Glucose and Several Mitogenic Agents Modulate the Glucagon-Like Peptide-2 Receptor Expression in Cultured Rat Astrocytes
Abstract
Background:
Glucagon-like peptide-2 (GLP-2) is an intestinal trophic factor that induces astrocyte proliferation through its own receptor (GLP-2R), but the control of its expression is not well known.
Objective:
To study the effects of glucose and of different mitogenic agents on the control of GLP-2R expression in cultured rat astrocytes.
Methods:
GLP-2R mRNA content was measured by quantitative RT-PCR.
Results:
GLP-2R expression was higher in proliferating than in resting cells. The expression was dependent of glucose concentration both in the absence and in the presence of GLP-2. In the presence of a high glucose concentration, GLP-2, PDGF, and PDGF plus GLP-2 presented opposite effects depending on the incubation time. However, insulin, IGF-1, and EGF alone, and plus GLP-2 had no effect. IGF-2, but not IGF-2 plus GLP-2, increased the expression. On the contrary, NGF decreased the GLP-2R expression, but NGF plus GLP-2 increased it even until values similar to those obtained with GLP-2 alone. Interestingly, in the presence of a low glucose concentration, leptin and NPY produced a significant reduction of GLP-2R expression.
Conclusion:
Astrocytes are distributed throughout the brain, where GLP-2 appears to have important functions. Since these cells express the GLP-2R, the results of this study could be considered of interest to advance the knowledge of the role of GLP-2 signaling in the CNS, which should lead a better understanding of the events that occur under normal and pathophysiological conditions.
INTRODUCTION
Glucagon-like peptide-2 (GLP-2) is a 33-amino-acid peptide derived from the proglucagon gene which is expressed in the same way in the enteroendocrine L cells of distal jejunum, ileum, and colon, and in the neurons of the caudal brain stem and hypothalamus [1, 2].
GLP-2 mediates its biological actions through a G-protein coupled receptor (GLP-2R) whose expression was first studied in the digestive tract of mice, as well in many other tissues and cell cultures [3–6].
The regulation of the intestinal GLP-2R expression has been studied in mice under different nutritional conditions, being very low during fasting but increased when the animals were fed with high-fat diets (HFD) [7, 8]. In addition, GLP-2R expression was also upregulated in hepatic stellate cells of mice with hepatosteatosis [9]. Likewise, GLP-2R expression was upregulated in the jejunum of mice subjected to acute exercise [10]. In turn, regulation of the GLP-2R expression has also been studied in several pathologies [11–14] and under diabetogenic conditions [15].
The GLP-2R expression has also been described in different neuroendocrine cells and in enteric neurons of the gastrointestinal tract from various species [16]. In addition, in several regions of the central nervous system (CNS), including the hypothalamus, hippocampus, and cortex [17], GLP-2R is involved in neuromodulatory and neuroprotective functions [18] and in controlling energy balance and glucose homeostasis [19]. Since GLP-2 induces memory improvement, it could be used in the treatment of cognitive deficits in patients suffering from neurodegenerative diseases [20, 21].
Astrocytes are the most abundant and diverse non-neuronal cell type in the CNS, which in addition to providing nutrients to neurons, also seem to be important in maintaining their optimal function. One of the classic roles attributed to astrocytes is that of anatomical support for neurons. They play very important homeostatic roles in the brain, such as regulating the synaptic function over long distances, maintaining the blood–brain barrier and fluid and ion homeostasis, as well as participating in glucose and lipid metabolism to control energy homeostasis driven by insulin and leptin. Likewise, astrocytes can carry out opposite responses, such as beneficial neuromodulatory effects or harmful inflammatory effects, depending on the intensity and exposure time of the different stimuli, including HFD or obesity, but also of the cytokines secreted by these cells, such as interleukin 6, interleukin 1 beta, or tumor necrosis factor alpha [22, 23].
Glia are activated in response to brain damage [24], although the response to this phenomenon is still unclear. Upon activation, astrocytes express receptors for numerous molecules, some of which could be involved in the healing process. We believe that GLP-1 and GLP-2 receptors should be also added to that long list because both are expressed in the brain cortex [17, 24] and even GLP-2 stimulates astrocyte proliferation through its own receptor [17]. Furthermore, the copy number of the transcript was time- and dose-dependent on heat inactivated fetal bovine serum (hiFBS) and on the presence of GLP-2 and insulin-like growth factor-1 (IGF-1) [25].
Recently, an association between body mass index (BMI) and GLP-2R mRNA expression was observed in the dorsolateral prefrontal cortex of individuals with mood disorders compared to healthy control subjects [26]. However, the regulation of GLP-2R expression in the CNS is still poorly understood. Therefore, given the relevant role that proglucagon-derived peptides are acquiring in maintaining the functionality of various brain areas, the main objective of this work was to study the effect of GLP-2, in the presence of various concentrations of glucose, on the expression of GLP-2R mRNA in rat astrocyte cultures. Another objective of this work was to study whether certain growth factors (IGF-1, PDGF, IGF-2, NGF, and EGF), hormones (insulin and leptin), and neuropeptide Y (NPY) alone, and plus GLP-2, also modulated the content of GLP-2R mRNA in these cells. We choose these ligands because, as GLP-2, they play important roles in the CNS, including various biological effects on glial cells, such as stimulating cell proliferation [25, 27, 28] or transporting and metabolizing diverse substances, such as glucose [29].
MATERIALS AND METHODS
Materials
Rat GLP-2 was provided by Bachem (St. Helens, UK). Recombinant human insulin (Arg+Zn) and NGF were supplied by Calbiochem (La Jolla, CA, USA). Recombinant IGF-1 and IGF-2, PDGF, EGF, leptin, and NPY came from PeproTech EC Ltd (London, UK). Fetal bovine serum (FBS) was from Gibco (Waltham, MA, USA). Bovine serum albumin (BSA), Dulbecco’s Modified Eagle Medium/Nutrient Mixture F-12 (DMEM/F-12, containing 17.5 mM glucose), DMEM (with 0, 5.5, and 25 mM glucose), Lipopolisaccharide (E. coli) were from Sigma (Darmstadt, Germany), and tissue culture flasks and plates were from TPP Techno Plastic Products AG (Trasadingen, Switzerland). All the other chemicals were reagent grade or molecular biology grade.
Animals
The animals were provided by the Center for Assistance to Research located in the Faculty of Medicine of the Complutense University. All the procedures involving animals were approved by the appropriate institutional review committee for animal experimentation and met the guidelines for the care and use of laboratory animals specified by the EuropeanUnion.
Rat astrocyte cultures and treatments
Primary rat astroglial cell cultures were prepared from cerebral hemispheres of one-day-old postnatal Wistar rat pups, as previously described [30, 31]. To minimize the number of animals, male and female astroglial cells were analyzed together. Mechanically dissociated cells were plated onto 75 cm2 tissue culture flasks at low density and grown in a DMEM/F-12 medium containing 15 mM of 4-(2-hydroxyethyl)-1-piperazineethanesulfonic acid (HEPES) in the presence of 1% (v/v) of penicillin and streptomycin and supplemented with 10% (v/v) FBS. The cells were maintained at 37°C with 5% CO2. The culture medium was changed every 2-3 days. After 21–28 days, astroglial cells were dispersed by treatment with trypsin/ethylenediaminetetraacitic acid (Gibco) for 1-2 min at 37°C. Enzymatic activity was inhibited by adding FBS. The cellular contents of all the flasks were mixed and homogenized. Then replated at approximately 2.3–3×104 cells/cm2 on P-100 plates and sub-cultured in DMEM/F-12 again or in DMEM 5.5 mM glucose, depending on the experiment. The primary cultures were immunocytochemically analyzed, revealing that at least 95% of the cells were positive for glial fibrillary acidic protein (GFAP), the specific marker for astrocytes. The percentage of cells positive for GFAP was determined by analyzing several photomicrographs (20x magnification) taken at random from the surface of the culture plates with a digital camera attached to a microscope (Leica DRMB). Using scientific image processing software (ImageJ), GFAP positive cells in each photograph were counted and compared to negative cells.
