Alzheimer’s Disease and Herbal Combination Therapy: A Comprehensive Review
Abstract
Alzheimer’s disease (AD) was first described in 1907 and got its name after Alois Alzheimer, a German psychiatrist and neuropathologist. This disease starts slow, increasing gradually to worsen in the due course of time. AD is mainly characterized by the associated dementia, which is a decline of cognitive effects such as memory, praxis, and orientation. The dementia is further highlighted by the presence of psychological and behavioral symptoms. Additionally, AD is also associated with the multiple interconnected pathways linked neuropathological changes such as the formation of neurofibrillary tangles and amyloid-β plaques inside the brain. AD therapeutics have been of prime concern over the decades, resulting in the elucidation of promising therapeutic targets. The requirement of AD stage dependent optimized conditions has necessitated a combinatorial approach toward treatment. The priority in AD research has remained to develop disease-modifying and development-reducing drugs for treatment regimens followed during the early and later stages, respectively.
INTRODUCTION
Alzheimer’s disease (AD) is a common neurodegenerative disorder and is mainly characterized by several symptoms such as decline of cognitive function coupled with progressive memory loss. AD is detected by the presence of several molecular lesions that lead to the inflammatory and oxidative damage due to the presence of misfolded proteins accumulated in the aging brain, amounting to synaptic dysfunction and energy loss [1]. Globally, over 24 million people are estimated to have dementia, of who a majority suffer from AD. Thereby imperatively prioritizing AD research, which represents a major public health concern. As of today, the treatments available focus on reducing the symptoms of AD. Implying the need to understand better the disease pathogenesis in an attempt to discover/develop the kind of treatment that could subside the symptoms or repair the done damage [2]. Age remains the main risk factor associated with the AD; with most of victims aged over 65 years [3]. Early diagnosis and investigation remain the most crucial factor to focus the therapeutic ventures involving both pharmaceutical and psychosocial support systems.
Fig.1
Histopathological variations between a) healthy neuron and b) AD neuron.
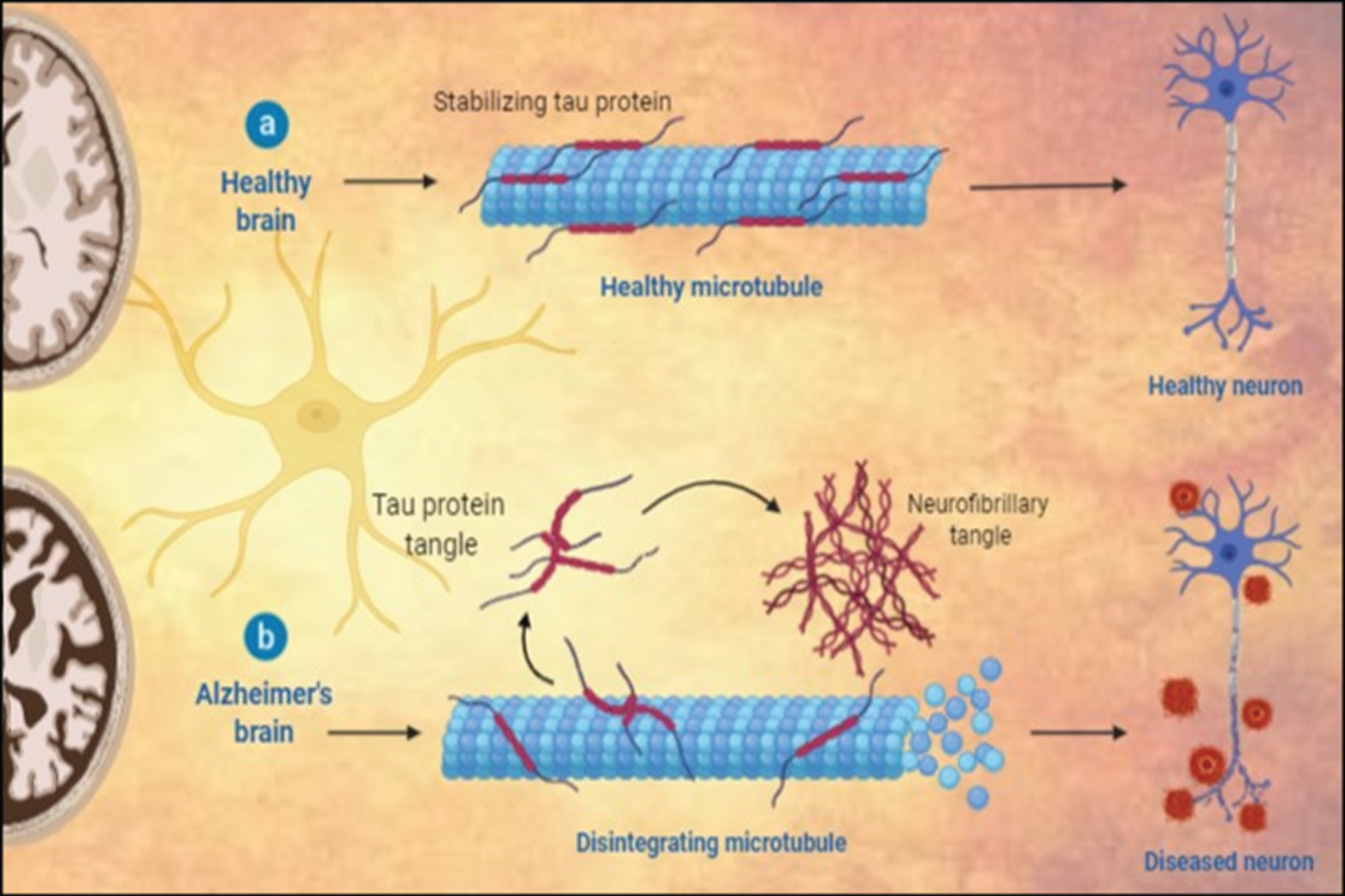
The end stages of AD are marked by the continuous loss of physical and mental autonomy, culminating in dependency and burden on others due to motor function deterioration. The advance stages of AD usually include mood swings, language disorientation, self-care mismanagement, loss of motivation, and behavioral integrity. A sense of social and familial withdrawal is also observed as the condition worsens [3, 4]. Ultimately body functions are lost gradually leading to death. The rate of disease progression varies among individuals, and the average life expectancy in an event of AD may range from 3–9 years after the initial diagnosis [1, 5].
Knowledge about the causative factors of AD is limited; however, genetic inheritance of the disease is believed to be the major associated risk factor [2]. Other risk factors include head trauma, depression, and hypertension [3]. Notwithstanding, AD remains a multifactorial neurodegenerative disease which includes both risk factors and genetic mutations occurring due to abnormal accumulation of amyloid-β (Aβ) and tau proteins. While the presence of phosphorylated tau has been identified in the brain during early stages, the later stages are characterized by the presence of insoluble Aβ protein deposits. Studies indicate that the amount of insoluble Aβ protein deposits is directly proportional to the extent of dementia in those suffering with AD [3]. In addition, Aβ protein is believed to cause further inflammatory reactions that promote development of AD and cognitive decline. The Aβ hypothesis postulates that Aβ protein accumulation is the primary cause or effect of the pathogenesis of AD and the disease further progresses due to the imbalance caused by the Aβ production and clearance. Several primary strategies have been deployed against AD using this hypothesis [2].
AD diagnosis mostly comprises of cognitive testing, apart from the regular blood tests and medical imaging [6]. However, the definite diagnosis of AD relies upon a clear brain tissue examination. Although studies suggest that the risk of AD can be reduced by performing physical and mental exercises to reduce obesity, there are no such specific therapies limited to risk reduction for AD [7].
Although some medication can help reduce the symptoms, no such treatment exists which promises either reversing or stopping the AD progression [4]. The prescribed antipsychotic drugs are limited to the treatment of psychosis, behavioral changes, or dementia, with no or little benefit toward the patient as they promote an early death [8].
