Interictal Epileptiform Activity in the Foramen Ovale Electrodes of a Frontotemporal Dementia Patient
Abstract
Frontotemporal dementia (FTD) is a frequent cause of cognitive decline. While epilepsy is an important comorbidity of Alzheimer’s disease, we lack studies on its presence in FTD. We report on an FTD patient with transient, short-term changes of behavior and cognitive performance suggesting non-convulsive epilepsy. Video-EEG recording with foramen ovale (FO) electrodes revealed mesio-temporal epileptiform potentials, undetectable by scalp leads. We also found beta spindles in the FO electrodes, not described in the literature. We conclude that video-EEG monitoring with FO electrodes might usefully complement the assessment of dementia-associated epilepsy opening new perspectives in dementia-research.
INTRODUCTION
The increase in the incidence of major neurocognitive disorders represents an enormous socio-medical burden. Since we are still unable to modify the progression of neurocognitive disorders, recent studies have focused on preventive measures and on early identification of comorbid medical conditions including epilepsy [1]. In Alzheimer’s disease (AD), increased risk for developing epileptiform discharges and epileptic seizures have been demonstrated [2]. Apparently, epileptiform activity is associated with accelerated disease progression in both animal [3] and human studies [4]. In AD, therapeutic trials with antiepileptic drugs are promising as well [5]. However, a recent paper demonstrated that epileptiform activity in some AD cases might be detectable only by foramen ovale (FO) electrodes and could be invisible on scalp electrodes [6].
Frontotemporal dementia (FTD) is a group of clinically and neuropathologically different disorders representing approximately 15% of all neurodegenerative dementia cases [7]; making it a prominent cause of cognitive deterioration. Its clinical symptoms are progressive changes in social behavior and personality as well as the loss of sensory and motor speech functions [8]. The pathological hallmark is the lobar degeneration of the frontal and temporal lobes. AD and FTD are similar in several respects, e.g., overlapping imaging findings and clinical syndrome [9]. While in AD, the primarily affected structure is the hippocampus leading to disturbed short-term memory and orientation, frontal lobe symptoms dominate in FTD. Common protein aggregations including tau neurofibrils have been neuropathologically demonstrated as well [10].
While epilepsy is considered an important co-morbidity of AD, only a few case studies investigated the co-occurrence of epilepsy in FTD [11–12], although the first epileptic FTD case has been described in 1958 [13]. The only study that focused on the prevalence of epilepsy in FTD demonstrated seizures in 5% of FTD patients [14]. Unfortunately, no data are available about the impact of epilepsy on FTD progression and clinical presentation.
With our report, we aim to highlight the importance of epileptological considerations in FTD. We explored epilepsy in a non-epileptic FTD patient by a well-known, but in this field not utilized diagnostic method – video-EEG monitoring with implanted FO electrodes – for the assessment of dementia-associated epilepsy.
CASE REPORT
A 74-year-old retired white female accountant was admitted to our neurology department for progressive cognitive decline, which had started insidiously, about four years prior to admission. Her symptoms were difficulty with language, sensory dysphasia, impairment of memory, and overall disorientation. According to her husband, her first symptom was the inability to form uncommon and complex words, so aphasia was the most prominent deficit at symptom onset leading to impaired daily living activities. Her condition slowly deteriorated leading to a loss of everyday activities, becoming unable to perform simple tasks related to household, then to her personal hygiene. Her husband reported that the executive performance as well as her spatial and temporal orientation varied over time and showed frequent daily alterations. She had type-2 diabetes for 4 years well controlled with diet and metformin. She had atrial fibrillation necessitating preventive apixaban treatment. She has had hypertension and hypercholesterinemia for 5 years treated with the combination of simvastatin, betaxolol, valsartan, furosemide, and digoxin. She was taking paroxetin for depression. Her body mass index was 25 with normal vital parameters apart from arrhythmia. She was passive and apathic while in hospital; lying inactively in bed the whole time, often in a sleep-like state during the day and apparently remaining so in the night. She was sometimes cheerful, tolerating and cooperating reasonably well with any queries or interventions; she seemed friendly and quiet with no interest, motivations, or denial.
Her blood screen, TSH, and coagulation parameters were normal. Her cerebrospinal fluid (CSF) tau-level was 239 pg/ml (normal range: 341±171 pg/ml), p-tau level was 24 pg/ml (normal range: 35.84–66.26 pg/ml), amyloid-β (Aβ) level was 717 pg/ml (normal range: 797±230 pg/ml), the Aβ/tau ratio was 3, while the Aβ/p-tau ratio was 29.88. Thus, the amyloid and tau levels in the CSF were in the normal range excluding AD. CSF ubiquitin or TDP-43 testing could not be performed.
The MRI brain scan revealed moderate small vessel disease with important diffuse cerebral atrophy, mainly affecting the frontal and temporal lobes with left side dominance (Fig. 1).