The different treatments were carried out as indicated in the figure legends when the cultures were about 85–90% confluent and after 24–48 h incubation with FBS-free medium (DMEM/F-12 or DMEM 5.5 mM glucose). When we studied the effect of glucose concentration, cells were also maintained for 2–4 h in glucose-free DMEM before treatment. The range of glucose concentrations used in one experiment was between 1.4 and 25 mM, which, compared to normal serum glucose levels, ranges from very severe hypoglycemia to overt hyperglycemia. Furthermore, other experiments were performed in the presence of a fixed concentration of glucose (1.4 or 17.5 mM). Reactions were stopped at the end of the incubation period by removing of supernatants, and cells were immediately washed three times with ice cold phosphate buffered saline. Dry plates were then stored at –80°C until use and harvested as described below.
Preparation of ribonucleic acids (RNA) and the real-time quantitative polymerase chain reaction (RT-PCR) of the GLP-2 receptor (GLP-2R)
Total cellular RNA was isolated following the method described by Chomczynski and Sacchi with slight modifications [32]. Briefly, the guanidinium isothiocyanate reagent was added to dry plates and, after scraping, the cell extract was passed 20 times through an insulin syringe and transferred to Eppendorf. Immediately, 50μl of 2M sodium acetate, 500μl of water saturated phenol, and 100μl of chloroform-isoamyl alcohol mixture (24:1) were added sequentially. After vigorous shaking, the aqueous phase was transferred to a new Eppendorf, 500μl of isopropanol was added, and the tube was kept at –20°C for at least 30 min. After centrifugation (15000 rpm, 4°C, 30 min) and careful elimination of the supernatant, 500μl guanidinium isothiocynate reagent and 500μl isopropanol were added to the RNA pellet. Again, the tube was kept at –20°C, centrifuged and the supernatant discarded. The sediment was resuspended in 1.4 ml of 80% ethanol and cooled at –20°C for at least 2 h. After a new centrifugation, the ethanol was removed. This last step was repeated one more time. Finally, the RNA pellet was dried at room temperature, suspended in sterile milliQ water, and stored at –80°C until use. Quantification was performed using a nucleic acid concentration microanalyzer (NanoDrop 2000, Thermo Scientific), and the integrity of the samples was evaluated on 1% (w/v) agarose gels. First-strand complementary DNA was synthesized from 1μg of total RNA using a commercial kit (RETROscript™, Cat No. 1710, Ambion®, Austin, TX, USA).
The polymerase chain reaction was performed with the TaqMan universal PCR master mix, using the gene expression assay (FAM-labeled TaqMan predesigned assay) following the standard protocol suggested by the supplier (Applied Biosystems, Warrington, UK). Real-time reverse transcription analysis for the GLP-2r gen (Rn 00573037_ml, Applied Biosystems) was performed starting with 75–110 ng of the reverse transcript of the total RNA. PCR was performed in a 96-well multiplate using the 7300 Real-Time PCR System (Applied Biosystems). The data were analyzed using 7300 System SDS software (version 1.2.3, 2004). The 2–ΔΔCt method was used to calculate the relative quantification of GLP-2 R mRNA levels. Cyclophilin A gen (Rn 00574762_ ml, Applied Biosystems) expression was assessed for normalizing the GLP-2 receptor expression. The results are expressed in relative units with respect to the control indicated in each figure, which has the value one.
Statistical analysis
Values are expressed as means±standard error of the means (SEM) and analyzed using one-way analysis of variance (ANOVA) or two-way ANOVA as appropriate. When the ANOVA was significant, individual differences were evaluated using Dunnet’s post hoc test to compare samples of equal size with a control or followed by Bonferroni or Tukey’s post hoc tests to compare samples of different sizes. The number of independent individual experiments and statistical significance are indicated in the figure legends. Only differences with adjusted p-values <0.05 were considered statistically significant.
RESULTS
To determine whether the expression of GLP-2R mRNA was dependent on cellular proliferation capacity, we grew cortical astrocytes at low density in DMEM/F-12, supplemented with 10% FBS. The content of GLP-2R mRNA was measured by RT-PCR at 7, 10, 14, and 17 days of incubation, which approximately corresponded with an optically evaluated degree of confluence of 50%, 65%, 80%, and 95%, respectively. Figure 1 shows that the expression peaked in the first assessment and decreased approximately linearly over the following days (F = 124.2; p < 0.0001), being approximately 50% lower on the 17th day of incubation, when the cells reached sub-confluence. Likewise, when we removed the FBS and incubated the cells only with DMEM/F-12 for a further 48 h, the GLP-2r mRNA expression decreased again in half, and compared to the value used as a reference (the first assessment), the expression was 75% lower. This last experimental condition was the starting point for the following studies.
Fig. 1
Relationship between the day of subculture of rat astrocytes and the expression of GLP-2R mRNA. Effect of FBS removal. Astrocytes were seeded on P-100 plates and grown for 7 (approximate 50% confluence), 10 (65%), 14 (80%), and 17 (95%) days in DMEM/F-12 (17.5 mM glucose) supplemented with 10% FBS, or 19 days (the last 48 h in the absence of FBS). At the end of the incubation period, total RNA was extracted from each plate as indicated in the experimental procedures, and GLP-2R mRNA expression was assessed by real-time quantitative PCR (RT-PCR). Data are presented as the mean±SEM (n = 6). **p < 0.01, ***p < 0.001, significant effect of time of subculture on gene expression, using 1-way ANOVA with Dunnet correction for comparisons with the first assessment (7 days). §§p < 0.01, significant effect on gene expression two days after FBS removal, using 2-way ANOVA with Bonferroni and Tukey corrections.