The initiation of AD occurrence involves a complex pathogenic process which may have started decades before the symptomatic phase arrives. Early stage treatment addressing the problem at molecular levels at which the initial structural alterations occur would translate into a successful therapy. Currently, such disease-modifying drugs designed to be used in the early stages of AD are in the developmental stage. Multifactorial pathogenesis of AD addressing various molecular targets seems to be the possible approach to be followed modify the disease progression. Thus, the drugs having multimodal action or the combination of drugs targeting the single molecular mechanism promise alternative AD therapy.
DISEASE PREVALENCE STATUS
AD was first described more than 100 years ago [9]. As of 2015, about 29.8 million people were affected by AD globally [9]. It mostly affects the people over 65 years of age, resulting in the death of 1.9 million affected patients. This form of dementia or AD is expected to double every two decades meaning that the number of AD cases would be around 42 million in the ongoing year of 2020 and reach nearly 81 million by 2040 [10]. Regarded as a costly disease due to its expensive treatment costs, the cause of AD is poorly understood. While 70% risk of AD may be inherited from the person’s familial history. Hypertension, head injuries, and depression are a few other risk factors leading to the cause of AD. The patients usually rely on others for their assistance by being a burden to them. Survival and incidence are the other factors on which the prevalence of AD depends. In the year 2000, AD prevalence was estimated to be 1.6% in the age group 65–74, as compared to 19% in the age group 75–84. However, there is a low prevalence rate in the less developed regions. In 2005, 0.379% of global population suffered from dementia, with the World Health Organization (WHO) estimating an increase in the prevalence rate from 0.441% in the 2015 to 0.556% in 2030. If this trend continued, the rate of prevalence would triple in the upcoming years [10].
STAGES OF ALZHEIMER’S DISEASE
AD is characterized into give different stages: 1) Mild cognitive impairment (MCI); 2) Mild AD; 3) Moderate AD; 4) Severe AD; and 5) Very Severe AD. Several studies suggest that the very early phase may be regarded MCI, which may remain unchanged for years, characterized by predominant loss of memory along with cognitive deficits. Whatsoever, there is no clear evidence for this disease. An ambiguity does prevail regarding MCI, with a few concluding it to be an AD precursor, while others classify it a normal aging phenomenon. Despite the debate, few studies have reported that cognitive impairment is the precursor causing dementia which is 15-fold more likely than normal aging to progress into AD. Presently, both early metabolic and structural changes in the brain can be identified using imaging techniques, whereas there is no single screening technique/test to identify the individuals affected with MCI. In such a situation, people with cognitive decline can be accurately predicted utilizing the combination tests involving neuroimaging and neuropsychology.
Fig.2
Different stages involved in Alzheimer’s disease.
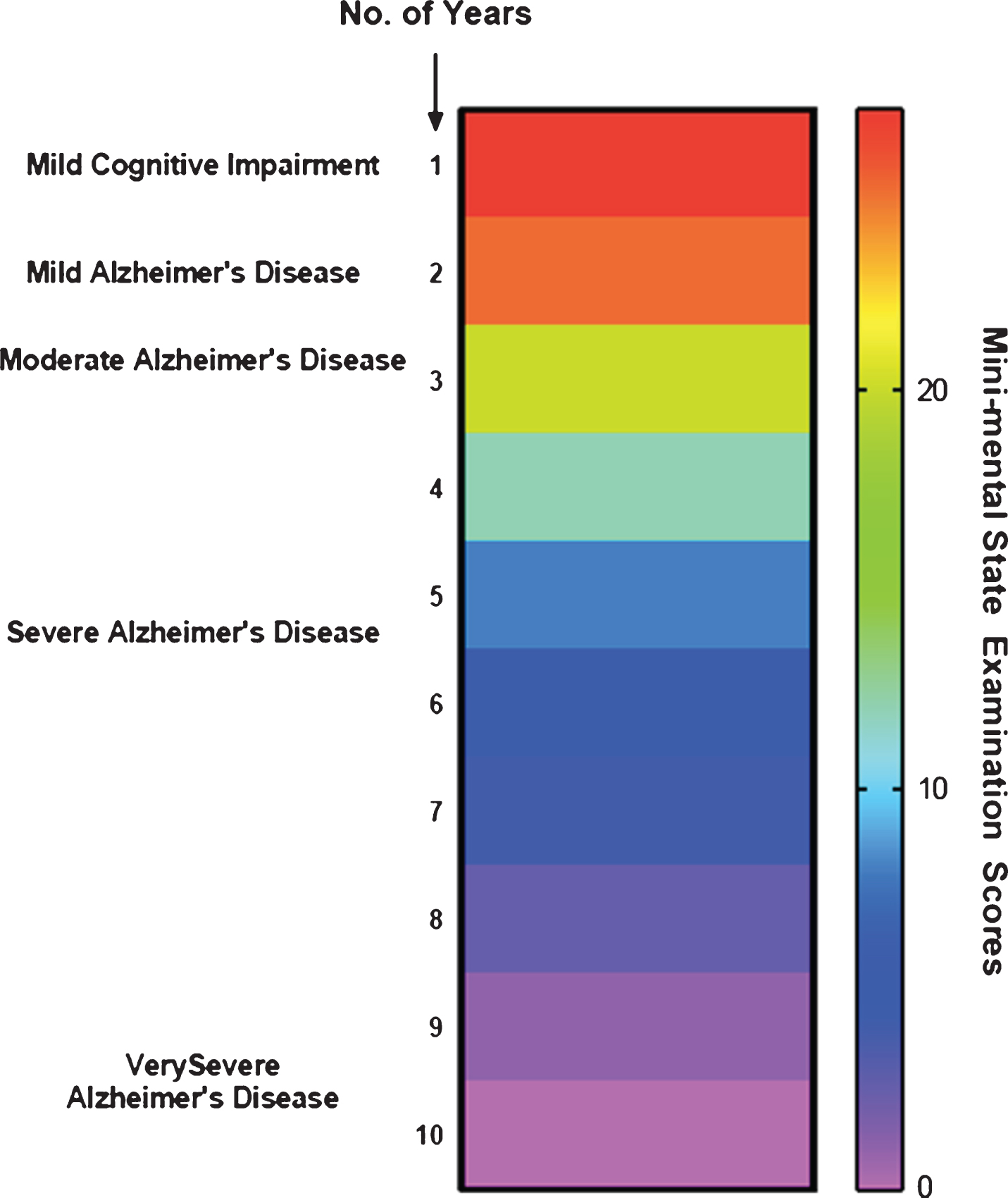
The stable MCI phase ends up with cognitive function decline that may last for about two to five years with the degradation of implicit memory (related to experience) and semantic memory (of stored general knowledge and facts). However, there is no evidence that may link such loss of memory to AD. Nonetheless, evidence suggests that these risks can be reduced only if an early recognition and assessment of mild cognitive impairment can be conducted [5].
Mild AD stage is noted for short term memory loss coupled with forgetfulness, loss of interest and hobbies, repetitive questioning, and change in the routine [10]. The patients may also suffer from lingual disorientation caused due to shrinking vocabulary and troubled fluency. Upon disease progression, the patients may be incapable to perform several tasks independently, particularly requiring supervision or assistance for the cognitively demanding activities [11].
When it comes to moderate AD, there are further drastic changes and impaired routine observed alongside symptoms of emerging psychological behaviors of dementia, following the continuous cognitive deficits coupled with the transitions in care. Long term memory loss is observed at this stage where 30% of patients may also develop illusionary misidentifications.
The fourth stage, severe AD, is characterized by altered and agitated sleep patterns with progressive symptoms pertaining to psychological behaviors of dementia and may require assistance even to bathe, feed, or dress. Thereby implying a complete dependence of the patients on caretakers.
The final stage is called very severe AD where reduced language or oration, like the use of one word or simple phrases eventually leading to no speech, and being bedbound with loss of basic psychomotor skills, can be observed. Ultimately the patients will lose the capacity to carry out any task on their own. In this stage, death may occur not just due to AD but also due to comorbidities such as pneumonia or ulcers [11].
Flowchart 1
Symptoms associated with Alzheimer’s disease.
