Neem Derivatives Inhibits Tau Aggregation1
Abstract
Tau is a phosphoprotein with natively unfolded conformation that functions to stabilize microtubules in axons. Alzheimer’s disease pathology triggers several modifications in tau, which causes it to lose its affinity towards microtubule, thus, leading to microtubule disassembly and loss of axonal integrity. This elicit accumulation of tau as paired helical filaments is followed by stable neurofibrillary tangles formation. A large number of small molecules have been isolated from Azadirachta indica with varied medicinal applications. The intermediate and final limonoids, nimbin and salannin respectively, isolated from Azadirachta indica, were screened against tau aggregation. ThS and ANS fluorescence assay showed the role of intermediate and final limonoids in preventing heparin induced cross-β sheet formation and also decreased hydrophobicity, which are characteristic nature of tau aggregation. Transmission electron microscopy studies revealed that limonoids restricted the aggregation of tau to fibrils; in turn, limonoids led to the formation of short and fragile aggregates. Both the limonoids were non-toxic to HEK293T cells thus, substantiating limonoids as a potential lead in overcoming Alzheimer’s disease.
INTRODUCTION
Alzheimer’s disease (AD) is one of the major neurodegenerative diseases related to decline in cognitive functions and an estimate of 47 million people worldwide are suffering from AD [1]. The normal functioning of the neuronal cells is usually interrupted by accumulation of aberrant proteins, leading to AD. The axonal tau and trans-membrane amyloid-β are the two majorly effected proteins [2]. The processing of amyloid-β from its precursor protein by secretases is essential for cellular functions [3, 4]. In healthy conditions, amyloid-β is processed by α-secretase followed by γ-secretase; the intervention of β-secretase instead of α-secretase generates extracellular amyloid plaques that deposits on the neuronal cells [5]. On the other hand, pathology of tau is predominantly associated with frontotemporal dementia with parkinsonism-17 (FTDP-17) mutations and its post-translational modifications [6]. These modifications change the overall charge of tau thus, hindering its interaction with microtubules, tau aggregation and eventually neuronal death. Molecules from natural and synthetic origin have been screened to validate their role in overcoming AD. Natural molecules widely includes extracts form plant and fungal origin such as oleocanthal, curcumin, tabersonine, cinnamaldehyde, azaphilones, 2, ω-dihydroxyemodin, asperthecin, asperbenzaldehyde, etc. [7–10]. Oleocanthal, cinnamon, and curcumin prevent aggregation in both amyloid-β and tau. Oleocanthal increased amyloid-β clearance in the brain by increasing levels of receptors, LDL lipoprotein receptor related protein-1 that was essential for amyloid-β transport. Oleocanthal induced the expression of neprilysin and insulin degrading enzymes that were responsible for degradation of amyloid-β [11]. In vitro studies reveal the efficacy of oleocanthal in inhibiting full-length tau by modifying lysine residues [12]. The medicinal values of cinnamon is used against various ailment such as wound healing, cardiovascular diseases, etc. Cinnamon administration in AD transgenic mice reduced the formation of amyloid-β oligomers and enhanced cognitive behavior [13]. Cinnamon disintegrate paired helical filaments in AD brain and prevent in vitro tau aggregation [14]. Curcumin has multifaceted role in preventing aggregation of tau and amyloid-β, and is a very well-known molecule for AD; it mitigates synaptic loss, oxidative stress, and behavioral deficits [15–17]. The poor bioavailability and metabolic instability of curcumin limits its therapeutics application hence, leading to the development of curcumin derivatives [18–20]. To overcome the limitations of natural molecules, a large number of synthetic molecules such as phenothiazine dyes, edaravone, cyanine dyes, modified peptides, etc., have been developed [21–23]. Among them, phenothiazine and cyanine dyes alleviated AD pathology by targeting amyloid-β and tau aggregates [21, 24–26]. Apart from tau and amyloid-β, several kinases are responsible for tau hyperphosphorylation such as cyclin-dependent kinase 5 (CDK5), fyn kinase, and GSK3β [27–30]. A large number of kinase inhibitors were identified and screened for their therapeutic role in AD [31–33]. Furthermore, the application of these molecules are limited to their pharmacokinetic property and permeabilization across blood-brain barrier [34]. Despite a huge library of small molecules against AD, most of them are a failure in various phase of clinical trials [35, 36]. Hence, there is a necessity to screen molecules against different targets of AD.
In the present study, we isolated intermediate and final limonoids, nimbin and salannin respectively, from the fruit of Azadirachta indica (Fig. 1A, B). Limonoids are tetranortriterpenoid class of molecules with large number of biological activity that includes, anti-fungal, anti-bacterial, anti-inflammatory, anti-cancer, and anti-feedant [37]. Previously, a wide range of limonoids were isolated from Azadirachta indica and screened for their anti-cancer property [38]. The role of limonoids in neurodegenerative disorders are poorly known [39]. In present study, the effect of limonoids against aggregation of tau was performed by fluorescence assay and analyzing the morphology of tau aggregates followed by cell viability assay. The current results suggest that intermediate and final limonoids prevents tau aggregation and increases cell viability. The limonoids being non-toxic to cells and their role in preventing in vitro tau aggregation propose them to be a promising molecule in overcoming tau pathology.
Fig.1
Intermediate and final limonoids decrease aggregation of tau. A,B) The final limonoids were isolated from neem kernel of Azadirachta indica and the structure of nimbin and salannin. C) The full-length tau (hTau40 wt) is composed of 441 amino acids and has two inserts towards the N-terminal. The number of inserts vary from 0 to 2 based on the isoforms. This is followed by a proline rich region which is known to involve in interaction with various proteins such as microtubule, kinases, etc. The four repeats towards the C-terminal are also known as microtubule-binding domain, named on the basis of its function. Tau undergoes alternative splicing and results in six isoforms which have either three or four repeats. The expression of these isoforms is developmentally regulated and is altered during AD pathology. D, E) The inhibition of full-length tau aggregation by nimbin and salannin was probed by ThS fluorescence and plotted in terms of percentage inhibition at 120 h. F) The aggregation inhibition in terms of percentage was plotted. It indicated that nimbin and salannin showed similar effects in inhibiting tau aggregation. G) In in vitro condition heparin induces tau aggregation into thick and extended fibril like structure. H, I) The presence of nimbin and salannin prevents extensive aggregation of tau and instead leads to the formation of thin, short, and fragile aggregates. The significance was calculated using SigmaPlot 10.0 and ***, ** and * indicates p value <0.001, <0.01 and <0.05 respectively. ns is not significant where p≥0.05.