Fig.1
Preoperative brain MRIs (left: coronal view; right: sagittal view) of the patient. MRI brain scan revealed diffuse cortical atrophy; the frontal and temporal lobes were the most affected, predominantly on the left side.
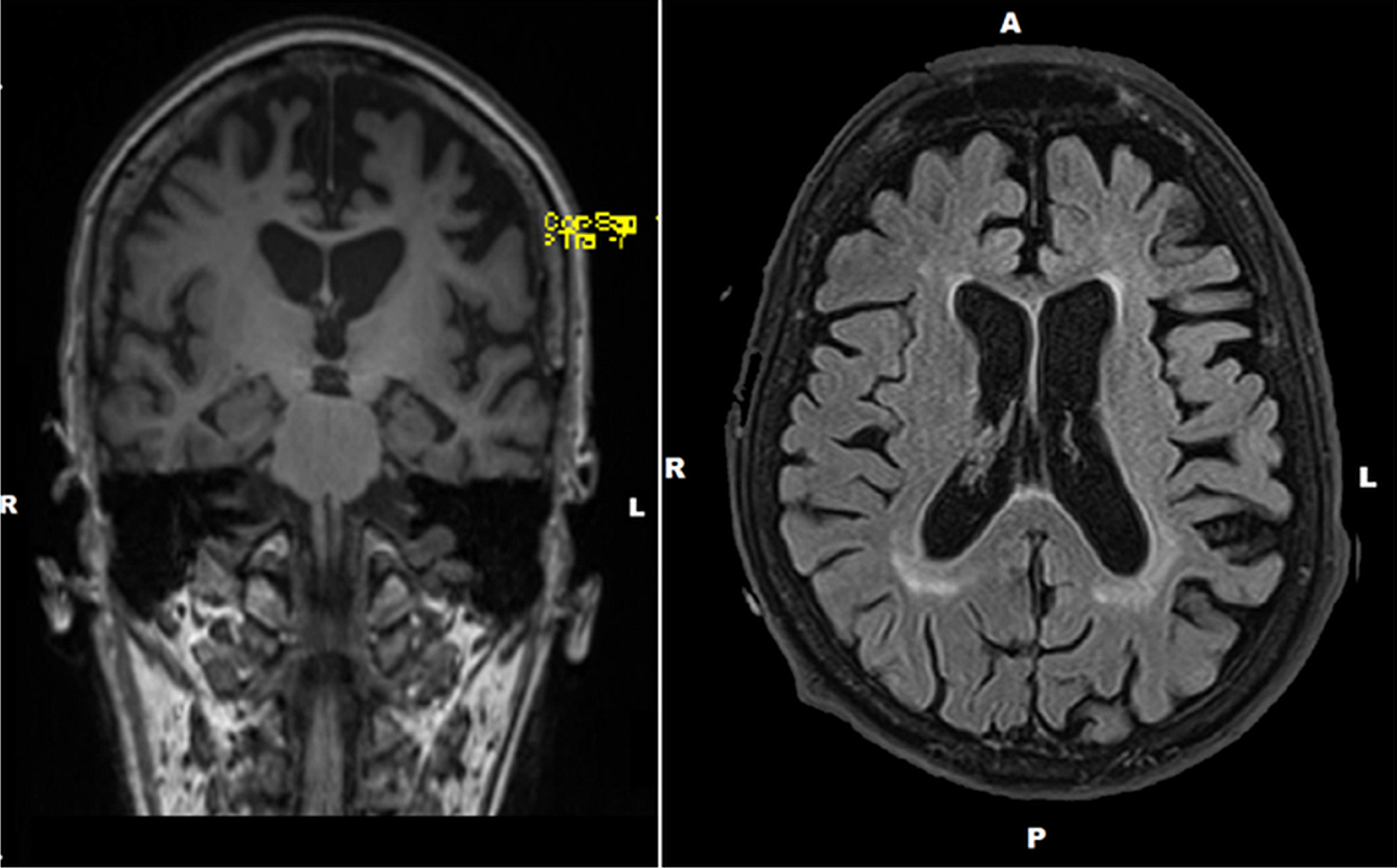
To analyze structural results more precisely, hippocampal volumes and cortical thickness were calculated with FreeSurfer5.3 image analysis suite (freely available for download: http://surfer.nmr.mgh.harvard.edu). The technical details are demonstrated in previous reports, no changes were made to the pipeline. The image process contains motion correction, removal of non-brain tissue, automated Talairach transformation, segmentation of deep gray matter structures and subcortical white matter, intensity normalization, tessellation of white-gray matter boundary, automated topology correction and surface deformation following intensity gradients. Following the cortical models, Freesurfer managed deformable procedures such as surface inflation, registration to spherical atlas, parcellation of cerebral cortex into units and creation of different surface based data. Cortical models and results of segmentation were quality controlled.