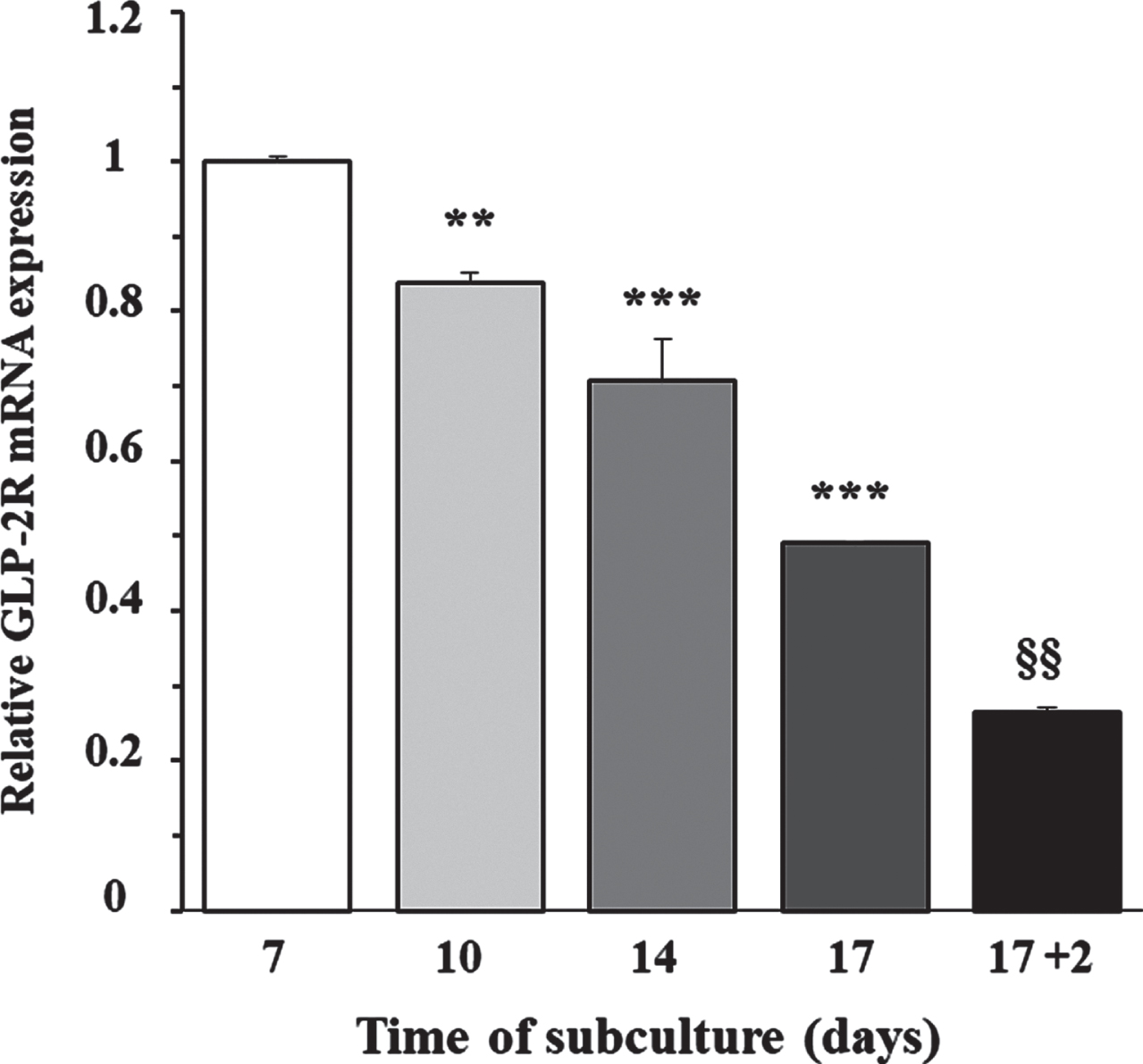
As shown in Fig. 2, glucose produced a significant increase on the GLP-2R mRNA expression (F = 38.0; p < 0.0001) at 4 h of incubation. The maximum effect was obtained with 17.5 mM glucose, being 2.3 times higher than with 1.4 mM (the concentration used as reference), and then decreased to 1.65 times with 25 mM glucose. The effect of glucose was also significant in cells incubated in the presence of 10 nM GLP-2 (F = 18.53; p < 0.0001), being maximum in those containing 5.6 and 12.5 mM of glucose and 1.9 times higher compared to those incubated with 1.4 mM. Likewise, our results show a significant interaction (F = 11.2; p < 0.0001) between the effects of glucose and those of GLP-2. Thus, the addition of GLP-2 increased (13%–40%) the GLP-2R mRNA content compared to their respective control cells that contained between 1.4 and 12.5 mM of glucose but was slightly lower (14%) in the presence of 17.5 mM, and with equal value when cells were incubated with 25 mM glucose.
Fig. 2
Effect of glucose concentration in the absence and in the presence of GLP-2 on the expression of GLP-2R mRNA in cultured rat astrocytes. Astrocytes were incubated for 4 h in serum-free DMEM medium, supplemented with 2 mg/mL BSA, containing the specified concentrations of glucose in the absence (∘) and the presence of 10 nM GLP-2 (•). GLP-2R mRNA was analyzed using real-time quantitative RT-PCR as indicated in the experimental procedures. Results are presented as the mean±SEM (n = 12 for the lowest glucose concentration; n = 6 for the others). ***p < 0.001, significant effect of glucose content on gene expression compared to 1.4 mM in the absence of GLP-2, and §p < 0.05; §§p < 0.01; §§§p < 0.001, significant effect of glucose content on gene expression compared to 1.4 mM in the presence of GLP-2, using 1-way ANOVA with Dunnet correction. #p < 0.05 (†p < 0.06), significant effect when comparing GLP-2 treated cells with untreated ones, using 2-way ANOVA with Bonferroni and Tukey corrections.
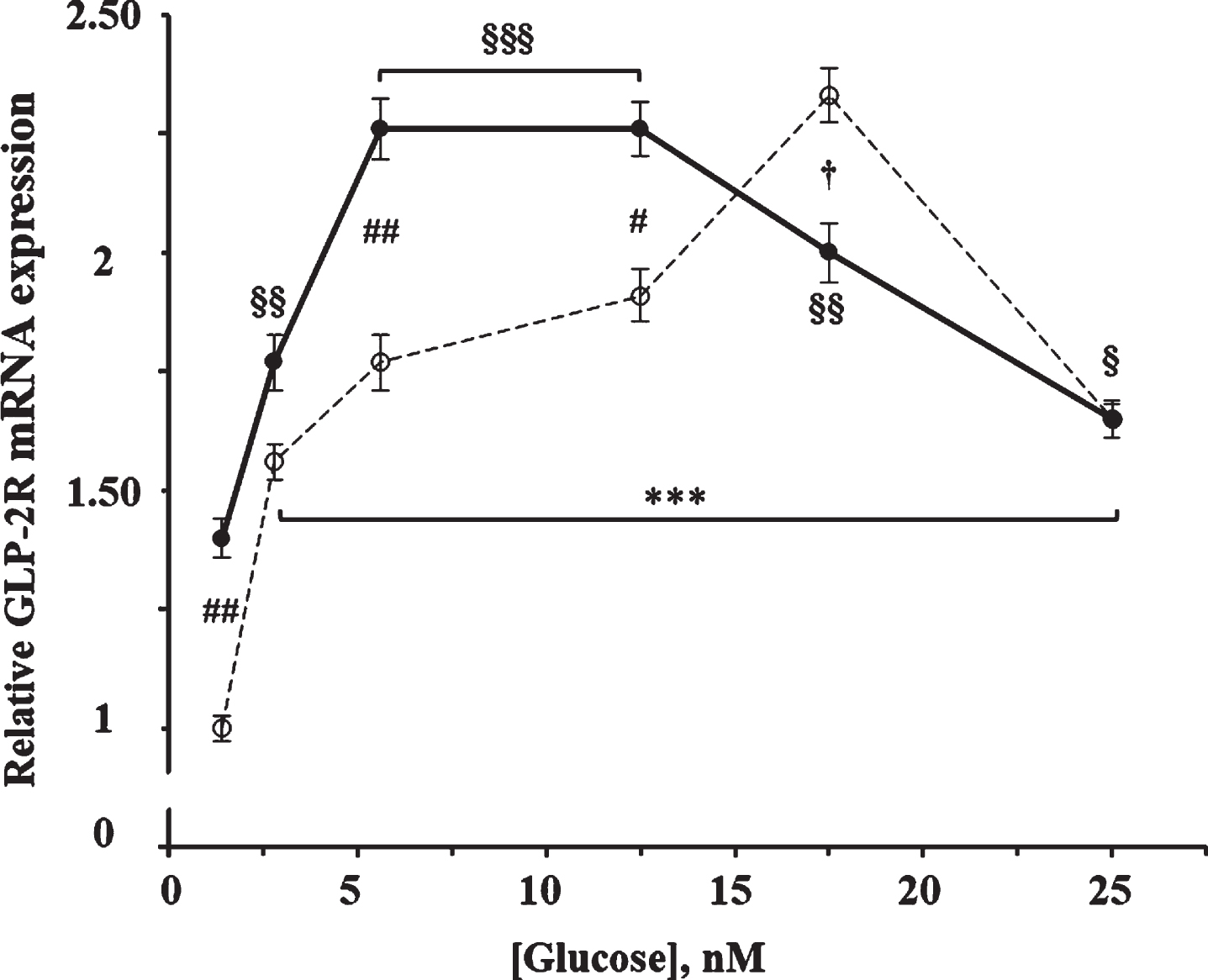
To check whether the effect of GLP-2 in the presence of 17.5 mM glucose was dependent on incubation time, we also performed stimulations at shorter and longer times. In fact, while 10 nM GLP-2 led to a 60% increase in GLP-2R mRNA expression at 30 min incubation (Fig. 3A), the transcript copy number was 24% lower than in control cells after 18 h of incubation (Fig. 3B).
