Explore the Mechanism of β-Asarone on Improving Cognitive Dysfunction in Rats with Diabetic Encephalopathy
Abstract
Background:
The number of people with diabetes is increasing, and many patients have significantly impaired cognitive function. For patients with diabetic encephalopathy (DE), simply lowering blood sugar does not improve learning and memory. Studies have shown that β-asarone can significantly improve cognitive impairment in patients with DE, but the specific mechanism of action is unclear.
Objective:
This experiment hopes to use a variety of experimental methods to clarify the protective effect and mechanism of β-asarone on brain neurons during the development of DE disease.
Methods:
A high-sugar and high-fat diet and streptozotocin injection-induced DE rat model was used. β-asarone was administered for four weeks. The experiment used the Morris water maze test, biochemical index detection, and many methods to evaluate the neuroprotective effect of β-asarone on DE rats from various aspects and understand its mechanism.
Results:
β-asarone reduced neuronal cell damage and significantly improved the learning and memory ability of DE rats. In addition, β-asarone can reduce the oxidative stress response and amyloid-β accumulation in the brain of DE model rats and increase the content of brain-derived neurotrophic factor (BDNF) in the brain tissue, thereby reducing neuronal cell apoptosis and playing a protective role.
Conclusion:
β-asarone can reduce the accumulation of oxidative stress and amyloid-β in the brain, increase the content of BDNF, reduce the apoptosis of neuronal cells, and exert neuronal protection, thereby improving the learning and memory ability of DE model rats.
INTRODUCTION
Diabetes is a metabolic disorder resulting from a defect in insulin production, secretion, resistance in surrounding tissues, or all of these. One of its characteristics is the high level of glucose in the blood [1]. Type 1 and 2 are the two types, with the prevalence of type 2 accounting for the majority of diabetes [2]. With the changes in people’s eating habits in China, the incidence of diabetes is increasing year by year, and its age group is gradually becoming younger [3]. Long-term high blood sugar in the body can cause damage to many tissues and organs of the human body, including the feet [4], kidney [5], skeleton [6], etc. The existing literature on diabetes complications is extensive and focuses particularly on damage to the central nervous system called diabetic encephalopathy (DE); it can cause cognitive decline, learning and memory deficits, a lack of attention, and increase the risk of developing Alzheimer’s disease (AD) [7]. With the fast-paced development of society, this long-term increasingly serious complication is a huge mental pressure for patients and their families. In recent years, with the emphasis on DE, research has gradually deepened, and it has been found that the incidence of DE is affected by many factors, such as alterations in the brain structure, electrophysiological deficits, and so on. Although some potential mechanisms have been proposed, there is still a big knowledge gap in identifying protein levels and molecular mechanisms of DE.
As we know, brain hyperglycemia can cause hyperglycemia, oxidative stress, inflammation, and damage brain neurons. Previous research has established that long-term exposure to a high-glycemic environment will cause the death of brain neurons, which will affect the cognitive function of the patient and form DE [8]. In addition, studies have shown that insulin resistance in diabetic patients can lead to the accumulation of amyloid-β (Aβ), which continuously accumulates to form plaques through the formation of oligomers and fibers, thereby aggravating neurotoxicity and damage to the brain [7, 9]. And Aβ can also induce neuronal death by increasing oxidative stress, and oxidative stress will also increase the production of Aβ, causing more oxidative damage [10, 11]. During the occurrence of DE, the brain is more susceptible to oxidative stress than other organs. Most of the components of neurons (lipid, protein, and nucleic acid) may be oxidized, thereby promoting the apoptosis of related neurons [12]. The brain-derived neurotrophic factor (BDNF) is the most prominent neurotrophic factor in the human body, and it plays an important role in neuronal development, survival, and synaptic plasticity [13]. It is now well established from a variety of studies that BDNF plays a critical role in learning and memory by acting on hippocampal long-term potentiation [14].
β-asarone is an effective active ingredient in the traditional Chinese medicine acorus tatarinowii. It belongs to the volatile oil component and has a wide range of biological activities. Studies have shown that it can reduce the destruction of Schwann cells after peripheral nerve injury, accelerate their proliferation, and have significant pharmacological effects on the central nervous system [15]. In addition, β-asarone can also exert neuroprotective effects on AD patients in a variety of ways [16, 17]. β-asarone also showed a good therapeutic effect in depression model rats. It can significantly reduce the apoptosis rate of rat hippocampal neurons and increase the levels of BDNF and Bcl-2 in the hippocampus of rats [18]. However, we do not know much about whether β-asarone can play a protective effect on neurons and improve the cognitive dysfunction of DE rats through a variety of adjustment methods.
Accordingly, in this study, we used an animal model of type 2 diabetes and focused on the mechanism of action of β-asarone in improving DE to investigate whether β-asarone improves cognitive dysfunction by reducing Aβ deposition-mediated oxidative stress and increasing BDNF levels to reduce neuronal cell apoptosis. We found that β-asarone exerts neuron protection in multiple ways that might be an effective mechanism in DE model mice. The entire experimental process is shown in Fig. 1.
Fig. 1
The diagram for animal experimental design.
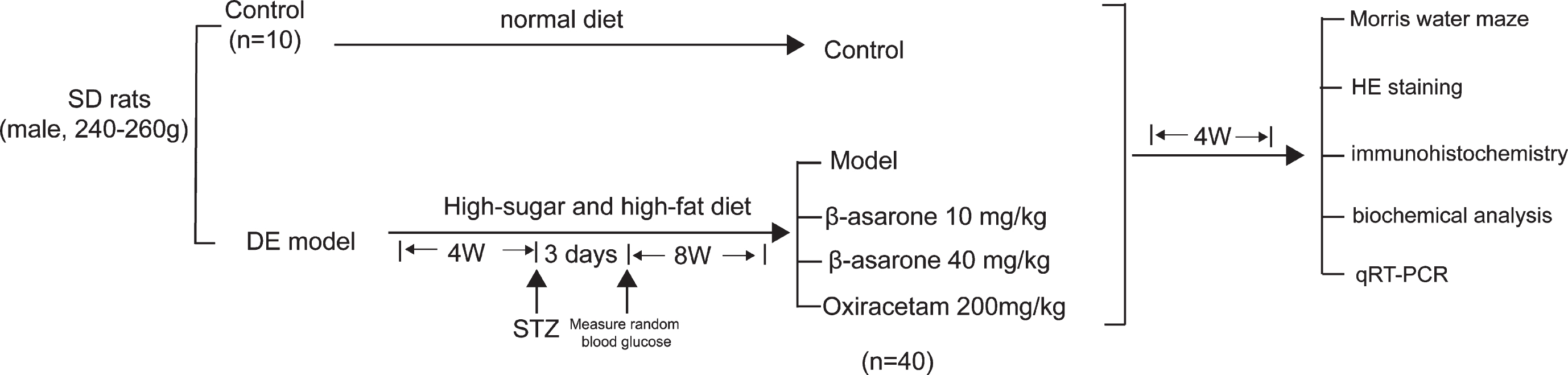
METHODS
Establishment of DE model
There were 200 SPF male SD rats, weighing between 240–260 g, provided by Jinan Pengyue Experimental Animal Breeding Co., Ltd. (Certificate: SCXK (LU)20190003) and allowed free access to food and water. All rats were randomly divided into 10 rats as the control group, and they were fed with ordinary basic diet. The remaining 190 rats were given a high-sugar and high-fat diet (10% lard, 10% sugar, 2.5% cholesterol, 77.5% basic diet as a model) and fed for 4 weeks, while recording the body weight and blood glucose levels. After 4 weeks, 190 rats were given a one-time intraperitoneal injection of streptozotocin (STZ) (purity > 98%, Germany Biofrxx, EZ34148220) 35 mg/kg. After 3 days, blood was collected from the tail vein of all rats to measure random blood glucose. If the blood glucose value was higher than 16.7 mmol/L, the diabetic rat model was established successfully, and the rest were eliminated. Then the weight and blood glucose of the rats that successfully established the diabetes model were continually monitored for 8 weeks. The Morris water maze was used to observe the rats’ learning and memory abilities to determine whether the DE model was successfully established.