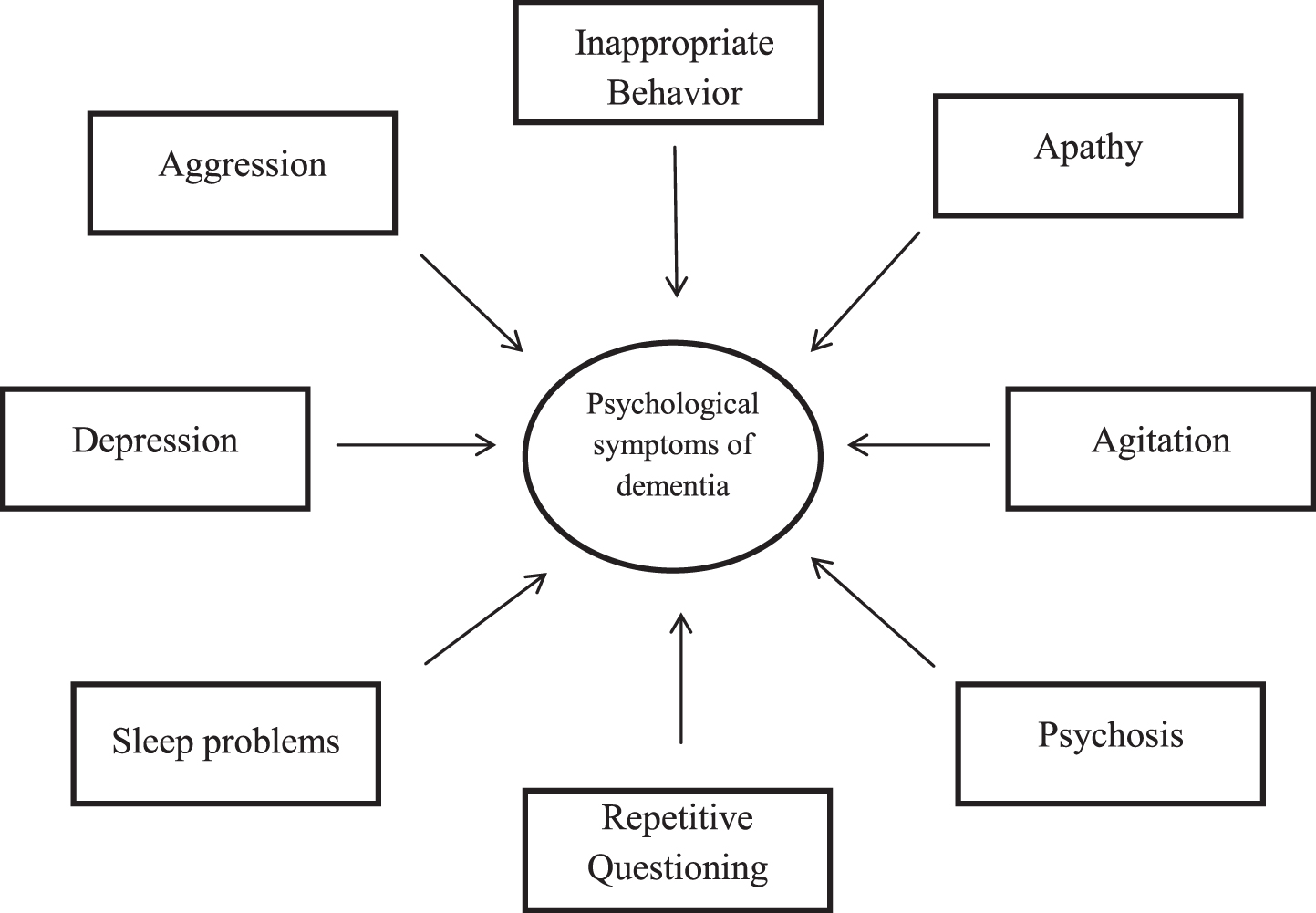
CAUSES AND RISK FACTORS ASSOCIATED WITH ALZHEIMER’S DISEASE
Delaying or slowing down AD progression as well as postponing or preventing the disease in an older age can assure a consequential quality of life and health improvement. The high/elevated amounts of saturated fats may have negative effects on the age-related MCI and cognitive decline [12]. Case studies have shown that several risk factors are involved in causing AD. They include family history, depression, head injury, age, hypertension, apolipoprotein E4 (APOE4) concentrations, low cognitive and physical activity, high cholesterol and diabetes. Promisingly, a few of the above-mentioned risk factors are modifiable [3]. Recent studies have shown that the risk of dementia and cognitive decline could be reduced with the possible association toward fish consumption resulting in uptake of polyunsaturated fatty acids and monounsaturated fatty acids. On the contrary, consumption of dairy products with whole fats by elderly people might lead to cognitive decline [12]. Moderate or light consumption of alcohol is also associated with a reduced risk of AD and incidence of dementia. There is limited evidence on how vegetable and fruit consumption may generally play a neuroprotective role against dementia, AD, and cognitive decline [13].
Notwithstanding, there is no clear evidence for the cause(s) of AD, except for the fact that about 1 to 5% of the reported cases indicate genetic reasons. However, a few hypotheses have been put forth in an attempt to explain the cause and prevalence of AD.
Hereditary factors
AD caused due to genetic heritability ranges from 49% to 79%, according to the studies conducted on familial history [14]. Of the above, only 0.1% of the cases have been symptomatic at the age of 65 or earlier, mostly due to the autosomal dominant inheritance, amounting to an early-onset disease [15]. In any such case, the autosomal changes or mutations have been reported to occur in one of the three genes, viz. the genes encoding for presenilins 1 and 2, and the genes encoding amyloid precursor protein (APP) [16]. There is a reported upregulation of Aβ42, a small protein that happens to be the main component of senile plaques, production due to the above-mentioned gene mutation [17]. Additionally, two other genes, SORL1 (Sortilin related receptor 1) and ABCA7 (ATP Binding Cassette Subfamily A member 7), have been identified to be associated with the autosomal dominant inheritance in AD [18].
Surprisingly, AD is not usually inherited due to autosomal inheritance but due to mutational risk factors such as the genetic and environmental differences, so termed sporadic AD. The best-known example for such sporadic causes is the genetic inheritance of ɛ4 allele of APOE. Supporting this, about 40% to 80% of the reported cases indicate at least one APOE allele [19, 20].
Cholinergic hypothesis
According to the cholinergic hypothesis, it was postulated that AD occurred due to the decreased synthesis of the neurotransmitter acetylcholine (ACh). This is the oldest known hypothesis and the currently available therapies for AD are mostly based on this [21]. Nonetheless, limited support has been provided to the cholinergic hypothesis since the medications/drugs available to treat the deficiency of ACh are not that effective [22].
This hypothesis is based on the fact that cholinergic neurons use ACh for cognitive processes of central and peripheral nervous systems apart from modulating several important neural functions. The cholinergic neurons, mainly found in the basal forebrain, spinal cord, striatum, medial habenula, and olfactory tubercle, are involved in vital physiological aspects such as memory, wakefulness and sleep, learning, sensory information, attention, and stress response-management. The CA3 cholinergic receptor is mainly involved in memory management, thus blocking this receptor might impair the functions of memory and information management in the brain. This consequent dopaminergic impairment and loss of cholinergic neurons was presumed to be the main factor responsible for the psychiatric symptoms related to AD [23].
Furthermore, AD patients with terminal AD were said to have decreased enzymatic activity pertaining to the synthesis and degradation of acetylcholinesterase. Few research groups have confirmed the above hypothesis through evidence that some patients with AD have experienced a cognitive decline as a result of dysfunctional cholinergic system [24].
Amyloid hypothesis
The amyloid hypothesis, proposed in 1991, reasoned that AD occurred due to the presence of accumulated Aβ proteins [25]. The APP gene, responsible for the production of Aβ protein, is located on the 21st chromosome and people exhibiting Down’s syndrome (trisomy 21) carried an extra copy of this gene thus having the earliest recorded symptom(s) of AD by the age of 40 years [26]. APOE4 has been considered the major risk factor for AD as it helps in the breakdown of Aβ, while some isoforms of APOE4 are not very effective resulting in excess neural deposition of the amyloid [27]. Researchers suspect that non-plaque Aβ oligomers referred to as ADDLs (amyloid-derived diffusible ligands) are the aggregates of several monomers which are the pathogenic primary forms of Aβ. The ADDLs modify the structure of synapse by binding to the neurons through surface receptor, causing a breakdown of neuronal communication [28].