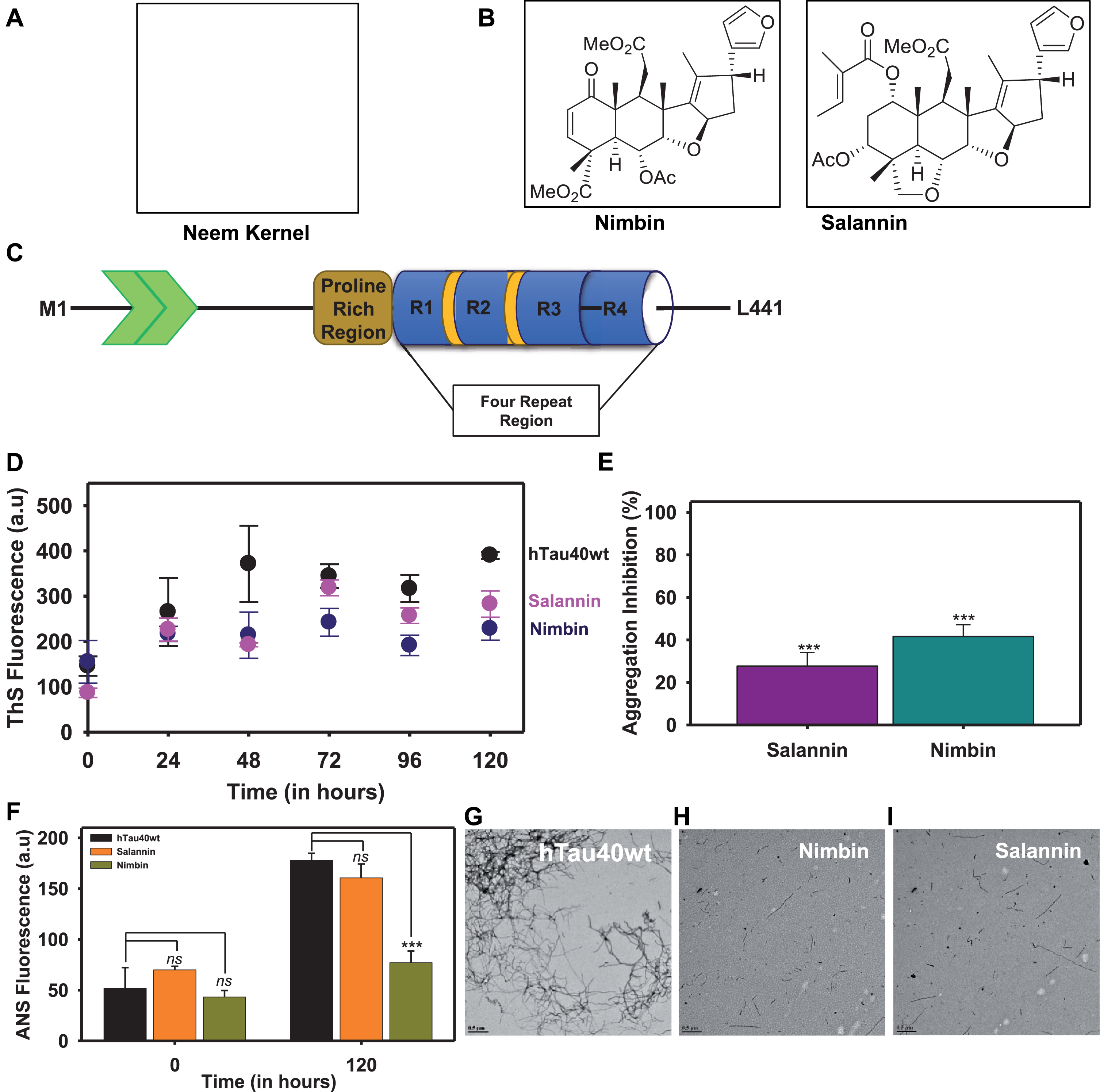
MATERIALS AND METHODS
Plant material and chemicals
Neem fruits were collected from Aurangabad, Maharashtra (India). HPLC grade solvents were procured from Sigma. LC-MS grade solvents were purchased from JT Baker.
Extraction of neem fruit coat
Neem fruit coat separated from the kernel (1 Kg) was crushed and homogenized using blender. The homogenized tissue was subjected to repeated methanol extraction for three times over a period of 3 h (2.5 L X 3). The pooled methanol extract was concentrated under reduced pressure and then partitioned between ethyl acetate and water. Organic layer obtained was concentrated to obtain crude extract (24.4 g).
Isolation of C-seco limonoids by MPLC
Crude limonoids extract of 2 g adsorbed on 60–120 mesh silica gel and fractionated on 40 g normal phase silica gel prepacked cartridge having flow rate 25 ml/min and monitored by using UV detector at 240 nm. A gradient solvent system of ethylacetate in petroleumether (20% EtOAc: Petether; 0.0–10.0 min, 25% EtOAc: Petether; 15.0 min, 10.0 min hold, 30% EtOAc: Petether; 30.0 min, 10.0 min hold, 35% EtOAc: Petether; 45.0 min, 10.0 min hold, 40% EtOAc: Petether; 60.0 min, 10.0 min hold, 45% EtOAc: Petether; 75.0 min, 10.0 min hold, 70% EtOAc: Petether; 90.0 min, 15.0 min hold) was used. Fractions were collected in 25 ml tubes. Similar fractions were combined and concentrated to get six fractions. These fractions were adsorbed on celite and individually subjected to reverse phase column chromatography by using 43 g reverse phase silica gel prepacked cartridge with 30 ml/min flow rate. Gradient solvent system of 50% MeOH: H2O; 0.0–10.0 min, 55% MeOH: H2O; 15.0 min, 15 min hold, 60% MeOH: H2O; 35.0 min, 25.0 min hold, 70% MeOH: H2O; 65.0 min, 15.0 min hold, was used. The purified compounds were identified as nimbin and salannin based on various spectral data such as HRPM, MS/MS, and NMR with that of reported [58].
LC-(ESI)-HRMS condition
The purity of the isolated limonoids were further confirmed by subjecting to ESI-Orbitrap MS associated with Accela 1250 pump and Accela open AS (Thermoscientific Q Exactivetrademark). 5.0μl of the purified limonoids dissolved in methanol (at concentration of 0.1 mg/mL MeOH) was injected on to a Waters Acquity BEH C18 column (1.7μm, 2.1 mm X 100 mm) with gradient solvent system of methanol:water (0.1% formic acid) with a flow rate of 300μL/min. The samples were analyzed in positive ionization mode in the scan range of m/z 100 to 1000. Limonoids isolated were found to be over 99.5% pure (Supplementary Figures 2 and 3).