Average left frontal thickness (in mm) was 1.79±0.16, right frontal thickness was 2.01±0.21, left temporal thickness was 2.33±0.32, right temporal thickness was 2.45±0.36, left parietal thickness was 2.01±0.2 and right parietal thickness was 2.18±0.29. The temporal and frontal values are below the normal ranges if we compare it to data from the literature, especially on the left side, while the parietals are relatively preserved, being in line with the previous reports on FTD [15]. The volume of the left hippocampus was 3301 mm3, and of the right was 3997 mm3. These values are in the normal range based on our previous study with the same analysis method [16]. However, the left hippocampus was significantly smaller showing 17.5% asymmetry which suggests pathological changes in the volume of left hippocampus besides the normal values. Volumetric asymmetry could be considered significant above 10%. The volume of the left entorhinal cortex was 1881 mm3, while that of the right was 1811 mm3. These values and the disappearance of the difference between the two sides indicate Alzheimer or frontotemporal pathology based on previous data [17]. Altogether, the left dominant frontotemporal thinning and normal parietal thickness, and the involvement of the entorhinal cortex suggested the morphometry of FTD [18]. The routine EEG showed 20–40 uV, 9-10 Hz alpha background with frontal beta activity. We detected occasional left temporal focal slowing. No specific epileptiform activity were seen. Detailed neuropsychological analysis including Addenbrooke’s Cognitive Examination (ACE) was performed. This test has a high sensitivity (0.9) and specificity (0.93) in the differentiation of cognitive disorders, especially in the diagnosis of AD and FTD [19]. Her ACE score was 32/100. The Verbal-Language/Orientation-Memory ratio of ACE, which sensitively discriminates AD and FTD [20], scored 1.9 suggesting FTD. The Mini-Mental State Examination score extracted from ACE [21] was 16/30. The neuropsychological results were consistent with a moderate cognitive decline. The most impaired cognitive domains were single-word retrieval in spontaneous speech and naming, reading, verbal short-term memory; whereas repetition, spatial orientation and grammar were relatively preserved. No early behavioral disinhibition, apathy/inertia, early loss of sympathy/empathy, presence of perseverative behavior and hyperorality were reported, so the diagnosis of behavior variant FTD was improbable [22]. Based on current diagnostic recommendations, we diagnosed primary progressive aphasia related to FTD [23–24]. Based on the caregiver’s report on the significant daily variability of her cognitive functions the presence of diffuse Lewy-body dementia was also considered. However, its core criteria as Parkinsonism or visual hallucinations were not present, therefore it was excluded. To assess the severity of cognitive fluctuation we used an easily applicable, bed-side test with high sensitivity in measuring visuo-spatial performance, the Corsi Block-Tapping Task (CBTT) [25]. We carried out CBTT with 2-3 items four times in one day; in better and worse cognitive periods (reported by the medical staff and her husband) and compared the number of correct answers with ANOVA analysis using the IBM SPSS20 software. We revealed significant differences in the results of the four trials (df: 3; Mean Square: 1.098; F: 4.567; p: 0.004). Based on both the neuropsychology scores and the history suggesting the possibility of non-convulsive seizure-episodes, we suspected concomitant epilepsy. Risk factors for developing epileptic seizures including definitive structural lesions on MRI, previous history of head trauma, childhood seizures, CNS infection, drug or alcohol dependency or electroconvulsive therapy were absent.
To disclose the potential epileptiform activity, we performed a 24-h Holter-EEG, followed by a 3-day-long continuous scalp video-EEG monitoring. We recorded one episode of slightly irregular rhythmic theta activity lasting for 20 s during light sleep in the right temporal leads. We also detected very rare bitemporal independent sharp waves with slight right dominance, possibly consistent with epileptiform activity [26]. The long-term EEG revealed disturbed sleep macrostructure with superficial night sleep, no deep sleep and very short REM sleep episodes; no sleep spindles or K complexes were found.
The EEG changes supported our suspicion that the significant fluctuations of her cognitive performance might be related to temporal lobe epileptic activity. Therefore, FO electrodes were surgically implanted (Fig. 2) under general anesthesia; and continuous video-EEG recording using both FO and scalp electrodes were performed for 4 days.
Fig.2
Postoperative cranial CT of the patient. The postoperative CT demonstrated the position of foramen ovale electrodes inside the subarachnoidal space of the cisterna ambiens below the parahippocampal gyri (indicated by arrows).