Grouping and treatment
The rats that successfully established the DE model were randomly divided into model group (purified water), β-asarone low-dose group (10 mg/kg), β-asarone high-dose group (40 mg/kg), and Olacetam capsule positive drug group (200 mg/kg). Since β-asarone (purity≥95%, Hubei widelychemical, 2019121603) is a lipophilic compound, β-asarone was dissolved in 1% sodium carboxymethyl cellulose (CMC-Na), and it was intragastric introduced to rats after conversion of the dose. There were 10 rats in each group. Ten normal male rats were taken as the control group and were given purified water intragastrically once a day. During this period, all rats were allowed to drink and eat freely for 4 consecutive weeks.
Morris water maze
The positioning navigation experiment was carried out for 4 consecutive days, choosing 4 water entry points outside the platform quadrant (different every day), and gently putting the animal into the water facing the pool wall during the experiment, without disturbing the water surface, recording the rat from entering the water to climbing. The time elapsed on the platform is the incubation period of escape, and then letting the rats stay on the platform for 15 s. If the platform was not found within 120 s, the incubation period of absconding was recorded as 120 s, and the rat was placed on the platform to rest for 15 s. The arithmetic mean of the 4 absconding incubation periods was used as the score of this day, and statistical analysis was carried out. The absconding incubation period represents the spatial learning ability of rats. One day after the experiment, the platform was removed for space exploration experiments. The rat was put into the water from the quadrant opposite to the quadrant where the platform was located, the swimming path of the rat in the pool within 120 s after entering the water was recorded, observing whether the rat can enter the target area, and evaluating the memory ability of the rat [19].
Hematoxylin-eosin (HE) staining
Rat hippocampal tissues were fixed in 4% paraformaldehyde, and each group of tissues was put into a dehydration box and numbered. They were then dehydrated with different concentrations of alcohol and waxed with xylene. Then the tissue was embedded, sliced, and dried. For HE staining, the dried tissue sections were deparaffinized and washed with different concentrations of alcohol, then stained and dehydrated with hematoxylin and eosin dyes, finally mounted with neutral gum, dried, and observed.
Immunohistochemistry
Dried hippocampal tissue sections were separately taken to be deparaffinized and washed with water, then heat repaired and treated with 3% hydrogen peroxide deionized water. The treated sections were incubated with primary and secondary antibodies respectively. Diaminobenzidine method (DAB) was used for color development, and finally hematoxylin was used for re-staining and mounting the film for observation. Anti-mouse/rabbit two-step detection kit (Ebiogo, 11162110), BDNF antibody (Abcam GR3227037-1), and Aβ antibody (Abcam GR3235744-9) were used.
Rat serum and brain tissue homogenate biochemical analysis
Rat serum and brain tissue homogenate was taken for biochemical analysis. Low-density lipoprotein (LDL-C) (Shanghai zhenke 201906211), triglyceride (TG) (Shanghai zhenke 201907311), and total cholesterol (T-CHO) (Shanghai zhenke 201906511), high-density lipoprotein (HDL-C) (Shanghai zhenke 201911271), lipid peroxide (LPO) (Nanjing jiancheng, 20190718) content, and nitric oxide synthase (NOS) (Nanjing jiancheng, 20190711) activity were determined by content test kit, according to the manufacturer’s instructions.
Total RNA extraction and qRT-PCR analysis
After weighing 50–100 mg of rat hippocampal tissue and grinding with liquid nitrogen, 1 ml of TRIzol homogenate (Life Technologies, 204403) and chloroform (Shanghai Suyi Chemical Reagent Co., Ltd., 20191013) were added to extract RNA. Afterwards, the extracted RNA was subjected to RT reaction, and cDNA Synthesis Kit (Thermo Scientific, 00691399) was used to perform reverse transcription to obtain cDNA. Finally, taking cDNA as a template for fluorescence quantification, and performing a fluorescence quantitative PCR reaction. β-actin was used as internal control, with the 2–ΔΔCt values normalized to β-actin levels. Primer sequences for qRT-PCR used in this study are provided below: Bax (forward primer, 5′-ACTAAAGTGCCCGAGCTGAT-3′, reverse primer: 5′-ATGGTCACTGTCTGCCATGT-3′), Bcl-2 (forward primer, 5′-GAGTACCTGAACCGGCATCT-3′, reverse primer: 5′-GAAATCAAACAGAGGTCGCA-3′).
RESULTS
Effect of β-asarone on random blood glucose and body weight of DE model rats
The most obvious feature in the development of diabetes is not to drink and eat but to lose weight. With the construction of the model experiment, the weight of the DE model rat dropped rapidly, but its blood sugar rose rapidly. In addition, we grouped the DE model rats after the 12th week and found that the body weight and blood sugar of the rats were not significant compared with the model group, regardless of the low-dose β-asarone group or the high-dose β-asarone group. The difference is shown in Fig. 2. These results indicate that in the course of DE disease, β-asarone does not significantly improve body weight and blood sugar.
Fig. 2
Changes in body weight and blood glucose of DE model rats. Weights (A), random blood glucose level (B). Data was expressed as mean±SD. N = 10. Compared with control group: *p < 0.05, **p < 0.01; compared with Model group: #p < 0.05, # #p < 0.01.
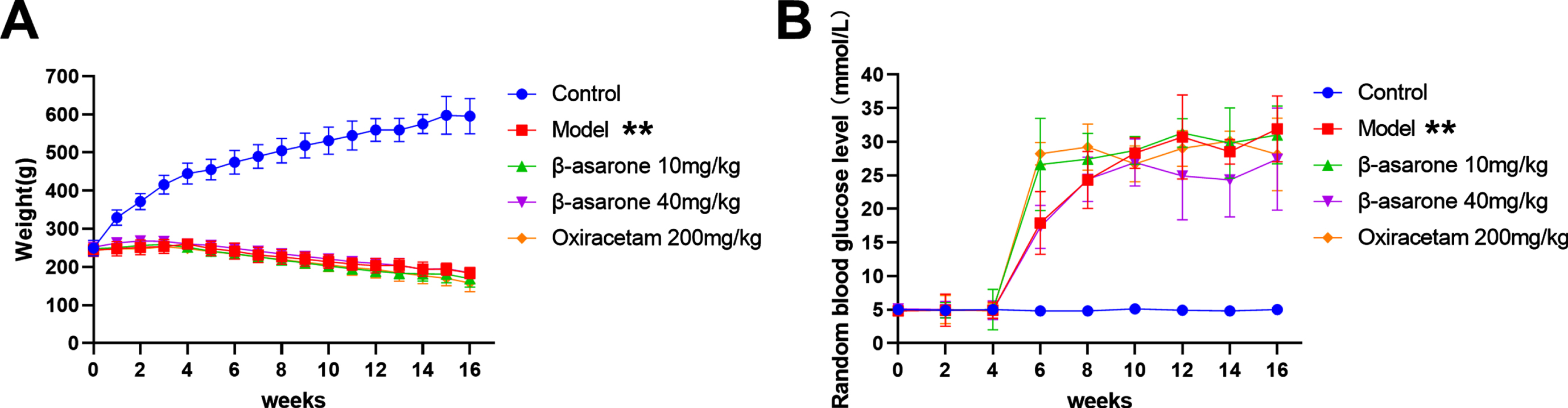
The effect of β-asarone on the learning and memory ability of DE model rats
Due to the long-term high blood sugar environment, the neurons of DE model rats are damaged due to a series of internal reactions, and their external performance is the lack of learning and memory ability. Morris water maze was used to evaluate the cognitive function of DE model rats. The spatial learning ability of DE model rats was judged by recording the time when the rats found a platform in the water within a limited time. The experimental results show that compared with the control group of rats, the time it taken for the model group to find the platform was significantly increased. After the DE rats were treated with β-asarone, the time taken for them to find a platform was significantly shorter than that of the model group, especially in the β-asarone high-dose group, as shown in Fig. 3A. After that, the experiment removed the underwater platform and observed the path of DE rats to the designated area. We found that compared with the control group of rats, the path of the model group rats was more complicated and could not reach the designated area within the specified time. Compared with the model group, after β-asarone drug intervention treatment, the road map showed that DE rats bypassed the target area significantly less and could find the designated area faster, as shown in Fig. 3B. After β-asarone intervention treatment, the rats of DE exhibited better performance than the model group. These results show that β-asarone can significantly improve the learning and cognitive ability of DE rats, and the high-dose β-asarone group is better than the low-dose group.
