Medical Plants and Nutraceuticals for Amyloid-β Fibrillation Inhibition
Abstract
Plaque formation due to amyloid-β oligomerization and fibrillation is a key issue for its deposition in the brains of dementia and Alzheimer’s disease patients. Related drugs preventing this peptide fibril accumulation bear the potential of considerable medical and social value. In this study, we performed in vitro fibrillation inhibition tests with eight different medical plant extracts and nutraceuticals using fluorescence spectroscopy. Successful inhibition of the following plant extracts and nutraceuticals were obtained: Withania somnifera, Centella asiatica, Bacopa monnieri, and Convolvulus pluricaulis, providing new drug candidates for the prevention and treatment of Alzheimer’s disease.
INTRODUCTION
Plaques [1, 2] were defined and specified as one specific reason of Alzheimer’s disease, most notable amyloid-β (Aβ) fibrils arising under brain environmental conditions to form clusters, which block the neurotransmitter transfer between nerve cells. Without critical communication, cell death is initiated. Such clusters located exteriors of the cells are called plaques, while intracellular situated neurofibrillary tangles are identified as tau proteins [2–7].
Therein exist more than 500 explored plants from the Ayurvedic medicine for which access to a considerable collection of herbs traditionally cultivated and applied for daily treatments relevant for different diseases has been provided [8]. There are medical plants which are especially helpful for improving nervous functioning, increasing mental focus, and supporting memorizing abilities. Also, it has been shown that certain medical treatments affect nerve cell regeneration [9, 10]. For this work, we have selected eight of them for the investigation of in vitro inhibition tests to prevent oligomerization/fibrillation of amyloid-β 1–40 (Aβ40) and Methionine amyloid-β 1–40 (MAβ40). Both peptides are of major relevance in Alzheimer’s disease research and considered toxic directly or via transformation to Aβ42 [11] destroying nerve cells: destructing relevant molecule- and affecting ion-transport. This decline in interaction abilities of nerve cells is well-known, leading to loss of memory and physical control of critical body functions. Nevertheless, Aβ is a hallmark, and not necessarily the cause of dementia, the peptide appears due to cleavage of amyloid-β protein precursor (AβPP) by BACE (beta-site APP cleaving enzyme), therefore, further studies with in vitro cell cultures and animal investigations (mice brain and blood analysis) are warranted, and in progress all over the world [12–32].
In this work, different herb extracts and nutraceuticals were investigated for oligomerization/fibrillation in vitro inhibition tests with the aim of preventing formation of plaques; hence, they are possible candidates for Alzheimer’s disease treatment and prevention. Extra- and intracellular degeneration of the peptides is promoted due to proteolysis regardless of the stage of the disease. Patients could extend and increase life quality using available nutraceuticals or self-made extracts especially during the starting phase of the disease.
A long tradition of treatment using Ayurvedic plants provides evidence of reduced or even absence of harmful side-effects, and may thus promote approval of the practical application as a cure.
MATERIALS AND METHODS
Plant material
Withania somnifera (Ashwangandha) and Centella asiatica (Gotu kola) were cultivated from seeds obtained from the Swiss company Botanik Sämereien. Bacopa monnieri (Brahmi) juvenile plants were provided by the gardening center Hellwig (Germany). Ashwangandha dried roots and another charge of Gotu kola seeds were directly imported from India and obtained at the common daily market.
Breeding conditions were kept stable in a growing chamber at room temperature (20 to 23°C) with humidity between 40 to 60%. The LED-based illumination system provided a light distribution from 380 to 780 nm wavelength (white light), and for breeding of juvenile Brahmi plants, additional red light LEDs (550 to 680 nm) and blue light LEDs (380 to 470 nm) were introduced. Applying such LED-system 24 h defined light conditions were achieved: daylight from 8:00 to 20:00 and darkness from 20:00 to 8:00.
Nutraceuticals
Shankha pushpi (Convolvulus pluricaulis) syrup (also containing Brahmi) was obtained from Dabur India Ltd., Sahibabad.
Brahmi (Bacopa monnieri), Ashwangandha (Withania somnifera), and Shuddha guggulu (Commiphora wightii) capsules were purchased from the Himalaya Drug company, Bangalore, India, filled with powder. In the case of Ashwangandha root powder and for all other plants leaf powder was supplied.
Jatamansi (Nardostachys grandiflora DC.) was obtained from Churna, Sadvaidya Ayurveda Pharmacy, Bangalore, India, as capsules filled with leaf powder.
Root powder from Curcuma longa (Haridra powder, Tumeric) was delivered from Bhuvanendra Herbals, Bangalore, India.
Jyotishamti (Celastrus paniculatus) was purchased as syrup from Sadvaidyasala Private Ltd., Nanjangud, India.
Gotu kola (Centella asiatica) was received from SHAG PsoriasisEX, Berlin, Germany, in form of capsules filled with leaf powder.
All nutraceuticals were stored at 4°C. Powders were taken from the inside of the capsules. Materials were handled at room temperature.
Amyloid-β
For in vitro inhibition tests of oligomerization/ fibrillation, we used lyophilized amyloid-β 1–40 (Aβ40) and lyophilized Methionine amyloid-β 1–40 (MAβ40) [33] which were bought from rPeptide LLC (Bogart, GA, USA).
For sample preparation, the first weighing of amyloid-β (Aβ) with a high precision balance was completed, then Hexafluoroisopropanol (HFIP; Sigma Aldrich Company) was added to achieve 5–20 μM solutions for concentration adjustments related to pre-measurements of the peptide quality. Furthermore, such treated Aβ were pipetted into marked tubes, vacuum evaporated for 24 h and finally frozen at –80°C. These samples were stored at the same temperature for a minimum of one day but less than two weeks until usage [34–37].
Thioflavin-T
Thioflavin-T (ThT; Sigma Aldrich Company) was used as the marker for the Aβ oligomerization/fibrillation test [38]. ThT has been found to feature a remarkable property to bind to aggregates, but not to very flexible monomeric peptides, and exerts increased fluorescence intensity in the bound state [39]. ThT was chosen due to the fact that it does not affect the fibrillation kinetics itself. Also, the interaction between ThT and Aβ is stoichiometric and reaches saturation at reasonably low ThT concentration [38–47]: 5 μM ThT was used for all fluorescence fibrillation inhibition measurements.
Fibrillation solvents
As a basic solvent for the fibrillation inhibition tests, HEPES buffer [48] was made from 4-(2-hydroxyethyl)-1-piperazineethanesulfonic acid powder and sodium chloride powder (final concentrations were: 40 mM HEPES and 200 mM NaCl). HEPES buffer was stored at –20°C until use. Ammonium hydroxide solution (0.02% NH4OH) was made from 25% stock standard and used at 4°C. All solutions were prepared with MilliQ water.
Fibrillation inhibition test
The in vitro experiments of Aβ fibrillation inhibition was carried out with two different Perkin Elmer Fluorescence Spectrometers (Luminescence Spectrometer: LS, the older machine was named LS45, the newer LS55). The fluorescence intensities versus time were measured from the Aβ samples containing different drugs or herbal extracts. NH4OH solution was added to the frozen Aβ sample tube and processed with a Vortex machine for 1 min at room temperature. HEPES buffer was added after and mixed with Vortex machine for 10 s. The Aβ sample was filled into 10 mm cuvette under magnetic stirring and ThT was added. Finally, the cuvette was closed with Parafilm and assembled into the LS machine.
Hereby, in order to ensure that the applied Aβ samples are active and fibrillate, the first experiment was carried out as the negative inhibition control: when plateau phase had been reached, the nutraceutical or medical plant extract was added. The positive control was performed with ethanol under the same conditions.
Grinding plant material
Dried Ashwangandha roots were crushed by planetary ball milling (Tanchen Powder, China) using iron vials (internal volume of 50 ml) applying 20 balls of different sizes (3, 5, and 7.5 mm in diameter) to 10 g of plant material with a ball to biomass ratio of roughly 1:1. We used two different preparations: 1) small root parts (1-2 cm length, 2-3 mm diameter) and 2) bigger root parts (3–5 cm length and 4–8 mm diameter). Ball milling time was set to 10 h. The smaller initial plant root material gave a yellow powder, and the bigger root parts finally a grey-black powder.
Extraction methods
For the plant extraction, three different methods were applied. 1) Maceration method, where 3 g plant material and 30 ml ethanol, MilliQ water, and a mixture of both in different concentrations were utilized. At first, fresh harvested green leaves of the test plant material were sliced into small pieces with a sharp kitchen knife on a ceramic plate. After this, 10 ml of the chosen solvent (ethanol, water-ethanol mixture, etc.) were given to the sliced plant material. Then the maceration extracts were made with mortar and pestle. Additional 20 ml of the same solvent were added and gently mixed again with the pestle under soft pressure for 1 min. Finally, the softened mass was leached with filter paper into a sterile container in which the extracts were stored. For LS fibrillation tests, maceration extracts were always made fresh, for better reproducibility. 2) As second method, Soxhlet Extraction (SE), was applied to obtain ingredients of the fresh and green plant material. The stock solvent of 96.7% ethanol and MilliQ water were used to prepare 65% and 50% ethanol solution (EtS). The 65% EtS was added to 15 g fresh plant material shortly before the extraction process which was initialized at a temperature of 95°C for 30 min and continued at a stable temperature of 90°C for 16 h. The SE of 50% EtS with 15 g green and fresh plant material run at a stabilized temperature of 100°C for 14 h. Here, an initializing temperature of 120°C for 30 min was needed. The SEs were cooled down and stored at 4°C and at –80°C in 2 ml tubes till further usage [49–53]. 3) The third extraction method had been similar to “tea brewing”: adding boiled water into a cup with fresh leaves. 150 ml MilliQ water was boiled and cooled down to 80°C and added to the 3 g fresh harvested and cut plant material. The first sample was collected after 5 min of brewing and then further samples were stored every following minute after 25 min for further testing. The biomaterial was freshly collected from plants in the laboratory under sterile conditions. The extracts were stored for short terms in aseptic bottles at 4°C and for long-term at –80°C in 2 ml tubes until use.
All prepared extracts were centrifuged (15.000 rpm at 4°C) to separate the solution from suspended particles and poorly insoluble materials. The upper solvent layer was pipetted into marked tubes and stored in an ice box until experimental usage.
Alcohol evaporation
Aβ samples containing HFIP were kept in a vacuum evaporator for 24 h to dispose of isopropanol. Brahmi extract samples (BrE) consisting of SE solution containing ethanol were placed under vacuum for 24 or 48 h, depending on the sample volume (1 or 2 ml, respectively).
Statistical analysis
Experimental inhibition data were averaged and standard deviations calculated accordingly. Figures represent only a selection of measured inhibition graphs.
RESULTS
An overview of the investigated medical plants and nutraceuticals, usage, the investigation method, and in vitro inhibition test results are provided in (Table 1).