This hypothesis was updated recently to suggest that there is a major culprit, N-APP, that not only causes AD but was also closely related to the Aβ protein. N-APP has been identified to be a fragment of the amyloid-β protein precursor (AβPP) peptide’s N-terminus, eventually cleaved or broken-down from AβPP that is adjacent to the Aβ protein. This fragment, N-APP, binds to the neural receptor DR6 (death receptor 6). Also known as the TNFRSF21, to trigger a self-destruction pathway in the neuronal cells. The N-APP-DR6 pathway was reported to further damage the aging human brain as those brain regions affected by AD overexpress DR6 [29].
Role of AβPP in normal conditions
AβPP is a resourceful molecule occurring in three isoforms and these isoforms are not functionally limited to the brain. They are reportedly present throughout the body, implying that AβPP must have important functions. At the cellular level, AβPP has been found to actively contribute toward gene transcription, cell adhesion, axonal transport, and cholesterol metabolism [30].
Fig.3
Representation of formation of amyloid-β plaques and neurofibrillary tangles.
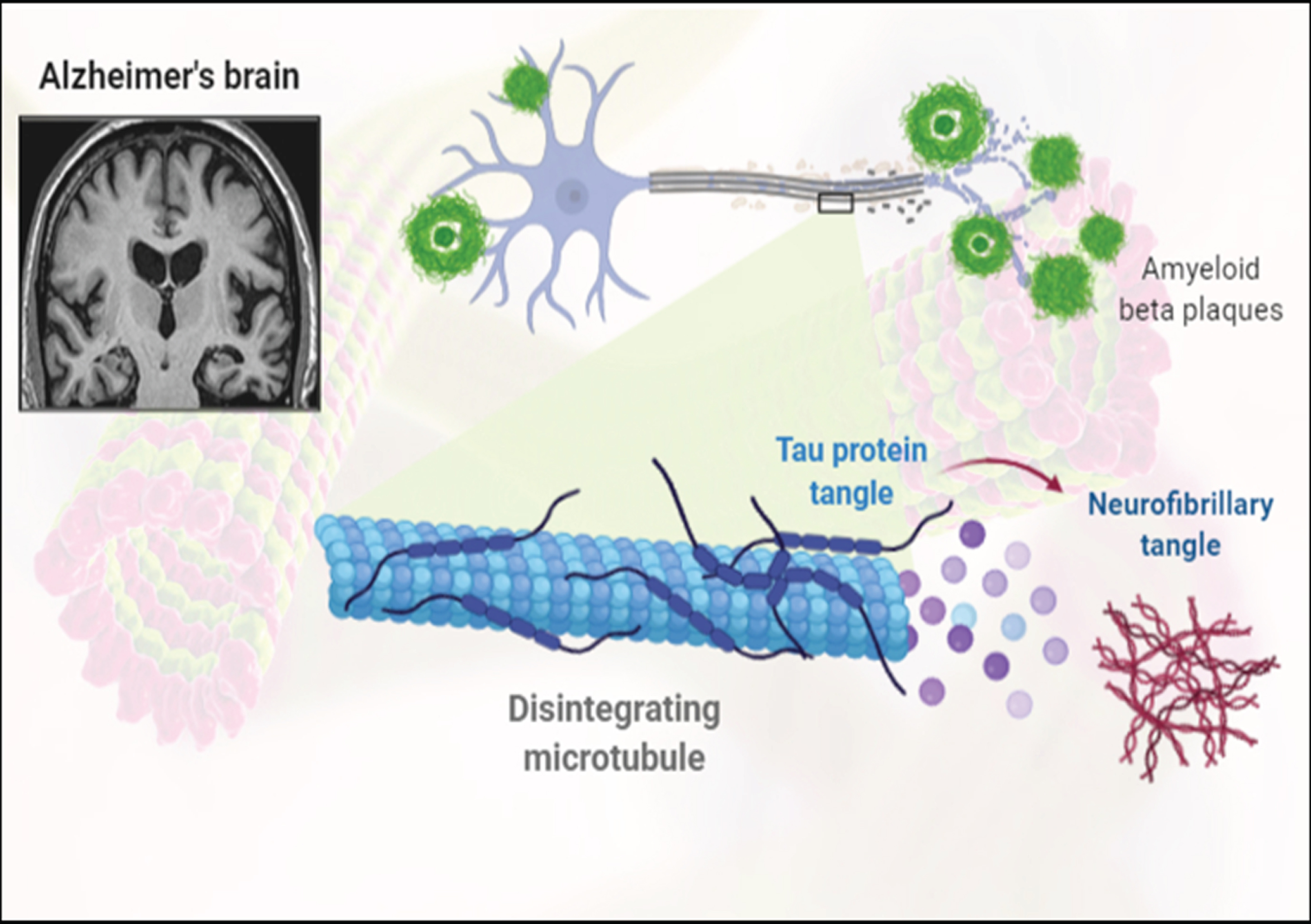
Chemistry of AβPP
AβPP is a single membrane protein with a large amino-terminal domain in the extracellular region and a small cytoplasmic domain in the intracellular region. Numerous sub-domains of the protein have been recognized in the extracellular region, and the cysteine-rich sub-domains are present in the amino terminus, following which is an acidic sub-domain and other two subdomains that are found to be neuroprotective. Several other subdomains associated with putative functions have been recognized in the extracellular region [29].
Isoforms of AβPP
As mentioned earlier, the APP gene is located on chromosome 21 and is expressed in specific cells of the body including glia, neurons, and endothelia [20]. AβPP occurs naturally in three major isoforms, AβPP770, AβPP751, and AβPP695, obtained as the result of alternate splicing of pre-mRNA. The AβPP695 isoform lacks a KPI (Kunitz protease inhibitor) domain, in the extracellular region. In an event of AD, AβPP695 has been reported to be overexpressed as compared to the other isoforms, at a ratio of 1:10:20 (AβPP770:AβPP751:AβPP695) [28, 29].
Putative functions of AβPP
As a G protein-coupled receptor: G protein-coupled receptor(s) typically binds to a ligand(S) to induce a G protein complex trimer, eventually dissociating into the Gα and Gβγ dimer subunits. These subunits are used to activate the intracellular downstream signaling pathways. G protein-mediated AβPP activation is not very well understood owing to the complexities involved in understanding the G protein coupling and pathways linked to it. Notwithstanding, its involvement in synaptic and in neural activity is very well established [27].
Cell adhesion: Several domains of AβPP help in binding to substrates such as collagen, laminin, and heparin. The cell surface AβPP is relatively suitable as a cell adhesion protein given its stability and time taken to degrade. The possible role of AβPP in cell adhesion is that it involves in the tightly regulated synapse formation [30].
Tau hypothesis
The tau hypothesis postulated that AD was caused due to the formation of abnormal tau proteins [31]. As the hyperphosphorylated tau proteins occurring in the neuron were found to pair with the other tau proteins to form the neurofibrillary tangles [32]. This resulted in a collapse of the neuronal transport system caused due to cytoskeleton destruction characterized by microtubule disintegration [33]. Eventually leading to the breakdown of neuronal signaling and their death [34].
NEUROPATHOLOGY OF ALZHEIMER’S DISEASE
AD is mainly characterized by the loss of brain cells and synapses in subcortical regions and cerebral cortex, resulting in the degeneration of the parts of cingulate gyrus and frontal cortex including the parietal and temporal lobes. Therefore, numerous cases of MCI are diagnosed with a reduced brain size as compared to that of a healthy individual [35]. Furthermore, microscopic examination of AD brain tissues provides for a clear visibility of neurofibrillary tangles and amyloid plaques [36]. While plaques are formed around the neurons due to the deposition of dense and insoluble Aβ proteins, neurofibrillary tangles are the aggregates of hyperphosphorylated tau proteins accumulated inside the neurons. As a consequence of aging, fewer individuals might develop certain tangles and plaques when compared with the patients suffering from AD, who would display a greater number of tangles and plaques in the temporal lobe region [35].