Fig. 3
β-asarone improves learning and memory impairment in DE rats. Escape latency of rats (A) and swimming paths in different groups (B). Data was expressed as mean±SD. N = 10. Compared with control group: *p < 0.05, **p < 0.01; compared with Model group: #p < 0.05, # #p < 0.01.
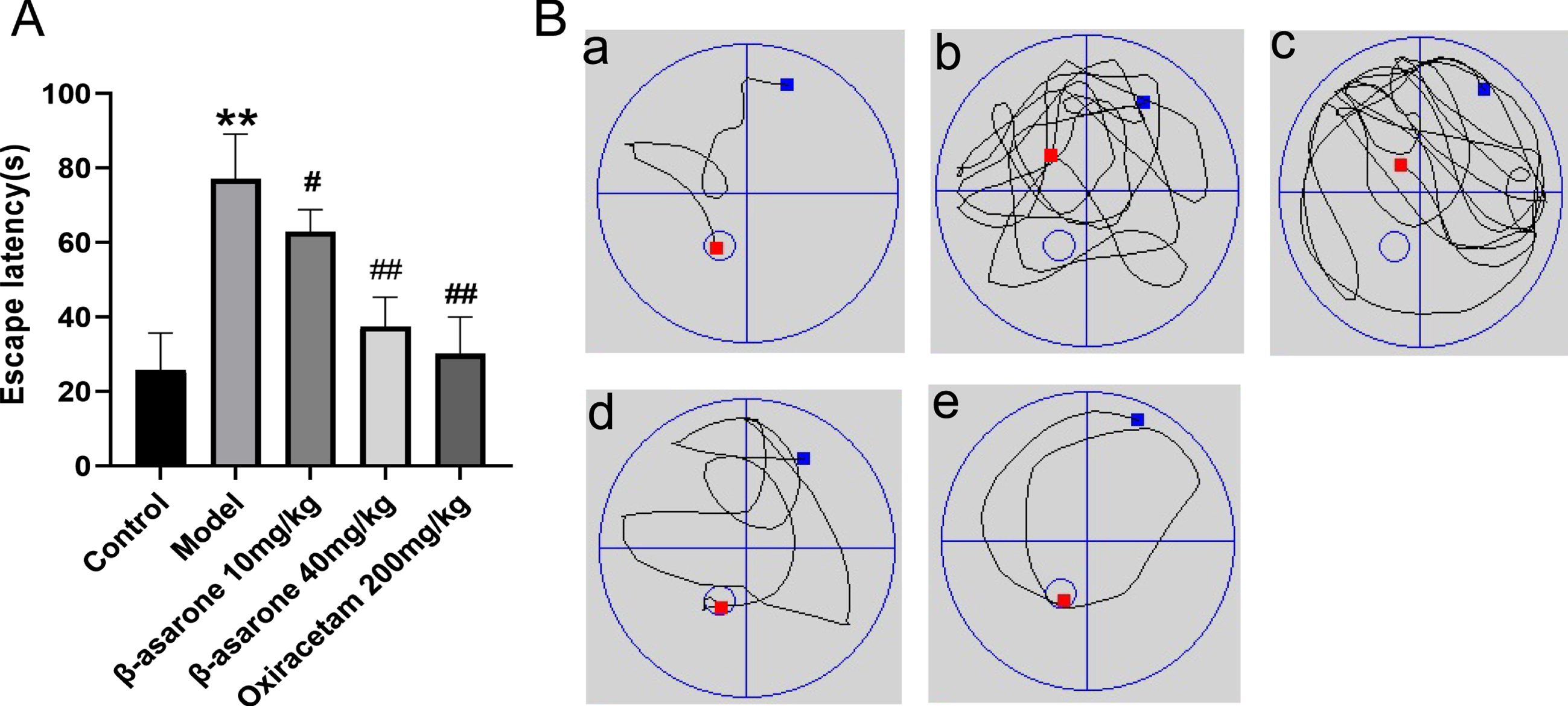
The effect of β-asarone on related indexes in DE rats’ serum
DE model rats have long-term consumption of high-sugar and high-fat feeds and are in a state of high blood sugar, and their blood lipids and other related indicators will also change. The detection of four indicators of serum lipids in different groups of rats found that compared with the control group of rats, the model group’s LDL-C, TG, and T-CHO was significantly increased. After β-asarone administration, this phenomenon was reversed. Compared with the model group, the three indicators of β-asarone administration group were significantly decreased, and the effect of β-asarone high-dose group was better, as shown in Fig. 4A-C. However, different groups expressed the opposite in terms of HDL-C content as shown in Fig. 4D. Compared with the control group, the HDL-C content of rats in the model group was significantly reduced. The HDL-C content of DE rats in the administration group was significantly increased, compared with the model group. Therefore, the results show that the intervention treatment of β-asarone can reduce the content of LDL-C, TG, and T-CHO in DE rats, and increase the content of HDL-C, thereby improving the blood lipid level of DE rats.
Fig. 4
The effect of β-asarone on LDL-C (A), HDL-C (B), TG (C), T-CHO (D), insulin (E) in DE rats. Data was expressed as mean±SD. N = 10. Compared with control group: *p < 0.05, **p < 0.01; compared with Model group: #p < 0.05, # #p < 0.01.
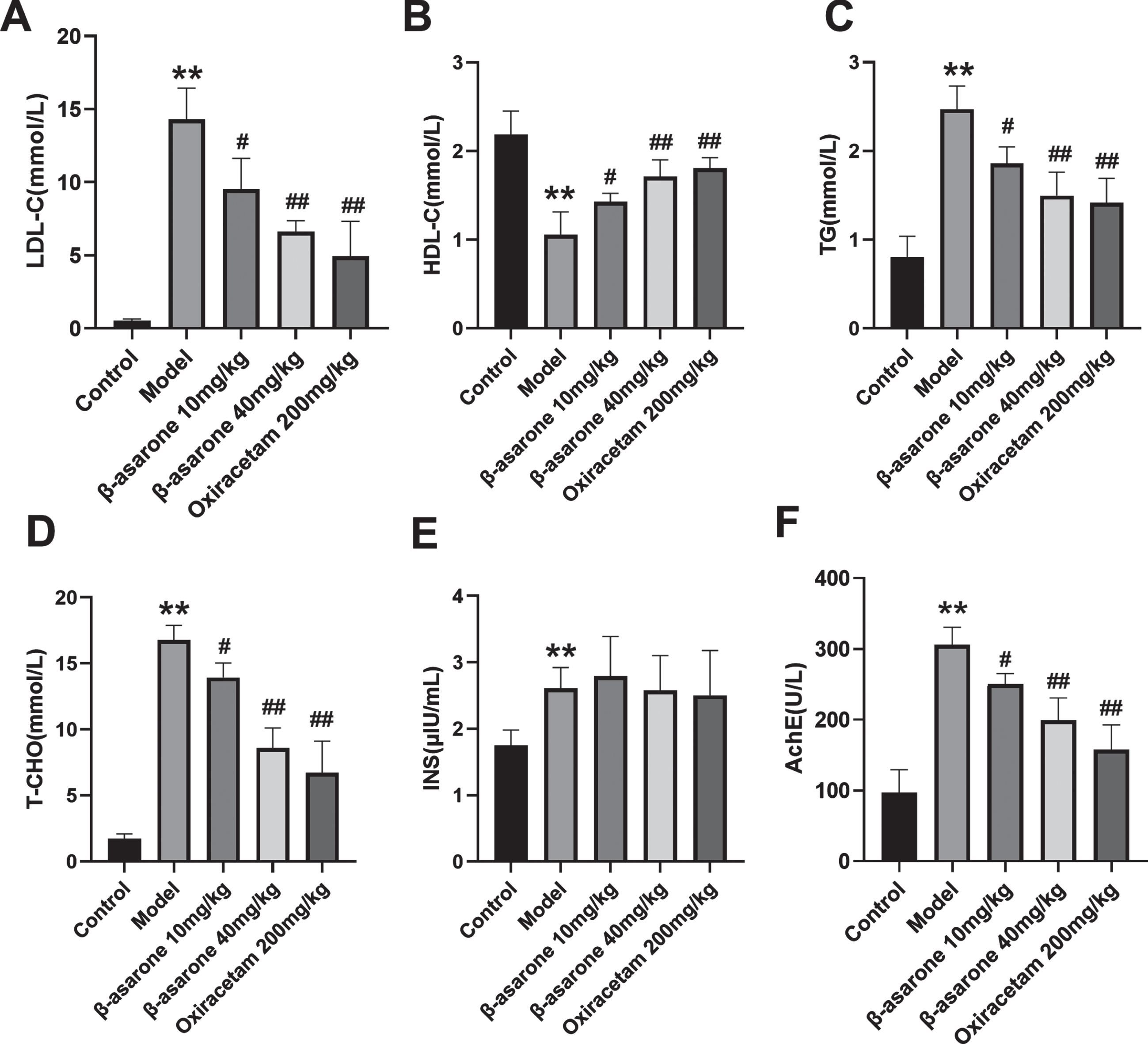
The experiment also tested the content of serum insulin in DE rats. The results showed that compared with the control group, the serum insulin of the model group was significantly increased, but there was no significant difference between the administration group and the model group, as shown in Fig. 4E. In addition, β-asarone in the serum of DE rats can inhibit the activity of acetylcholinesterase (AChE) in DE rats in a dose-dependent manner, as shown in Fig. 4F.