Preparation of hTau40 wt
hTau40 wt was expressed in E. coli as described previously [59]. The transformed E. coli was grown at 37°C till the OD at A600 reached 0.5 to 0.6. The cells were induced by 0.5 mM IPTG and allowed to grow for further 4 h before harvesting. The cells were pelleted at 4000 rpm for 10 min, the obtained pellet was re-suspended in cell lysis buffer composed of 50 mM MES at pH 6.8, 1 mM EGTA, 2 mM MgCl2, 5 mM DTT, 1 mM PMSF, and protease inhibitor cocktail. The protein was extracted by lysing the cells at 15,000 psi in constant cell disruption system. The obtained lysate was boiled to 90°C after adding 0.5 M NaCl and 5 mM DTT to final concentration. The resultant was subjected to centrifugation at 40,000 rpm at 4°C for 45 min. The supernatant was dialyzed against buffer A constituted with 50 mM NaCl. The protein was subjected to centrifugation after overnight dialysis, following which it was loaded onto pre-equilibrated cation exchange column. The protein bound to the column was eluted by gradually increasing the NaCl gradient to 1 M. The eluted protein quality was analyzed by SDS-PAGE and the confirmed fractions were pooled, concentrated through size-exclusion chromatography by using 1 M PBS and 2 mM DTT. The eluted protein was analyzed by SDS-PAGE. Thus confirmed fractions were pooled and concentrated using 3 kDa cut-off centricons. The protein concentration was estimated by bichinchoninic acid (BCA) method.
Aggregation of tau
In vitro the aggregation of 20μM hTau40 wt was induced by poly-anionic co-factors such as RNA, arachidonic acid, and heparin. Tau aggregation was induced by heparin (17,500 Da) at the ratio of 4:1 (tau:heparin) in presence of 20 mM BES pH 7.4, 25 mM NaCl, 1 mM DTT, 0.01% NaN3 and protease inhibitor cocktail. Tau was incubated at 37°C for aggregation. The role of limonoids in preventing aggregation was analyzed by incubating tau in presence of nimbin and salannin at 400μM concentrations.
Fluorescence assay
The aggregation of tau was monitored by Thioflavin S (ThS) and 8-anilinonaphthalene-1-sulfonic acid (ANS), which reveals the content of β-sheet and hydrophobicity in tau during aggregation. 2μM tau was incubated with 8μM ThS and 40μM ANS prepared in 50 mM ammonium acetate at pH 7.0. The fluorescence measurement for ThS was carried out at the excitation of 440 nm and the spectra was recorded at 521 nm. Similarly, the ANS was measured by exciting at 390 nm and recording the spectra at 475 nm. The measurements were taken in Tecan Infinite 200 PRO multimode micro plate reader.
TEM analysis
Tau aggregates exhibit a typical morphology of filament like structure which was analyzed by TEM at 120 h. Tau was diluted to 1μM and applied on 400 mesh copper grids that were carbon coated. The grid was incubated with 2% uranyl acetate to produce an electron dense layer. The sample was analyzed by Tecnai T20 at 200 kV.
SDS-PAGE analysis
Tau aggregation leads to the formation of higher order aggregates, which were analyzed by SDS-PAGE at 0, 24, and 120 h. Tau was centrifuged at 60,000 rpm for 1 h, followed by separation of supernatant (S) and pellet (P) that was analyzed against the total (T) tau that was not subjected to sedimentation. The extent of aggregation was revealed by the presence of tau in pellet as analyzed by SDS-PAGE. hTau40 wt was resolved by using miniVE Vertical Electrophoresis System purchased from GE Healthcare Life Sciences.
Cell viability assay
HEK293T cell line was cultured in DMEM (Dulbecco modified eagle’s media) with 10% FBS and 100μg/mL of penicillin and streptomycin. 10,000 cells/well in 96-well plates were seeded and incubated at 37°C for 12 h at 5% CO2. Limonoids were added at a concentration of 50 nM and 50μM; in presence of 1μM tau aggregates limonoids were added at 1, 2, and 5μM concentration at final volume of 100μL with 5% DMSO as a control and incubated for 24 h at 37°C, 5% CO2. After the incubation, MTT (Thiazolyl blue tetrazolium bromide) reagent was added at a concentration of 0.5 mg/mL in each well and incubated for 3 h at 37°C, 5% CO2. The formazan end product was solubilized by adding 100μL DMSO and the absorption was measured at 570 nm in Tecan Infinite 200 PRO multimode micro plate reader.
Statistical analysis
The triplicates in each data sets were analyzed by unpaired Student’s t-test using SigmaPlot 10.0. Data are represented as mean±s.e.m. The statistical significance (p-value) was represented as ***, **, and * which indicates p < 0.001, <0.01, and 0.05 respectively. p value >0.05 indicates not significant and is represented as ‘ns’.
RESULTS
Tau aggregation modulated by limonoids
The longest isoform of tau, hTau40 wt has 441 amino acids comprising of two inserts, a proline rich region and four imperfect repeats (Fig. 1C). The effect of intermediate and final limonoids were studied by inducing in vitro tau aggregation. The signature cross-β structure and hydrophobic patches formed during tau aggregation were analyzed by ThS and ANS respectively (Fig. 1D, E). In presence of nimbin and salannin the ThS fluorescence assay showed decrease in intensity [40]. This suggest that limonoids prevent the formation of β-sheet structure in tau. Similar observations were obtained with ANS fluorescence, which indicate lack of hydrophobic patches that are typically present in aggregates [41]. Nimbin exhibits 41.5% inhibition in tau aggregation whereas; salannin exhibits 27.6% (Fig. 1F).
Characterization of tau aggregates by TEM
The morphology of tau aggregates monitored by TEM are observed as long and thick filamentous structure with periodic twists (Fig. 1G). At 0 h, tau aggregation was not observed in presence or absence of limonoids (Supplementary Figure 1). Limonoids prevented extensive aggregation of tau and resulted in thin, short and fragile fragments of tau (Fig. 1H, I). These results indicate that intermediate and final limonoids play potential role in inhibiting tau aggregation.
Higher order aggregates of tau analyzed by SDS-PAGE
Sedimentation of tau was carried out at 60,000 rpm for 1 h, after which the supernatant was separated from pellet and analyzed by SDS-PAGE. The higher order aggregates are analyzed by SDS-PAGE in distinct manner [42]. At 0 h, nimbin formed soluble higher order aggregates that were separated in supernatant (Fig. 2A). After 24 h of incubation, tau was separated in pellet, which indicates tau aggregation. In case of nimbin, tau was separated in supernatant and pellet. In presence of salannin, tau was sedimented in the pellet (Fig. 2B). At 120 h of incubation, salannin showed degradation of tau in supernatant. By comparing with hTau40 wt, the results suggest that tau aggregation was more in presence of salannin when compared to nimbin (Fig. 2C).
Fig.2
SDS-PAGE analysis of tau aggregates. A) Tau was subjected to sedimentation assay, followed by the analysis of supernatant and pellet in comparison with the total. At 0 h, full-length tau does not form higher molecular weight aggregates. But in the presence of limonoids, these higher molecular weight species are observed, which were more prominent in presence of nimbin. B) At 24 h of incubation, tau exhibits the formation of aggregates as majority of tau was getting separated in pellet. Moreover, similar observation was obtained in presence of salannin. In case of nimbin, tau exhibited partial aggregation as equal amounts of tau was observed in supernatant and pellet. C) Later at 120 h, tau exhibited maximal aggregation which was witnessed by the presence of higher order species in pellet. Nimbin and salannin also showed maximal aggregation, where salannin led to complete aggregation of tau.