Fig. 3
A) Effect of GLP-2 and insulin, PDGF, IGF-2, and NGF in the absence and presence of GLP-2 on the expression of GLP-2R mRNA in cultured rat astrocytes at 30 min of incubation in a medium containing high glucose concentration. B) Effect of GLP-2 and insulin, PDGF, IGF-1 and EGF in the absence and presence of GLP-2 at 18 h. Astrocytes were incubated in a serum-free DMEM/F-12 medium (17.5 mM glucose), supplemented with 2 mg/mL BSA (control), and 10 nM GLP-2 and with 100 nM insulin, 1 nM PDGF, 10 nM IGF-2, 1 nM NGF, 10 nM IGF-1, or 10 nM EGF for the times indicated, in the absence and in the presence of 50 nM GLP-2. The GLP-2R mRNA was analyzed using real-time quantitative RT-PCR as indicated in the experimental procedures. Results are presented as the mean±SEM (n = 6 in all of treatments). *p < 0.05 (†p < 0.06); **p < 0.01, significant effect of stimuli on gene expression compared to control cells, using 1-way ANOVA with Dunnet correction. §p < 0.05; §§p < 0.01, when comparing GLP-2 treated and untreated ones, using 1-way ANOVA with Bonferroni correction.
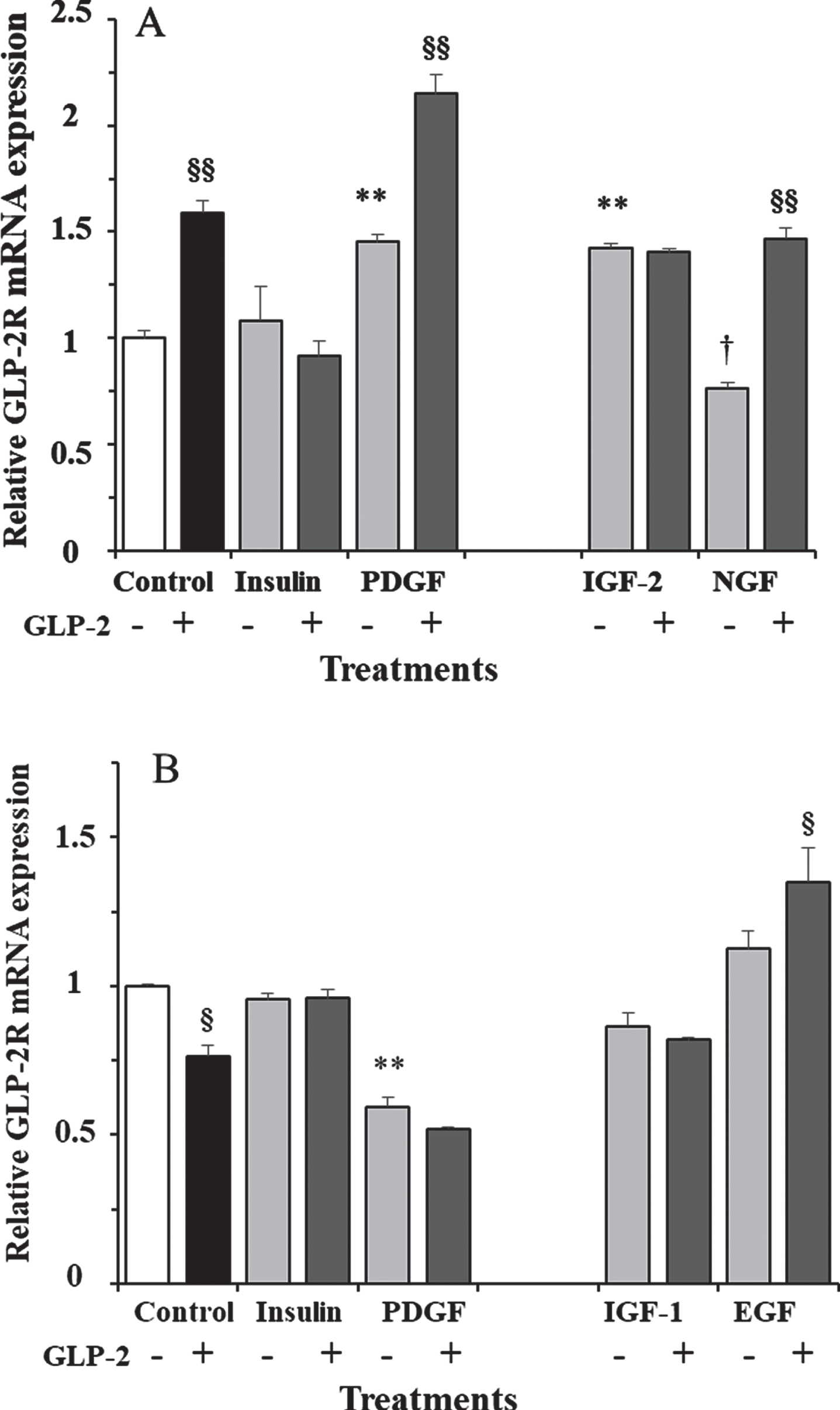
In addition to GLP-2, we also measured the effects of insulin and various growth factors alone or plus GLP-2 in the presence of 17.5 mM glucose. Significant differences were obtained both at 30 min (F = 31.5; p < 0.0001; Fig. 3A) and at 18 h (F = 61.04; p < 0.0001; Fig. 3B) of incubation. We studied the effects of insulin and PDGF at the two incubation times. As shown, insulin alone or plus GLP-2 did not modify GLP-2R expression at any of the assayed times. However, PDGF induced a 45% increase and 40% decrease at 30 min and 18 h of incubation, respectively. The addition of GLP-2 produced synergistic effects on the expression of GLP-2R mRNA, being 48% higher at 30 min and 13% lower, although not significant, at 18 h of incubation, compared to cells treated with PDGF alone. Compared to untreated cells (controls), expression was about two-fold and one-half, respectively.
We only measured the effects of IGF-2 and NGF at 30 min of incubation (Fig. 3A), and the expression of GLP-2R mRNA was 42% higher and 24% lower, respectively, compared to control cells. The addition of GLP-2 did not modify the effect of IGF-2, but it almost doubled the expression obtained with NGF alone, and it was 47% higher compared to untreated cells. On the other hand, the effects of IGF-1 and EGF were only estimated at 18 h of incubation (Fig. 3B) and were not significant (approximately 13% lower and higher, respectively) compared to vehicle-treated cells. As shown, the addition of GLP-2 produced the same trends, with expression being 5% lower and 20% higher compared to cells treated with IGF-1 and EGF alone, respectively. Compared to untreated cells, IGF-1 plus GLP-2 produced a non-significant 18% decrease, and EGF plus GLP-2 a significant 35% increase in GLP-2R mRNA content.
We determined the effects of leptin and NPY on the expression of GLP-2R mRNA at 4 h in the presence of 1.4 mM glucose. Figure 4 shows that while GLP-2 produced a 40% increase in the expression of its own receptor, the number of copies of GLP-2R mRNA was 46% and 70% lower in cells treated with leptin and NPY, respectively, compared to the untreated cells.
