in neurons, which implies that targeting focal death may be the next therapeutic target for alleviating AD pathology [19]. There are many various inhibitors of the NLRP3 cascade to intervene in AD by blocking the expression of NLRP3, ASC, and caspase-1, neutralizing the expression of inflammatory cytokines, and inhibiting the activity of related enzymes at present [54]. Moreover, the small molecule MCC950 containing A dimeric sulfonylureas can block canonical and non-canonical NLRP3 activation and improve Aβ phagocytosis in cultured microglia, suggesting that compounds based on MCC950 and MCC950 are a promising treatment for AD [55].
Necroptosis and AD
In a recent study conducted by Tanaka et al., it was demonstrated that neuronal necrosis not only contributes to the development of dementia but also reaches its highest point during the stage of mild cognitive impairment preceding the clinical manifestations of AD [56]. The execution of necroptosis involves three core components, namely receptor-interacting protein kinases RIPK1, RIPK3, and mixed lineage kinase domain-like protein (MLKL). In specific conditions, necroptosis is activated by DRs, TNF-α-triggered necroptosis is the most widely studied mechanism of necroptosis, and IFN signaling is known as a signaling pathway that can activate necroptosis. IFN signaling is known as a signaling pathway that can activate necroptosis. Upon activation, MLKL binds to the cytoplasmic membrane via an affinity site that binds phosphatidylinositol phosphate, and when MLKL undergoes translocation to the cytoplasmic membrane, it disrupts membrane integrity in a dose-dependent manner. Necroptosis is activated to participate in several pathological processes of AD. For example, RIPK1/RIPK3 complex forms an insoluble amyloid complex for Aβ aggregation upon activation of necroptosis [57]. Aβ aggregates, in turn, promote neuronal necroptosis through the RIPK1-MLKL axis, that is, necroptosis and Aβ may form a cascade effect in AD. That is, necrotizing complexes promote Aβ aggregation to form plaques, and Aβ diffusion over time further exacerbates neurotoxicity and disease severity. In addition, tau by activating RIPK1/RIPK3/MLKL and the NfkappaB pathway mediated necrotic apoptosis and inflammation, to promote cell death [58, 59].
Of course, in addition to these two points, currently, numerous studies have evidenced necroptosis participates the involvement of necroptosis in AD pathology both in vitro and in vivo by neuronal death [22], blood-brain barrier damage [60], granulovacuolar degeneration [58], synaptic loss [61], restricted O-linked β-N-acetylglucosaminylation [62, 63], and mitochondrial impairment [64], leading to subsequent cognitive impairment [57].
The mechanism of necroptosis has prompted specifically targeting the regulatory constituents involved in the apoptotic mechanism namely RIPK1, RIPK3, and MLKL to develop inhibitors. In comparison to RIPK1 and RIPK3, the discovery of inhibitors targeting MLKL has been relatively limited [20], and inhibiting RIPK1 and using nanotechnology for delivery will be the most effective means of preventing additional cell death in the brain [23]. Disappointing, only a few inhibitors were applied to clinical, and most of the inhibitor has still not even entered clinical trials. Although the role of necroptosis in AD is multifaceted, and inhibition of necroptosis activation may serve as a multi-target treatment for AD, it is difficult to use proteins known to regulate these pathways because AD is a slowly progressive disease with varying degrees of necroptosis at different pathological stages and the complexity of necrosis pathways [65]. Therefore, we still need to continue to explore and study.
Limitation
This is the first study to describe “Ferroptosis in AD”, “Pyroptosis in AD”, and “Necroptosis in AD”. A bibliometric analysis was performed, but there are still some limitations. First, some keywords from the literature search might not have been included in the analysis, which could have an impact on the outcomes. Furthermore, the quality of the literature that was chosen for this study’s articles and reviews differed, which could undermine the validity of the analysis as a whole. However, a rapid overview of the study subjects, research hotspots, and trends in the areas of “Ferroptosis in AD”, “Pyroptosis in AD”, and “Necroptosis in AD” is unquestionably provided by the bibliometric analysis based on literature.