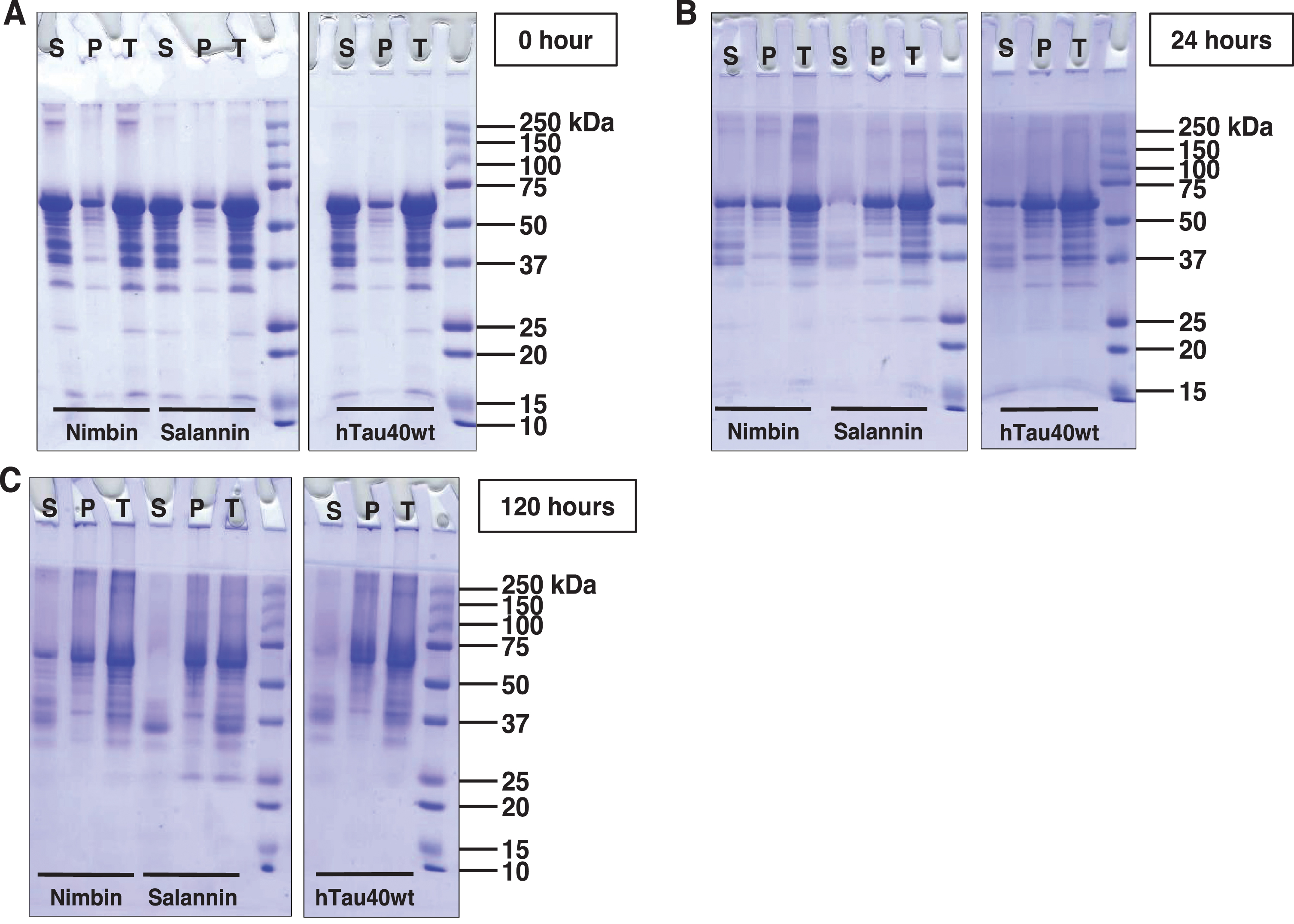
Limonoids inhibited tau mediated cytotoxicity
Nimbin and salannin are one of the major constituents of Azadirachta indica, and tauopathy includes cellular toxicity and mitochondrial dysfunction [43]. Cell-based studies were conducted to study whether tau aggregates are toxic to neuronal cells, or are modulated by limonoids. HEK293T cells were treated with limonoids at 50 nM and 50μM concentrations, nimbin showed 82% and 84% viability respectively and salannin showed 81% and 78% viability respectively (Fig. 3A). The pre-formed tau aggregates when added extracellularly to HEK293T it showed only 32% cellular viability at 10μM concentration (Fig. 3B). The tau-mediated cytotoxicity was rescued by treatment of nimbin and salannin even at lower concentration compared to cytotoxic dose. Nimbin and salannin reduced tau toxicity completely (100% cell viability) at 2μM and 5μM respectively, where salannin showed dose dependent rescue of cytotoxicity. These results clearly suggest the potency of limonoids in alleviating tau-mediated toxicity (Fig. 4).
Fig.3
Biocompatibility of limonoids. A) 104 cells/well were seeded in 96 well plate and incubated with 0.05 and 50μM concentrations of limonoids at 37°C 5% CO2 for 24 h, followed by MTT assay and calculation of % cell viability. Both nimbin and salannin have lesser cytotoxic effect and may be potent as drug candidate. B) HEK293T cells were seeded in 96 well plate and co-treated with 10μM human tau aggregates and then with different concentrations (1, 2, and 5μM) of limonoids separately and incubated for 24 h at 37°C 5% CO2. Cell viability was determined by MTT assay where nimbin (2μM) were more effective to reduce tau aggregates mediated toxicity at lower concentration than salannin (5μM) in HEK293T cells. The significance was calculated using SigmaPlot and ***, ** and * indicates p value <0.001, <0.01 and <0.05 respectively. ns is not significant where p≥0.05.