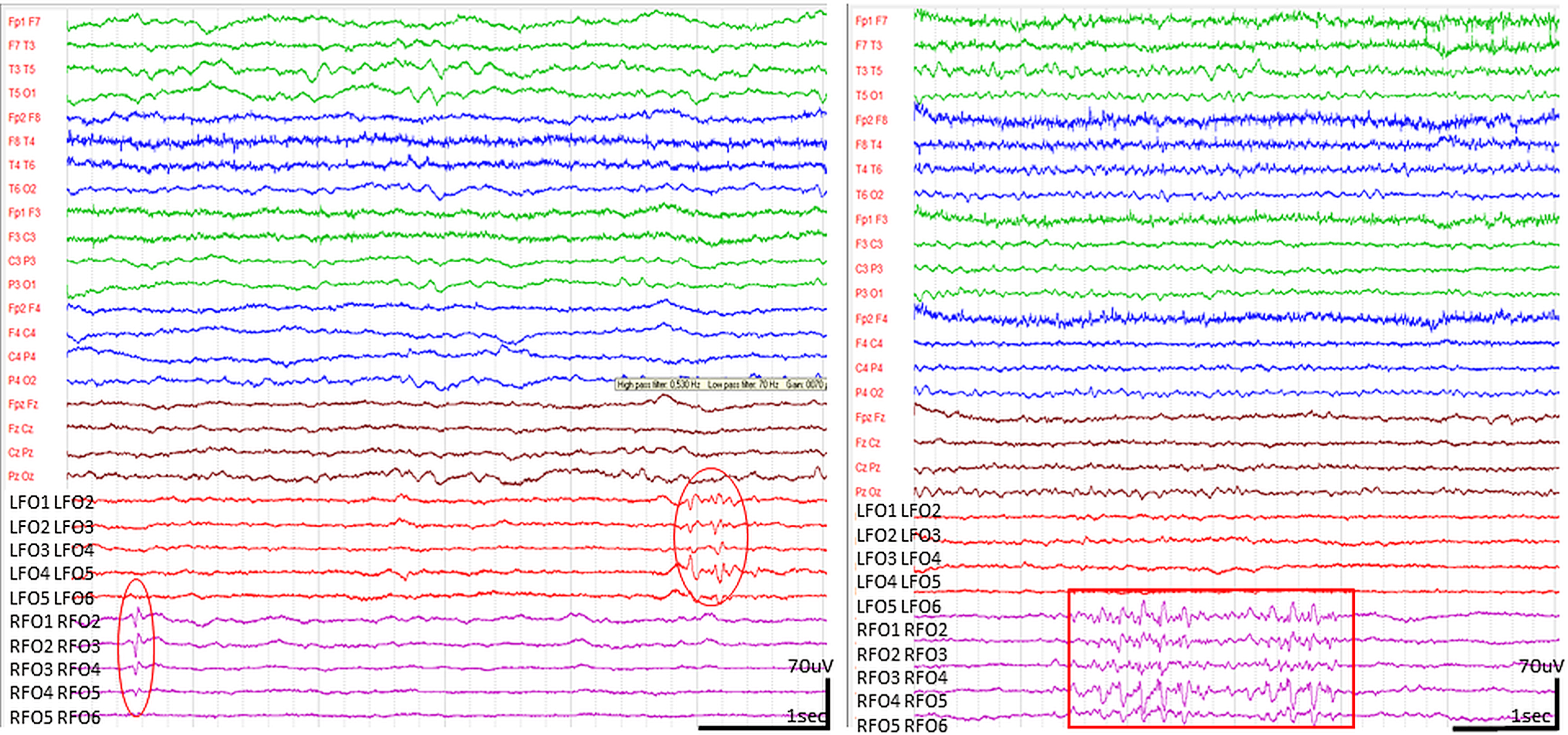
The national and institutional ethic committees approved the FO electrode implantations and the recording; the family of the patient gave written informed consent to the procedure. The FO electrode implantation and the video-EEG monitoring period were uneventful, no surgical complications, behavioral or cooperation issues occurred. Frequent neuropsychological testing with CBTT was carried out during monitoring. The patient perfectly complied so we could perform clean, artefact-free EEG recordings. The recordings revealed bilateral independent spikes on the FO leads; in average, 30 unambiguous single spikes on the right side and 4 on the left per day (Fig. 3). We considered these graphoelements interictal spikes because of their paroxysmal appearance, the abrupt change in polarity, the typical spike duration (less than 70 ms) and because of their consistent temporal field distribution [27]. The spike activity occurred mainly during superficial NREM sleep, similarly to typical temporal epileptiform activity. We recorded runs of 11-12 Hz beta waves with or without 5 Hz theta elements and spike-like components, mostly during light NREM sleep, much less during wakefulness and none during the short REM periods, as well as >1 s long runs of sharp waves more often on the left side (Figs. 3 and 4).
Fig.3
Epileptiform discharges seen in FO leads. (High pass filter 0.5 Hz, low pass filter 70 Hz). Bilateral, independent spikes on the left side (circles) with amplitudes lower than l00 μV. In some cases, spikes were associated to beta runs with 11-12 Hz activity superimposed on 5 Hz theta elements (right side, marked with a rectangle). The spikes and beta-run-related spikes occurred predominantly in the right FO leads. None of them propagated to the scalp.