Conclusion
AD is a complex and mainly multifactorial chronic neurodegenerative disease. It is very complex that a single drug or intervention can avoid, reduce, or reverse the disease [66]. With the continuous development of research by domestic and foreign scholars, ferroptosis, pyroptosis, and necroptosis, have been confirmed to play an important role in AD, but studies on them in AD are still in the initial stage. In addition, the upstream and downstream roles of most cell death-related AD pathogenesis have not been thoroughly studied, and the relevant signaling pathways remain to be explored [67]. Therefore, researchers need to consider different therapeutic targets at different stages of AD, and the efficacy and safety of these therapies in the treatment process need to be further verified to provide new concepts and effective strategies for the prevention and treatment of AD in the future [68, 69].
ACKNOWLEDGMENTS
The authors have no acknowledgments to report.
FUNDING
This work was supported by the Chengdu Medical College-Chengdu Eighth People’s Hospital Clinical Science Research Fund Project (YLLNZD2301) and the Development and Regeneration Key Laboratory of Sichuan Province (SYS13-006); the National College Student Innovation and Entrepreneurship Training Program Project (202213705036); Sichuan Provincial College Student Innovation and Entrepreneurship Training Program Project (S202313705089).
CONFLICT OF INTEREST
There are no conflicts of interest that the authors are required to disclose.
DATA AVAILABILITY
The data supporting the findings of this study are available within the article and its supplementary material.
SUPPLEMENTARY MATERIAL
[1] The supplementary material is available in the electronic version of this article: https://dx.doi.org/10.3233/ADR-230092.
REFERENCES
[1] | ((1987) ) About a peculiar disease of the cerebral cortex. By Alois Alzheimer, 1907 (Translated by L. Jarvik and H. Greenson), Alzheimer Dis Assoc Disord 1: , 3–8. |
[2] | Hou Y , Dan X , Babbar M , Wei Y , Hasselbalch SG , Croteau DL , Bohr VA ((2019) ) Ageing as a risk factor for neurodegenerative disease. Nat Rev Neurol 15: , 565–581. |
[3] | Chen YG ((2018) ) Research progress in the pathogenesis of Alzheimer’s disease. Chin Med J (Engl) 131: , 1618–1624. |
[4] | Hulshof LA , van Nuijs D , Hol EM , Middeldorp J ((2022) ) The role of astrocytes in synapse loss in Alzheimer’s disease: A systematic review. Front Cell Neurosci 16: , 899251. |
[5] | van Bokhoven P , de Wilde A , Vermunt L , Leferink PS , Heetveld S , Cummings J , Scheltens P , Vijverberg EGB ((2021) ) The Alzheimer’s disease drug development landscape. Alzheimers Res Ther 13: , 186. |
[6] | Lei P , Ayton S , Bush AI ((2021) ) The essential elements of Alzheimer’s disease. J Biol Chem 296: , 100105. |
[7] | Dixon SJ , Lemberg KM , Lamprecht MR , Skouta R , Zaitsev EM , Gleason CE , Patel DN , Bauer AJ , Cantley AM , Yang WS , Morrison B 3rd , Stockwell BR ((2012) ) Ferroptosis: An iron-dependent form of nonapoptotic cell death. Cell 149: , 1060–1072. |