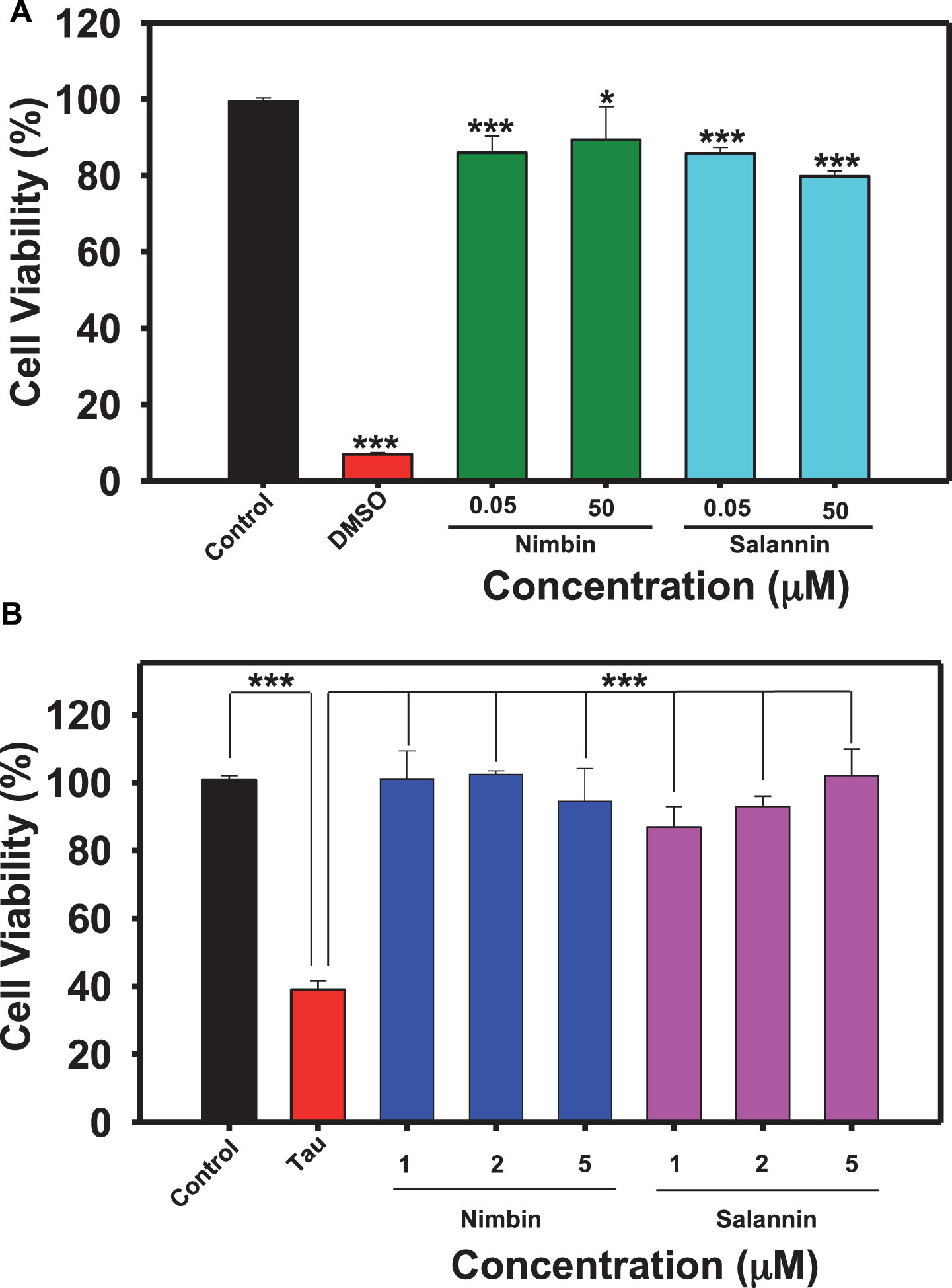
Fig.4
Inhibitory effect and biocompatibility of limonoids. The tendency of tau protein to aggregate was prevented by intermediate and final limonoids. These limonoids were non-toxic to HEK293T cells and also rescued HEK293T from tau aggregates induced toxicity.
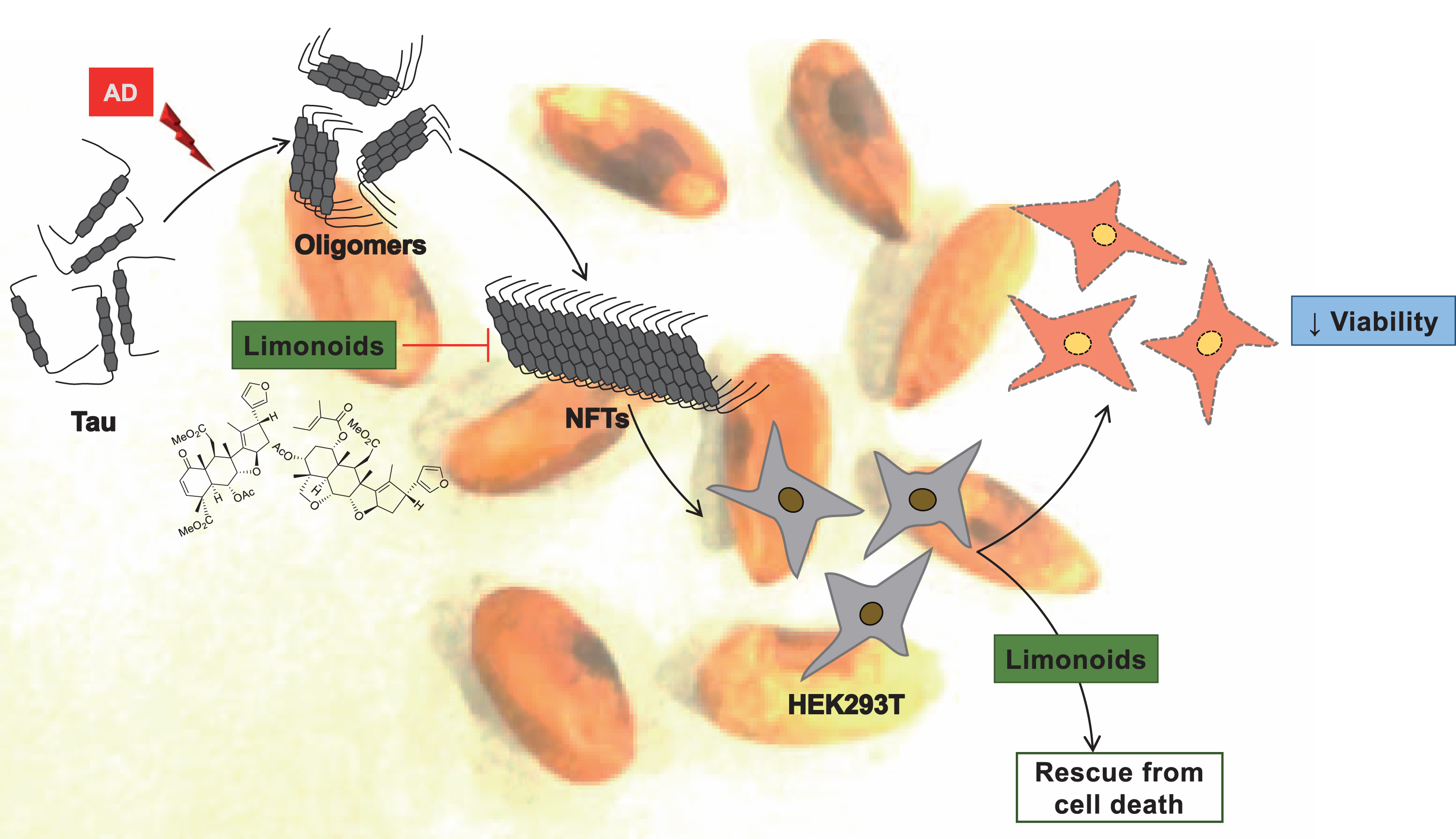
DISCUSSION
It has become challenging over years to understand the multi-faceted pathology of AD, as novel pathways and effectors associated with it are being disclosed. The physiological functions of neuronal cell are disturbed due to the transition of native proteins to pathological state and their accumulation. Thus, depriving the protein to execute their normal function and leading to neuronal death. Several studies have been carried out to understand the AD pathology, but even after 70 years of its identification the complex network of pathology is still unresolved. Researchers are screening several molecules against amyloid-β and tau aggregation, as well as other effectors of tau such as kinases and proteases [44, 45]. These molecules although are initially effective, they failed in the later clinical trials. Recently, methylene blue and idalopirdine failed in phase III clinical trials [46, 47]. Hence, there is a great need to screen small molecules and explore their role in overcoming AD. In the present work, we have isolated intermediate and final limonoids form Azadirachta indica, and analyzed its potential role in preventing tau aggregation in vitro. We studied the effect of final limonoids in overcoming toxicity due to tau aggregates in HEK293T cells.