DIAGNOSING ALZHEIMER’S DISEASE
AD is primarily diagnosed based on the parameters that include the patient’s medical history of behavioral observations and familial history. In addition, the presence or absence of neuropsychological and neurological characteristic conditions are also taken into consideration [37]. Assessment of patient’s memory impairment by the means of their thinking skills, behavior changes, and functional abilities will have to be carried out in order to characterize stage of a prevailing AD [38]. Currently, there are standardized diagnostic protocols provided by various medical organizations, easing the AD detection process. Several tasks may be assigned by the consulting physician to analyze the individual’s cognitive skills such as problem-solving, memory, thinking-ability, and language usage. Additionally, the below mentioned standard tests can be carried out to differentiate a case of dementia from that of AD.
Mental status testing
A physician can test a patient’s memory and thinking skills by checking their mental status and use scores to evaluate the degree or stage of cognitive impairment.
Brain imaging tests
The patient may be tested to evaluate their mental health and brain conditions associated with the cognitive skills. The interpretation of symptoms might indicate a wide range of conditions, like vitamin B-12 deficiency, thyroid disorder, dementia, or AD. To rule out or overcome this problem, certain confirmatory test will have to be conducted at a laboratory. Medical imaging with magnetic resonance imaging (MRI), computed tomography (CT), positron emission tomography (PET), and/or single-photon emission computed tomography (SPECT) has been extensively utilized to diagnose the AD. Notwithstanding, they may not be useful to differentiate between the other conditions mentioned above [39]. But these techniques are definitely helpful in predicting the different stages of AD [40]. The loss or degeneration of brain cells associated with AD can be shown up in many ways. The present generation imaging techniques have evolved to help identify and differentiate brain tumors, hemorrhages and strokes, apart from the different types of neurodegenerative diseases [40].
Table 1
Approved drugs for Alzheimer’s disease with their mechanism of action and side effects
Drug Name | Drug prescribed | Mechanism of action |
Donepezil | Mild, moderate, and severe AD | Prevents the breakdown of acetylcholine in the brain |
Rivastigmine | Mild to moderate AD | Prevents the breakdown of acetylcholine and butyrylcholine |
Memantine | Moderate to severe AD | Blocks the toxic effects associated with excess glutamate and regulates glutamate activation |
Galantamine | Moderate to severe AD | Blocks the toxic effects associated with excess glutamate and prevents the breakdown of acetylcholine in the brain |
Memantine and Donepezil | Mild to moderate AD | Prevents the breakdown of acetylcholine and stimulates nicotinic receptors to release more acetylcholine in the brain |
Magnetic resonance imaging
Detailed view of the brain powerful magnetic and radio waves.
Computerized tomography
Cross-sectional images of the brain are obtained using the X-rays.
Positron emission tomography
Uses radioactive tracers to scan and detect unusual substances. FDG (fluorodeoxyglucose) PET scan is the most common and effective scanning procedure used to identify the decrease in neural glucose metabolism, an indicator of different degenerative brain disease based on the metabolic pattern obtained. Also, the amyloid plaques formed during AD can be identified through this technique.
Benefits of early AD diagnosis
The lack of cure for AD discourages patients from approaching doctors for the diagnosis. They tend to hide the symptoms due to the loss of independence. Studies indicate that if the right choice is made at the right time, an early diagnosis could be beneficial to initiate either drug or non-drug therapies to control the progression of the disease by slowing down the decline of cognitive skills and memory. Additionally, sustenance strategies may be suggested to the caretakers to minimize the disease progression by planning activities to change the patient’s everyday routine and enhance their living environment. An early diagnosis also it prepares the patient’s family members to plan their future accordingly by prioritizing issues such as finances, community, and home care services [39].
PREVENTING ALZHEIMER’S DISEASE
Certain studies have shown that there is no support or evidence to cure the disease solely via pharmaceutical means. Globally, measures have been taken to delay or even prevent the disease. However, a few studies have shown inconsistent results. Epidemiologically, various studies have suggested that several factors, such as the intellectual activities, diet, and cardiovascular risk factors, can be modified to delay the development of AD. Notwithstanding, clinical evidence is pending to conclude if the above can really help control AD occurrence. Yet, AD remains associated with very high-risk factors such as hypertension, hypercholesterolemia, smoking, and diabetes, which may worsen the course of the disease [36].
TREATMENT FOR THE DISEASE
AD and dementia have gained major public health concern owing to their lack of treatment. AD is a neurodegenerative disorder that progresses day by day. The therapeutic agents used to treat AD must either completely cure the disease or slow down the progression. Presently, there are a few first-line medications/drugs, most of them working as acetylcholinesterase inhibitors, available and with a Food and Drug Administration USA approval to treat AD. Researchers worldwide are focusing on modifying the disease to prevent or to stop further brain damage occurring in the patients. Treatment of patients with acetylcholinesterase inhibitors can provide comfort by increasing the time of independence. Albeit, only treatments carried out on early or middle stage AD can have a positive effect and reduce the memory-related symptoms. Notwithstanding, none of these medications can completely reverse or stop the progression of this disease [41].
The below table provides the details of acetylcholinesterase inhibitory drugs used to treat the mild to moderate to severe AD treatment with their listed mode of action and side effects.
The mechanistic route behind the use of acetylcholinesterase inhibitors as a treatment for AD is still unclear. A recent study suggested that the breakdown of ACh is prevented by these drugs. As the disease progresses, there would be a dip in amount of ACh in the brain, resulting in non-functional cholinesterase inhibitors. None of the above-listed medications can neither completely cures the disease nor enhances the patient’s cognitive or memory skills as they are not designed to improve the neural functions. Hence, a need for an alternate source of medication to cure the AD arises [41]. Ayurveda is a traditional medicinal practice that utilizes numerous plants and herbs to cure effectively a wide range of diseased conditions. Certain herbs have also been used to treat neurocognitive disorders like AD. This review looks into a few such herbal lead compounds for their activity against AD [42].
HERBAL TREATMENT TO CURE ALZHEIMER’S DISEASE
The process of AD drug discovery is critical, riskier, and not cost-effective [43]. Medicinal plants and herbs constitute a complex mixture of phytocompounds of diverse pharmaco-biological significance, which is why herbal therapeutics are recommended to treat several diseases. In fact, plants have remained a major source of drugs across different medicinal practices. Few plants are used in the anti-aging therapies to help maintain homeostasis [44]. In the recent years, herbal formulations have gained significant commercial as well as scientific importance. Those medicinal plants/phytochemicals with the potential to restore memory, stimulate, and strengthen neuronal activity are termed ‘Nervines’. Phytochemical components such as tannins, sterols, flavonoids, alkaloids, lignins, triterpenes, and polyphenols are responsible for the plant’s pharmacological activities such as antioxidants, anti-cholinesterase, anti-inflammatory, and anti-amyloidogenic effects [45]. The studies have shown that using herbs against AD have a remarkable effect on the patient in improving their memory skills due to the increased activity of cholinesterase in the brain [46]. The below mentioned medicinal plants have been extensively evaluated in AD phyto-therapeutics.
Areca catechu
Areca catechu belongs to palm species and is commonly called Betel nut or Betel palm tree. Areca palms are mostly grown in the south and south-east Asian countries of Taiwan, India, Malaysia, Indonesia, Vietnam, Myanmar, and Bangladesh as an important seed crop. The tree is medium-sized with a trunk diameter of 20–30 cm and 20 m tall, with ∼1.5–2 m long leaves which form crowded leaflets. A. catechu seeds are aromatic, consisting of alkaloids such as arecoline and arecaidine, which is said to be of an intoxicating nature. A. catechu nuts have been widely used in pharma industries and for medicinal purposes due to the presence of pharmacological properties. Powdered A. catechu nuts are used to treat urinary disorders and diarrhea [47]. This palm variety has gained a lot of scientific importance owing to the presence of bioactive phytocompounds. Arecanut consists of several phytochemical constitutes like fats (8.1–15.1%), ash (1.1–2.5%), fibers (8.2–15.4%), polyphenols (11.1–29.8%), alkaloids (0.11–0.24%), polysaccharides (17.3–25.7%), and proteins (6.2–9.4%) [48].