Fig. 4
Effect of GLP-2, leptin, and Neuropeptide Y on the expression of GLP-2R mRNA in cultured rat astrocytes at 4 h of incubation in a medium containing low glucose concentration. Astrocytes were incubated in a serum-free DMEM medium, containing 1.4 mM glucose, and supplemented with 2 mg/mL BSA (control, n = 12), and 10 nM GLP-2 (n = 12), 10 nM leptin (n = 6), or 100 nM NPY (n = 6) for 4 h. The GLP-2R mRNA was analyzed using real time quantitative RT-PCR as indicated in the experimental procedures. Data are presented as the mean±SEM. **p < 0.01; ***p < 0.001, significant effect of treatments on gene expression compared to control cells, using 1-way ANOVA with Bonferroni correction.
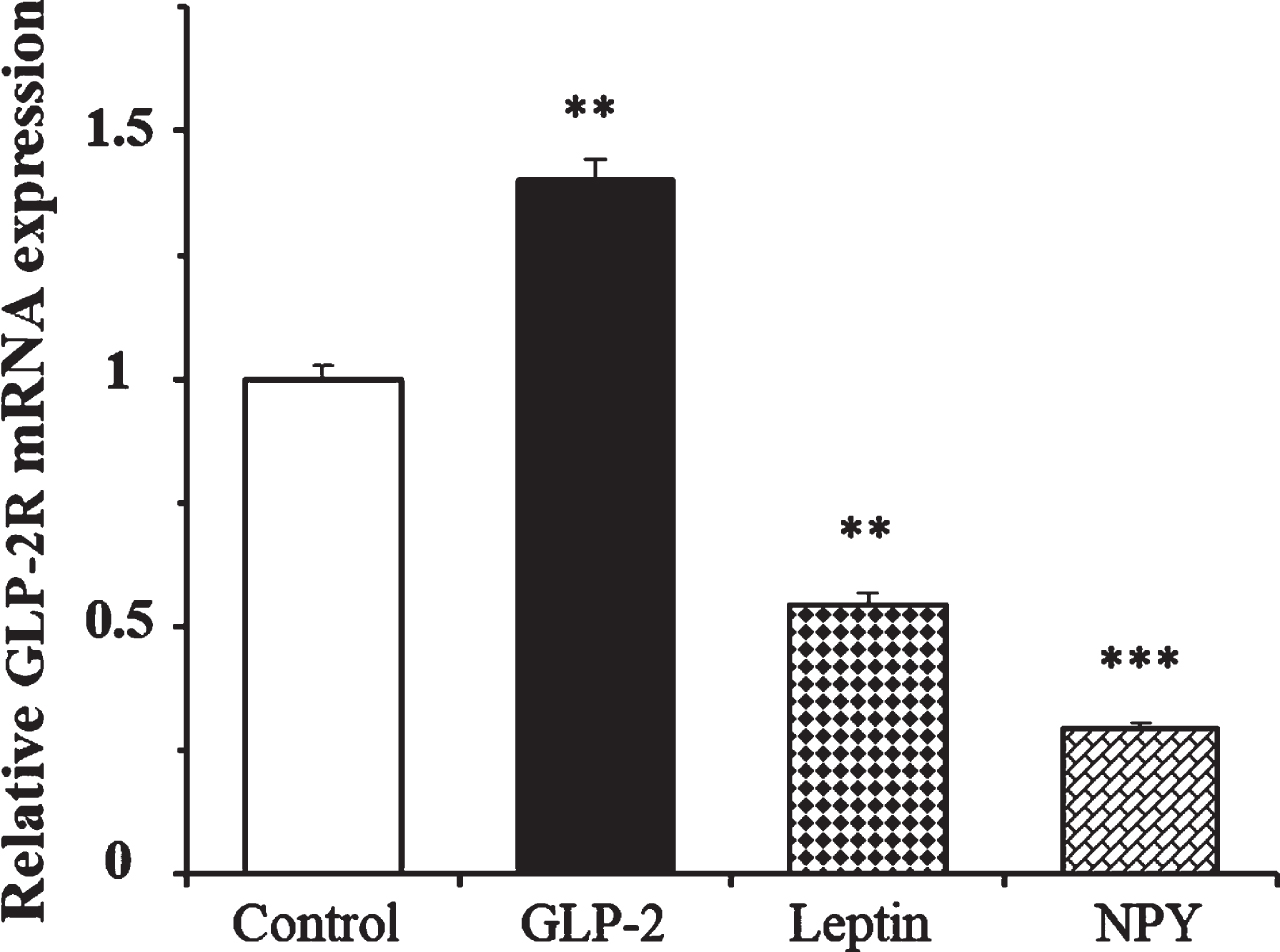
DISCUSSION
The copy number of GLP-2R gene transcript is dependent on the proliferative capacity of astrocytes in culture
Primary cultures of cortical astrocytes grown in a high-glucose medium containing FBS increase their cellular density until reaching confluence. The removal of the serum immediately decreases cell proliferative capacity followed by increased cell mortality. However, whether growth conditions had any effect on the GLP-2R mRNA expression is unknown. Our results clearly show that the copy number of the GLP-2r transcript decreases with the age of the subculture, reaching a minimum when cells were near the confluence, and still being lower two days after removing the serum. As there are no known data linking proliferation conditions and the regulation of GLP-2 receptor expression in isolated cells, we compare our results with those obtained in vivo. Thus, GLP-2R mRNA levels are higher in the bowels of the fetus and neonate of rats and pigs than in those from the adult specimens, which may be consistent with the lower proliferative capacity of the adult cells [33, 34].
Control of the GLP-2R mRNA expression by glucose and GLP-2 in cultured rat astrocytes
In addition to their involvement in transporting glucose from the circulation into the brain, astrocytes are also the main cells of the CNS responsible for synthetizing and storing glycogen, which can be later mobilized in response to decreases in local glucose levels and be metabolized until lactate, and thus providing neurons with an energy source [35].
GLP-2R expression depends on glucose levels, and the addition of GLP-2 produces different effects depending on glucose content but also of the incubation time. These results may be consistent with others we have previously reported [25], which show time-dependent differences in the hiFBS-induced GLP-2R expression in the presence of high glucose levels both with and without GLP-2. We believe that it is due to the fact that the GLP-2R transduced signaling is involved in many processes, including stimulating the proliferation and inhibition of apoptosis [17], repairing and protecting the CNS cells after certain mechanical and chemical injuries [18, 36, 37], and inducing memory improvement and mitigating depression symptoms [20]. Probably, GLP-2 may be involved in other as-yet unknown processes in astrocytes, thereby new studies should made to determine other roles of the GLP-2R in these cells.
The opposite effects of GLP-2 on the expression of its own receptor, depending on the concentration of nutrients (glucose) described here, are in agreement with those reported in vivo showing the effect of several nutrients, which increase GLP-2 circulating levels, on the GLP-2R expression. Thus, the expression was increased in the intestine of mice fed with an HFD [7], reduced in the small intestine of enteral fed pigs during development [38] and in the mucosal of total parenteral nutrition (TPN) mice [39], and not modified in the small intestine of rats with a pectin supplemented diet [40]. Likewise, in studies with different animal models, the intraperitoneal administration of GLP-2 also showed contradictory results. Thus, the GLP-2R expression was downregulated in the ileum of 21-day-old sows [41], in the mucosal of TPN-mice [39], and in the jejunum of rats with short bowel syndrome, but not in rats with a distal bowel resection [42]. Likewise, the GLP-2R expression was reduced in both the anastomotic segments and in the ileal samples recovered after ileal resection and isolated laparotomy of rats after teduglutide administration [11]. In contrast, the GLP-2R expression was increased in the cells of the crypts of mice with short bowel syndrome after treatment with a dipeptidylpeptidase inhibitor, which increases the half-life of GLP-2 [43]. However, no significant differences in GLP-2R expression were observed in the scraped mucosa from fed and unfed ileal segments of patients with planned loop ileostomy takedowns [39]. Although all these studies are in the gut, we believe that our results on the expression of GLP-2R in isolated astrocytes are very interesting because they seem to have some similarity with the results obtained in vivo. Given the importance that certain neuropeptides, including GLP-2, have in the control of food intake, our results may represent the first link of a new line of research.