[8] | Stockwell BR , Angeli JPF , Bayir H , Bush AI , Conrad M , Dixon SJ , Fulda S , Gascon S , Hatzios SK , Kagan VE , Noel K , Jiang XJ , Linkermann A , Murphy ME , Overholtzer M , Oyagi A , Pagnussat GC , Park J , Ran Q , Rosenfeld CS , Salnikow K , Tang DL , Torti FM , Torti SV , Toyokuni S , Woerpel KA , Zhang DD ((2017) ) Ferroptosis: A regulated cell death nexus linking metabolism, redox biology, and disease. Cell 171: , 273–285. |
[9] | Deng L , He S , Guo N , Tian W , Zhang W , Luo L ((2023) ) Molecular mechanisms of ferroptosis and relevance to inflammation. Inflamm Res 72: , 281–299. |
[10] | Costa I , Barbosa DJ , Benfeito S , Silva V , Chavarria D , Borges F , Remião F , Silva R ((2023) ) Molecular mechanisms of ferroptosis and their involvement in brain diseases. Pharmacol Ther 244: , 108373. |
[11] | Becerril-Ortega J , Bordji K , Freret T , Rush T , Buisson A ((2014) ) Iron overload accelerates neuronal amyloid-β production and cognitive impairment in transgenic mice model of Alzheimer’s disease. Neurobiol Aging 35: , 2288–2301. |
[12] | Kim AC , Lim S , Kim YK ((2018) ) Metal ion effects on Aβ and tau aggregation. Int J Mol Sci 19: , 128. |
[13] | Vande Walle L , Lamkanfi M ((2016) ) Pyroptosis, Curr Biol 26: , R568–r572. |
[14] | Huang Y , Xu W , Zhou R ((2021) ) NLRP3 inflammasome activation and cell death. Cell Mol Immunol 18: , 2114–2127. |
[15] | Jorgensen I , Miao EA ((2015) ) Pyroptotic cell death defends against intracellular pathogens. Immunol Rev 265: , 130–142. |
[16] | Man SM , Karki R , Kanneganti TD ((2017) ) Molecular mechanisms and functions of pyroptosis, inflammatory caspases and inflammasomes in infectious diseases. Immunol Rev 277: , 61–75. |
[17] | Griffin WS , Liu L , Li Y , Mrak RE , Barger SW ((2006) ) Interleukin-1 mediates Alzheimer and Lewy body pathologies. J Neuroinflammation 3: , 5. |
[18] | Shi J , Gao W , Shao F ((2017) ) Pyroptosis: Gasdermin-mediated programmed necrotic cell death. Trends Biochem Sci 42: , 245–254. |
[19] | Shen H , Han C , Yang Y , Guo L , Sheng Y , Wang J , Li W , Zhai L , Wang G , Guan Q ((2021) ) Pyroptosis executive protein GSDMD as a biomarker for diagnosis and identification of Alzheimer’s disease, Brain Behav 11: , e02063. |
[20] | Khoury MK , Gupta K , Franco SR , Liu B ((2020) ) Necroptosis in the pathophysiology of disease. Am J Pathol 190: , 272–285. |
[21] | Mifflin L , Ofengeim D , Yuan J ((2020) ) Receptor-interacting protein kinase 1 (RIPK1) as a therapeutic target. Nat Rev Drug Discov 19: , 553–571. |
[22] | Jayaraman A , Htike TT , James R , Picon C , Reynolds R ((2021) ) TNF-mediated neuroinflammation is linked to neuronal necroptosis in Alzheimer’s disease hippocampus. Acta Neuropathol Commun 9: , 159. |
[23] | Richard R , Mousa S ((2022) ) Necroptosis in Alzheimer’s disease: Potential therapeutic target. Biomed Pharmacother 152: , 113203. |
[24] | Zhou W , Yuan J ((2014) ) Necroptosis in health and diseases. Semin Cell Dev Biol 35: , 14–23. |
[25] | Dhuriya YK , Sharma D ((2018) ) Necroptosis: A regulated inflammatory mode of cell death. J Neuroinflammation 15: , 199. |