Several studies have reported six A. catechu alkaloids, identified as isoguvacine, homoarecoline, arecaidine, arecoline, guavacine, and guavacoline [48]. Reports suggest that guavacine and arecaidine are the hydrolyzed products of guavacoline and arecoline, respectively. Among these alkaloids, areciadine (0.31–0.66%) and arecoline (0.30–0.63%) were found more in number as compared to guavacine (0.19–0.72%) and guavacoline (0.03–0.06%). Additionally, various fatty acid constituents, decanoic acid, stearic acid and monoethylenic acids, are present in minor proportions, while linoleic acid (5.4%), oleic acid (6.2%), hexadecenoic acid (7.2%), palmitic acid (12.7%), lauric acid (19.5%), and myristic acid (46.2%) can be found in major proportions. On the maturity of nut, there is an increase in the number of fibers, alkaloids, polysaccharides, and fats, while the polyphenol levels are found to decrease [48].
Arecoline, a major constituent alkaloid, has been investigated for its toxic and pharmacological effects. The studies reported that Arecoline has a wide range of pharmacological effects over cardiovascular, digestive, nervous and endocrine systems [49]. It is the main active component with significant physiological activities that helps in stimulating the cognitive effects in the central nervous system.
A. catechu in AD therapeutics
The effects of arecoline on the nervous system was investigated to know its effect on dementia. Results suggested an improved memory and cognitive skills by stimulating the M receptor (muscarinic receptor). Thereby, concluding that arecoline is one of the muscarinic agonists that work on memory improvement. The hydrolyzed product of arecoline, arecaidine also showed an inhibitory effect towards the central inhibitory transmitter. Arecoline bypasses the blood-brain barrier to activate the M receptor in the central nervous system. Further investigations proved that arecoline can clinically improve memory, thinking skills, and cognitive functions in patients suffering from AD [50]. Areca has been traditionally used to treat both dementia and depression [47].
Clinical trials were out carried by intravenously administering low dose(s) of Areca extract to patients suffering from mild to moderate AD to confirm its usefulness with no side effects [48].
Acorus calamus
Acorus calamus, belonging to the Acoraceae family, is a tall monocot plant and perennial aromatic herb grown along the river banks. The plant is indigenous to India and can now be found in Sri Lanka, Japan, China, Burma, Russia, Europe, and northern parts of Asia. The rhizomes and leaves of A. calamus are traditionally used as medicine and powdered rhizome is also used as a substitute for nutmeg, ginger, and cinnamon, as a spice. The A. calamus is a very important plant in Ayurveda and is known for its rejuvenating activity against the nervous system and brain, and is also prescribed for digestive problems like bloating, gas, and poor digestive function [51].
A. calamus is one of the widely used medicinal plants as it consists of various amounts of phytoconstituents like alkaloid, lignin, sesquiterpenes, steroids, glycosides, polyphenols, mucilage, flavonoids, tannins, monoterpenes, volatile oil, and saponin compounds. Among these, alpha-asarone and beta-asarone were the two main compounds isolated [52]. Various studies have reported A. calamus to be comprising of anti-ulcer, anti-inflammatory, anti-allergic, anti-microbial, anti-fungal, anti-oxidant, anti-diabetic, anti-cancer, neuroprotective, cardioprotective, insecticidal, and pesticidal properties [53]. α-asarone and β-asrone are the major phytocomponent found in leaves, very well known for their memory enhancement, behavioral modifications, learning performance, and antioxidant potentials [54].
A. calamus is reported to have a mixed composition of fatty acids like the myristic (1.3%), arachidic (3.2%), stearic (7.3%), palmitoleic (16.4%), palmitic (18.2%), linoleic (24.5%), and oleic (29.1%), as identified using gas chromatography. Similarly, the carbohydrates such as fructose (79.1%), maltose (0.2%), and glucose (20.7%) were indicated by paper chromatography [55]. The presence of all these phytoconstituents attribute towards the choice of A. calamus as the best treatment for AD [55].
A. calamus in AD therapeutics
According to Ayurvedic literature and practices, A. calamus plant extract was prescribed for several problems related to digestion, insomnia, epilepsy, cough, fever, and neurosis. The active compounds of this plant were reportedly involved in the acetylcholinesterase degradation [56]. Studies have shown that A. calamus is an effective source of medication that can help in preventing memory impairment or increasing memory by stress reduction by controlling inflammation and reactive oxygen species [57]. Indicating that the plant can be a potential candidate for AD therapeutics.
Eclipta alba
Eclipta alba, commonly called false daisy in English and bhringaraja in Kannada, is an annual weed grown in moist places [58]. It is an herbaceous plant that belongs to family Asteraceae and is very well-known component of the African traditional medicinal practices reported in Asia, used to treat several metabolic and microbial disorders. In Asian countries, E. alba is used for its neuro- and hepato-protection potential(s), apart from its abilities to resolve gastrointestinal issues, hair fall, and snake-venom poisoning [59]. The plant exerts several properties such as antioxidant, relaxant, anti-hypertensive, anti-acetylcholinesterase, lipid-lowering, and anti-leptospiral activities [58]. Such properties of E. alba are owed to presence of phytochemical constituents such as alkaloids, flavonoids (Luteoline, apigenin and orobol), fatty alcohols (hentriacontanol and heptacosanol) polyacetate, phenolic compounds, sterols (stigmasterol and daucosterol), polysaccharides, and saponins (Eclalbatin and dasyscyphin C) [60].
Since ancient times E. alba is used as a traditional medicine to treat epilepsy in most regions of Southern India. The mixture of Eclipta alba leaves with pepper and garlic juice has been orally administered to treat epilepsy [61].
Eclipta alba against Alzheimer’s disease
Traditional African literature highlights the neuropharmacological potentials of E. alba. Research suggests that the plant shows learning and memory modulating properties. Luteolin, an active component of the plant, helps in minimizing the cognitive and memory deficits formed due to cholinergic dysfunction. It also can control the neuronal tissue degeneration obtained as the result of stress perturbations. Thereby indicating that Eclipta alba can be treated against AD, as a potential memory modulator [62].
COMBINATION THERAPY
The currently approved drugs used to treat AD are all cholinesterase inhibitors, which only intend to inhibit the activity of cholinesterase enzyme and not seem to modify or improve the cognitive or memory skills declined due to the disease. AD pathogenesis involves several pathophysiological pathways, indicating it to be a multifactorial disease that needs medication which can act on multimode targets involved in several pathways. This strategy of treatment is realistic and can significantly modify the disease progression [63]. Herbal combination therapy, involving a combination of two or more potent plant extracts in a single dose, can help acquire synergistic effects to reduce the progression of the AD. Such a treatment may also be referred to as a fixed-dose combination. Combinational therapies are not found to be associated with adverse effects as they can overcome toxicity by accessing multi-target mechanisms [64].
Benefits of combination therapy over monotherapy
Monotherapy, as compared to the combination therapy, has several limitations over AD regarding safety and efficacy. Single drug treatment is usually effective at higher doses, causing several adverse effects. Such drugs increase the efficacy of the drug at the cost of the hosts’ tolerance to it in a dose-dependent manner. The different modes of action that are considered in the treatment of combination therapy may provide effectiveness over monotherapy. Herbal combinatorial therapy enhances the efficacy by providing the synergistic effects, tolerability and safety at lower doses, apart from providing additive effects focused toward lowering the disease progression [65]. Based on such reports, relatively new herbal combination treatment approach has different modes of action that may provide additive effects over monotherapy for the effective management of AD. Thereby making it an imperative yet feasible approach to be investigated further to be induced into AD treatment protocols [66].