Table 1
A summary of tested nutraceuticals and plants
Latin name | Inhibitor | IE | Drug tests | Plant tests | ThT interaction | Type of Aβ | Application |
Withania somnifera | yes | 49% (±8) | LS | LS | no | Aβ40, MAβ40 | Stress reduction, migraine, headache, neuralgia, swelling tumors, epilepsy, lack of memory [8–10, 58] |
Bacopa monnieri (Linn.) | yes | 74% (±13) | LS | LS | no | MAβ40 | Epilepsy, manic psychosis, depression, promoting memory [8, 9] |
Centella asiatica | yes | 78% (±5) | LS | LS | no | MAβ40 | Chronic rheumatism, increase memory, epilepsy, hysteria [8, 9] |
Nardostachys grandiflora DC. | no | 9% (±11) | LS | na | no | Aβ40, MAβ40 | Hypertension, epilepsy, hysteria, hepatitis, migraine, depression, headache, amnesia [8, 9] |
Celastrus paniculatus | no | –55% (±120) | LS | na | no | Aβ40, MAβ40 | Intellect promoting, sharpening/increase memory, diabetes mellitus [8, 9] |
Convolvulus pluricaulis | nd | 48% (±7) | LS | na | no | Aβ40 | Epilepsy, uterine disorders, anxiety, hypertension, chronic headache [8, 9] |
Commiphora wightii | no | 10% (±9) | LS | na | no | Aβ40, MAβ40 | Anxiety, epilepsy, chronic headache, uterine disorders, hypertension [8, 9] |
Curcuma longa | nd | nd | LS | na | Yes | MAβ40 | Bronchitis, allergies, high blood pressure, high Cholesterol, skin diseases [8, 9, 54] |
na, not available; LS, luminescence spectrometer; nd, not distinguishable; IE, inhibition efficiency.
Nutraceuticals and plant extracts
Withania somnifera (Ashwangandha)
Ashwagandha was tested for fibrillation inhibition in four different ways of sample preparation: 1) as dissolved nutraceutical drug powder (Fig. 1), 2) maceration solvent from fresh leaves, 3) tea-like brewing extract from green and fresh leaves (Fig. 2), and 4) SE solvents from these, all samples successfully inhibited Aβ fibrillation.
Fig.1
Fluorescence graph of Ashwangandha drug powder (ASD) solvent (1 mg in 1 ml) inhibition test: redline shows the MAβ40 fibrillation process with an added inhibitor substance of 20 μl at the start of the fibrillation test, the green curve with added 30 μl and the black line shows the negative reference of pure fibril formation.
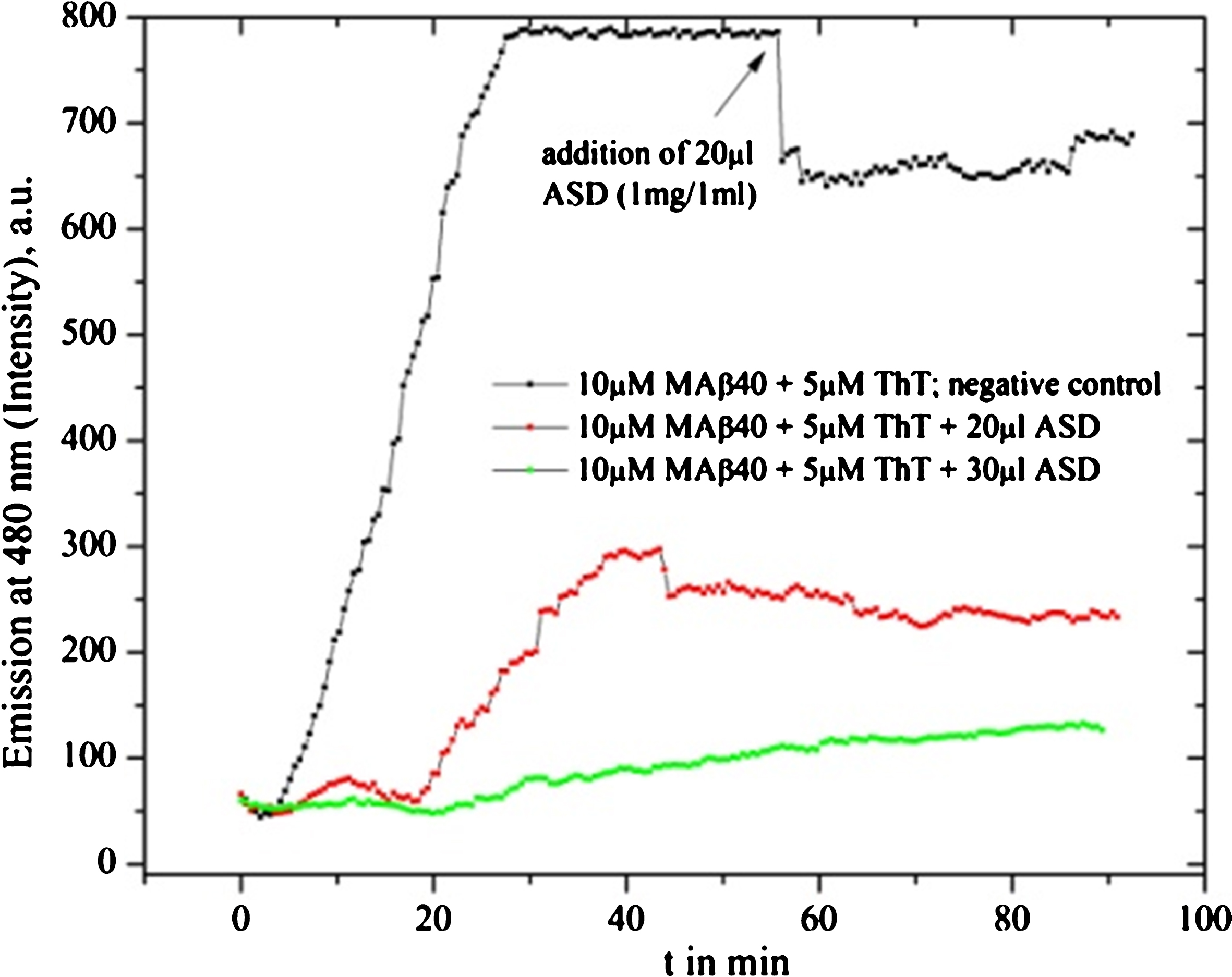
Fig.2
Fluorescence graph of 10 μl Ashwangandha tea extract (ASTE, 3 g in150 ml MilliQ water): black line is the reference where extract had been added after 45 min, the red line is a test of 10 μl ASTE brewed for 3 min added from the beginning, blue line with 10 μl ASTE brewing for 5 min, pink line with 10 μl 22 min, and green line with 10 μl 25 min extract.
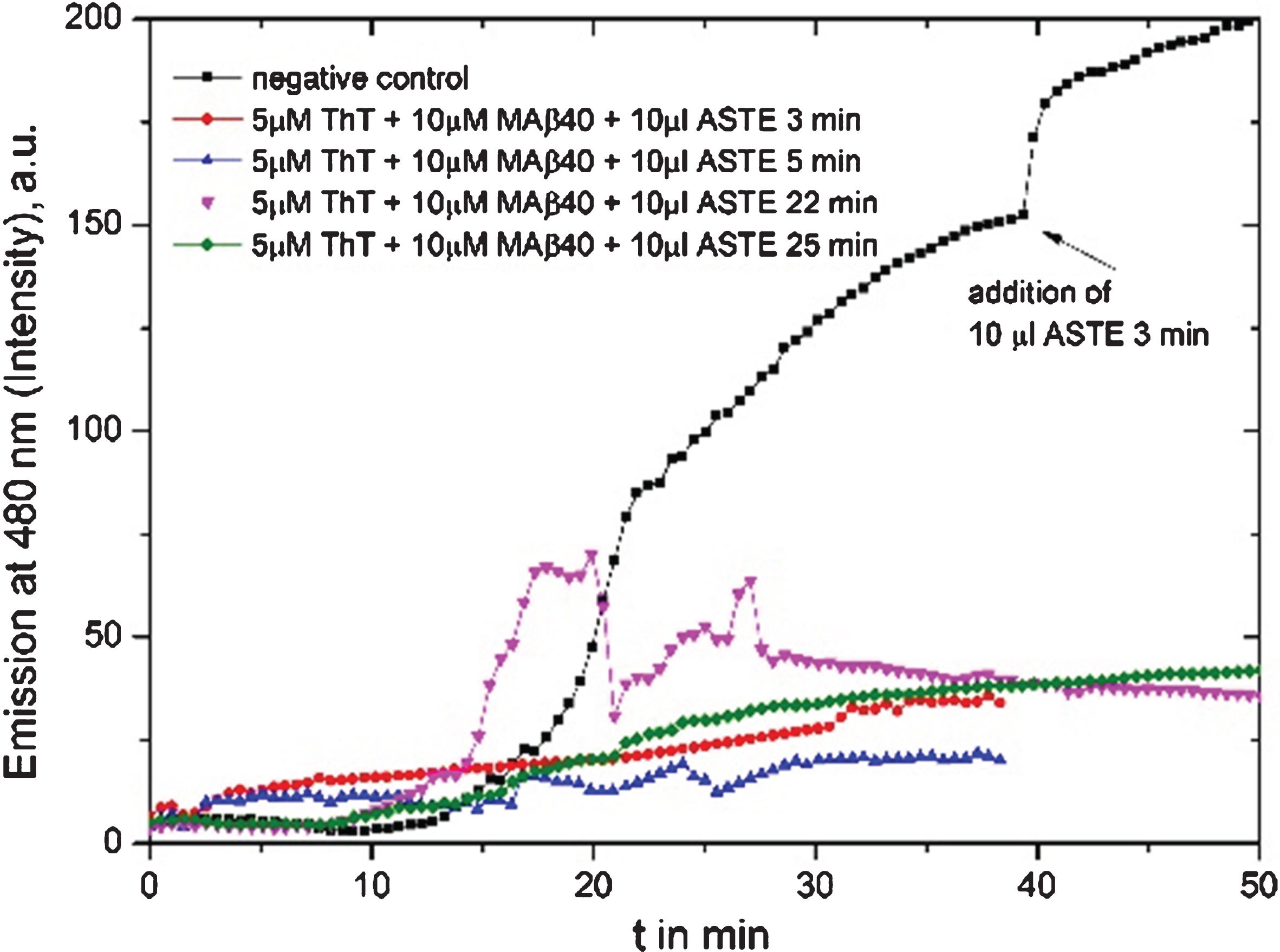
Bacopa monnieri (Brahmi)
Brahmi provided very good MAβ40 fibrillation inhibition for all three sample preparation methods: 2) in MilliQ water dissolved nutraceutical drug powder (Fig. 3), 2) maceration MilliQ water solvent from fresh leaves and stems, and 3) SE solvent of fresh leaves and stems (Figs. 4 and 5).
Fig.3
Brahmi drug powder solvent (1 mg in 1 ml MilliQ water) 10 μl was added after 35 min (black line). The inhibition reaction can be clearly recognized. The blue line shows purification step 1 (one time centrifuged to separate insoluble material from solved compounds) while the green line shows purification step 2 (two times centrifuged to separate insoluble material from solved compounds) and the red line purification step 3 (three times centrifuged to separate insoluble material from solved compounds). All three provide evidence of inhibition.