The GLP-2R mRNA expression in astrocytes is modulated in a different manner by growth factors, hormones, and neuropeptides
There is currently no information on the effect that specific treatments have on the regulation of GLP-2R expression both in vivo and in vitro. Here, we have studied the effect of insulin, PDGF, IGF-1, IGF-2, EGF, leptin, and NPY because they induce the proliferation of astrocytes [25, 28, 44] or neural stem cells [45–47], as well as the effect of NGF, which attenuates cell proliferation [48]. In addition to those of GLP-2, the effects of insulin and PDGF alone and with GLP-2 were conducted at two different incubation times (30 min and 18 h), in the presence of 17.5 mM glucose. Interestingly, the effects of PDGF were very similar to those of GLP-2, increasing the expression in the short term, and decreasing it in the long term. However, PDGF plus GLP-2 only produced effects on the expression when treatment was performed for a short period of time. Under these experimental conditions, both IGF-2 and NGF plus GLP-2 also increased GLP-2R mRNA levels compared to untreated control cells. At 18 h, IGF-1, IGF-1 plus GLP-2, and EGF treatments had no effect, but EGF plus GLP-2 increased expression. Interestingly, we had previously reported that IGF-1 upregulated GLP-2R expression in cultured rat astrocytes, although under different experimental conditions [25]. Likewise, oral administration of EGF restored the GLP-2R expression in the mucosal cells of TPN-treated mice until levels of WT animals [39]. Finally, unlike the results obtained with GLP-2, in the presence of a low glucose concentration, both leptin and NPY inhibited GLP-2R expression at 4 h of incubation.
The GLP-2R mRNA expression data could have been strengthened with immunoblot data for this receptor, but the absence of a good antibody against GLP-2R has represented a limitation for the approach of this study.
It has been reported that lipopolysaccharide (LPS) slightly increases GLP-2R expression in the ileum of pigs, which returned to the levels of control animals in those previously treated with polyethylene glycosylated porcine GLP-2 [49]. This may be explained because the stable GLP-2 analog teduglutide inhibits the uptake and transport of LPS across the small intestinal barrier via the lipid raft and CD36-mediated mechanisms in the portal vein in vivo [50]. Likewise, treatment of isolated human islets for 24 h with LPS, which induces an increase in active GLP-2 secretion, produced a significant reduction in GLP-2R mRNA expression [15]. We have observed that LPS reduces the viability of cortical rat astrocytes in culture, which is dose-dependent reversed by the addition of GLP-2 (Fig. 5). These results suggest an inverse relationship between cell viability and GLP-2R expression, which may open a new way to study the role of GLP-2/GLP-2R in the brain.
Fig. 5
Effect of GLP-2, LPS, and LPS plus GLP-2 on the cellular density of cultured rat astrocytes. Astrocytes (in 24-well plates) were incubated in a serum-free DMEM/F-12 medium, supplemented with 2 mg/mL BSA, with the treatments indicated in the figure for 24 h. After the end of the incubation time, the reactions were stopped by removing the supernatants and immediately washing the cells three times with ice cold phosphate buffered saline. Then 250μL of 2 mg/mL crystal violet in 2% (v/v) ethanol was added to each well. After 1 h at room temperature, the cells were washed several times with distilled water, air-dried, and then eluted in 1% (w/v) SDS. Optical density at 560 nm was determined on 96-well multi-plates in a Varioskan Thermo Electron Corporation reader (Software ScanIt 2.0.91). Results are expressed as absorbance per well and presented as the mean±standard error of the means (n = 8). **p < 0.01; ***p < 0.005, significant effect of GLP-2 and LPS treatments on cell density compared to untreated cells. §p < 0.05; §§§p < 0.005, significant effect of LPS+GLP-2 compared to LPS alone. ###p < 0.005; ####p < 0.001, significant effect of LPS+GLP-2 compared to GLP-2 alone, using 1-way ANOVA with Tukey correction.
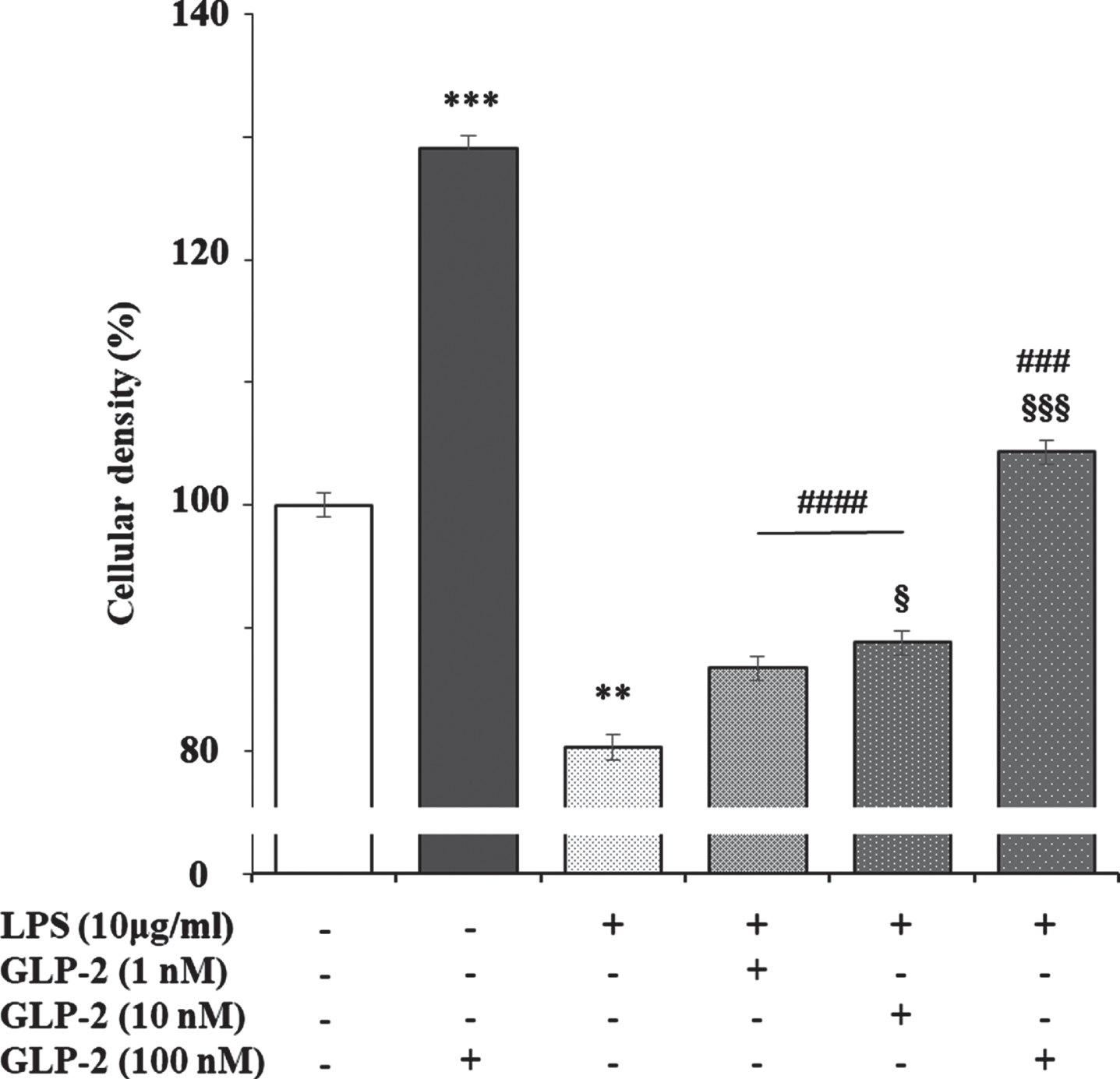
In conclusion, our results suggest that glucose, like certain hormones, growth factors, and neuropeptides, modulate GLP-2R expression in a time-dependent manner. These ligands, including GLP-2, play an important role in the CNS, which open a new insight for the study of the cerebral metabolism of glucose and other biomolecules in health and in certain neurodegenerative diseases, such as Alzheimer’s disease. However, more experimental work is required to elucidate the mechanisms involved in the control of GLP-2R expression in astrocytes. In addition, studying the role of GLP-2/GLP-2R in co-cultures of astrocytes and neurons could help us better understand the interactions of these two cell types in both normal and pathological situations.