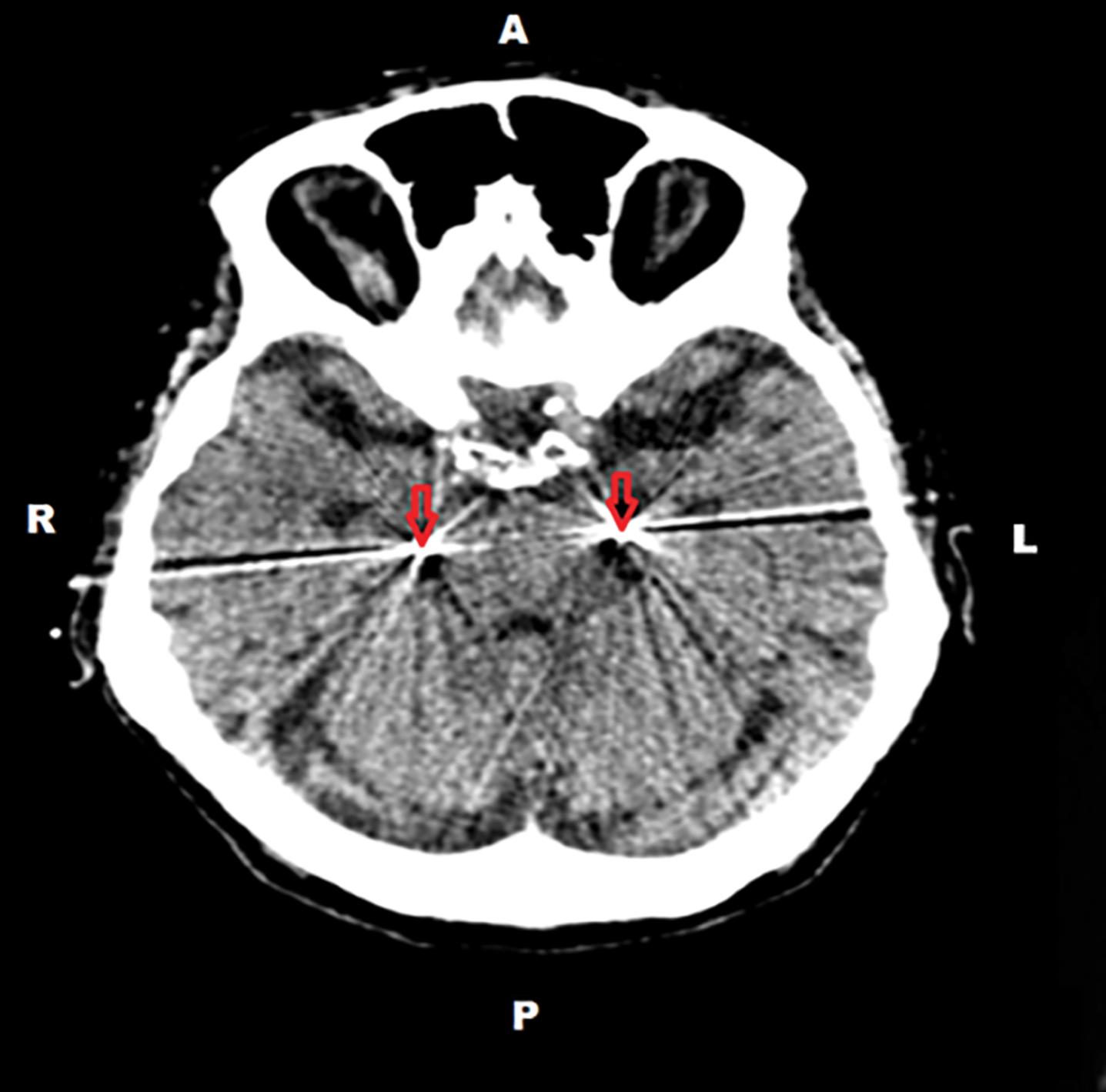
Fig.4
Spindle-like graphoelements in the FO leads during wakefulness. (High pass filter 0.5 Hz low pass filter 70 Hz). Runs of bi-, or unilateral, spindle-shape sharp elements with 11-12 Hz frequency appeared in the FO leads on both sides, predominantly during NREM sleep (left side, marked with rectangle). In wakefulness, variable length (1 s-15 s) beta spindles with 15-16 Hz frequency were often seen in the FO leads, preceding eye movements, blinking or other muscle activity (right side, marked by rectangle). The circle demonstrates the EEG artefact of blinking.