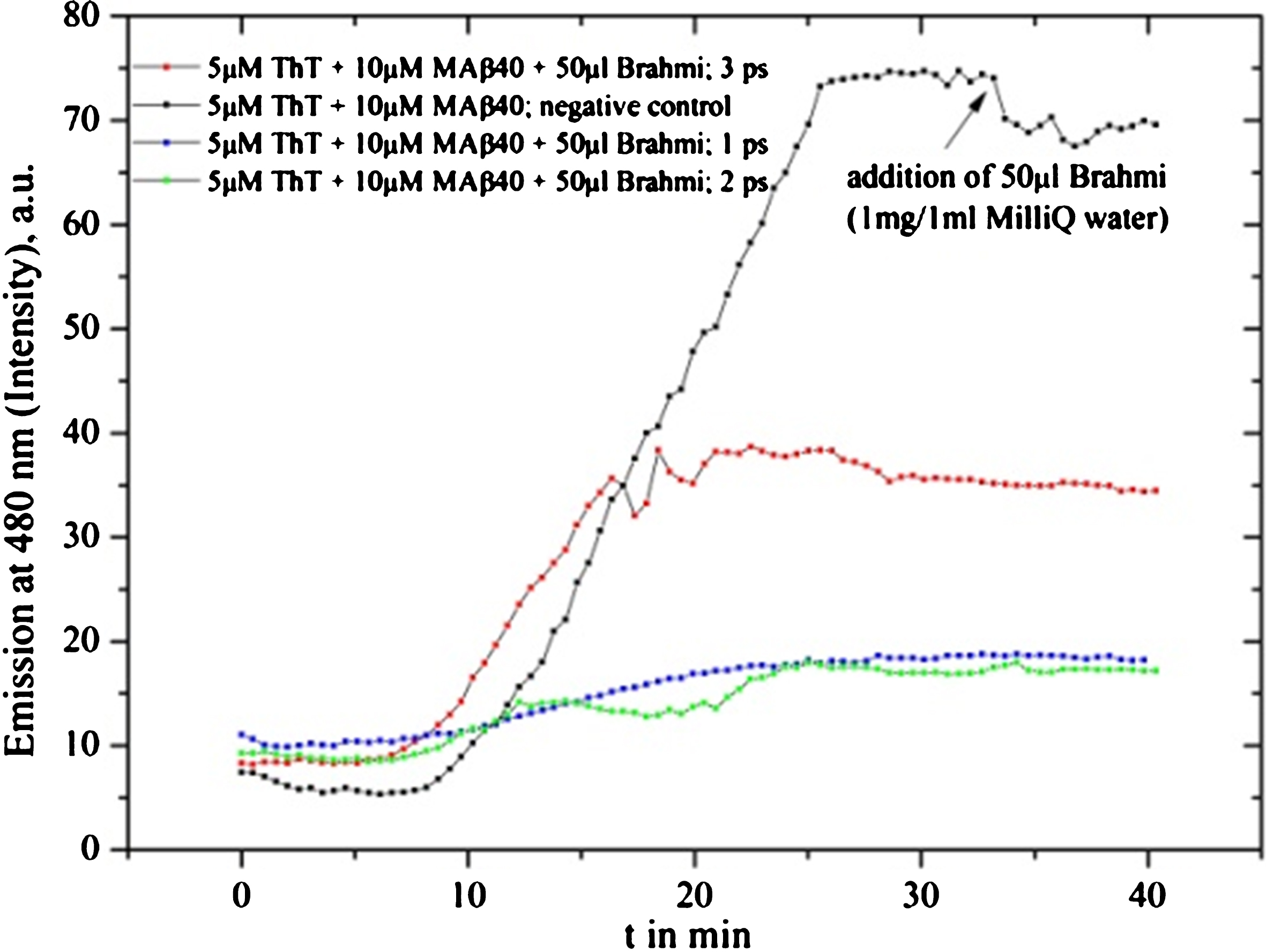
Fig.4
Brahmi SE tests with different amounts (25 g leaf material in 1000 ml 50% ethanol solution) from 10 to 40 μl are shown. The inhibition increases from the 10 to 30 μl sample while the 40 μl solution can be identified to be less efficient.
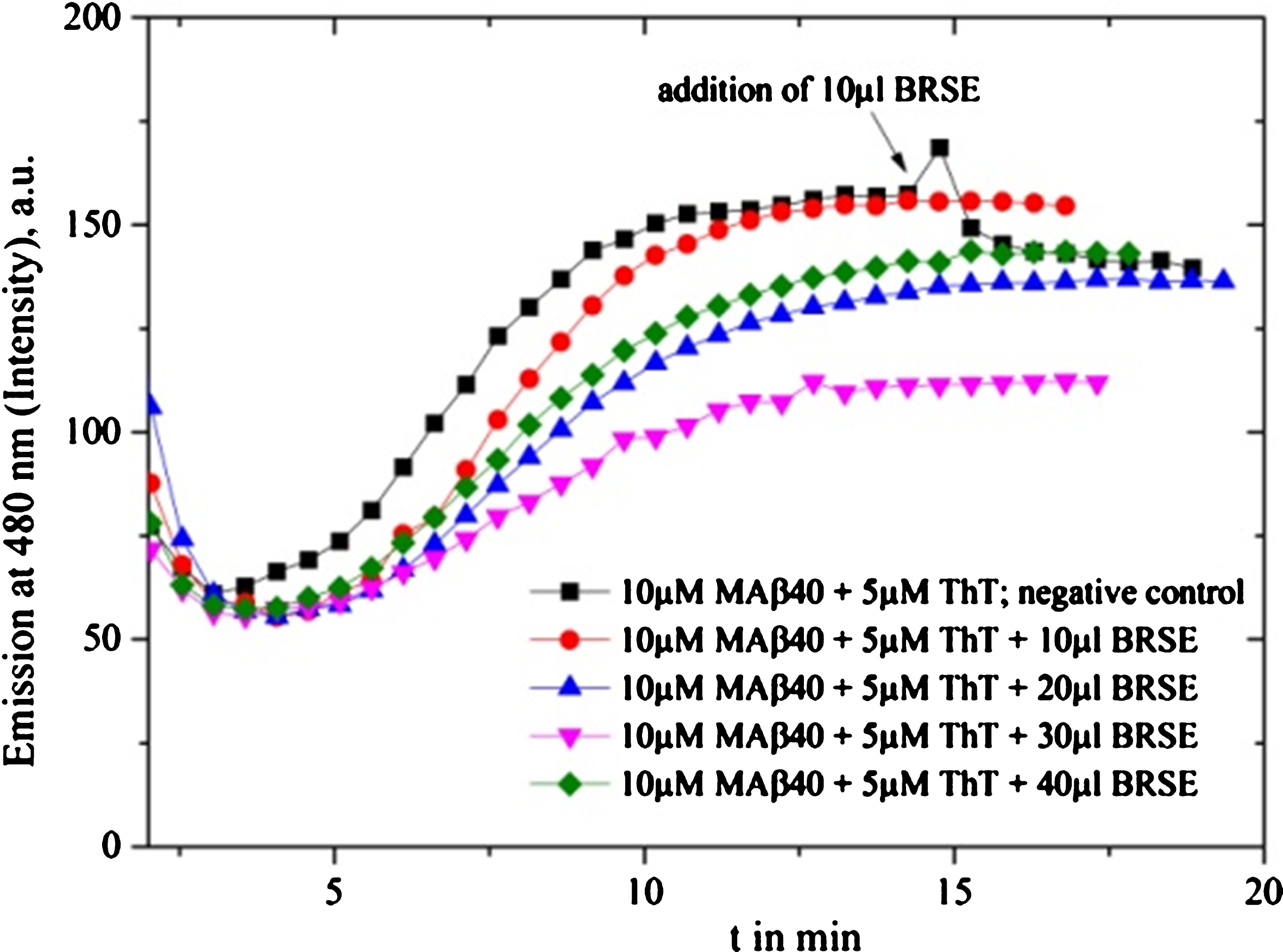
Fig.5
Brahmi SE (BRSE) tests for different volumes between 29 and 31 μl are provided: maximum inhibition efficiency appears at 30 μl BRSE.
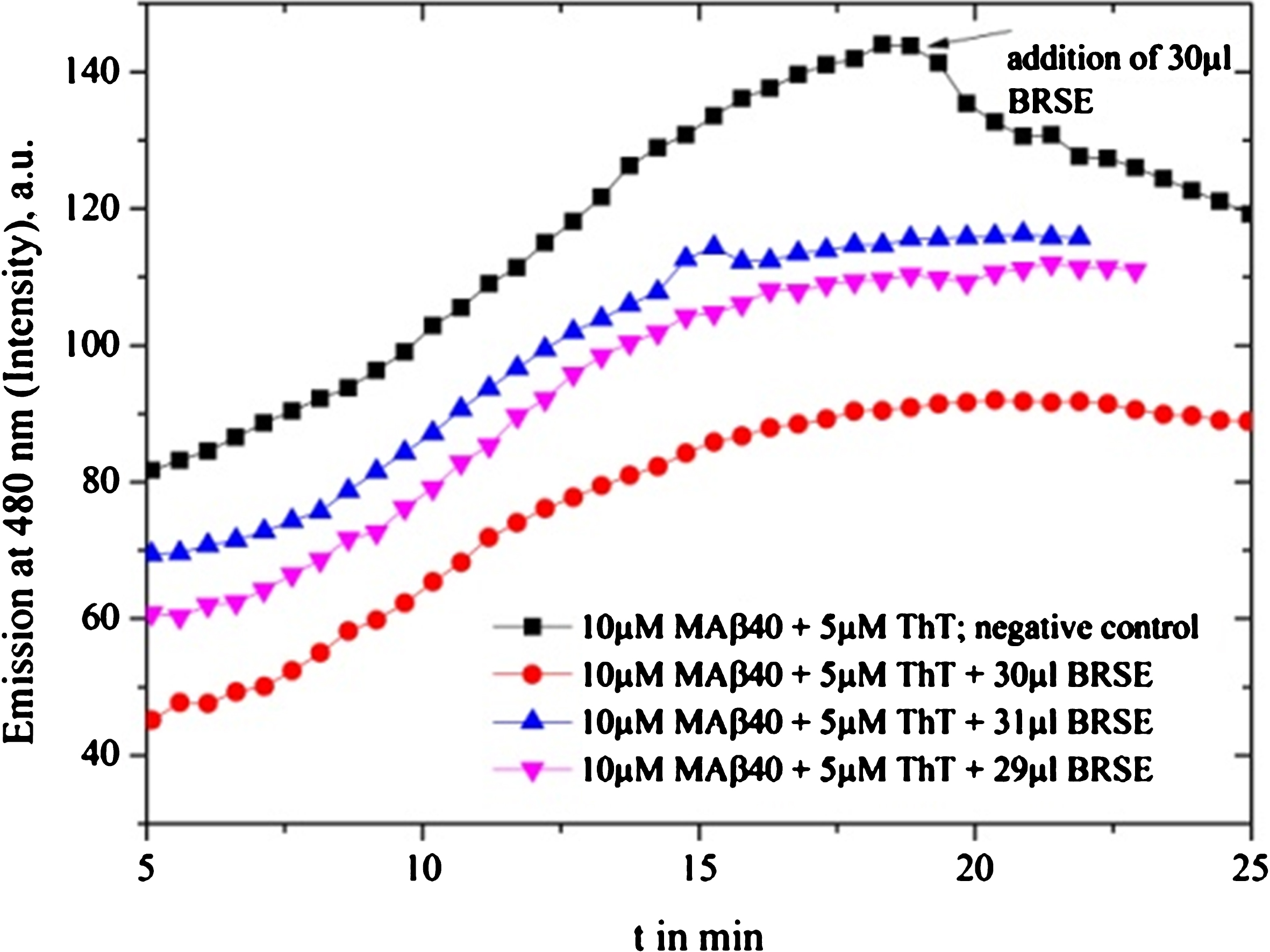
Fig.6
Inhibition tests on Shankha pushpi (SP) syrup (included Brahmi), diluted by 1:1000 with MilliQ water are depicted: the black graph is the reference graph where after 90 min 10 μl syrup was added, providing maximum intensity. The 10 μl sample clearly inhibits the fibrillation process, while the 5 μl sample delays the oligomerization process and provides decreased inhibition intensity.