ACKNOWLEDGMENTS
We are grateful to Dr. Francisco Javier Carricondo Orejana at the Department of Immunology, Ophthalmology and Otorhinolaryngology, Faculty of Medicine, Complutense University of Madrid, for providing the animals.
FUNDING
This work was funded by the E. Rodríguez Pascual (07.2018) and Ramón Areces (CIVP 1958173) Foundations.
CONFLICT OF INTEREST
The authors have no conflict of interest to report.
REFERENCES
[1] | Marathe CS , Rayner CK , Jones KL , Horowitz M ((2013) ) Glucagon-like peptides 1 and 2 in health and disease: A review. Peptides 44: , 75–86. |
[2] | Drucker DJ , Habener JF , Holst JJ ((2017) ) Discovery, characterization, and clinical development of the glucagon-like peptides. J Clin Invest 127: , 4217–4227. |
[3] | Drucker DJ , Yusta B ((2014) ) Physiology and pharmacology of the enteroendocrine hormone glucagon-like peptide-2. Annu Rev Physiol 76: , 561–583. |
[4] | Wismann P , Barkholt P , Secher T , Vrang N , Hansen HB , Jeppesen PB , Baggio LL , Koehler JA , Drucker DJ , Sandoval DA , Jelsing J ((2017) ) The endogenous preproglucagon system is not essential for gut growth homeostasis in mice. Mol Metab 6: , 681–692. |
[5] | Yusta B , Matthews D , Koehler JA , Pujadas G , Kaur KD , Drucker DJ ((2019) ) Localization of glucagon-like peptide-2 receptor expression in the mouse. Endocrinology 160: , 1950–1963. |
[6] | Khan D , Vasu S , Moffett RC , Irwin N , Flatt PR ((2017) ) Differential expression of glucagon-like peptide-2 (GLP-2) is involved in pancreatic islet cell adaptations to stress and beta-cell survival. Peptides 95: , 68–75. |
[7] | Baldassano S , Amato A , Cappello F , Rappa F , Mulè F ((2013) ) Glucagon-like peptide-2 and mouse intestinal adaptation to a high-fat diet. J Endocrinol 217: , 11–20. |
[8] | Rotondo A , Amato A , Baldassano S , Lentini L , Mulè F ((2011) ) Gastric relaxation induced by glucagon-like peptide-2 in mice fed a high-fat diet or fasted. Peptides 32: , 1587–1592. |
[9] | Fuchs S , Yusta B , Baggio LL , Varin EM , Matthews D , Drucker DJ ((2020) ) Loss of Glp2r signaling activates hepatic stellate cells and exacerbates diet-induced steatohepatitis in mice. JCI Insight 5: , 136907. |
[10] | Aoki K , Suzuki T , Hui F , Nakano T , Yanazawa K , Yonamine M , Fujita S , Sugasawa T , Yoshida Y , Omi N , Kawakami Y , Takekoshi K ((2021) ) Acute low-intensity treadmill running upregulates the expression of intestinal glucose transporters via GLP-2 in mice. Nutrients 13: , 1735. |
[11] | Costa BP , Gonçalves AC , Abrantes AM , Alves R , Matafome P , Seiça R , Sarmento-Ribeiro AB , Botelho MF , Castro-Sousa F ((2018) ) Tissular growth factors profile after teduglutide administration on an animal model of intestinal anastomosis. Nutr Hosp 35: , 185–193. |
[12] | Schoeler M , Klag T , Wendler J , Bernhard S , Adolph M , Kirschniak A , Goetz M , Malek N , Wehkamp J ((2018) ) GLP-2 analog teduglutide significantly reduces need for parenteral nutrition and stool frequency in a real-life setting. Therap Adv Gastroenterol 11: , 1756284818793343.1–1756284818793343.11. |
[13] | Li F , Lu L , Peng Y , Zhang Y , Gao J , Zhou D , Zhou D , Sheng H , Qu S ((2017) ) Increased GLP2R expression in gastric chief cells of patients with severe obesity regardless of diabetes status. Int J Obes (Lond) 41: , 1303–1305. |
[14] | Iacobellis G , Camarena V , Sant DW , Wang G ((2017) ) Human epicardial fat expresses glucagon-like peptide 1 and 2 receptors genes. Horm Metab Res 49: , 625–630. |
[15] | He W , Rebello OD , Henne A , Nikolka F , Klein T , Maedler K ((2021) ) GLP-2 Is locally produced from human islets and balances inflammation through an inter-islet-immune cell crosstalk. Front Endocrinol (Lausanne) 12: , 697120. |
[16] | Dubé PE , Brubaker PL ((2007) ) Frontiers in glucagon-like peptide-2: Multiple actions, multiple mediators. Am J Physiol Endocrinol Metab 293: , E460–E465. |
[17] | Velázquez E , Ruiz-Albusac JM , Blázquez E ((2003) ) Glucagon-like peptide-2 stimulates the proliferation of cultured rat astrocytes. Eur J Biochem 270: , 3001–3009. |
[18] | Amato A , Mulé F ((2019) ) Protective potential of glucagon like peptide 2 (GLP-2) against the neurodegeneration. Neural Regen Res 14: , 1901–1902. |
[19] | Guan X ((2014) ) The CNS glucagon-like peptide-2 receptor in the control of energy balance and glucose homeostasis. Am J Physiol Regul Integr Comp Physiol 307: , R585–R596. |
[20] | Iwai T , Jin K , Ohnuki T , Sasaki-Hamada S , Nakamura M , Saitoh A , Sugiyama A , Ikeda M , Tanabe M , Oka J ((2015) ) Glucagon-like peptide-2-induced memory improvement and anxiolytic effects in mice. Neuropeptides 49: , 7–14. |
[21] | Sasaki-Hamada S , Fujiwara A , Satoh S , Iwai T , Oka J-I ((2021) ) GLP-2 restores impairments in spatial working memory and hippocampal LTD via the MEK/ERK pathway in juvenile-onset diabetes rats. Behav Brain Res 406: , 113235. |
[22] | Sofroniew MV , Vinters HV ((2010) ) Astrocytes: Biology and pathology. Acta Neuropathol 119: , 7–35. |
[23] | Argente-Arizón P , Freire-Regatillo A , Argente J , Chowen JA ((2015) ) Role of non-neuronal cells in body weight and appetite control. Front Endocrinol (Lausanne) 6: , 42. |
[24] | Chowen J , de Fonseca F , Alvarez E , Navarro M , García-Segura L , Blázquez E ((1999) ) Increased glucagon-like peptide-1 receptor expression in glia after mechanical lesion of the rat brain. Neuropeptides 33: , 212–215. |
[25] | Velázquez E , Blázquez E , Ruiz-Albusac JM ((2009) ) Synergistic effect of glucagon-like peptide 2 (GLP-2) and of key growth factors on the proliferation of cultured rat astrocytes. Evidence for reciprocal upregulation of the mRNAs for GLP-2 and IGF-I receptors. Mol Neurobiol 40: , 183–193. |
[26] | Mansur RB , Fries GR , Trevizol AP , Subramaniapillai M , Lovshin J , Lin K , Vinberg M , Ho RC , Brietzke E , McIntyre RS ((2019) ) The effect of body mass index on glucagon-like peptide receptor gene expression in the post mortem brain from individuals with mood and psychotic disorders. Eur Neuropsychopharmacol 29: , 137–146. |