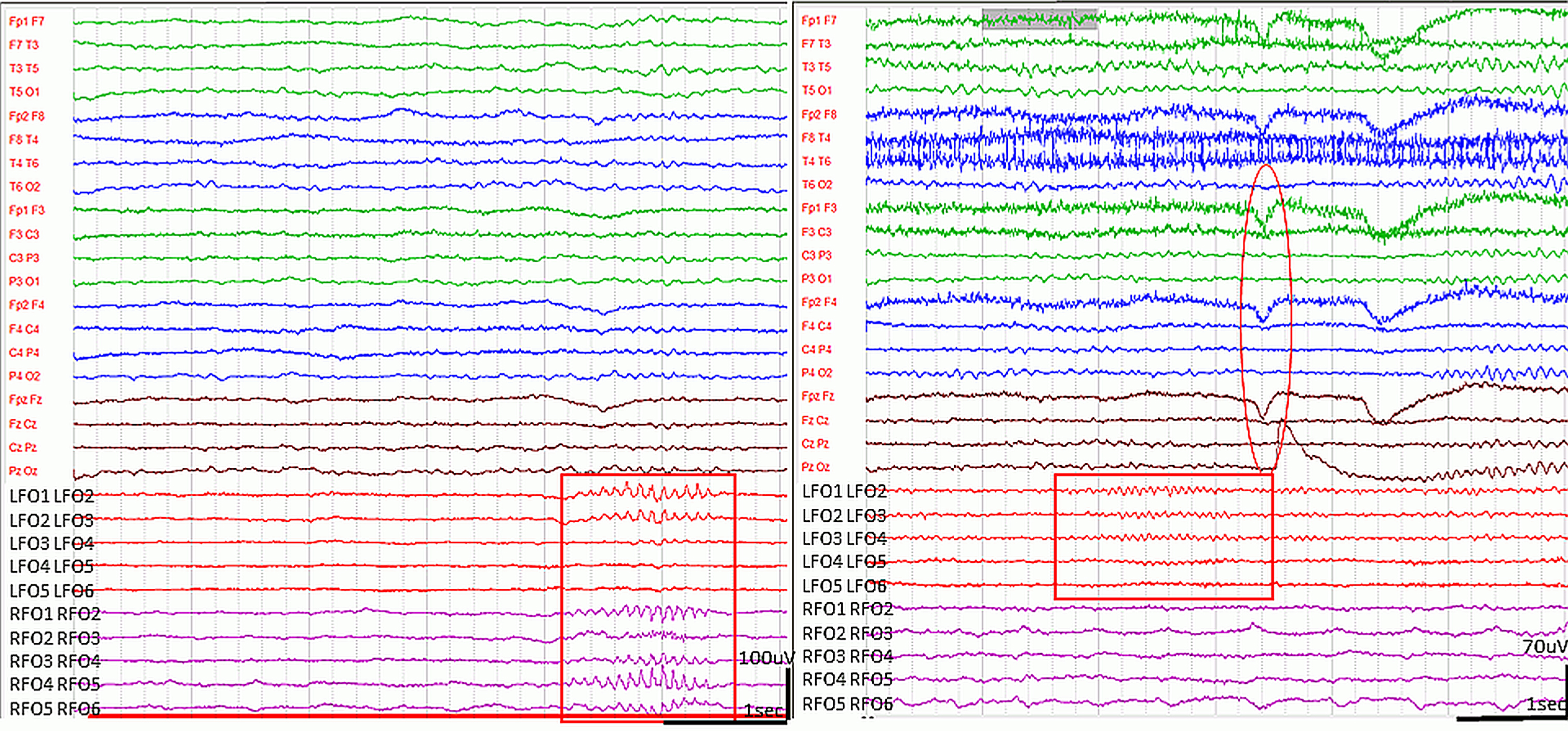
None of the epileptiform EEG events propagated onto the scalp electrodes. The spikes and sharp waves had an amplitude <l00 μV, and occurred frequently in the FO electrodes. The FO revealed 15-16 Hz, crescendo-decrescendo beta spindles during wakefulness, frequently preceding movements (Fig. 4).
Because of the occurrence of epileptiform discharges, we initiated clobazam treatment during the monitoring period, however there were no changes in the occurrence of epileptiform potentials and the neuropsychological performance following the drug administration in the next 2 days. According to some data, levetiracetam might be effective in AD-related epilepsy and it is easier to reach a steady state much earlier than clobazam [28–29], therefore we started levetiracetam and we plan close follow-up.
DISCUSSION
To our knowledge, this is the first report in the literature, exploring epilepsy using FO electrodes in a patient with FTD. Our report reinforces the findings of a recent FO study demonstrating hidden epileptic activity in two AD patients without a previous history of epileptic seizures; epileptiform activity could be hidden on scalp EEG in neurocognitive disorders being demonstrable only by deep electrodes [6]. Our 74-year-old female patient suffering from a subtype of FTD has never had overt epileptic seizures; however, an important daily variability of her cognitive performance has raised the possibility of underlying undetected epilepsy. Her routine EEG showed focal temporal slowing; the 24-hour EEG revealed a rhythmic theta run in the right temporal region with bitemporal sharp waves raising the suspicion of undetected interictal and dubious seizure activity. Monitoring with FO electrodes confirmed our suspicion of an underlying epilepsy; revealing different types of spikes and sharp waves. The finding of waking beta spindling in the FO leads is another gain, needing further studies and explanation.