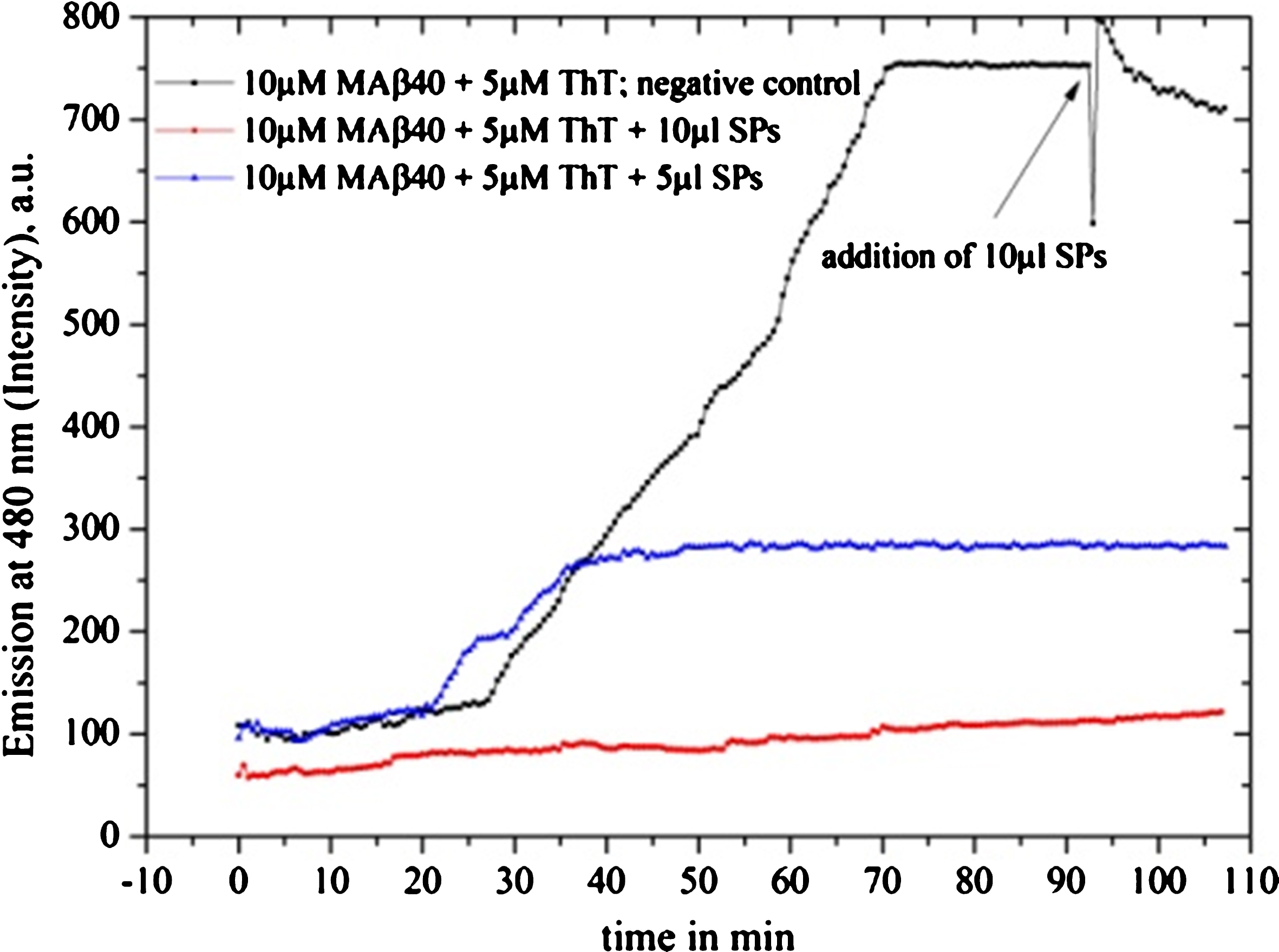
Centella asiatica (Gotu kola)
The medical plant extract and nutraceutical form Gotu kola performed as an active substance. The SE and drug powder inhibit at a similar concentration (1 mg/1 ml MilliQ water). In order to remove remaining ethanol from the SE, vacuum evaporation was applied (Figs. 8 and 9). The fibrillation measurements were probably influenced by self-fluorescence; see Fig. 10, providing increased fluorescence intensities at higher plant extract concentrations.
Fig.7
Shuddha guggulu (SG) drug (1 mg in 1 ml MilliQ water) shows alternating inhibition behavior under assumed similar experimental conditions and with the same amount of dissolved drug material: red and green line. The negative control, black line, shows a down spike in the moment of adding the drug (well-known fluorescence fluctuation due to initiation and equilibration). The intensity gets a rapid increase but turns to a down slope.
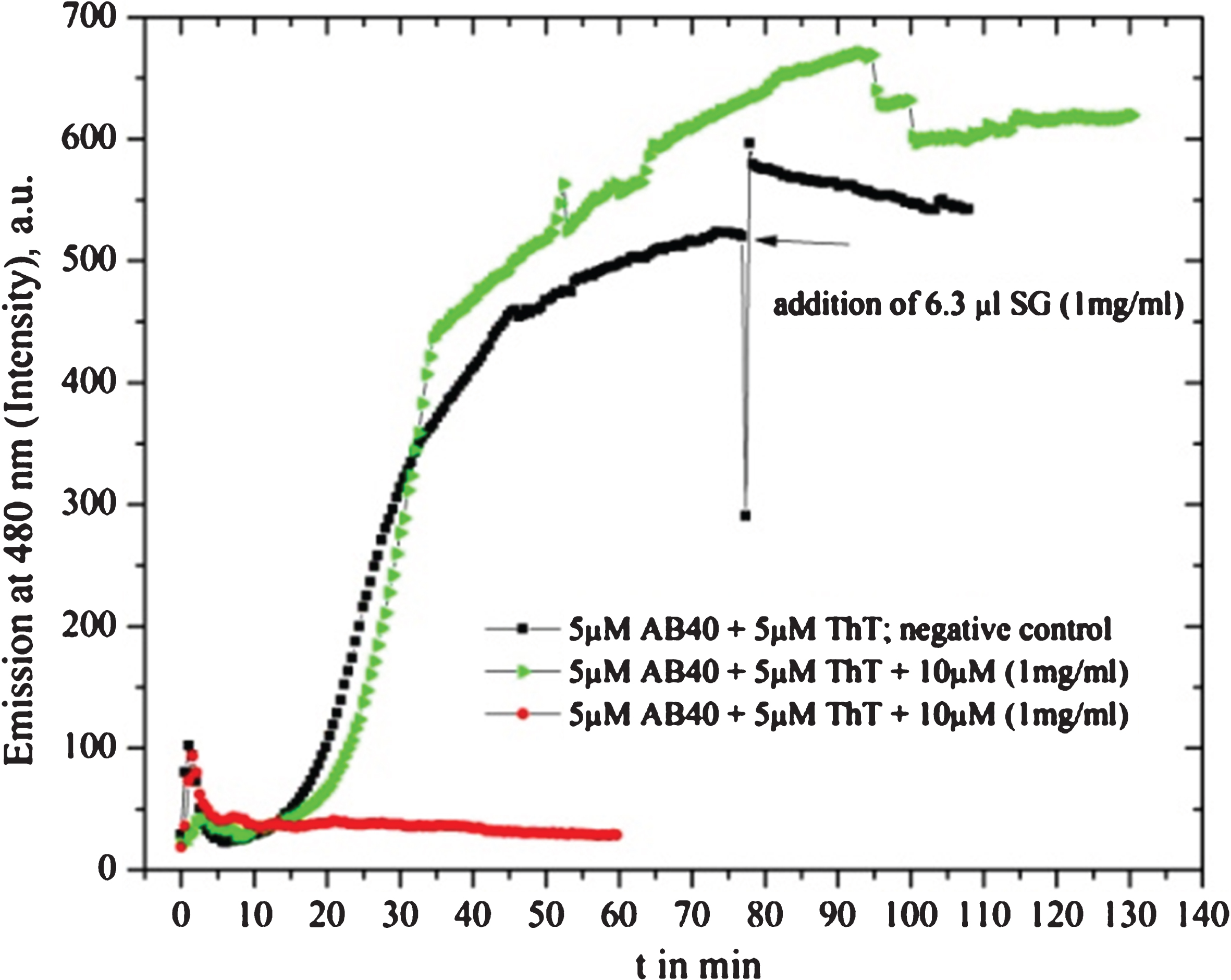
Fig.8
Gotu kola SE (GKSEK, 10 mg dry weight dissolved in 1 ml of MilliQ water) was used for MAβ40 fibril formation inhibition. A mostly complete suppression was observed: blue line 15 μl (10 mg in 1 ml solution), also strong inhibition with 10 μl GKSEK (green line) and delayed weak fibrillation with 7.5 μl (red line).