CONCLUSION
Combination drugs are gaining importance and progress in pharmaceutical technology as they act on multi-mode targets responsible for the cause of AD. These products increase clinical effectiveness, reduce administrative costs, and enhance the patients’ adherence capacity. Further, the plants A. catechu, A. calamus, and E. alba, which possess potential neuroprotective properties, may provide for an efficient yet sustainable pharmaceutical practice if considered for combinational therapies of AD after a detailed mechanistic evaluation.
CONFLICT OF INTEREST
The authors have no conflict of interest to report.
ACKNOWLEDGMENTS
SP, ASJ, CD, SKP and CS acknowledge the support and infrastructure provided by the JSS Academy of Higher Education and Research (JSSAHER), Mysuru, India. KSP thankfully acknowledge the Director, Amrita Vishwa Vidyapeetham, Mysuru campus for various supports. The authors extend their appreciation to the Deanship of Scientific Research at King Saud University for funding this work through Research Group no. RG-1441-313.
REFERENCES
[1] | Querfurth HW , LaFerla FM ((2010) ) Alzheimer’s disease. N Engl J Med 362: , 329–344. |
[2] | Ballard C , Gauthier S , Corbett A , Brayne C , Aarsland D , Jones E ((2011) ) Alzheimer’s disease. Lancet 377: , 1019–1031. |
[3] | Burns A , Iliffe S ((2009) ) Alzheimer’s disease. BMJ 338: , b158. |
[4] | World Health Organization ((2019) ) Dementia. https://www.who.int/news-room/fact-sheets/detail/dementia. |
[5] | Todd S , Barr S , Roberts M , Passmore AP ((2013) ) Survival in dementia and predictors of mortality: A review. Int J Geriatric Psychiatry 28: , 1109–1124. |
[6] | Nationa Health Service ((2014) ) Dementia Diagnosis and Assessment. https://www.nhs.uk/conditions/dementia/diagnosis-tests/. |
[7] | Hsu D , Marshall GA ((2017) ) Primary and secondary prevention trials in Alzheimer disease: Looking back, moving forward. Curr Alzheimer Res 14: , 426–440. |
[8] | Steinberg M , Lyketsos CG ((2012) ) A typical antipsychotic use in patients with dementia: Managing safety concerns. Am J Psychiatry 169: , 900–906. |
[9] | Disease and Injury incidence and Prevalence Collaborators ((2017) ) Global, regional, and national incidence, prevalence, and years lived with disability for 310 diseases and injuries, 1990-2015: A systematic analysis for the Global Burden of Disease Study 2015. Lancet 388: , 1545–1602. |
[10] | Mortality and causes of Death Collaborators ((2017) ) Global, regional, and national life expectancy, all-cause mortality, and cause-specific mortality for 249 causes of death, 1980-2015: A systematic analysis for the Global Burden of Disease Study 2015. Lancet 388: , 1459–1544. |
[11] | Förstl H , Kurz A ((1999) ) Clinical features of Alzheimer’s disease. Eur Arch Psychiatry Clin Neurosci 249: , 288–290. |
[12] | Solfrizzi V , Panza F , Frisardi V , Seripa D , Logroscino G , Imbimbo BP , Pilotto A ((2011) ) Diet and Alzheimer’s disease risk factors or prevention: The current evidence. Expert Rev Neurother 11: , 677–708. |
[13] | Heneka MT , Carson MJ , El Khoury J , Landreth GE , Brosseron F , Feinstein DL , Jacobs AH , Wyss-Coray T , Vitorica J , Ransohoff RM , Herrup K , Frautschy SA , Finsen B , Brown GC , Verkhratsky A , Yamanaka K , Koistinaho J , Latz E , Halle A , Petzold GC , Town T , Morgan D , Shinohara ML , Perry VH , Holmes C , Bazan NG , Brooks DJ , Hunot S , Joseph B , Deigendesch N , Garaschuk O , Boddeke E , Dinarello CA , Breitner JC , Cole GM , Golenbock DT , Kummer MP ((2015) ) Neuroinflammation in Alzheimer’s disease. Lancet Neurol 14: , 388–405. |
[14] | Wilson RS , Barral S , Lee JH , Leurgans SE , Foroud TM , Sweet RA , Graff-Radford N , Bird TD , Mayeux R , Bennett DA ((2011) ) Heritability of different forms of memory in the late onset Alzheimer’s disease family study. J Alzheimers Dis 23: , 249–255. |
[15] | Blennow K , de Leon MJ , Zetterberg H ((2006) ) Alzheimer’s disease. Lancet 368: , 387–403. |
[16] | Waring SC , Rosenberg RN ((2008) ) Genome-wide association studies in Alzheimer disease. Arch Neurol 65: , 329–334. |
[17] | Selkoe DJ ((1999) ) Translating cell biology into therapeutic advances in Alzheimer’s disease. Nature 399: (6738 Suppl), A23–A31. |
[18] | Kim JH ((2018) ) Genetics of Alzheimer’s disease. Dement Neurocogn Disord 17: , 131–136. |
[19] | Strittmatter WJ , Saunders AM , Schmechel D , Pericak-Vance M , Enghild J , Salvesen GS , Roses AD ((1993) ) Apolipoprotein E: High-avidity binding to beta-amyloid and increased frequency of type 4 allele in late-onset familial Alzheimer disease. Proc Natl Acad Sci U S A 90: , 1977–1981. |
[20] | Mahley RW , Weisgraber KH , Huang Y ((2006) ) Apolipoprotein E4: A causative factor and therapeutic target in neuropathology, including Alzheimer’s disease. Proc Natl Acad Sci U S A 103: , 5644–5651. |
[21] | Francis PT , Palmer AM , Snape M , Wilcock GK ((1999) ) The cholinergic hypothesis of Alzheimer’s disease: A review of progress. J Neurol Neurosurg Psychiatry 66: , 137–147. |
[22] | Martorana A , Esposito Z , Koch G ((2010) ) Beyond the cholinergic hypothesis: Do current drugs work in Alzheimer’s disease. CNS Neurosci Ther 16: , 235–45. |
[23] | Ferreira-Vieira TH , Guimaraes IM , Silva FR , Ribeiro FM ((2016) ) Alzheimer’s disease: Targeting the cholinergic system. Curr Neuropharmacol 14: , 101–115. |
[24] | Davies P ((1999) ) Challenging the cholinergic hypothesis in Alzheimer disease. JAMA 281: , 1433–1434. |
[25] | Hardy J , Allsop D ((1991) ) Amyloid deposition as the central event in the aetiology of Alzheimer’s disease. Trends Pharmacol Sci 12: , 383–388. |
[26] | Nistor M , Don M , Parekh M , Sarsoza F , Goodus M , Lopez GE , Kawas C , Leverenz J , Doran E , Lott IT , Hill M , Head E ((2007) ) Alpha- and beta-secretase activity as a function of age and beta-amyloid in Down syndrome and normal brain. Neurobiol Aging 28: , 1493–1506. |
[27] | Polvikoski T , Sulkava R , Haltia M , Kainulainen K , Vuorio A , Verkkoniemi A , Niinistö L , Halonen P , Kontula K ((1995) ) Apolipoprotein E, dementia, and cortical deposition of beta amyloid protein. N Engl J Med 333: , 1242–1247. |
[28] | Lacor PN , Buniel MC , Furlow PW , Clemente AS , Velasco PT , Wood M , Viola KL , Klein WL ((2007) ) Aβ oligomer-induced aberrations in synapse composition, shape, and density provide a molecular basis for loss of connectivity in Alzheimer’s disease. J Neurosci 27: , 796–807. |
[29] | Nikolaev A , McLaughlin T , O’Leary DD , Tessier-Lavigne M ((2009) ) APP binds DR6 to trigger axon pruning and neuron death via distinct caspases. Nature 457: , 981–989. |
[30] | Paul RT , Kate OC , Warren PT , Wickliffe CA ((2003) ) Roles of amyloid precursor protein and its fragments in regulating neural activity, plasticity and memory. Prog Neurobiol 70: , 1–32. |