[26] | Hicks D , Wouters P , Waltman L , de Rijcke S , Rafols I ((2015) ) Bibliometrics: The Leiden Manifesto for research metrics. Nature 520: , 429–431. |
[27] | Deng P , Shi H , Pan X , Liang H , Wang S , Wu J , Zhang W , Huang F , Sun X , Zhu H , Chen Z ((2022) ) Worldwide research trends on diabetic foot ulcers (2004–2020): Suggestions for researchers. J Diabetes Res 2022: , 7991031. |
[28] | Ninkov A , Frank JR , Maggio LA ((2022) ) Bibliometrics: Methods for studying academic publishing. Perspect Med Educ 11: , 173–176. |
[29] | Chen C ((2004) ) Searching for intellectual turning points: Progressive knowledge domain visualization. Proc Natl Acad Sci U S A 101 Suppl 1: , 5303–5310. |
[30] | Chen C , Hu Z , Liu S , Tseng H ((2012) ) Emerging trends in regenerative medicine: A scientometric analysis in CiteSpace. Expert Opin Biol Ther 12: , 593–608. |
[31] | van Eck NJ , Waltman L ((2010) ) Software survey: VOSviewer, a computer program for bibliometric mapping. Scientometrics 84: , 523–538. |
[32] | Hambright WS , Fonseca RS , Chen LJ , Na R , Ran QT ((2017) ) Ablation of ferroptosis regulator glutathione peroxidase 4 in forebrain neurons promotes cognitive impairment and neurodegeneration. Redox Biol 12: , 8–17. |
[33] | Ising C , Venegas C , Zhang S , Scheiblich H , Schmidt SV , Vieira-Saecker A , Schwartz S , Albasset S , McManus RM , Tejera D , Griep A , Santarelli F , Brosseron F , Opitz S , Stunden J , Merten M , Kayed R , Golenbock DT , Blum D , Latz E , Buee L , Heneka MT ((2019) ) NLRP3 inflammasome activation drives tau pathology. Nature 575: , 669–673. |
[34] | Caccamo A , Branca C , Piras IS , Ferreira E , Huentelman MJ , Liang WS , Readhead B , Dudley JT , Spangenberg EE , Green KN , Belfiore R , Winslow W , Oddo S ((2017) ) Necroptosis activation in Alzheimer’s disease. Nat Neurosci 20: , 1236–1246. |
[35] | Jakaria M , Belaidi AA , Bush AI , Ayton S ((2021) ) Ferroptosis as a mechanism of neurodegeneration in Alzheimer’s disease. J Neurochem 159: , 804–825. |
[36] | Hu YC , Wang BW , Li S , Yang S ((2022) ) Pyroptosis, and its role in central nervous system disease. J Mol Biol 434: , 167379. |
[37] | Chen P , Zhong C , Jin S , Zhang Y , Li Y , Xia Q , Cheng J , Fan X , Lin H ((2022) ) Global trends in research of lipid metabolism in T lymphocytes from 1985 to 2022: A bibliometric analysis. Front Immunol 13: , 884030. |
[38] | Hare D , Ayton S , Bush A , Lei P ((2013) ) A delicate balance: Iron metabolism and diseases of the brain. Front Aging Neurosci 5: , 34. |
[39] | Ward RJ , Zucca FA , Duyn JH , Crichton RR , Zecca L ((2014) ) The role of iron in brain ageing and neurodegenerative disorders. Lancet Neurol 13: , 1045–1060. |
[40] | Yan N , Zhang J ((2019) ) Iron metabolism, ferroptosis, and the links with Alzheimer’s disease. Front Neurosci 13: , 1443. |
[41] | Silvestri L , Camaschella C ((2008) ) A potential pathogenetic role of iron in Alzheimer’s disease. J Cell Mol Med 12: , 1548–1550. |
[42] | Guillemot J , Canuel M , Essalmani R , Prat A , Seidah NG ((2013) ) Implication of the proprotein convertases in iron homeostasis: Proprotein convertase 7 sheds human transferrin receptor 1 and furin activates hepcidin. Hepatology 57: , 2514–2524. |