Whereas it has recently been highlighted that epilepsy represents an important co-morbidity of AD [2], studies are lacking on the prevalence of epileptic activity in FTD [30]. Because AD and FTD share a number of clinical and pathological features, the importance of comorbid epilepsy is possibly underestimated in FTD. As in AD, epileptiform discharges may accelerate the progression of the disease [3, 4], therefore, there is an urgent need to pay more attention to FTD-associated epilepsy.
It is hard to diagnose epilepsy in dementia patients, especially the non-convulsive or clinically hidden seizures [31]. EEG is not frequently utilized in the assessment of cognitive decline; because dementia protocols [32] do not recommend it. The routine, 30 min long EEG performed during wakefulness has limited sensitivity even in patients with documented epilepsy, identifying only about 50% of epilepsy patients [33, 34]. Prolonged, serial and especially sleep EEGs have higher sensitivity [33, 35], however, the few studies examining dementia patients with this method all focused on AD only [4, 35, 36]. FO implantation is a semi-invasive method providing the opportunity for parahippocampal recording without skull opening [37]. It has been found to be a relatively safe and highly sensitive method in the assessment of mesio-temporal epilepsy [38]. In the study of Torre et al. on temporal lobe epilepsy patients, 70% of 2,280 epileptiform discharges were captured only by FO electrodes and were not detected by scalp electrodes [39]. In the study of Clemens et al., FO spiking rate was 2–10 times higher than scalp spiking rate [40]. In a more recent study, monitoring with FO-electrodes proved to be accurate in 90% of presurgical evaluations in mesio-temporal epilepsy [41]. A new study demonstrated hidden epileptic activity in an AD patients with FO electrodes, where 95% of the epileptform spikes were not visible on the scalp electrodes [6]. Our findings are in line with these reports; the epileptiform discharges seen on the FO leads of our patient did not propagate to the scalp.
The identification of epileptiform discharges and epileptic seizures in primary dementia seems essential because they could augment the progression of neurodegeneration [3, 4] and compromise cognitive performance [42]. Based on our results, video-EEG monitoring with FO electrodes might importantly contribute to the clarification of dementia-associated epilepsy. Because FO implantation is a sophisticated neurosurgical intervention with the possibility of some complications, a careful patient selection is essential.
The additional gain of long-term EEG is that it can clarify the sleep structure and comorbid sleep disorders which can be treated improving the quality of life and also the cognitive performance of dementia patients [2, 43]. The lack of deep sleep is not surprising based upon the known data on slow wave sleep disruption [44] and peculiar microstructural sleep changes of FTD patients [45]. Apparently, sleep parameters are significantly affected and sleep disruption might start even earlier in FTD than in AD [46]. Studies focusing on the early preventive strategies in dementia are showing promising results [1] and it might be related to the increased physical activity which has a positive effect on the cardiovascular status and additionally; increases the quantity of deep sleep [47]. Medication with a potential to augment slow-wave sleep might be interesting future direction in dementia [48]. Further sleep studies might contribute to the early differentiation of the disorders as well [49].
Finding 15-16 Hz beta spindle-like runs in the FO leads during active wakefulness in our patient is curious. To our knowledge, we are the first reporting spindle-like 16 Hz beta activity originating from the deep temporal regions in an awake patient with cortical dementia. The beta runs and spindles in our study were not seen on the scalp, only in the FO leads and were predominantly attached to motor and cognitive activity indicating that these phenomena might differ from sleep spindles. The elaboration of the biological significance of this activity requires further studies and comparisons to the findings of epilepsy studies using FO recordings.
In view of the morphological features and NREM sleep-related distribution of our patient’s spikes and sharp waves as well as the variable cognitive performance, we suspect an underlying epilepsy.
It is always difficult to draw conclusions from a single case, however, our study calls attention to the possibility of epilepsy being important and under investigated feature in FTD. A limitation of our study is the presence of concomitant vascular disease (diabetes, hypertension) being possible risk factors for developing seizures. However, these conditions were well controlled with medication, the patient did not have diabetic complications as neuropathy or retionpathy and the MRI did not show severe vascular encephalopathy. Furthermore, major drawbacks of the FO method are its invasivity and the lack of previous results regarding neurocognitive disorders; making it difficult to clearly explain our findings. However, EEG monitoring with FO electrodesis a promising approach which might support the diagnosis and guide the treatment of epilepsy in patients with cognitive impairment, and open new perspectives in the research of dementia.
CONFLICT OF INTEREST
The authors have no conflict of interest to report.
ACKNOWLEDGMENTS
Our research was supported by the National Brain Research Program (KTIA_NAP_13-1-2013-0001) and MET Hungary Ltd. Special thanks to Ádám Szabó for volumetry and thickness analysis and to Bence Németh for the quality control of the figures.