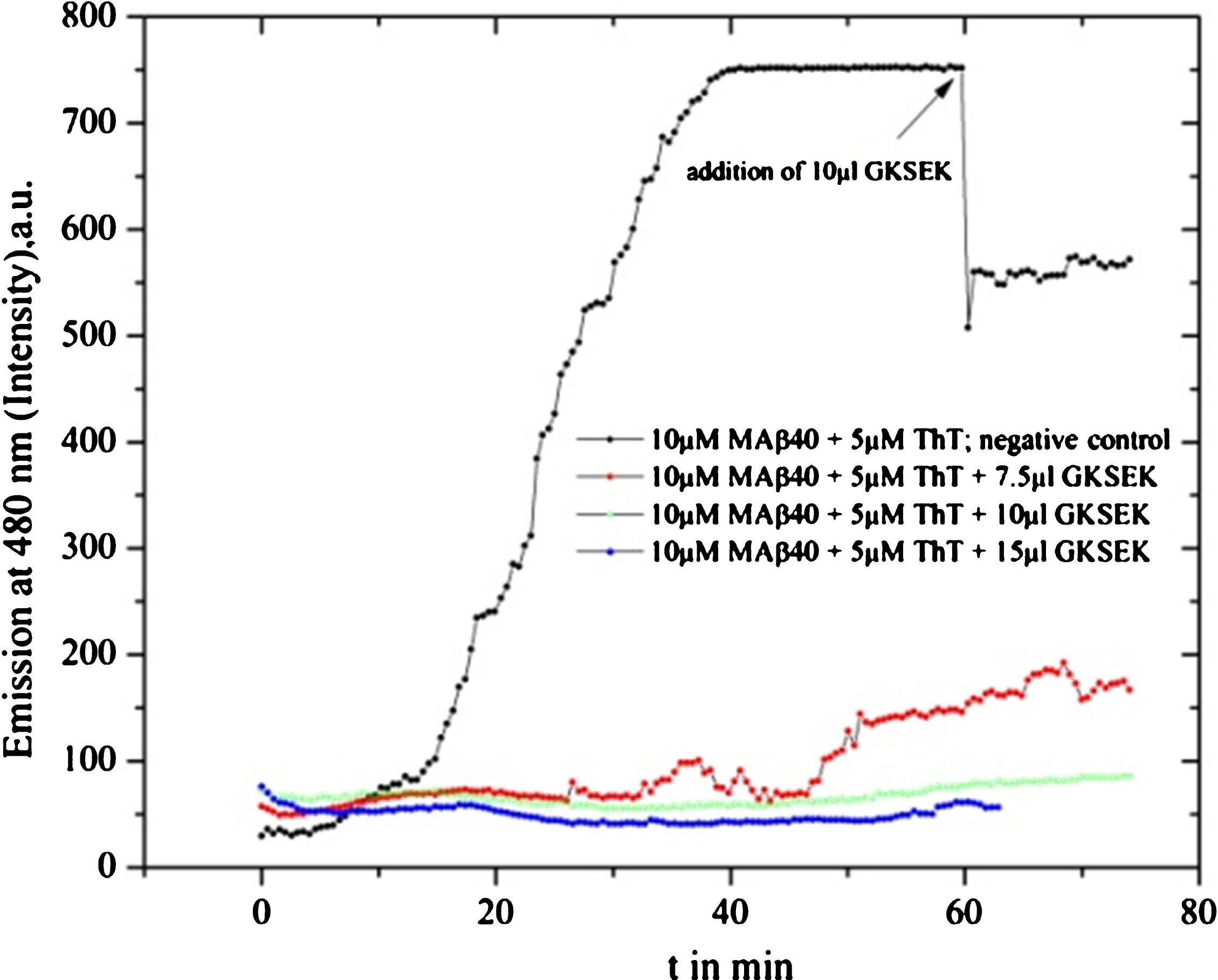
Fig.9
Gotu kola drug powder (GKDP; 1 mg in 1 ml MilliQ water) used for MAβ40 fibril formation inhibition tests is shown. Different drug solvent amounts were used: blue line 20 μl (in 1 ml solution), green line 15 μl, red line 10 μl, and black line as reference graph where 10 μl was added after 60 min. GKDP shows some self-fluorescence which leads to a slightly alternating starting behavior.
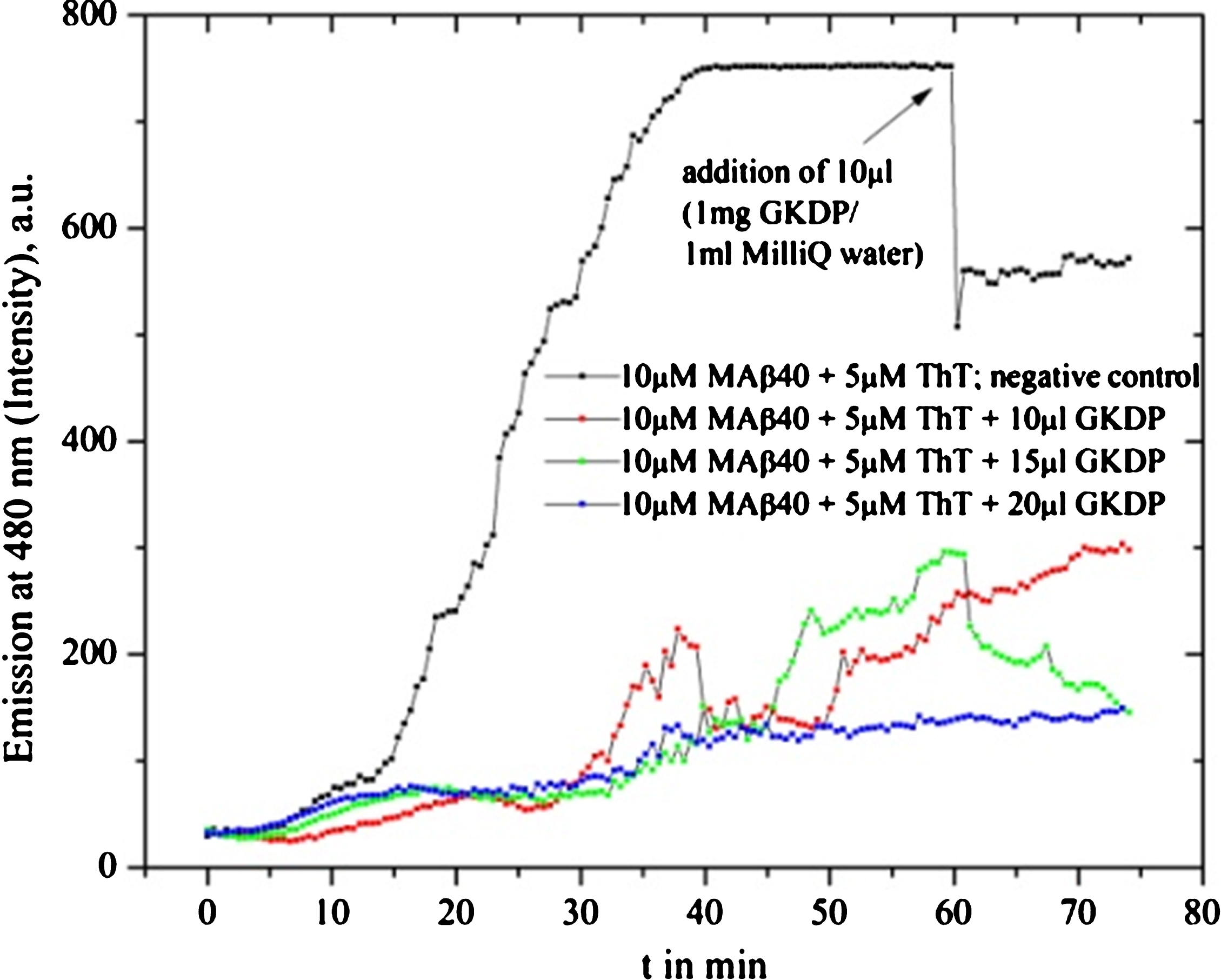
Fig.10
Fluorescence spectra of Gotu kola SE (GKSEK) are presented for different amounts oftest solvents (133 μl of 40 mM HEPES, 200 mM NaCl and 133 μl 0.02% NH4OH) and ThT as a control. Self-fluorescence becomes evident and gets very high in combination with ThT. This explains the different starting intensities of the measurements, so we could fit the start time and estimates the inhibition value (in percent).
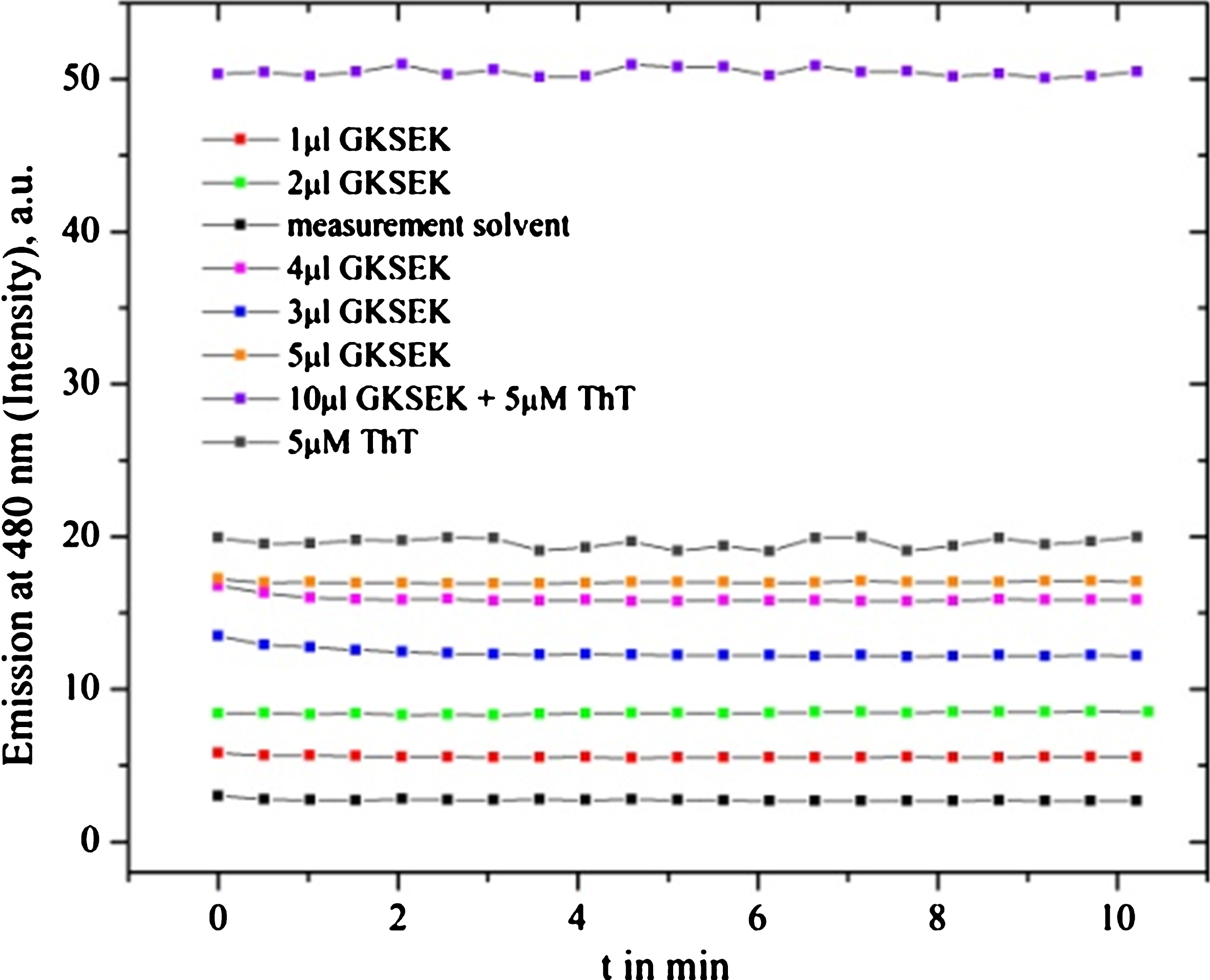
Nardostachys grandiflora (Jatamansi)
Jatamansi nutraceutical did not inhibit Aβ40 fibrillation but even increased the process compared to the reference experiment. One example is shown in Fig. 11.