The ThS fluorescence for aggregation inhibition indicates that nimbin has more potential in reducing tau aggregation when compared to salannin. This distinctive outcome would be driven by the side chain arrangement in nimbin and salannin. The intrinsic disordered nature of tau makes it feasible to interact with different small molecules. The inhibitory effect on tau aggregation is majorly driven by the motif of tau involved in interaction with small molecules, as oleocanthal prevents tau aggregation by interacting with lysine residue via formation of Schiff’s base [12, 48]. Morales et al. and Okuda et al. reported the effect of curcumin and its derivative PE859 in decreasing the ThS fluorescence, indicating the inhibitory role on tau aggregation [49]. The derivatives of azaphilones inhibited aggregation of tau by forming amorphous tau aggregates. This was due to change in the side chain of azaphilones [50]. Here in case of final limonoids, they prevent aggregation as evidenced by small and fragile fragments of tau. In sedimentation assay tau is separated in supernatant and pellet based on its nature, i.e., monomer, soluble oligomer or high molecular weight aggregates [48]. The tau protein was separated in both, supernatant and pellet fraction as SDS-stable higher molecular weight aggregates. Salannin formed insoluble higher molecular weight aggregates as most of the tau was seen in pellet. Furthermore, salannin also induced degradation of tau and it probably interacts with tau making it more flexible to degrade.
Previous studies elucidated neem leaf extracts to have profound anti-bacterial activity, pesticidal activity, as well as wound healing activity [51–53]. It has potential role as anti-inflammatory in smoke and LPS induced pulmonary inflammation and can reduce hepatotoxicity by decreasing the rate of apoptosis and increasing antioxidant activity in female rats [54, 55]. Moreover, the extracts have anti-diabetic, anti-ulcer, and anti-cancer properties [56]. Previous studies support the uptake of limonoids in transgenic mouse model indicating their nontoxic nature and bioavailability [39]. We initially analyzed the toxicity of final limonoids and further extended our study to understand if these compounds can reverse the toxicity induced by tau aggregates [57]. Here, we observed that limonoids were non-toxic and reverted tau mediated toxicity at 1μM concentration which, indicated efficacy of limonoids in AD pathology.
Conclusion
AD is one of the most common form of dementia with increasing rate of mortality each year. The drugs against various effectors of tau do not aid in preventing AD and moreover failure of several drugs in clinical trials encourage to screen and identify potential lead. Here, in our study we have isolated intermediate and final limonoids from Azadirachta indica, a medicinal plant, to study its effect on tau aggregation. The results suggest that limonoids are effective in inhibiting formation of tau aggregates. The cell-based studies suggest that limonoids form non-toxic conformers of tau aggregates and was able to overcome oxidative stress. All these observations suggest limonoids to be a potential lead molecule against AD.
CONFLICT OF INTEREST
The authors have no conflict of interest to report.
ACKNOWLEDGMENTS
This work was supported in part by grants from the Department of Science and Technology-Science and Engineering Research Board (DST-SERB, Young Investigator grant): SB/YS/LS-355/2013, DST-SERB, Health Sciences EMR/000306/2017 and in-house CSIR-National Chemical Laboratory grant MLP029526.
Nalini V. Gorantla and Rashmi Das acknowledges the University Grant Commission (UGC) for the fellowship. We acknowledge Prof. Roland Brandt from University of Osnabruck, Germany and Prof. Jeff Kuret from Ohio State University College of Medicine, USA for being generous in providing us tau constructs.
SUPPLEMENTARY MATERIAL
[1] The supplementary material is available in the electronic version of this article: http://dx.doi.org/10.3233/ADR-190118.
REFERENCES
[1] | Alzheimer’s Association ((2018) ) 2018 Alzheimer’s disease facts and figures. Alzheimers Dement 14: , 367–429. |
[2] | Chow VW , Mattson MP , Wong PC , Gleichmann M ((2010) ) An overview of APP processing enzymes and products. Neuromolecular Med 12: , 1–12. |
[3] | van der Kant R , Goldstein LS ((2015) ) Cellular functions of the amyloid precursor protein from development to dementia. Dev Cell 32: , 502–515. |
[4] | Pearson HA , Peers C ((2006) ) Physiological roles for amyloid β peptides. J Physiol 575: , 5–10. |
[5] | Zhang YW , Thompson R , Zhang H , Xu H ((2011) ) APP processing in Alzheimer’s disease. Mol Brain 4: , 3. |
[6] | Combs B , Gamblin TC ((2010) ) FTDP-17 tau mutations induce distinct effects on aggregation and microtubule interactions. Biochemistry 51: , 8597–8607. |
[7] | Calcul L , Zhang B , Jinwal UK , Dickey CA , Baker BJ ((2010) ) Natural products as a rich source of tau-targeting drugs for Alzheimer’s disease. Future Med Chem 4: , 1751–1761. |
[8] | Paranjape SR , Chiang YM , Sanchez JF , Entwistle R , Wang CC , Oakley BR , Gamblin TC ((2014) ) Inhibition of Tau aggregation by three Aspergillus nidulans secondary metabolites: 2, ω dihydroxyemodin, asperthecin, and asperbenzaldehyde. Planta Med 80: , 77–85. |
[9] | Tang M , Taghibiglou C ((2014) ) The mechanisms of action of curcumin in Alzheimer’s disease. J Alzheimers Dis 58: , 1003–1016. |
[10] | Kai T , Zhang L , Wang X , Jing A , Zhao B , Yu X , Zheng J , Zhou F ((2015) ) Tabersonine inhibits amyloid fibril formation and cytotoxicity of Aβ(1-42). ACS Chem Neurosci 17: , 879–888. |