β-asarone exerts a protective effect on the neurons of DE rats
Since the hippocampus is essential in learning and memory, we next examined changes to the neurons in DE rats. Neuronal changes were investigated in control group and model group in the CA1 and CA3 regions of the hippocampus. The reason is CA1 and CA3 areas play an important role in spatial learning and memory retrieval. At the same time, in the hippocampus injury process, CA1 area is one of the first affected areas, and then transferred to CA3 and other areas [20]. The results of HE staining showed that the neuronal cells in the CA1 and CA3 areas of the control group were arranged neatly, densely, evenly distributed, and the cell outline was clear, and the nucleus was round and obvious. Compared with the control group, the number of neurons in the CA1 and CA3 areas of the model group was significantly reduced, the arrangement structure was disordered, the cell outline was unclear, and the morphology was abnormal. In addition, a large number of neuronal cells in the CA1 area showed obvious degeneration and necrosis, and the coloration deepened. The neurons in the CA3 area showed sparse cell arrangement, obvious gaps, irregular shape and swelling, a large number of vacuole-like cells were observed, and the coloration was lighter. The intervention of β-asarone improved this situation, and significantly increased the number of normal neurons in the CA1 and CA3 areas. The morphology of neuronal cells was relatively normal, and the nuclei of some neuronal cells were round and obvious, and the structure was relatively complete, and the arrangement was relatively orderly. The result is shown in Fig. 5.
Fig. 5
HE staining of pathological sections of rats’ hippocampus. The expression of neurons in CA1 (A) and CA3 (B) areas in different groups. In the control group, neurons in CA1 and CA3 areas were neatly arranged, dense and evenly distributed, with clear cell outlines and obvious rounded nuclei. The number of neurons in the CA1 and CA3 regions of the model group was significantly reduced, with abnormal morphology and disordered arrangement. The cells in the CA1 and CA3 areas of the β-asarone group and the positive drug group were arranged neatly and evenly, the cell outline was clearer, and the shape returned to normal.
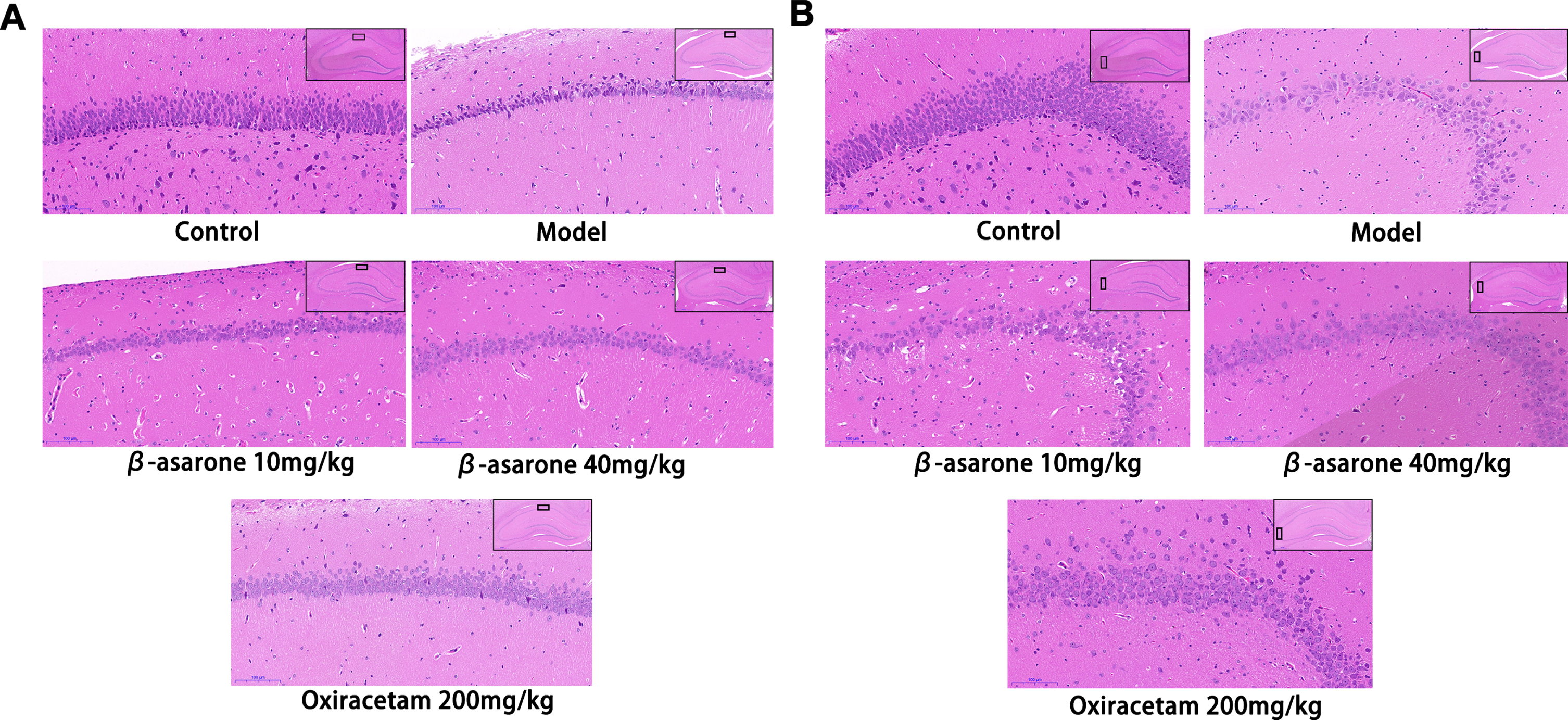
β-asarone reduces oxidative stress in DE rats
Oxidative stress has been considered as an important factor in the pathogenesis of AD, and it can promote neuronal cell apoptosis through a variety of ways. We observed the occurrence of oxidative stress in the body by detecting NOS and LPO in the brain homogenate of DE rats. As illustrated in Fig. 6A, B, compared with the control group, the LPO content and NOS activity in the model group increased significantly. Compared with the model group, after the administration of β-asarone, both indexes of DE rats were significantly reduced, and the performance of the high-dose β-asarone group was more obvious. The results show that β-asarone can significantly improve the oxidative stress level in DE rats, thereby exerting a certain protective effect on neuronal cells.
Fig. 6
The effect of β-asarone on LPO content (A) and NOS (B) in brain tissue homogenate of different groups of rats. Data was expressed as mean±SD. N = 10. Compared with control group: *p < 0.05, **p < 0.01; compared with Model group: #p < 0.05, # #p < 0.01.