Fig.11
Jatamansi fibrillation tests with MAβ40 show no inhibition, instead, the fibrillation seems to be increased.
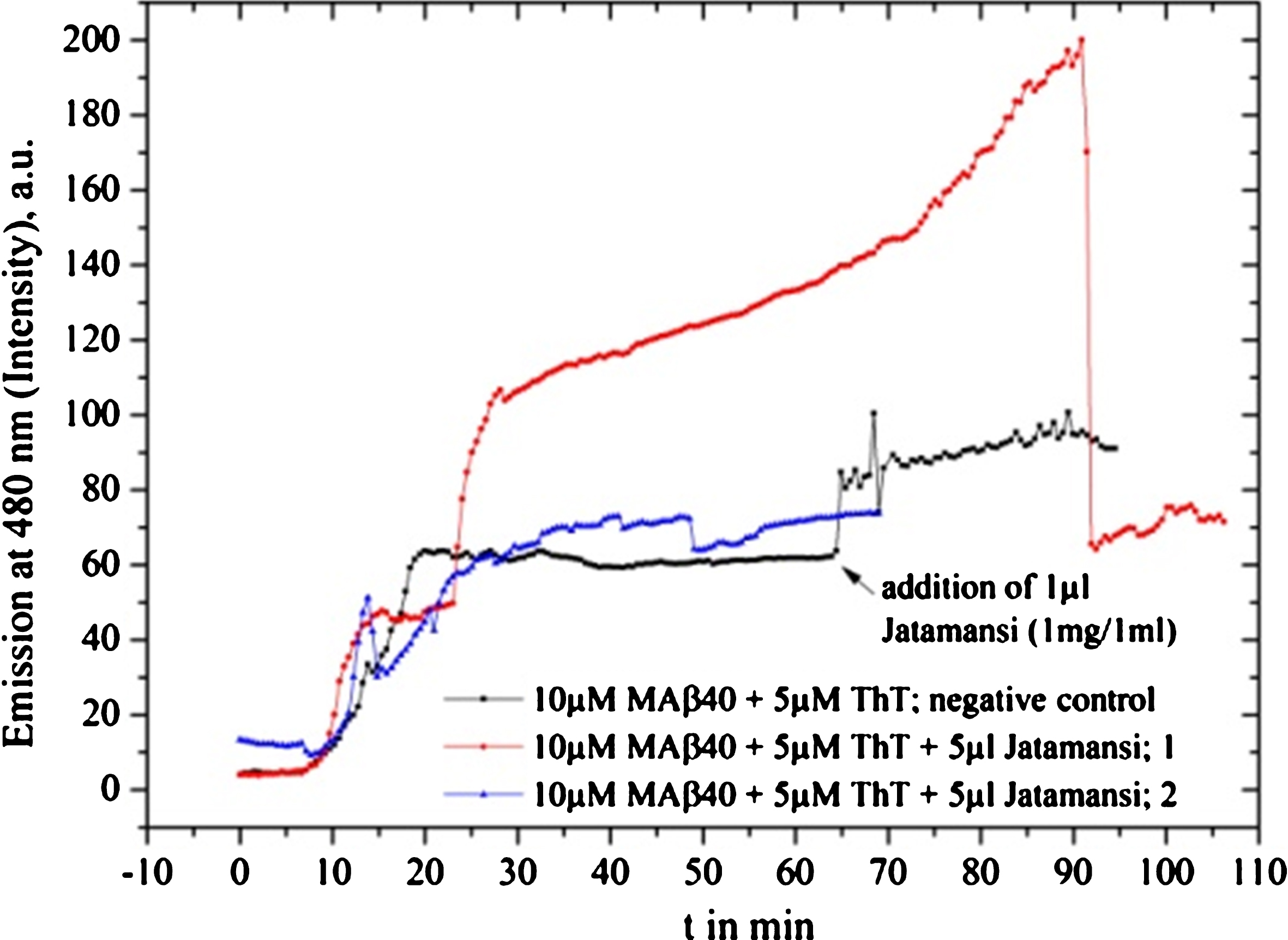
Celastrus paniculatus (Jyotishmati)
Jyotishmati did not perform as a fibrillation inhibitor; it has a neutral effect as, for instance, Shuddha guggulu (Fig. 12).
Fig.12
Jyotishmati provides a slight fibrillation inhibition.
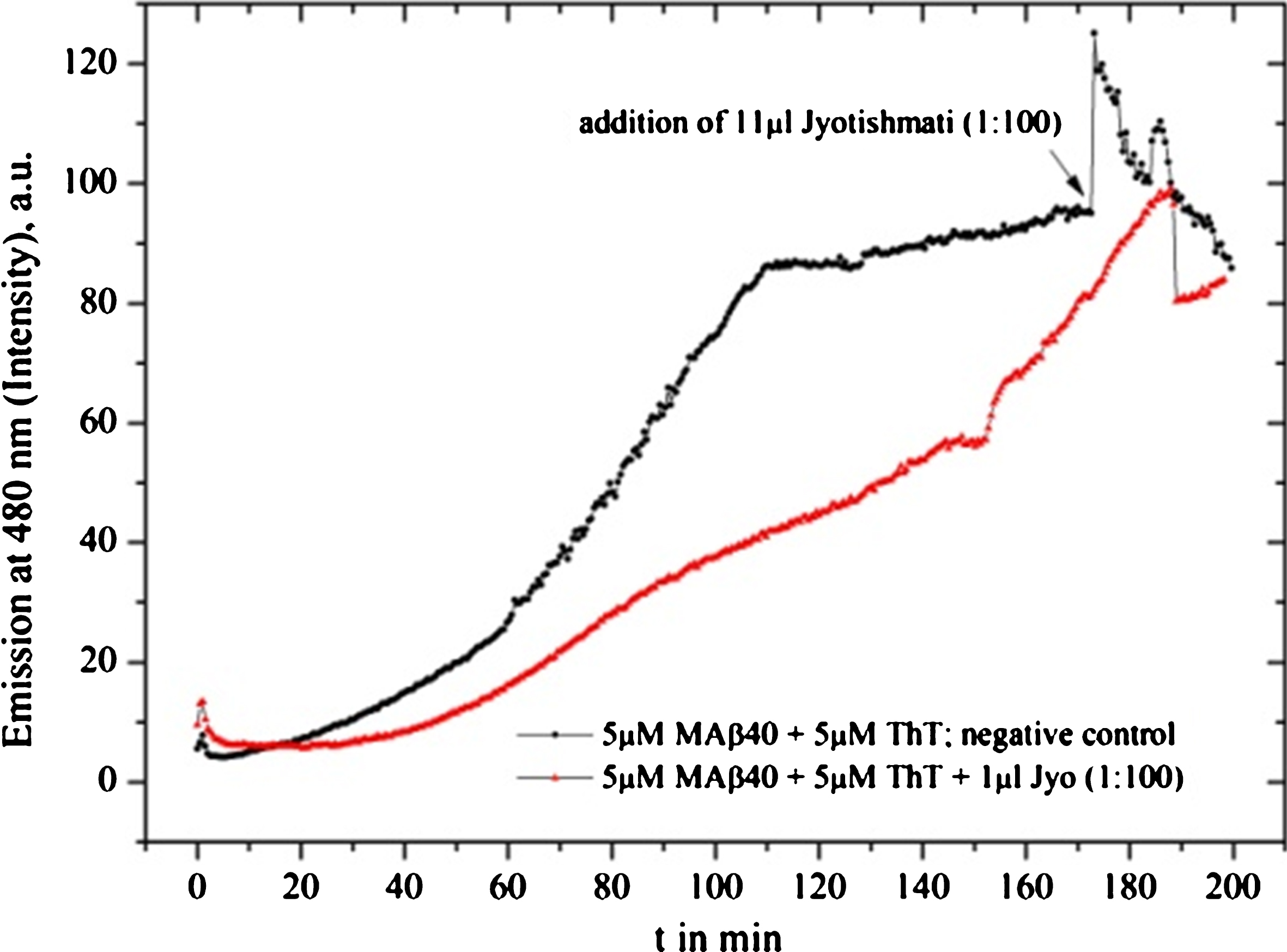
Convolvulus pluricaulis (Shankha pushpi)
The syrup of Shankha pushpi (included Brahmi) was tested directly from the product vial for in vitro fibrillation. Shankha pushpi proved to be the strongest inhibitor for the Aβ40 and MAβ40 fibrillation process, compared to all other tested extracts, drugs, and syrups in this work: a small syrup amount (1 μl in roughly 1 ml total solution) was sufficient for a positive inhibition result. 1 μl Shankha pushpi syrup was dissolved in 1 ml MilliQ water (1:1000) for LS fibrillation in vitro tests (Fig. 6).
Commiphora wightii (Shuddha guggulu)
The experiments with Shuddha guggulu resin nutraceutical were not reproducible. For the first test with 10 μl drug solvent (1 mg in 1 ml MilliQ water), we found 100% fibrillation inhibition. Repeating the test provided a negative result (Fig. 7). However, we observed that there is no interaction with ThT.
DISCUSSION
The inhibition efficiency of nutraceuticals and extracts turned out to show similar trends for both applied Aβ, shown in (Table 1). Depending on the quality of Aβ peptides [55–57], concentrations, micro/nano-culture sample-environmental conditions, age of the test materials, and possible self-fluorescence (Fig. 10), inhibition deviations (SD) are observed.
We applied Withania somniferra as a reference plant to ensure our experimental conditions, and confirm that fresh material, as well as root based nutraceuticals, are inhibitors of the MAβ40 and Aβ40 fibrillation process [58]. The usage of different extraction methods for the fresh plant material has shown no significant difference compared to the root powder nutraceutical. Since mostly the use of roots had been described in the literature, this result had been not straightforwardly expected. Leaves are non-toxic, grow faster, are easy to be harvested, while keeping the plant alive considerably increases agricultural productivity, and hence this information may contribute to treatment improvements. Also, Withania somniferra shows some different inhibition activity depending on the kind of Aβ: 74% for Aβ40 with SD of±7 and 49% for MAβ40 with SD of±8. The altered inhibition level is probably related to varying amounts of nutraceutical and extract’s solvent, due to the fact that the dissolved bioactive components might have different concentrations depending on solubility in MilliQ water and ethanol. Nevertheless, even the lowest amount of the tested material provided a minimal inhibition of 22%.
From the literature, it is known that more than 40 Withanolides are identified as ingredients of Ashwangandha [59–65] from which Withaferin A seems to be the most important and possibly one of the bioactive inhibiting compounds.
For the first time, we could show that Bacopa monnieri acts as a promising inhibitor of the peptide fibrillation. It provides inhibition under all considered experimental conditions. Even with lower concentrations, it reaches a minimum of 31%. Previous reports provide the information that Bacosides A and B may be the main bioactive compounds of the test material [66–68]: further investigations are advised.