[11] | Abuznait AH , Qosa H , Busnena BA , El Sayed KA , Kaddoumi A ((2013) ) Olive-oil-derived oleocanthal enhances β-amyloid clearance as a potential neuroprotective mechanism against Alzheimer’s disease: in vitro and in vivo studies. ACS Chem Neurosci 19: , 973–982. |
[12] | Li W , Sperry JB , Crowe A , Trojanowski JQ , Smith AB 3rd , Lee VM ((2009) ) Inhibition of tau fibrillization by oleocanthal via reaction with the amino groups of tau. J Neurochem 110: , 1339–1351. |
[13] | Frydman-Marom A , Levin A , Farfara D , Benromano T , Scherzer-Attali R , Peled S , Vassar R , Segal D , Gazit E , Frenkel D , Ovadia M ((2011) ) Orally administrated cinnamon extract reduces β-amyloid oligomerization and corrects cognitive impairment in Alzheimer’s disease animal models. PLoS One 6: , e16564. |
[14] | Peterson DW , George RC , Scaramozzino F , LaPointe NE , Anderson RA , Graves DJ , Lew J ((2009) ) Cinnamon extract inhibits tau aggregation associated with Alzheimer’s disease in vitro. J Alzheimers Dis 17: , 585–597. |
[15] | Yang F , Lim GP , Begum AN , Ubeda OJ , Simmons MR , Ambegaokar SS , Chen PP , Kayed R , Glabe CG , Frautschy SA , Cole GM ((2005) ) Curcumin inhibits formation of amyloid beta oligomers and fibrils, binds plaques, and reduces amyloid in vivo. J Biol Chem 280: , 5892–5901. |
[16] | Ma QL , Zuo X , Yang F , Ubeda OJ , Gant DJ , Alaverdyan M , Teng E , Hu S , Chen PP , Maiti P , Teter B , Cole GM , Frautschy SA ((2013) ) Curcumin suppresses soluble tau dimers and corrects molecular chaperone, synaptic, and behavioural deficits in aged human tau transgenic mice. J Biol Chem 288: , 4056–4065. |
[17] | Tang M , Taghibiglou C ((2017) ) The mechanisms of action of curcumin in Alzheimer’s disease. J Alzheimers Dis 58: , 1003–1016. |
[18] | Battisti A , Piccionello AP , Sgarbossa A , Vilasi S , Ricci C , Ghetti F , Spinozzi F , Gammazza AM , Giacalone V , Martorana A , Lauria A , Ferrero C , Bulone D , Mangione MR , Biagio PLS , Ortore MG ((2017) ) Curcumin-like compounds designed to modify amyloid beta peptide aggregation patterns. RSC Adv 7: , 31714. |
[19] | Lakey-Beitia J , González Y , Doens D , Stephens DE , Santamaría R , Murillo E , Gutiérrez M , Fernández PL , Rao KS , Larionov OV , Durant-Archibold AA ((2017) ) Assessment of novel curcumin derivatives as potent inhibitors of inflammation and amyloid-β aggregation in Alzheimer’s disease. J Alzheimers Dis 60: , S59–S68. |
[20] | Yanagisawa D , Taguchi H , Morikawa S , Kato T , Hirao K , Shirai N , Tooyama I ((2015) ) Novel curcumin derivatives as potent inhibitors of amyloid β aggregation. Biochem Biophys Rep 4: , 357–368. |
[21] | Akoury E , Pickhardt M , Gajda M , Biernat J , Mandelkow E , Zweckstetter M ((2013) ) Mechanistic basis of phenothiazine-driven inhibition of tau aggregation. Angew Chem Int Ed Engl 52: , 3511–3515. |
[22] | Necula M , Chirita CN , Kuret J ((2005) ) Cyanine dye N744 inhibits tau fibrillization by blocking filament extension: Implications for the treatment of tauopathic neurodegenerative diseases. Biochemistry 44: , 10227–10237. |
[23] | Jiao SS , Yao XQ , Liu YH , Wang QH , Zeng F , Lu JJ , Liu J , Zhu C , Shen LL , Liu CH , Wang YR , Zeng GH , Parikh A , Chen J , Liang CR , Xiang Y , Bu XL , Deng J , Li J , Xu J , Zeng YQ , Xu X4 , Xu HW , Zhong JH , Zhou HD , Zhou XF , Wang YJ ((2015) ) Edaravone alleviates Alzheimer’s disease-type pathologies and cognitive deficits. Proc Natl Acad Sci U S A 112: , 5225–5230. |
[24] | Yuksel M , Biberoglu K , Onder S , Akbulut KG , Tacal O ((2017) ) Effects of phenothiazine-structured compounds on APP processing in Alzheimer’s disease cellular model. Biochimie 138: , 82–89. |
[25] | Congdon EE , Figueroa YH , Wang L , Toneva G , Chang E , Kuret J , Conrad C , Duff KE ((2009) ) Inhibition of tau polymerization with a cyanine dye in two distinct model systems. J Biol Chem 284: , 20830–20839. |
[26] | Tong H , Lou K , Wang W ((2015) ) Near-infrared fluorescent probes for imaging of amyloid plaques in Alzheimer’s disease. Acta Pharm Sin B 5: , 25–33. |
[27] | Hensley K , Carney JM , Mattson MP , Aksenova M , Harris M , Wu JF , Floyd RA , Butterfield DA ((1994) ) A model for beta-amyloid aggregation and neurotoxicity based on free radical generation by the peptide: Relevance to Alzheimer disease. Proc Natl Acad Sci U S A 91: , 3270–3274. |
[28] | Noble W , Olm V , Takata K , Casey E , Mary O , Meyerson J , Gaynor K , LaFrancois J , Wang L , Kondo T , Davies P , Burns M , Veeranna , Nixon R , Dickson D , Matsuoka Y , Ahlijanian M , Lau LF , Duff K ((2003) ) Cdk5 is a key factor in tau aggregation and tangle formation in vivo. Neuron 38: , 555–565. |
[29] | Pei JJ , Grundke-Iqbal I , Iqbal K , Bogdanovic N , Winblad B , Cowburn RF ((1998) ) Accumulation of cyclin-dependent kinase 5 cdk5 in neurons with early stages of Alzheimer’s disease neurofibrillary degeneration. Brain Res 797: , 267–277. |
[30] | Sun W , Qureshi HY , Cafferty PW , Sobue K , Agarwal-Mawal A , Neufield KD , Paudel HK ((2016) ) Glycogen synthase kinase-3beta is complexed with tau protein in brain microtubules. J Biol Chem 277: , 11933–11940. |
[31] | Mushtaq G , Greig NH2 , Anwar F , Al-Abbasi FA , Zamzami MA , Al-Talhi HA , Kamal MA ((2016) ) Neuroprotective mechanisms mediated by CDK5 inhibition. Curr Pharm Des 22: , 527–534. |
[32] | Nygaard HB ((2018) ) Targeting Fyn kinase in Alzheimer’s disease. Biol Psychiatry 83: , 369–376. |