[43] | Uranga RM , Salvador GA ((2018) ) Unraveling the burden of iron in neurodegeneration: Intersections with amyloid beta peptide pathology. Oxid Med Cell Longev 2018: , 2850341. |
[44] | Wojtunik-Kulesza K , Oniszczuk A , Waksmundzka-Hajnos M ((2019) ) An attempt to elucidate the role of iron and zinc ions in development of Alzheimer’s and Parkinson’s diseases. Biomed Pharmacother 111: , 1277–1289. |
[45] | Lei P , Ayton S , Finkelstein DI , Spoerri L , Ciccotosto GD , Wright DK , Wong BX , Adlard PA , Cherny RA , Lam LQ , Roberts BR , Volitakis I , Egan GF , McLean CA , Cappai R , Duce JA , Bush AI ((2012) ) Tau deficiency induces parkinsonism with dementia by impairing APP-mediated iron export. Nat Med 18: , 291–295. |
[46] | Ndayisaba A , Kaindlstorfer C , Wenning GK ((2019) ) Iron in neurodegeneration - cause or consequence? , Front Neurosci 13: , 180. |
[47] | Liu JL , Fan YG , Yang ZS , Wang ZY , Guo C ((2018) ) Iron and Alzheimer’s disease: From pathogenesis to therapeutic implications. Front Neurosci 12: , 632. |
[48] | Masaldan S , Bush AI , Devos D , Rolland AS , Moreau C ((2019) ) Striking while the iron is hot: Iron metabolism and ferroptosis in neurodegeneration. Free Radic Biol Med 133: , 221–233. |
[49] | Lane DJR , Metselaar B , Greenough M , Bush AI , Ayton SJ ((2021) ) Ferroptosis and NRF2: An emerging battlefield in the neurodegeneration of Alzheimer’s disease. Essays Biochem 65: , 925–940. |
[50] | Wang F , Wang J , Shen Y , Li H , Rausch WD , Huang X ((2022) ) Iron dyshomeostasis and ferroptosis: A new Alzheimer’s disease hypothesis? Front Aging Neurosci 14: , 830569. |
[51] | Han C , Yang Y , Guan Q , Zhang X , Shen H , Sheng Y , Wang J , Zhou X , Li W , Guo L , Jiao Q ((2020) ) New mechanism of nerve injury in Alzheimer’s disease: β-amyloid-induced neuronal pyroptosis. J Cell Mol Med 24: , 8078–8090. |
[52] | Yap JKY , Pickard BS , Chan EWL , Gan SY ((2019) ) The role of neuronal NLRP1 inflammasome in Alzheimer’s disease: Bringing neurons into the neuroinflammation game. Mol Neurobiol 56: , 7741–7753. |
[53] | Rajesh Y , Kanneganti TD ((2022) ) Innate immune cell death in neuroinflammation and Alzheimer’s disease. Cells 11: , 1885. |
[54] | Saresella M , La Rosa F , Piancone F , Zoppis M , Marventano I , Calabrese E , Rainone V , Nemni R , Mancuso R , Clerici M ((2016) ) The NLRP3 and NLRP1 inflammasomes are activated in Alzheimer’s disease. Mol Neurodegener 11: , 23. |
[55] | Xue W , Cui D , Qiu Y ((2022) ) Research progress of pyroptosis in Alzheimer’s disease. Front Mol Neurosci 15: , 872471. |
[56] | Tanaka H , Homma H , Fujita K , Kondo K , Yamada S , Jin X , Waragai M , Ohtomo G , Iwata A , Tagawa K , Atsuta N , Katsuno M , Tomita N , Furukawa K , Saito Y , Saito T , Ichise A , Shibata S , Arai H , Saido T , Sudol M , Muramatsu SI , Okano H , Mufson EJ , Sobue G , Murayama S , Okazawa H ((2020) ) YAP-dependent necrosis occurs in early stages of Alzheimer’s disease and regulates mouse model pathology. Nat Commun 11: , 507. |