Centella asiatica nutraceuticals and leaf extracts provided evidence acting as one of the strongest inhibitors of our study and showed considerable interaction with the MAβ40 peptides. We could not observe significant differences between extracts and the nutraceuticals. Minimum inhibition was found to be 30% which is related to a low Gotu kola concentration. Higher values inhibit at least at a level of 72%. Nevertheless, the main bioactive compounds seem to be Asiaticosides [69].
All three plants, Ashwangandha, Gotu kola, and Brahmi, were tested for different extraction methods to examine their applicability for practical usage: a simple “tea brewing” should be preferred compared to more complicated extractions, if there is no significant difference in efficiency. Hereby, the optimal brewing time for Ashwangandha turned out to be around 5 min, 3 min for Gotu kola, and 10 min for Brahmi.
An evidence of inhibition was also discovered for the fresh Shankha pushpi syrup. We observed degradation of inhibition depending on age and storage conditions. After three months of delaying and transportation under undefined circumstances for the second experimental test, the inhibition efficiency was reduced or even did not show any effect on the fibrillation process (Fig. 6).
In contrast to the above-mentioned active samples, we identified several neutral or non- inhibiting species: Shuddha guggulu resin (Commiphora wightii, Fig. 7) and Jyotishmati (Fig. 12). Both might be investigated for the usage of other mental disorders’ in the future, see (Table 1). Nevertheless, Shuddha guggulu seems to interact with Aβ and ThT under specific circumstances.
Notably, we observed, that Jatamansi provided not only a non-inhibiting behavior but instead, seemingly even to increase the fibrillation, compared to the reference measurement (Fig. 11). Obviously, in light of the present study, any use of Jatamansi should not be encouraged without additional research.
Turmeric (Curcuma longa) showed evidence of interaction with the fibrillation marker ThT. With the applied methods, it was not possible to discover whether it acts with MAβ40, Aβ40, or ThT during the in vitro process. The measurements show positive inhibition interaction, but it may be false positive. Previous work on Curcuma provided information that it acts as an inhibitor against Aβ formation, while a different marker had been used under alternative test circumstances [39]. Future investigations might provide more insight into how ThT interacts with Curcuma nutraceutical [40, 54].
Considering natural plant extracts, often a mixture of components provide the best Alzheimer’s disease treatment effect. In order to obtain further insight here, analysis of the extracts’ ingredients and identification of primary/secondary plant bioactive compounds are requested. Finally, studies on rats, mice, and humans are advised.
CONFLICTS OF INTEREST
The authors declare no conflict of interest.
ACKNOWLEDGMENTS
We gratefully acknowledged the financial support from the Estonian Research Council for projects PUT126, PUT1534, and IUT19-27 and the European social Fund for MOTT68. The authors also thank Tallinn University of Technology and Institute of Nanotechnology of Karlsruhe Institute of Technology. Special thanks to Karin Valmsen, Peep Palumaa, Andra Noormägi, and Merlin Friedemann for experimental advice and discussions, Palanivel Moloiyan for the test material from India, and Cecilia Maria Sarmiento for co-reading and advice. This work has been conducted in motivating memory of Heinz Edmund Witter and Ingeburg Thomas.
This work has been partially supported by “TUT Institutional Development Program for 2016-2022” Graduate School in Biomedicine and Biotechnology receiving funding from the European Regional Development Fund under program ASTRA 2014-2020.4.01.16-0032 in Estonia.
REFERENCES
[1] | Meynen G , van Stralen H , Smit JH , Kamphorst W , Swaab DF , Hoogendijk WJG ((2010) ) Relation between neuritic plaques and depressive state in Alzheimer’s disease. Acta Neuropsychiatr 22: , 14–20. |
[2] | Murphy GM , Tamminga CA ((1995) ) Amyloid plaques. Am J Psychiatry 152: , 1258–1258. |
[3] | Tomlinson BE ((1982) ) Plaques, tangles and Alzheimer’s disease. Psychol Med 12: , 449–459. |
[4] | Cork LC , Walker LC , Price DL ((1989) ) Neurofibrillary tangles and senile plaques in a cognitively impaired, aged nonhuman primate. J Neuropathol Exp Neurol 48: , 378–378. |
[5] | Teixeira F ((1994) ) Amyloid, neurofibrillary tangles and argyrophilic plaques - neuropathology of Alzheimer’s disease. Rev Mex Psicol 11: , 15–17. |
[6] | Younkin SG ((1998) ) Hot papers - Alzheimer’s disease - Secreted amyloid beta-protein similar to that in the senile plaques of Alzheimer’s disease is increased in vivo by the presenilin 1 and 2 and APP mutations linked to familial Alzheimer’s disease by D. Scheuner, C. Eckman, M. Jensen, X. Song, M. Citron, N. Suzuki, T.D. Bird, J. Hardy, M. Hutton, W. Kukull, E. Larson, E. Levy-Lahad, M. Viitanen, E. Peskind, P. Poorkaj, G. Schellenberg, R. Tanzi, W. Wasco, L. Lannfelt, D. Selkoe, S. Younkin - Comments. Scientist 12: , 11. |
[7] | Tateno A , Sakayori T , Senzaki A , Okubo Y ((2014) ) Amyloid plaque among patients with mild cognitive impairment following traumatic brain injury detected by [18F] florbetapir. Brain Inj 28: , 742–743. |
[8] | Bhūtyā RaKr ((2011) ) Ayurvedic medicinal plants of India, Scientific Publishers (India), Jodhpur. |
[9] | Rao RV , Descamps O , John V , Bredesen DE ((2012) ) Ayurvedic medicinal plants for Alzheimer’s disease: A review. Alzheimers Res Ther 4: , 22. |
[10] | Kuboyama T , Tohda C , Komatsu K ((2005) ) Neuritic regeneration and synaptic reconstruction induced by withanolide A. Br J Pharmacol 144: , 961–971. |
[11] | Yoo BK , Xiao Y , McElheny D , Ishii Y ((2018) ) E22G pathogenic mutation of beta-amyloid (Abeta) enhances misfolding of Abeta40 by unexpected prion-like cross talk between Abeta42 and Abeta40. J Am Chem Soc 140: , 2781–2784. |
[12] | Berti V , Walters M , Sterling J , Quinn CG , Logue M , Andrews R , Matthews DC , Osorio RS , Pupi A , Vallabhajosula S , Isaacson RS , de Leon MJ , Mosconi L ((2018) ) Mediterranean diet and 3-year Alzheimer brain biomarker changes in middle-aged adults. Neurology 90: , e1789–e1798. |
[13] | Willemse EAJ , De Vos A , Herries EM , Andreasson U , Engelborghs S , van der Flier WM , Scheltens P , Crimmins D , Ladenson JH , Vanmechelen E , Zetterberg H , Fagan AM , Blennow K , Bjerke M , Teunissen CE ((2018) ) Neurogranin as cerebrospinal fluid biomarker for Alzheimer disease: An assay comparison study. Clin Chem 64: , 927–937. |
[14] | Chiong W ((2018) ) Challenges in communicating and understanding predictive biomarker imaging for Alzheimer disease. JAMA Neurol 75: , 18–19. |
[15] | Woodward MR , Amrutkar CV , Shah HC , Benedict RH , Rajakrishnan S , Doody RS , Yan L , Szigeti K ((2017) ) Validation of olfactory deficit as a biomarker of Alzheimer disease. Neurol Clin Pract 7: , 5–14. |
[16] | Babulal GM , Ghoshal N , Head D , Vernon EK , Holtzman DM , Benzinger TLS , Fagan AM , Morris JC , Roe CM ((2016) ) Mood changes in cognitively normal older adults are linked to Alzheimer disease biomarker levels. Am J Geriatr Psychiatry 24: , 1095–1104. |
[17] | Beeri MS , Sonnen J ((2016) ) Brain BDNF expression as a biomarker for cognitive reserve against Alzheimer disease progression. Neurology 86: , 702–703. |
[18] | Evered L , Silbert B , Scott DA , Ames D , Maruff P , Blennow K ((2016) ) Cerebrospinal fluid biomarker for Alzheimer disease predicts postoperative cognitive dysfunction. Anesthesiology 124: , 353–361. |
[19] | Kester MI , Teunissen CE , Crimmins DL , Herries EM , Ladenson JH , Scheltens P , van der Flier WM , Morris JC , Holtzman DM , Fagan AM ((2015) ) Neurogranin as a cerebrospinal fluid biomarker for synaptic loss in symptomatic Alzheimer disease. JAMA Neurol 72: , 1275–1280. |
[20] | Quiroz YT , Schultz AP , Chen K , Protas HD , Brickhouse M , Fleisher AS , Langbaum JB , Thiyyagura P , Fagan AM , Shah AR , Muniz M , Arboleda-Velasquez JF , Munoz C , Garcia G , Acosta-Baena N , Giraldo M , Tirado V , Ramirez DL , Tariot PN , Dickerson BC , Sperling RA , Lopera F , Reiman EM ((2015) ) Brain imaging and blood biomarker abnormalities in children with autosomal dominant Alzheimer disease: A cross-sectional study. JAMA Neurol 72: , 912–919. |
[21] | Ma L , Chen J , Wang R , Han Y , Zhang J , Dong W , Zhang X , Wu Y , Zhao Z ((2015) ) The level of Alzheimer-associated neuronal thread protein in urine may be an important biomarker of mild cognitive impairment. J Clin Neurosci 22: , 649–652. |
[22] | Rembach A ((2014) ) Alzheimer disease: The search for a blood-based biomarker for Alzheimer disease. Nat Rev Neurol 10: , 618–619. |
[23] | Mansoor Y , Jastrzab L , Dutt S , Miller BL , Seeley WW , Kramer JH ((2015) ) Memory profiles in pathology or biomarker confirmed Alzheimer disease and frontotemporal dementia. Alzheimer Dis Assoc Disord 29: , 135–140. |
[24] | Fortea J , Vilaplana E , Alcolea D , Carmona-Iragui M , Sanchez-Saudinos MB , Sala I , Anton-Aguirre S , Gonzalez S , Medrano S , Pegueroles J , Morenas E , Clarimon J , Blesa R , Lleo A , Alzheimer’s Disease Neuroimaging Initiative ((2014) ) Cerebrospinal fluid beta-amyloid and phospho-tau biomarker interactions affecting brain structure in preclinical Alzheimer disease. Ann Neurol 76: , 223–230. |
[25] | Trueba-Saiz A , Cavada C , Fernandez AM , Leon T , Gonzalez DA , Fortea Ormaechea J , Lleo A , Del Ser T , Nunez A , Torres-Aleman I ((2013) ) Loss of serum IGF-I input to the brain as an early biomarker of disease onset in Alzheimer mice. Transl Psychiatry 3: , e330. |