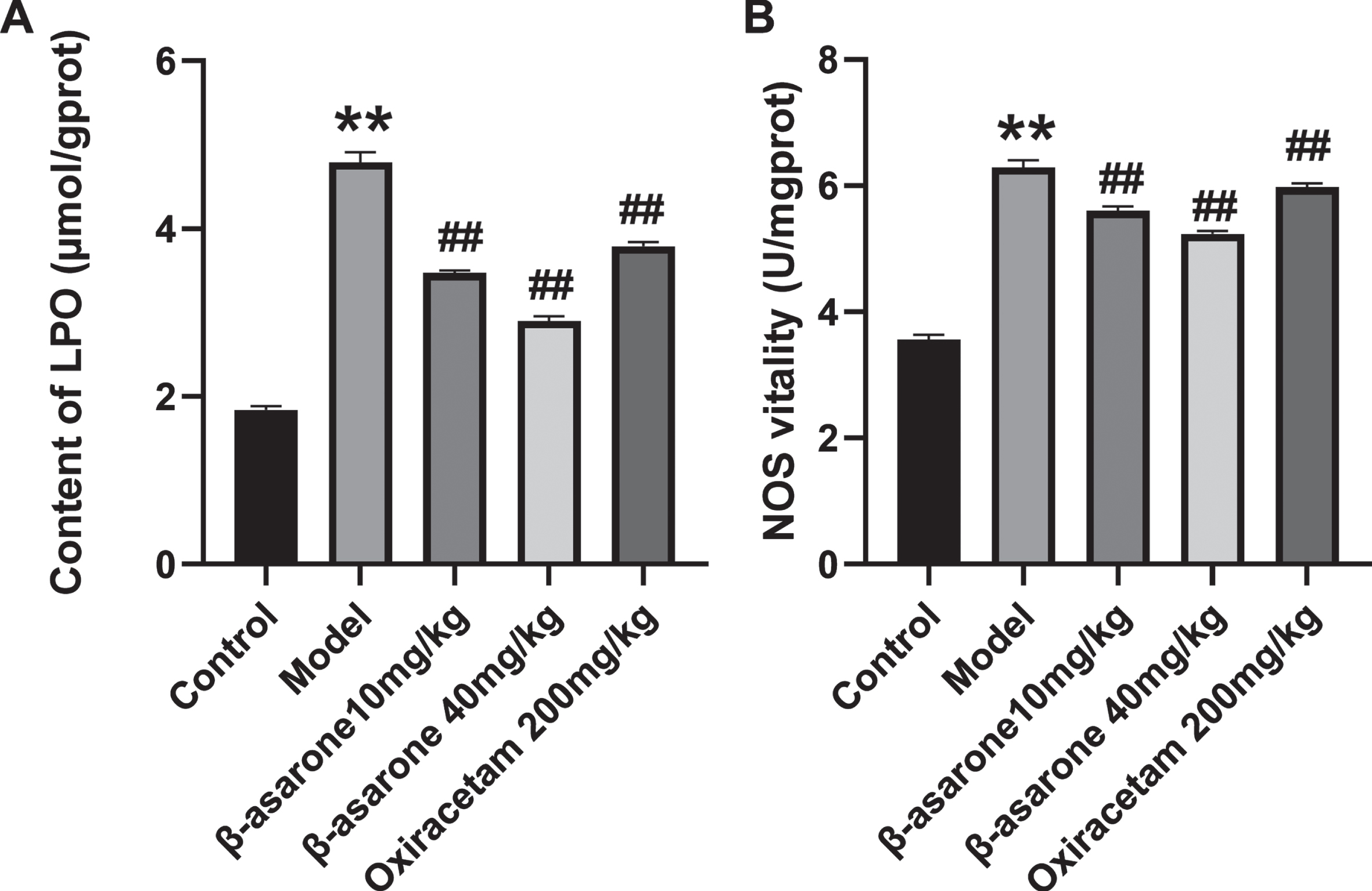
β-asarone exerts neuron protection in many ways
It is well known that the accumulation of Aβ in the brain will damage the ability of learning and memory, and then gradually develop into AD. In addition, there is an interactive relationship between oxidative stress in brain tissue and the production of Aβ. Based on the special function of CA1 and CA3 regions in the hippocampus, the experiment focused on the observation of the accumulation and deposition of Aβ in CA1 and CA3 regions. The experimental results showed that the Aβ-positive particles were brown, the neurons of the rats in the normal group were arranged neatly and densely, and there was almost no deposition of Aβ. In contrast, the rat neuronal cells in the CA1 area of the model group showed obvious degeneration and apoptosis, and the neuronal cells in the CA3 area showed swelling and sparse arrangement. The production of Aβ mainly existed in the Golgi apparatus and the cell membrane area. The staining results showed that there was a large amount of Aβ in the cell membrane and cytoplasm of neuronal cells in CA1 and CA3 areas. After the administration of β-asarone, the condition of neuronal cells and the deposition of Aβ improved, and it was dose-dependent, as shown in Fig. 7.
Fig. 7
Aβ deposition in hippocampus CA1 (A) and CA3 area (B) of rats in different groups. In the control group, there was no Aβ deposition in the neuronal cells of CA1 and CA2 regions, and the neuronal cells were clearly outlined. In the model group, the outlines of neurons in the CA1 and CA2 areas were blurred, most of them were in a necrotic state, the brown granules were significantly increased, and the Aβ deposition was obvious. In the β-asarone and positive drug groups, some neurons in the CA1 and CA2 regions had clear outlines, and the Aβ deposition was significantly reduced.
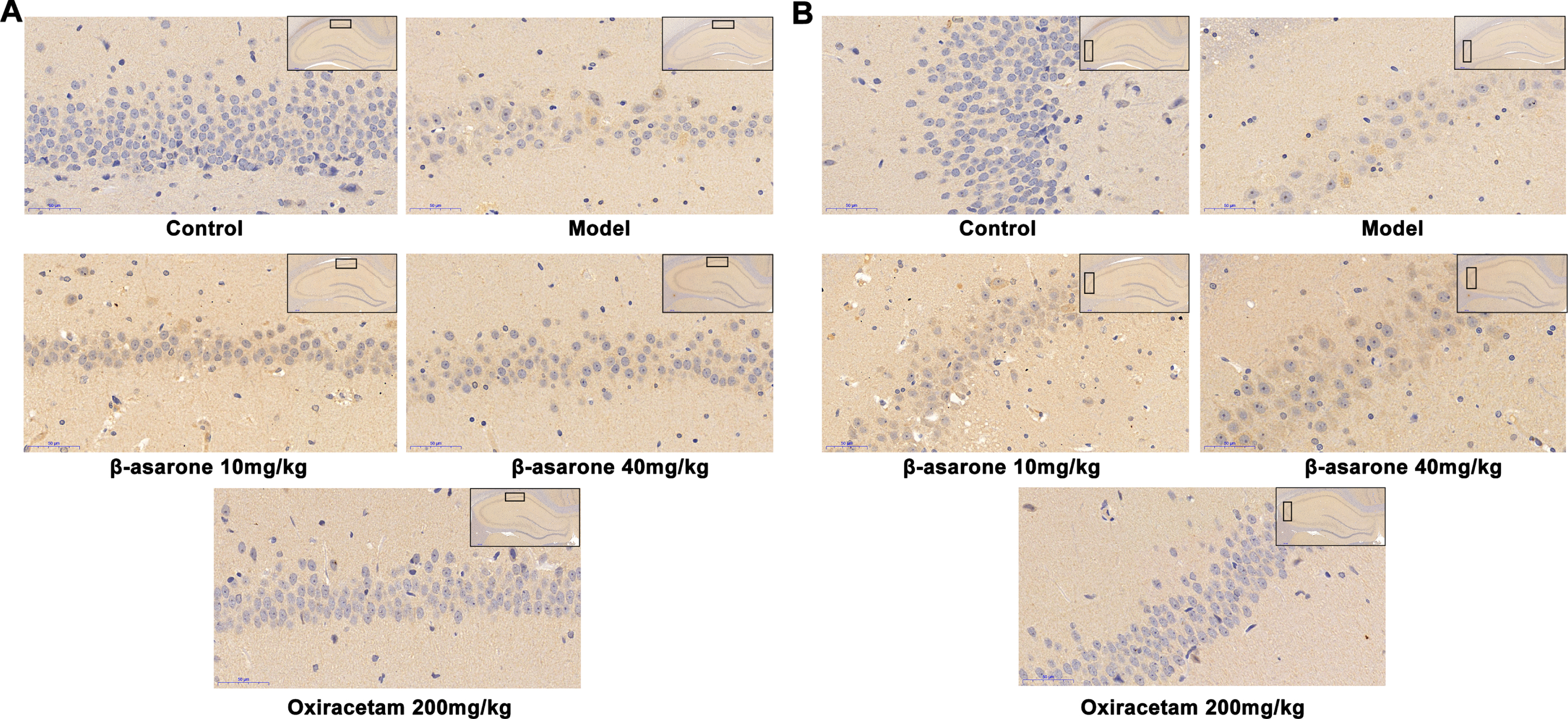
BDNF stands out for its high level of expression in the central nervous system. It regulates the survival and differentiation of neurons and plays a role in activity-dependent plasticity, such as learning and memory [21]. Therefore, we have done further research on the protective effect of β-asarone by regulating BDNF neurons. After immunohistochemical staining, the BDNF-positive particles showed a brown color as shown in Fig. 8. The results showed that BDNF is an important substance that promotes neuron growth. There was a significant expression in the hippocampus of rats in the normal group, while it was significantly reduced in the model group. After administration of β-asarone, the expression of BDNF in the hippocampus of DE rats was significantly increased, which played an important role in the restoration of the normal morphology of neuronal cells.