[31] | Mudher A , Lovestone S ((2002) ) Alzheimer’s disease-do tauists and baptists finally shake hands. Trends Neurosci 25: , 22–26. |
[32] | Goedert M , Spillantini MG , Crowther RA ((1991) ) Tau proteins and neurofibrillary degeneration. Brain Pathol 1: , 279–286. |
[33] | Iqbal K , Alonso Adel C , Chen S , Chohan MO , El-Akkad E , Gong CX , Khatoon S , Li B , Liu F , Rahman A , Tanimukai H , Grundke-Iqbal ((2005) ) Tau pathology in Alzheimer disease and other tauopathies. Biochim Biophys Acta 1739: , 198–210. |
[34] | Kametani F , Hasegawa M ((2018) ) Reconsideration of amyloid hypothesis and tau hypothesis in Alzheimer’s disease. Front Neurosci 12: , 25. |
[35] | Desikan RS , Cabral HJ , Hess CP , Dillon WP , Glastonbury CM , Weiner MW , Schmansky NJ , Greve DN , Salat DH , Buckner RL , Fischl B ; Alzheimer’s Disease Neuroimaging Initiative ((2009) ) Automated MRI measures identify individuals with mild cognitive impairment and Alzheimer’s disease. Brain 132: , 2048–2057. |
[36] | Tiraboschi P , Hansen LA , Thal LJ , Corey-Bloom J ((2004) ) The importance of neuritic plaques and tangles to the development and evolution of AD. Neurology 62: , 1984–1989. |
[37] | Mendez MF ((2006) ) The accurate diagnosis of early-onset dementia. Int J Psychiatry Med 36: , 401–412. |
[38] | Waldemar G , Dubois B , Emre M , Georges J , McKeith IG , Rossor M , Scheltens P , Tariska P , Winblad B ; EFNS ((2007) ) Recommendations for the diagnosis and management of Alzheimer’s disease and other disorders associated with dementia: EFNS Guideline. Eur J Neurol 14: , 1–26. |
[39] | National Collaborating, and Mental Health, Supporting people with dementia and their carers in health and social care ((2006) ) Quick Reference Guide. https://dementiapartnerships.com/wp-content/uploads/sites/2/nice-cg42.pdf. |
[40] | Schroeter ML , Stein T , Maslowski N , Neumann J ((2009) ) Neural correlates of Alzheimer’s disease and mild cognitive impairment: A systematic and quantitative meta-analysis involving 1351 patients. Neuroimage 47: , 1196–1206. |
[41] | How is Alzheimer’s disease treated? 2020,https://www.nia.nih.gov/health/how-alzheimers-disease-treated. |
[42] | Klafki HW , Staufenbiel M , Kornhuber J , Wiltfang J ((2006) ) Therapeutic approaches to Alzheimer’s disease. Brain 129: , 2840–2855. |
[43] | Rao RV , Descamps O , John V , Bredesen DE ((2012) ) Ayurvedic medicinal plants for Alzheimer’s disease: A review. Alzheimers Res Ther 4: , 22. |
[44] | Singh AK , Gupta A , Ak M , Gupta V , Bansal P , Kumar S ((2010) ) Medicinal plant for curing Alzheimer’s disease. Int J Pharm Biol Arch 1: , 108–114. |
[45] | Tian J , Shi J , Zhang X , Wang Y ((2010) ) Herbal therapy: A new pathway for the treatment of Alzheimer’s disease. Alzheimers Res Ther 2: , 30. |
[46] | Obulesu M , Rao DM ((2011) ) Effect of plant extracts on Alzheimer’s disease: An insight into therapeutic avenues. J Neurosci Rural Pract 2: , 56–61. |
[47] | Preetee J , Pradeep K , Singh VK , Singh DK ((2011) ) Areca catechu- A valuable herbal medicine against different health problems. Res J Med Plants 5: , 145–152. |
[48] | Bhat Keshava S , Ashwin D , Mythri S , Sukesh B ((2017) ) Arecanut (Areca catechu L) decreases Alzheimer’s disease symptoms: Compilation of research works. J Med Plants Stud 5: , 4–9. |
[49] | Malviya M , Sunil Kumar YC , Mythri RB , Venkateshappa C , Subhash MN , Rangappa KS ((2009) ) Muscarinic receptor 1 agonist activity of novel N-aryl carboxamide substituted 3-morpholino arecoline derivatives in Alzheimer’s presenile dementia models. Bioorg Med Chem 17: , 5526–5534. |
[50] | Liu YJ , Peng W , Hu MB , Xu M , Wu CJ ((2016) ) The pharmacology, toxicology and potential applications of arecoline: A review. Pharm Biol 54: , 2753–2760. |
[51] | Farooqui AA , Farooqui T , Madan A , Ong JH , Ong WY ((2018) ) Ayurvedic medicine for the treatment of dementia: Mechanistic aspects. Evid Based Complement Alternat Med 2018: , 2481076. |
[52] | Shukla PK , Khanna VK , Ali MM , Maurya RR , Handa SS , Srimal RC ((2002) ) Protective effect of acorus calamus against acrylamide induced neurotoxicity. Phytother Res 16: , 256–260. |
[53] | Das BK , Swamy AV , Koti BC , Gadad PC ((2019) ) Experimental evidence for use of Acorus calamus (Asarone) for cancer chemoprevention. Heliyon 5: , e01585. |
[54] | Subamalani S , Sasikumar A , Manikandan S , Ramaswamy C ((2020) ) A study of neurobehavioral and biochemical activities of Acorus calamus linn. on restraint stressed Wistar rats. Int J Pharm Sci Res 9: , 4832–4841. |
[55] | Balakumbahan R , Rajamani K , Kumanan K ((2010) ) Acorus calamus: An overview. J Med Plants Res 4: , 2740–2745. |
[56] | Sanka N , Santhipriya N , Nadendla RR ((2018) ) An updated review on Anti-Alzheimer’s herbal drugs. J Drug Deliv Ther 8: , 360–372. |
[57] | Esfandiari E , Ghanadian M , Rashidi B , Mokhtarian A , Vatankhah AM ((2018) ) The effects of Acorus calamus L. in preventing memory loss, anxiety, and oxidative stress on lipopolysaccharide-induced neuroinflammation rat models. Int J Prev Med 9: , 85. |
[58] | Vidyashree HM , Malathi S , Rajan R , Devi RS ((2018) ) Neuroprotective role of herb Eclipta alba Linn. on acute and chronic unpredictable stress induced behavioral, biochemical and neuro-transmitter alteration in Wistar male albino rat brain. Int J Pharm Sci Res 9: , 2244–2257. |
[59] | Guenné S , Ouattara N , Noufou O , Ciobica A , Hilou A , Kiendrebeogo M ((2019) ) Phytochemistry and neuroprotective effects of Eclipta Alba (L.). J Complement Integr Med 1: , /j/jcim.2019.17.issue-1/jcim-2019-0026/jcim-2019-0026.xml.. |
[60] | Jahan R , Al-Nahain A , Majumder S , Rahmatullah M ((2014) ) Ethnopharmacological significance of Eclipta alba (L.) Hassk. (Asteraceae). Int Sch Res Notices 2014: , 385969. |
[61] | Shaikh MF , Sancheti J , Sathaye S ((2013) ) Effect of Eclipta alba on acute seizure models: A GABAA-mediated effect. Indian J Pharm Sci 75: , 380–384. |
[62] | Monalisa J , Swati M , Pal A , Mishra SS ((2014) ) Memory enhancing activity of Eclipta alba in albino rats: A correlation with anticholinesterase activity. Int J Pharm Clin Res 6: , 179–185. |
[63] | Ãlvarez XA , Linares C , Masliah E ((2012) ) Combination drug therapy for the treatment of Alzheimer’s disease. Eur Neurol Rev 7: , 92–101. |
[64] | Wertheimer AI , Morrison A ((2002) ) Combination drugs: Innovation in pharmacotherapy. P T 27: , 44–49. |
[65] | Lu DY , Chen EH , Wu HY , Lu TR , Xu B , Ding J ((2016) ) Anticancer drug combinations, how far we can go through? Anticancer Agents Med Chem 17: , 21–28. |
[66] | Rotte A ((2019) ) Combination of CTLA-4 and PD-1 blockers for treatment of cancer. J Exp Clin Cancer Res 38: , 255. |