[27] | Luo J , Miller MW ((1999) ) Platelet-derived growth factor-mediated signal transduction underlying astrocyte proliferation: Site of ethanol action. J Neurosci 19: , 10014–10025. |
[28] | Velázquez E , Santos A , Montes A , Blázquez E , Ruiz-Albusac JM ((2006) ) 25-Hydroxycholesterol has a dual effect on the proliferation of cultured rat astrocytes. Neuropharmacology 51: , 229–237. |
[29] | Fuente-Martín E , García-Cáceres C , Granado M , de Ceballos ML , Sánchez-Garrido MÁ , Sarman B , Liu Z-W , Dietrich MO , Tena-Sempere M , Argente-Arizón P , Díaz F , Argente J , Horvath TL , Chowen JA ((2012) ) Leptin regulates glutamate and glucose transporters in hypothalamic astrocytes. J Clin Invest 122: , 3900–3913. |
[30] | Ruiz-Albusac JM , Velázquez E , Iglesias J , Jimenez E , Blázquez E ((1997) ) Insulin promotes the hydrolysis of a glycosyl phosphatidylinositol in cultured rat astroglial cells. J Neurochem 68: , 10–19. |
[31] | Velázquez E , Blázquez E , Ruiz-Albusac JM ((2012) ) Glucagon-like peptide-2 (GLP-2) modulates the cGMP signalling pathway by regulating the expression of the soluble guanylyl cyclase receptor subunits in cultured rat astrocytes. Mol Neurobiol 46: , 242–250. |
[32] | Chomczynski P , Sacchi N ((1987) ) Single-step method of RNA isolation by acid guanidinium thiocyanate-phenol-chloroform extraction. Anal Biochem 162: , 156–159. |
[33] | Lovshin J , Yusta B , Iliopoulos I , Migirdicyan A , Dableh L , Brubaker PL , Drucker DJ ((2000) ) Ontogeny of the glucagon-like peptide-2 receptor axis in the developing rat intestine. Endocrinology 141: , 4194–4201. |
[34] | Petersen YM , Burrin DG , Sangild PT ((2001) ) GLP-2 has differential effects on small intestine growth and function in fetal and neonatal pigs. Am J Physiol Regul Integr Comp Physiol 281: , R1986–R1993. |
[35] | Douglass JD , Dorfman MD , Thaler JP ((2017) ) Glia: Silent partners in energy homeostasis and obesity pathogenesis. Diabetologia 60: , 226–236. |
[36] | Topaloğlu N , Memi G , Kaner T , Deniz M , Şahin Ö , Güven M , Çoşar M ((2015) ) Does Glp-2 have a protective effect on cerebral ischemia/reperfusion model? Turk J Med Sci 45: , 467–473. |
[37] | Lovshin J , Huang Q , Seaberg R , Brubaker P , Drucker D ((2004) ) Extrahypothalamic expression of the glucagon-like peptide-2 receptor is coupled to reduction of glutamate-induced cell death in cultured hippocampal cells. Endocrinology 145: , 3495–3506. |
[38] | Petersen YM , Hartmann B , Holst JJ , Le Huerou-Luron I , Bjørnvad CR , Sangild PT ((2003) ) Introduction of enteral food increases plasma GLP-2 and decreases GLP-2 receptor mRNA abundance during pig development. J Nutr 133: , 1781–1786. |
[39] | Feng Y , Demehri FR , Xiao W , Tsai Y-H , Jones JC , Brindley CD , Threadgill DW , Holst JJ , Hartmann B , Barrett TA , Teitelbaum DH , Dempsey PJ ((2017) ) Interdependency of EGF and GLP-2 signaling in attenuating mucosal atrophy in a mouse model of parenteral nutrition. Cell Mol Gastroenterol Hepatol 3: , 447–468. |
[40] | Fukunaga T , Sasaki M , Araki Y , Okamoto T , Yasuoka T , Tsujikawa T , Fujiyama Y , Bamba T ((2003) ) Effects of the soluble fibre pectin on intestinal cell proliferation, fecal short chain fatty acid production and microbial population. Digestion 67: , 42–49. |
[41] | Wu J , Qi KK , Xu ZW ((2016) ) Porcine glucagon-like peptide-2 microspheres ameliorate inflammation in lipopolysaccharide-challenged weaning piglets. J Anim Sci 94: , 5286–5294. |
[42] | Lai SW , de Heuvel E , Wallace LE , Hartmann B , Holst JJ , Brindle ME , Chelikani PK , Sigalet DL ((2017) ) Effects of exogenous glucagon-like peptide-2 and distal bowel resection on intestinal and systemic adaptive responses in rats. PLoS One 12: , e0181453. |
[43] | Sueyoshi R , Woods Ignatoski KM , Okawada M , Hartmann B , Holst J , Teitelbaum DH ((2014) ) Stimulation of intestinal growth and function with DPP4 inhibition in a mouse short bowel syndrome model. Am J Physiol Gastrointest Liver Physiol 307: , G410–G419. |
[44] | Martín R , Cordova C , Gutiérrez B , Hernández M , Nieto ML ((2017) ) A dangerous liaison: Leptin and sPLA2-IIA join forces to induce proliferation and migration of astrocytoma cells. PLoS One 12: , e0170675. |
[45] | Rodrigo C , Zaben M , Lawrence T , Laskowski A , Howell OW , Gray WP ((2010) ) NPY augments the proliferative effect of FGF2 and increases the expression of FGFR1 on nestin positive postnatal hippocampal precursor cells, via the Y1 receptor. J Neurochem 113: , 615–627. |
[46] | Bracko O , Singer T , Aigner S , Knobloch M , Winner B , Ray J , Clemenson GD , Suh H , Couillard-Despres S , Aigner L , Gage FH , Jessberger S ((2012) ) Gene expression profiling of neural stem cells and their neuronal progeny reveals IGF2 as a regulator of adult hippocampal neurogenesis. J Neurosci 32: , 3376–3387. |
[47] | Erlandsson A , Brännvall K , Gustafsdottir S , Westermark B , Forsberg-Nilsson K ((2006) ) Autocrine/paracrine platelet-derived growth factor regulates proliferation of neural progenitor cells. Cancer Res 66: , 8042–8048. |
[48] | Cragnolini AB , Huang Y , Gokina P , Friedman WJ ((2009) ) Nerve growth factor attenuates proliferation of astrocytes via the p75 neurotrophin receptor. Glia 57: , 1386–1392. |
[49] | Qi K , Wu J , Deng B , Li Y , Xu Z ((2015) ) PEGylated porcine glucagon-like peptide-2 improved the intestinal digestive function and prevented inflammation of weaning piglets challenged with LPS. Animal 9: , 1481–1489. |
[50] | Akiba Y , Maruta K , Takajo T , Narimatsu K , Said H , Kato I , Kuwahara A , Kaunitz JD ((2020) ) Lipopolysaccharides transport during fat absorption in rodent small intestine. Am J Physiol Gastrointest Liver Physiol 318: , G1070–G1087. |