Fig. 8
The expression of BDNF in hippocampus CA1 (A) and CA3 (B) of different groups of rats. In the control group, the neuronal cells in the CA1 and CA2 regions were clearly outlined, and the expression of BDNF was active. In the model group, the outlines of neurons in CA1 and CA2 regions were blurred, most of them were in necrotic state, and the production of BDNF was significantly reduced. In the β-asarone and positive drug groups, some neurons in the CA1 and CA2 regions had clear outlines, and the production of BDNF was significantly increased.
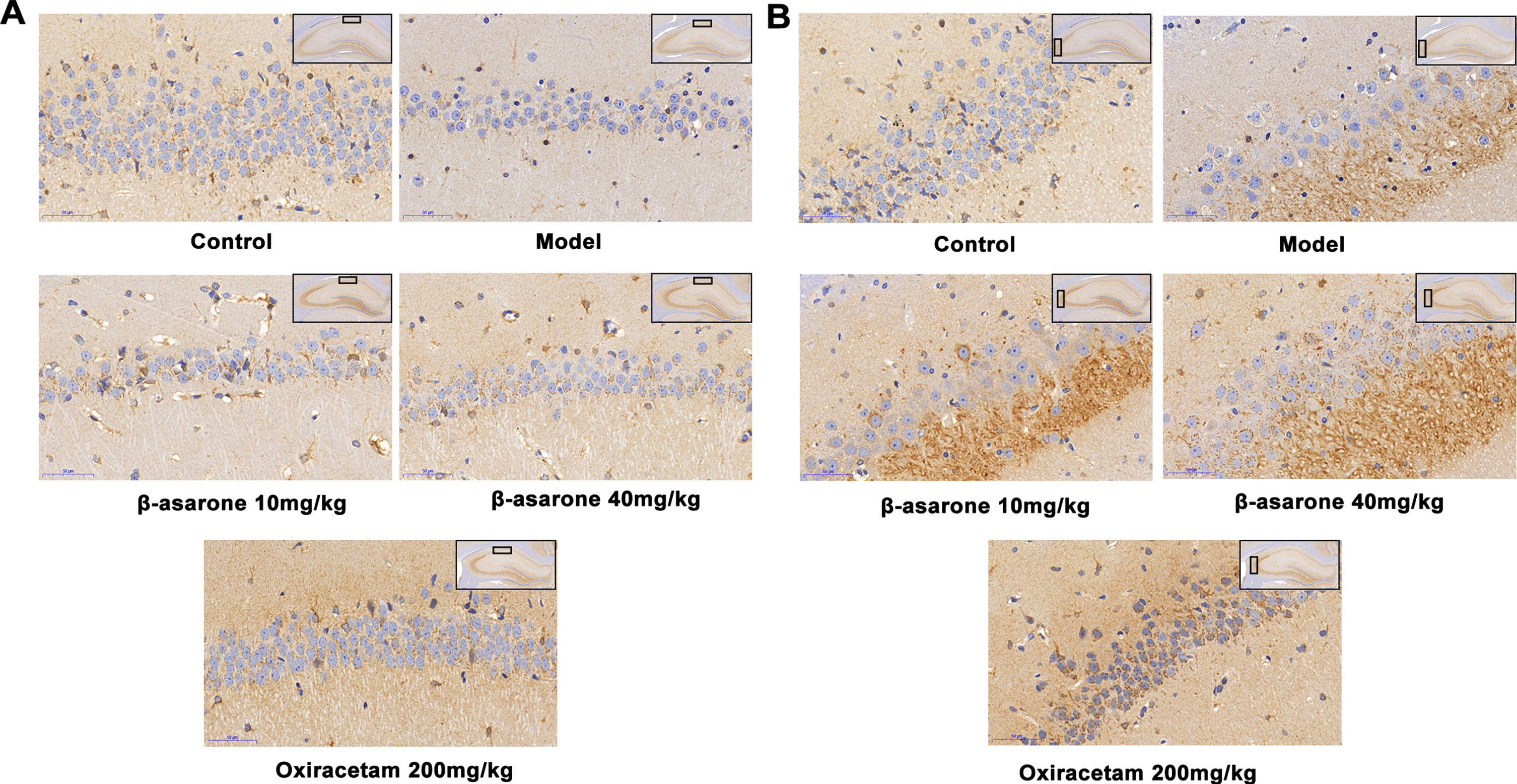
β-asarone reduces the expression of apoptotic genes in the brain of DE rats
During the pathogenesis of DE, the production of oxidative stress and the deposition of Aβ will affect the neuronal apoptosis in the brain of model rats. In order to understand more clearly how β-asarone can improve the neuronal apoptosis in the brain of DE rats, we tested the apoptotic genes in different groups of rats. The results showed that compared with the control group, the expression of Bcl-2 mRNA in the model group was significantly reduced, on the contrary, the expression of Bax mRNA in the model group was significantly increased. Compared with the model group, β-asarone intervention can significantly increase the expression of Bcl-2 mRNA and reduce the expression of Bax mRNA at the same time, thereby exerting an anti-neuronal apoptosis effect as shown in Fig. 9.
Fig. 9
β-asarone regulates the mRNA expression of Bcl-2 (A) and Bax (B). Data was expressed as mean±SD. N = 3. Compared with control group: *p < 0.05, **p < 0.01; compared with Model group: #p < 0.05, # #p < 0.01.