[33] | Lin CH , Hsieh YS , Wu YR , Hsu CJ , Chen HC , Huang WH , Chang KH , Hsieh-Li HM , Su MT , Sun YC , Lee GC , Lee-Chen GJ ((2016) ) Identifying GSK-3β kinase inhibitors of Alzheimer’s disease: Virtual screening, enzyme, and cell assays. Eur J Pharm Sci 89: , 11–19. |
[34] | Tell V , Hilgeroth A ((2013) ) Recent developments of protein kinase inhibitors as potential AD therapeutics. Front Cell Neurosci 7: , 189. |
[35] | Berk C , Sabbagh MN ((2013) ) Successes and failures for drugs in late-stage development for Alzheimer’s disease. Drugs Aging 30: , 783–792. |
[36] | Yaari R , Hake A ((2015) ) Alzheimer’s disease clinical trials: Past failures and future opportunities. Clin Invest 5: , 297–309. |
[37] | Roy A , Saraf S ((2006) ) Limonoids: Overview of significant bioactive triterpenes distributed in plants kingdom. Biol Pharm Bull 29: , 191–201. |
[38] | Gualtieri MJ , Malafronte N , Vassallo A , Braca A , Cotugno R , Vasaturo M , De Tommasi N , Dal Piaz F ((2014) ) Bioactive limonoids from the leaves of Azaridachta indica (Neem). J Nat Prod 77: , 596–602. |
[39] | Singh BK , Vatsa N , Nelson VK , Kumar V , Kumar SS , Mandal SC , Pal M , Jana NR ((2018) ) Azadiradione restores protein quality control and ameliorates the disease pathogenesis in a mouse model of Huntington’s disease. Mol Neurobiol 55: , 6337–6346. |
[40] | Santa-María I1 , Pérez M , Hernández F , Avila J , Moreno FJ ((2007) ) Characteristics of the binding of thioflavin S to tau paired helical filaments. J Alzheimers Dis 9: , 279–285. |
[41] | Morales I , Cerda-Troncoso C , Andrade V , Maccioni RB ((2017) ) The natural product curcumin as a potential coadjuvant in Alzheimer’s treatment. J Alzheimers Dis 60: , 451–460. |
[42] | Khlistunova I , Biernat J , Wang Y , Pickhardt M , von Bergen M , Gazova Z , Mandelkow E , Mandelkow EM ((2006) ) Inducible expression of tau repeat domain in cell models of tauopathy. J Biol Chem 281: , 1205–1214. |
[43] | Sidhu OP , Kumar V , Behl HM ((2004) ) Variability in triterpenoids (nimbin and salanin) composition of neem among different provenances of India. Ind Crops Prod 1: , 69–75. |
[44] | Hung SY , Fu WM ((2017) ) Drug candidates in clinical trials for Alzheimer’s disease. J Biomed Sci 24: , 47. |
[45] | Cummings J , Lee G , Ritter A , Zhong K ((2018) ) Alzheimer’s disease drug development pipeline: 2018. Alzheimers Dement (N Y) 4: , 195–214. |
[46] | Spires-Jones TL , Friedman T , Pitstick R , Polydoro M , Roe A , Carlson GA , Hyman BT ((2014) ) Methylene blue does not reverse existing neurofibrillary tangle pathology in the rTg4510 mouse model of Tauopathy. Neurosci Lett 6: , 63–68. |
[47] | Bennett DA ((2018) ) Lack of benefit with idalopirdine for Alzheimer disease another therapeutic failure in a complex disease process. JAMA 319: , 123–125. |
[48] | Pickhardt M , Neumann T , Schwizer D , Callaway K , Vendruscolo M , Schenk D , St George-Hyslop P , Mandelkow EM , Dobson CM , McConlogue L , Mandelkow E , Tóth G ((2015) ) Identification of small molecule inhibitors of tau aggregation by targeting monomeric tau as a potential therapeutic approach for tauopathies. Curr Alzheimer Res 12: , 814–828. |
[49] | Okuda M , Fujita Y , Hijikuro I , Wada M , Uemura T , Kobayashi Y , Waku T , Tanaka N , Nishimoto T , Izumi Y , Kume T , Akaike A , Takahashi T , Sugimoto H ((2017) ) PE859, a novel curcumin derivative, inhibits amyloid-β and tau aggregation, and ameliorates cognitive dysfunction in senescence-accelerated mouse prone 8. J Alzheimers Dis 59: , 313–328. |
[50] | Paranjape SR , Riley AP , Somoza AD , Oakley CE , Wang CC , Prisinzano TE , Oakley BR , Gamblin TC ((2015) ) Azaphilones inhibit tau aggregation and dissolve tau aggregates in vitro. ACS Chem Neurosci 6: , 751–760. |
[51] | Maragathavalli S , Brindha S , Kaviyarasi NS , Annadurai B , Gangwar SK ((2012) ) Antimicrobial activity in leaf extract of Neem. Int J Sci Nat 3: , 110–113. |
[52] | Khan M , Wassilew SW , Schmutterer H , Ascher KR ((1987) ) Natural pesticides from the neem tree and other tropical plants. Proceedings of 3rd International Neem Conference, pp. 460–466. |
[53] | Padal SB , Sandhya B , Chandrasekhar P , Vijayakumar Y ((2013) ) Folklore treatment of skin diseases by the tribes of G. Madugula Mandalam, Visakhapatnam District, Andhra Pradesh, India. IOSR J Environ Sci Toxicol Food Technol 4: , 26–29. |
[54] | Lee JW , Ryu HW , Park SY , Park HA , Kwon OK , Yuk HJ , Krishna KS , Park M , Kim JH , Lee S , Oh SR , Ahn KS ((2017) ) Protective effects of neem (Azadirachta indica A. Juss.) leaf extract against cigarette smoke- and lipopolysaccharide-induced pulmonary inflammation. Int J Mol Med 40: , 1932–1940. |
[55] | Dkhil MA , Al-Quraishy S , Aref AM , Othman MS , El-Deib KM , Abdel Moneim AE ((2013) ) The potential role of Azadirachta indica treatment on cisplatin-induced hepatotoxicity and oxidative stress in female rats. Oxid Med Cell Longev 2013: , 741817. |
[56] | Atawodi SE , Atawodi JC ((2009) ) Azadirachta indica (neem): A plant of multiple biological and pharmacological activities. Phytochem Rev 8: , 601–620. |
[57] | Asai H , Ikezu S , Tsunoda S , Medalla M , Luebke J , Haydar T , Wolozin B , Butovsky O , Kügler S , Ikezu T ((2015) ) Depletion of microglia and inhibition of exosome synthesis halt tau propagation. Nat Neurosci 18: , 1584–1593. |
[58] | Haldar S , Phapale PB , Kolet SP , Thulasiram HV ((2013) ) Expedient preparative isolation, quantification and characterization of limonoids from Neem fruits. Anal Methods 5: , 5386–5391. |
[59] | Barghorn S , Biernat J , Mandelkow E ((2005) ) Purification of recombinant tau protein and preparation of Alzheimer-paired helical filaments in vitro. Methods Mol Biol 299: , 35–51. |