[26] | Kingwell K ((2013) ) Alzheimer disease: CSF levels of mitochondrial DNA-a new biomarker for preclinical Alzheimer disease? Nat Rev Neurol 9: , 420. |
[27] | Mo J , Maudsley S , Martin B , Siddiqui S , Cheung H , Johnson CA ((2013) ) Classification of Alzheimer diagnosis from ADNI plasma biomarker data. ACM Conf Bioinform Comput Biol Biomed Inform (2013) 2013: , 569. |
[28] | Scheubert L , Lustrek M , Schmidt R , Repsilber D , Fuellen G ((2012) ) Tissue-based Alzheimer gene expression markers-comparison of multiple machine learning approaches and investigation of redundancy in small biomarker sets. BMC Bioinformatics 13: , 266. |
[29] | Hampel H , Lista S ((2012) ) Alzheimer disease: From inherited to sporadic AD-crossing the biomarker bridge. Nat Rev Neurol 8: , 598–600. |
[30] | Malpass K ((2011) ) Alzheimer disease: A novel biomarker to detect early-stage Alzheimer disease. Nat Rev Neurol 7: , 420. |
[31] | Dickerson BC , Stoub TR , Shah RC , Sperling RA , Killiany RJ , Albert MS , Hyman BT , Blacker D , Detoledo-Morrell L ((2011) ) Alzheimer-signature MRI biomarker predicts AD dementia in cognitively normal adults. Neurology 76: , 1395–1402. |
[32] | Takeda S , Sato N , Rakugi H , Morishita R ((2010) ) Plasma beta-amyloid as potential biomarker of Alzheimer disease: Possibility of diagnostic tool for Alzheimer disease. Mol Biosyst 6: , 1760–1766. |
[33] | Friedemann M , Helk E , Tiiman A , Zovo K , Palumaa P , Tougu V ((2015) ) Effect of methionine-35 oxidation on the aggregation of amyloid-beta peptide. Biochem Biophys Rep 3: , 94–99. |
[34] | Tougu V , Karafin A , Zovo K , Chung RS , Howells C , West AK , Palumaa P ((2009) ) Zn(II)- and Cu(II)-induced non-fibrillar aggregates of amyloid-beta (1-42) peptide are transformed to amyloid fibrils, both spontaneously and under the influence of metal chelators. J Neurochem 110: , 1784–1795. |
[35] | Chiti F , Dobson CM ((2009) ) Amyloid formation by globular proteins under native conditions. Nat Chem Biol 5: , 15–22. |
[36] | Aguzzi A , O’Connor T ((2010) ) Protein aggregation diseases: Pathogenicity and therapeutic perspectives. Nat Rev Drug Discov 9: , 237–248. |
[37] | Tiiman A , Noormagi A , Friedemann M , Krishtal J , Palumaa P , Tougu V ((2013) ) Effect of agitation on the peptide fibrillization: Alzheimer’s amyloid-beta peptide 1-42 but not amylin and insulin fibrils can grow under quiescent conditions. J Pept Sci 19: , 386–391. |
[38] | Hudson SA , Ecroyd H , Kee TW , Carver JA ((2009) ) The thioflavin T fluorescence assay for amyloid fibril detection can be biased by the presence of exogenous compounds. FEBS J 276: , 5960–5972. |
[39] | Noormagi A , Gavrilova J , Smirnova J , Tougu V , Palumaa P ((2010) ) Zn(II) ions co-secreted with insulin suppress inherent amyloidogenic properties of monomeric insulin. Biochem J 430: , 511–518. |
[40] | Noormagi A , Primar K , Tougu V , Palumaa P ((2012) ) Interference of low-molecular substances with the thioflavin-T fluorescence assay of amyloid fibrils. J Pept Sci 18: , 59–64. |
[41] | Wolfe LS , Calabrese MF , Nath A , Blaho DV , Miranker AD , Xiong Y ((2010) ) Protein-induced photophysical changes to the amyloid indicator dye thioflavin T. Proc Natl Acad Sci U S A 107: , 16863–16868. |
[42] | Krebs MR , Bromley EH , Donald AM ((2005) ) The binding of thioflavin-T to amyloid fibrils: Localisation and implications. J Struct Biol 149: , 30–37. |
[43] | Hawe A , Sutter M , Jiskoot W ((2008) ) Extrinsic fluorescent dyes as tools for protein characterization. Pharm Res 25: , 1487–1499. |
[44] | Biancalana M , Koide S ((2010) ) Molecular mechanism of Thioflavin-T binding to amyloid fibrils. Biochim Biophys Acta 1804: , 1405–1412. |
[45] | Batzli KM , Love BJ ((2015) ) Agitation of amyloid proteins to speed aggregation measured by ThT fluorescence: A call for standardization. Mater Sci Eng C Mater Biol Appl 48: , 359–364. |
[46] | Gade Malmos K , Blancas-Mejia LM , Weber B , Buchner J , Ramirez-Alvarado M , Naiki H , Otzen D ((2017) ) ThT 101: A primer on the use of thioflavin T to investigate amyloid formation. Amyloid 24: , 1–16. |
[47] | Younan ND , Viles JH ((2015) ) A comparison of three fluorophores for the detection of amyloid fibers and prefibrillar oligomeric assemblies. ThT (Thioflavin T); ANS (1-Anilinonaphthalene-8-sulfonic Acid); and bisANS (4,4’-Dianilino-1,1’-binaphthyl-5,5’-disulfonic Acid). Biochemistry 54: , 4297–4306. |
[48] | Garvey M , Tepper K , Haupt C , Knupfer U , Klement K , Meinhardt J , Horn U , Balbach J , Fandrich M ((2011) ) Phosphate and HEPES buffers potently affect the fibrillation and oligomerization mechanism of Alzheimer’s Abeta peptide. Biochem Biophys Res Commun 409: , 385–388. |
[49] | Perez-Serradilla JA , Ortiz MC , Sarabia L , de Castro MD ((2007) ) Focused microwave-assisted Soxhlet extraction of acorn oil for determination of the fatty acid profile by GC-MS. Comparison with conventional and standard methods. Anal Bioanal Chem 388: , 451–462. |
[50] | Campanella B , Pulidori E , Onor M , Passaglia E , Tegli S , Izquierdo CG , Bramanti E ((2016) ) New polymeric sorbent for the solid-phase extraction of indole-3-acetic acid from plants followed by liquid chromatography - Fluorescence detector. Microchem J 128: , 68–74. |
[51] | Godlewska K , Michalak I , Tuhy L , Chojnacka K ((2016) ) Plant growth biostimulants based on different methods of seaweed extraction with water. Biomed Res Int 2016: , 5973760. |
[52] | Pereira C , Barros L , Ferreira ICFR ((2016) ) Extraction, identification, fractionation and isolation of phenolic compounds in plants with hepatoprotective effects. J Sci Food Agric 96: , 1068–1084. |
[53] | Sibul FS , Orcic DZ , Svircev E , Mimica-Dukic NM ((2016) ) Optimization of extraction conditions for secondary biomolecules from various plant species. Hem Ind 70: , 473–483. |
[54] | Mishra S , Palanivelu K ((2008) ) The effect of curcumin (turmeric) on Alzheimer’s disease: An overview. Ann Indian Acad Neurol 11: , 13–19. |
[55] | Vandersteen A , Hubin E , Sarroukh R , De Baets G , Schymkowitz J , Rousseau F , Subramaniam V , Raussens V , Wenschuh H , Wildemann D , Broersen K ((2012) ) A comparative analysis of the aggregation behavior of amyloid-beta peptide variants. FEBS Lett 586: , 4088–4093. |
[56] | Iadanza MG , Jackson MP , Radford SE , Ranson NA ((2016) ) MpUL-multi: Software for calculation of amyloid fibril mass per unit length from TB-TEM images. Sci Rep 6: , 21078. |
[57] | Harris JR ((2008) ) Cholesterol binding to amyloid-beta fibrils: A TEM study. Micron 39: , 1192–1196. |
[58] | Mirjalili MH , Moyano E , Bonfill M , Cusido RM , Palazon J ((2009) ) Steroidal lactones from Withania somnifera, an ancient plant for novel medicine. Molecules 14: , 2373–2393. |
[59] | Devi PU , Akagi K , Ostapenko V , Tanaka Y , Sugahara T ((1996) ) Withaferin A: A new radiosensitizer from the Indian medicinal plant Withania somnifera. Int J Radiat Biol 69: , 193–197. |
[60] | Doma M , Abhayankar G , Reddy VD , Kavi Kishor PB ((2012) ) Carbohydrate and elicitor enhanced withanolide (withaferin A and withanolide A) accumulation in hairy root cultures of Withania somnifera (L.). Indian J Exp Biol 50: , 484–490. |
[61] | Khedgikar V , Ahmad N , Kushwaha P , Gautam J , Nagar GK , Singh D , Trivedi PK , Mishra PR , Sangwan NS , Trivedi R ((2015) ) Preventive effects of withaferin A isolated from the leaves of an Indian medicinal plant Withania somnifera (L.): Comparisons with 17-beta-estradiol and alendronate. Nutrition 31: , 205–213. |
[62] | Kumar S , Singh R , Gajbhiye N , Dhanani T ((2018) ) Extraction optimization for phenolic- and Withanolide-rich fractions from Withania somnifera roots: Identification and quantification of Withaferin A, 12-deoxywithastro-monolide, and Withanolide A in plant materials and marketed formulations using a reversed-phase HPLC-photodiode array detection method. J AOAC Int 101: , 1773–1780. |
[63] | Roy RV , Suman S , Das TP , Luevano JE , Damodaran C ((2013) ) Withaferin A, a steroidal lactone from Withania somnifera, induces mitotic catastrophe and growth arrest in prostate cancer cells. J Nat Prod 76: , 1909–1915. |
[64] | Siddique AA , Joshi P , Misra L , Sangwan NS , Darokar MP ((2014) ) 5,6-de-epoxy-5-en-7-one-17-hydroxy withaferin A, a new cytotoxic steroid from Withania somnifera L. Dunal leaves. Nat Prod Res 28: , 392–398. |
[65] | Szarc vel Szic K , Op de Beeck K , Ratman D , Wouters A , Beck IM , Declerck K , Heyninck K , Fransen E , Bracke M , De Bosscher K , Lardon F , Van Camp G , Vanden Berghe W ((2014) ) Pharmacological levels of Withaferin A (Withania somnifera) trigger clinically relevant anticancer effects specific to triple negative breast cancer cells. PLoS One 9: , e87850. |
[66] | Nathan PJ , Clarke J , Lloyd J , Hutchison CW , Downey L , Stough C ((2001) ) The acute effects of an extract of Bacopa monniera (Brahmi) on cognitive function in healthy normal subjects. Hum Psychopharmacol 16: , 345–351. |
[67] | Roodenrys S , Booth D , Bulzomi S , Phipps A , Micallef C , Smoker J ((2002) ) Chronic effects of Brahmi (Bacopa monnieri) on human memory. Neuropsychopharmacology 27: , 279–281. |
[68] | Stough C , Lloyd J , Clarke J , Downey LA , Hutchison CW , Rodgers T , Nathan PJ ((2001) ) The chronic effects of an extract of Bacopa monniera (Brahmi) on cognitive function in healthy human subjects. Psychopharmacology (Berl) 156: , 481–484. |
[69] | Zheng CJ , Qin LP ((2007) ) Chemical components of Centella asiatica and their bioactivities. Zhong Xi Yi Jie He Xue Bao 5: , 348–351. |