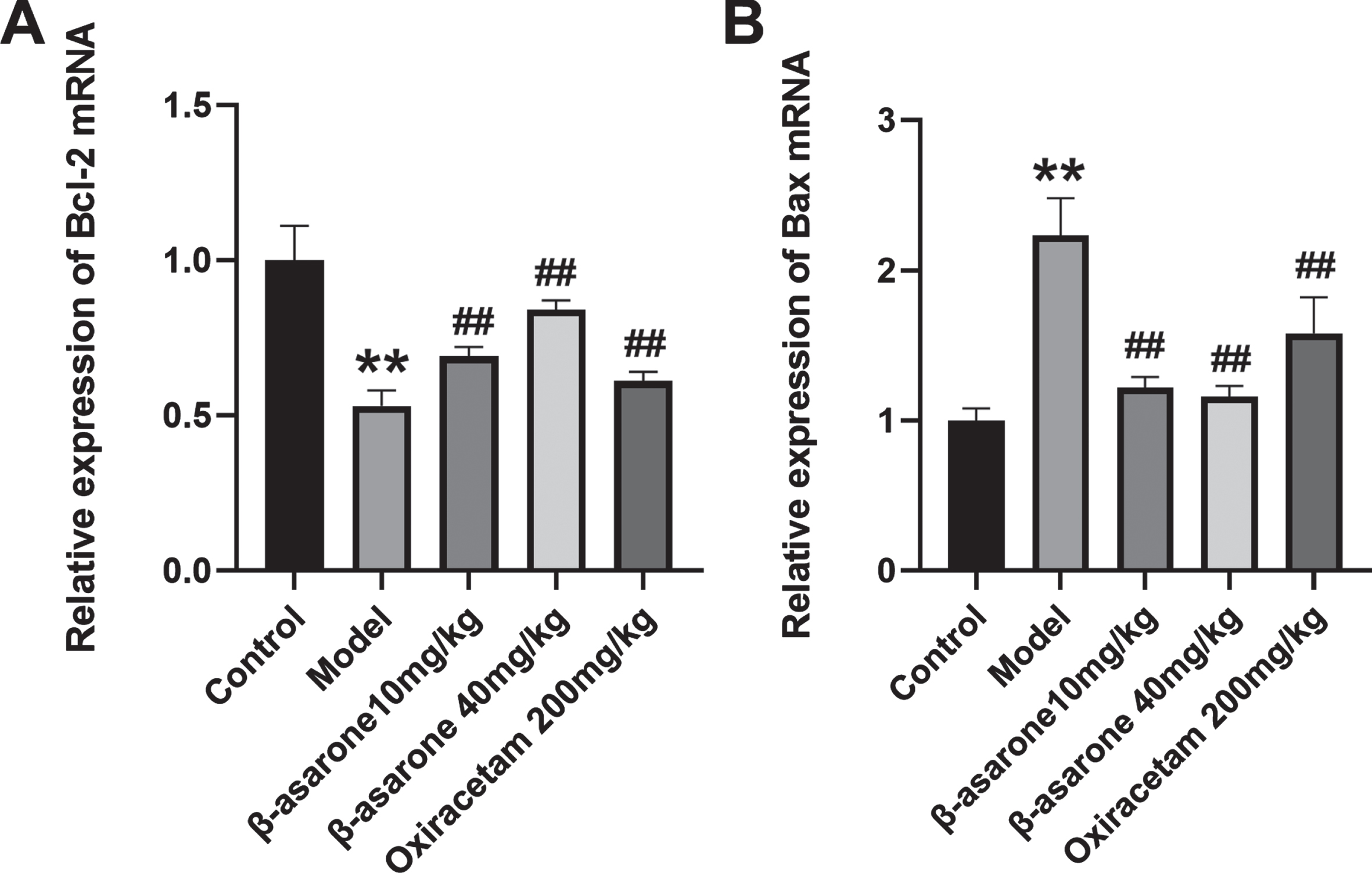
DISCUSSION
More and more evidence shows that the rapid increase in the prevalence of type 2 diabetes is closely related to obesity and blood lipids [22]. Experimental studies of DE have shown that through the feeding of high-sugar and high-fat diets and the induction of STZ injection, the blood sugar of rats will rise rapidly, and long-term hyperglycemia will damage the cognitive function of rats and develop DE [23]. Experimental studies of DE have shown that through the feeding of high-sugar and high-fat diets and the induction of STZ injection, the blood sugar of rats will rise rapidly, and long-term hyperglycemia will damage the cognitive function of rats and develop DE [22]. In the DE rat model, as expected, β-asarone can significantly improve the spatial learning and memory ability of DE model rats. Interestingly, during the entire experiment, we have been testing the weight and blood sugar of the rats and found that β-asarone did not significantly change their blood sugar and weight. In addition, by testing the serum insulin in different groups of rats, there was no significant difference in the insulin content of the administration group compared with the model group. Therefore, we speculate that β-asarone does not improve the learning and memory ability of DE model rats by promoting the release of insulin and lowering blood sugar. This also provides an idea for the combination of β-asarone and hypoglycemic drugs.
DE model rats are often accompanied by abnormal blood lipid levels. The dysfunction of blood vessels and the development of neurodegenerative diseases have a certain synergistic effect, which can aggravate cognitive dysfunction in AD [24]. And HDL-C has potential neuroprotective effects under conditions of hyperglycemia [25]. In addition, the central cholinergic neurons are important in the learning and memory performance of a variety of learned behaviors. AChE inhibitors can play a better neuroprotective effect, and this is currently the first drug for the treatment of AD [26]. In fact, we also found that β-asarone has a good regulatory effect on related blood lipid indexes and AChE.
Protecting neurons from death during oxidative and neuroexcitotoxic stress is key for preventing cognitive dysfunction [27]. Oxidative stress can promote the production of Aβ, promote cell apoptosis, and reduce the expression of LDL-C receptor related protein 1 [28]. On the contrary, Aβ can also induce oxidative stress, and there is a close relationship between them [29, 30]. By improving the lipid peroxidation reaction and reducing the deposition of Aβ, reducing the apoptosis of nerve cells, significantly improving the cognitive deficits of rats [31, 32]. BDNF regulates many different cellular processes involved in the development and maintenance of normal brain function [33]. Increasing the expression of BDNF in the hippocampus can significantly improve the neuroprotective effect of the brain [34]. BDNF increases the level of Bcl-2 by acting on its high-affinity receptor tropomyosin-related kinase B (TRKB), which can reduce cell apoptosis and improve neuronal damage [35]. Bcl-2 and Bax are important apoptotic proteins, the balance of these proteins has an important role in the death or life of a neuronal cell [36]. Studies have shown that higher ratios of Bcl-2/Bax can provide significant neuroprotection [37]. However, the current drug treatment of diabetes cannot prevent the development of DE, so there is an urgent need for an effective and low side effect therapeutic drug. β-asarone is derived from traditional Chinese medicine, and our research has proved its remarkable efficacy in the treatment of DE. It reduces Aβ and oxidative stress, increases the content of BDNF, thereby increasing the expression of ratio of Bcl-2 and Bax genes, and plays a role in protecting nerves from many aspects. Therefore, it cannot be replaced by only AChE inhibitors or antioxidants.
Conclusion
In summary, the evidence provided in this study shows that β-asarone can regulate the four indicators of blood lipids in the serum of DE rats and the activity of AChE, reduce oxidative stress and Aβ deposition, and increase the neuroprotective effect of BDNF, thereby increasing the expression of anti-apoptosis gene Bcl-2 which reduces the apoptosis of neuronal cells, exerts the protective effect of neurons, and improves the cognitive ability of rats.
ACKNOWLEDGMENTS
Thanks to Zamar Daka for the revision of the paper’s language grammar.
FUNDING
This work was supported by the National Science Foundation of China (Nos. 81673624 and 81802103), the Key Project of the National Science Fund of Anhui Province (No. KJ2017A277).
CONFLICT OF INTEREST
The authors have no conflict of interest to report.
REFERENCES
[1] | Kalinovich A , Dehvari N , Aslund A , van Beek S , Halleskog C , Olsen J , Forsberg E , Zacharewicz E , Schaart G , Rinde M , Sandstrom A , Berlin R , Ostenson CG , Hoeks J , Bengtsson T ((2020) ) Treatment with a beta-2-adrenoceptor agonist stimulates glucose uptake in skeletal muscle and improves glucose homeostasis, insulin resistance and hepatic steatosis in mice with diet-induced obesity. Diabetologia 63: , 1603–1615. |
[2] | Bidonde J , Fagerlund BC , FrØensuremath ønsdal KB , Lund UH , Robberstad B NIPH systematic reviews. In FreeStyle Libre Flash Glucose Self-Monitoring System: A Single-Technology Assessment, Knowledge Centre for the Health Services at The Norwegian Institute of Public Health (NIPH), Oslo, Norway. Report from the Norwegian Institute of Public Health No. 2017-07. |
[3] | Cui JQ , Tong R , Xu J , Tian YN , Pan J , Wang N , Chen HA , Peng YQ , Fei SJ , Ling W , Guo CY , Yao JC , Cui W ((2021) ) Association between STAT4 gene polymorphism and type 2 diabetes risk in Chinese Han population. BMC Med Genomics 14: , 169. |
[4] | Bedriñana-Marañón B , Rubio-Rodríguez M , Yovera-Aldana M , Garcia-Villasante E , Pinedo-Torres I ((2021) ) Association between the diabetes mellitus duration and the severity of diabetic foot disease in hospitalized patients in Latin America. Int J Low Extrem Wounds, doi:10.1177/15347346211063266. |
[5] | Xu W , Zhang H , Zhang Q , Xu J ((2022) ) β-Amyrin ameliorates diabetic nephropathy in mice and regulates the miR-181b-5p/HMGB2 axis in high glucose-stimulated HK-2 cells. Environ Toxicol 37: , 637–649. |
[6] | Bilha SC , Leustean L , Preda C , Branisteanu DD , Mihalache L , Ungureanu MC ((2021) ) Bone mineral density predictors in long-standing type 1 and type 2 diabetes mellitus. BMC Endocr Disord 21: , 156. |
[7] | Zhang Y , Huang NQ , Yan F , Jin H , Zhou SY , Shi JS , Jin F ((2018) ) Diabetes mellitus and Alzheimer’s disease: GSK-3β as a potential link. Behav Brain Res 339: , 57–65. |