[57] | Ofengeim D , Mazzitelli S , Ito Y , DeWitt JP , Mifflin L , Zou C , Das S , Adiconis X , Chen H , Zhu H , Kelliher MA , Levin JZ , Yuan J ((2017) ) RIPK1 mediates a disease-associated microglial response in Alzheimer’s disease, Proc Natl Acad Sci U S A 114: , E8788–e8797. |
[58] | Koper MJ , Van Schoor E , Ospitalieri S , Vandenberghe R , Vandenbulcke M , von Arnim CAF , Tousseyn T , Balusu S , De Strooper B , Thal DR ((2020) ) Necrosome complex detected in granulovacuolar degeneration is associated with neuronal loss in Alzheimer’s disease. Acta Neuropathol 139: , 463–484. |
[59] | Dong Y , Yu H , Li X , Bian K , Zheng Y , Dai M , Feng X , Sun Y , He Y , Yu B , Zhang H , Wu J , Yu X , Wu H , Kong W ((2022) ) Hyperphosphorylated tau mediates neuronal death by inducing necroptosis and inflammation in Alzheimer’s disease. J Neuroinflammation 19: , 205. |
[60] | Zou C , Mifflin L , Hu Z , Zhang T , Shan B , Wang H , Xing X , Zhu H , Adiconis X , Levin JZ , Li F , Liu CF , Liu JS , Yuan J ((2020) ) Reduction of mNAT1/hNAT2 contributes to cerebral endothelial necroptosis and Aβ accumulation in Alzheimer’s disease. Cell Rep 33: , 108447. |
[61] | Ma D , Li Y , Zhu Y , Wei W , Zhang L , Li Y , Li L , Zhang L ((2021) ) Cornel iridoid glycoside ameliorated Alzheimer’s disease-like pathologies and necroptosis through RIPK1/MLKL pathway in young and aged SAMP8 Mice. Evid Based Complement Alternat Med 2021: , 9920962. |
[62] | Yuzwa SA , Shan X , Jones BA , Zhao G , Woodward ML , Li X , Zhu Y , McEachern EJ , Silverman MA , Watson NV , Gong CX , Vocadlo DJ ((2014) ) Pharmacological inhibition of O-GlcNAcase (OGA) prevents cognitive decline and amyloid plaque formation in bigenic tau/APP mutant mice. Mol Neurodegener 9: , 42. |
[63] | Park J , Ha HJ , Chung ES , Baek SH , Cho Y , Kim HK , Han J , Sul JH , Lee J , Kim E , Kim J , Yang YR , Park M , Kim SH , Arumugam TV , Jang H , Seo SW , Suh PG , Jo DG ((2021) ) O-GlcNAcylation ameliorates the pathological manifestations of Alzheimer’s disease by inhibiting necroptosis, Sci Adv 7: , eabd3207. |
[64] | Wang Z , Jiang H , Chen S , Du F , Wang X ((2012) ) The mitochondrial phosphatase PGAM5 functions at the convergence point of multiple necrotic death pathways. Cell 148: , 228–243. |
[65] | Zhang R , Song Y , Su X ((2023) ) Necroptosis and Alzheimer’s disease: Pathogenic mechanisms and therapeutic opportunities, J Alzheimers Dis 94: , S367–S386. |
[66] | Plascencia-Villa G , Perry G ((2021) ) Preventive and therapeutic strategies in Alzheimer’s disease: Focus on oxidative stress, redox metals, and ferroptosis. Antioxid Redox Signal 34: , 591–610. |
[67] | Jayaraman A , Reynolds R ((2022) ) Diverse pathways to neuronal necroptosis in Alzheimer’s disease. Eur J Neurosci 56: , 5428–5441. |
[68] | Ma H , Dong Y , Chu Y , Guo Y , Li L ((2022) ) The mechanisms of ferroptosis and its role in alzheimer’s disease. Front Mol Biosci 9: , 965064. |
[69] | Zhao D , Yang K , Guo H , Zeng J , Wang S , Xu H , Ge A , Zeng L , Chen S , Ge J ((2023) ) Mechanisms of ferroptosis in Alzheimer’s disease and therapeutic effects of natural plant products: A review. Biomed Pharmacother 164: , 114312. |