[8] | Sasaki-Hamada S , Sanai E , Kanemaru M , Kamanaka G , Oka JI ((2021) ) Long-term exposure to high glucose induces changes in the expression of AMPA receptor subunits and glutamate transmission in primary cultured cortical neurons. Biochem Biophys Res Commun 589: , 48–54. |
[9] | Katzmarski N , Ziegler-Waldkirch S , Scheffler N , Witt C , Abou-Ajram C , Nuscher B , Prinz M , Haass C , Meyer-Luehmann M ((2020) ) Aβ oligomers trigger and accelerate Aβ seeding. Brain Pathol 30: , 36–45. |
[10] | Li Q , Cui J , Fang C , Liu M , Min G , Li L ((2017) ) S-adenosylmethionine attenuates oxidative stress and neuroinflammation induced by amyloid-β through modulation of glutathione metabolism. J Alzheimers Dis 58: , 549–558. |
[11] | Chauhan A , Chauhan V ((2020) ) Beneficial effects of walnuts on cognition and brain health. Nutrients 12: , 550. |
[12] | Yin Z , Geng X , Zhang Z , Wang Y , Gao X ((2021) ) Rhein relieves oxidative stress in an Aβ(1-42) oligomer-burdened neuron model by activating the SIRT1/PGC-1α-regulated mitochondrial biogenesis. Front Pharmacol 12: , 746711. |
[13] | Zhang SS , Xue R , Geng YP , Wang H , Li WJ ((2020) ) Fisetin prevents HT22 cells from high glucose-induced neurotoxicity via PI3K/Akt/CREB signaling pathway. Front Neurosci 14: , 241. |
[14] | Lu B , Nagappan G , Lu Y ((2014) ) BDNF and synaptic plasticity, cognitive function, and dysfunction. Handb Exp Pharmacol 220: , 223–250. |
[15] | Xu FB , Wu HY , Zhang K , Lv PZ , Zheng L , Zhao JM ((2016) ) Pro-neurogenic effect of beta-asarone on RSC96 Schwann cellsin vitro. In Vitro Cell Dev Biol Anim 52: , 278–286. |
[16] | Han YF , Wang NB , Kang J , Fang YQ ((2020) ) beta-Asarone improves learning and memory in A beta(1-42)-induced Alzheimer’s disease rats by regulating PINK1-Parkin-mediated mitophagy. Metab Brain Dis 35: , 1109–1117. |
[17] | Wang NB , Wang HY , Li LY , Li YC , Zhang RH ((2020) ) beta-asarone inhibits amyloid-beta by promoting autophagy in a cell model of Alzheimer’s disease. Front Pharmacol 10: , 1529. |
[18] | Dong H , Cong W , Guo X , Wang Y , Tong S , Li Q , Li C ((2019) ) β-asarone relieves chronic unpredictable mild stress induced depression by regulating the extracellular signal-regulated kinase signaling pathway. Exp Ther Med 18: , 3767–3774. |
[19] | Yan W , Zhang M , Yu Y , Yi X , Guo T , Hu H , Sun Q , Chen M , Xiong H , Chen L ((2019) ) Blockade of voltage-gated potassium channels ameliorates diabetes-associated cognitive dysfunction in vivo and in vitro . Exp Neurol 320: , 112988. |
[20] | Bartsch T , Wulff P ((2015) ) The hippocampus in aging and disease: From plasticity to vulnerability. Neuroscience 309: , 1–16. |
[21] | Kojima M , Mizui T ((2017) ) BDNF propeptide: A novel modulator of synaptic plasticity. Neurotrophins 104: , 19–28. |
[22] | Henning RJ ((2018) ) Type-2 diabetes mellitus and cardiovascular disease. Future Cardiol 14: , 491–509. |
[23] | Hu T , Shi JJ , Fang J , Wang Q , Chen YB , Zhang SJ ((2020) ) Quercetin ameliorates diabetic encephalopathy through SIRT1/ER stress pathway in db/db mice. Aging (Albany NY) 12: , 7015–7029. |
[24] | Frontinan-Rubio J , Rabanal-Ruiz Y , Duran-Prado M , Alcain FJ ((2021) ) The protective effect of ubiquinone against the amyloid peptide in endothelial cells is isoprenoid chain length-dependent. Antioxidants 10: , 1806. |
[25] | Couret D , Planesse C , Patche J , Diotel N , Nativel B , Bourane S , Meilhac O ((2021) ) Lack of neuroprotective effects of high-density lipoprotein therapy in stroke under acute hyperglycemic conditions. Molecules 26: , 6365. |
[26] | Kumar H , Kim BW , Song SY , Kim JS , Kim IS , Kwon YS , Koppula S , Choi DK ((2012) ) Cognitive enhancing effects of alpha asarone in amnesic mice by influencing cholinergic and antioxidant defense mechanisms. Biosci Biotechnol Biochem 76: , 1518–1522. |
[27] | Sharma VK , Yang X , Kim SK , Mafi A , Saiz-Sanchez D , Villanueva-Anguita P , Xiao L , Inoue A , Goddard WA , 3rd Loh YP ((2021) ) Novel interaction between neurotrophic factor-α1/carboxypeptidase E and serotonin receptor, 5-HTR1E, protects human neurons against oxidative/neuroexcitotoxic stress via β-arrestin/ERK signaling. Cell Mol Life Sci 79: , 24. |
[28] | Cai Z , Zhao B , Ratka A (2011) Oxidative stress and b-amyloid protein in Alzheimer’Z disease. |
[29] | Gardener SL , Rainey-Smith SR , Villemagne VL , Fripp J , Doré V , Bourgeat P , Taddei K , Fowler C , Masters CL , Maruff P , Rowe CC , Ames D , Martins RN ((2021) ) Higher coffee consumption is associated with slower cognitive decline and less cerebral Aβ-amyloid accumulation over 126 months: Data from the Australian Imaging, Biomarkers, and Lifestyle Study. Front Aging Neurosci 13: , 744872. |
[30] | Ikram M , Jo MH , Choe K , Khan A , Ahmad S , Saeed K , Kim MW , Kim MO ((2021) ) Cycloastragenol, a triterpenoid saponin, regulates oxidative stress, neurotrophic dysfunctions, neuroinflammation and apoptotic cell death in neurodegenerative conditions. Cells 10: , 2719. |
[31] | Jeong ES , Bajgai J , You IS , Rahman MH , Fadriquela A , Sharma S , Kwon HU , Lee SY , Kim CS , Lee KJ ((2021) ) Therapeutic effects of hydrogen gas inhalation on trimethyltin-induced neurotoxicity and cognitive impairment in the C57BL/6 mice model. Int J Mol Sci 22: , 13313. |
[32] | Qu XX , He JH , Cui ZQ , Yang T , Sun XH ((2021) ) PPAR-α agonist GW7647 protects against oxidative stress and iron deposit via GPx4 in a transgenic mouse model of Alzheimer’s diseases. ACS Chem Neurosci 13: , 207–216. |
[33] | Colucci-D’Amato L , Speranza L , Volpicelli F ((2020) ) Neurotrophic factor BDNF, physiological functions and therapeutic potential in depression, neurodegeneration and brain cancer. Int J Mol Sci 21: , 7777. |
[34] | Umeda T , Sakai A , Shigemori K , Yokota A , Kumagai T , Tomiyama T ((2021) ) Oligomer-targeting prevention of neurodegenerative dementia by intranasal rifampicin and resveratrol combination - a preclinical study in model mice.Front Neurosci15: , 763476. |
[35] | Zhai Y , Liu BG , Mo XN , Zou M , Mei XP , Chen W , Huang GD , Wu L ((2022) ) Gingerol ameliorates neuronal damage induced by hypoxia-reoxygenation via the miR-210/brain- derived neurotrophic factor axis. Kaohsiung J Med Sci 38: , 367–377. |
[36] | Erdal ME , Yilmaz SG , Ay ME , Kara HG , Ozge AA , Tasdelen B ((2020) ) A study investigating the role of 2 candidate SNPs in Bax and Bcl-2 genes in Alzheimer’s disease. P R Health Sci J 39: , 264–269. |
[37] | Zhang Y , Yang X , Ge XH , Zhang FY ((2019) ) Puerarin attenuates neurological deficits via Bcl-2/Bax/cleaved caspase-3 and Sirt3/SOD2 apoptotic pathways in subarachnoid hemorrhage mice. Biomed Pharmacother 109: , 726–733. |