REFERENCES
[1] | Kivipelto M , Solomon A , Ahtiluoto S , Ngandu T , Lehtisalo J , Antikainen R , Lindström J ((2013) ) The Finnish geriatric intervention study to prevent cognitive impairment and disability (FINGER): Study design and progress. Alzheimers Dement 9: , 657–665. |
[2] | Horvath A , Szucs A , Barcs G , Noebels JL , Kamondi A ((2016) ) Epileptic seizures in Alzheimer disease: A review. Alzheimer Dis Assoc Disord 30: , 186–192. |
[3] | Dolev I , Fogel H , Milshtein H , Berdichevsky Y , Lipstein N , Brose N , Slutsky I ((2013) ) Spike bursts increase amyloid-beta 40/42 ratio by inducing a presenilin-1 conformational change. Nat Neurosci 16: , 587–595. |
[4] | Vossel KA , Ranasinghe KG , Beagle AJ , Mizuiri D , Honma SM , Dowling AF , Karydas AM ((2016) ) Incidence and impact of subclinical epileptiform activity in Alzheimer’s disease. Ann Neurol 80: , 858–870. |
[5] | Cumbo E , Ligori LD ((2010) ) Levetiracetam, lamotrigine, and phenobarbital in patients with epileptic seizures and Alzheimer’s disease. Epilepsy Behav 17: , 461–466. |
[6] | Lam AD , Deck G , Goldman A , Eskandar EN , Noebels J , Cole AJ ((2017) ) Silent hippocampal seizures and spikes identified by foramen ovale electrodes in Alzheimer’s disease. Nat Med 23: , 678–680. |
[7] | Ratnavall EB , Rayne C , Dawson K , Hodges JR ((2002) ) The prevalence of frontotemporal dementia. Neurology 58: , 1615–1621. |
[8] | Ichikawa H , Kawamura M ((2009) ) Symptoms of frontotemporal dementia [Japanese]. Brain Nerve 61: , 1227–1235. |
[9] | Frings L , Yew B , Flanagan E , Lam BY , Hüll M , Huppertz HJ , Hornberger M ((2014) ) Longitudinal grey and white matter changes infrontotemporal dementia and Alzheimer’s disease. PLoS One 9: , e90814. |
[10] | McKhann GM , Albert MS , Grossman M , Miller B , Dickson D , Trojanowski JQ ((2001) ) Clinical and pathological diagnosis of frontotemporal dementia: Report of the Work Group on Frontotemporal Dementia and Pick’s Disease. Arch Neurol 58: , 1803–1809. |
[11] | Castro-Suarez S , Martinez P , Meza M ((2016) ) Pathological gambling and epilepsy in patients with frontotemporal dementia: Two case reports. Rev Peru Med Exp Salud Publica 33: , 588–592. |
[12] | Le Ber I , De Septenville A , Guerreiro R , Bras J , Camuzat A , Caroppo P , Dubois B ((2014) ) Homozygous TREM2 mutation in a family with atypical frontotemporal dementia. Neurobiol Aging 35: , 2419–e23. |
[13] | Agostini L , Riccio A , Petiziol A ((1958) ) Case of presenile dementia associated with epileptic crises & with biopsy findings of Pick’s diseases; case report. Riv Neurol 28: , 376. |
[14] | Sarkis RA , Dickerson BC , Cole AJ , Chemali ZN ((2016) ) Clinical andneurophysiologic characteristics of unprovoked seizures inpatients diagnosed with dementia. J Neuropsy Clin Neurosci 28: , 56–61. |
[15] | Du AT , Schuff N , Kramer JH , Rosen HJ , Gorno-Tempini ML , Rankin K , Weiner MW ((2007) ) Different regional patterns of cortical thinning in Alzheimer’s disease and frontotemporal dementia. Brain 130: , 1159–1166. |
[16] | Csukly G , Sirály E , Fodor Z , Horváth A , Salacz P , Hidasi Z , Szabó Á ((2016) ) The differentiation of amnestic type MCI from the non-amnestic types by structural MRI. Front Aging Neurosci 8: , 52. |
[17] | Frisoni GB , Laakso MP , Beltramello A , Geroldi C , Bianchetti A , Soininen H , Trabucchi M ((1999) ) Hippocampal and entorhinal cortex atrophy in frontotemporal dementia and Alzheimer’s disease. Neurology 52: , 91–91. |
[18] | Laakso MP , Frisoni GB , Könönen M , Mikkonen M , Beltramello A , Geroldi C , Aronen HJ ((2000) ) Hippocampus andentorhinal cortex in frontotemporal dementia and Alzheimer’sdisease: A morphometric MRI study. Biol Psychiatry 47: , 1056–1063. |
[19] | Dudas RB , German EB , Hodges JR ((2005) ) The Addenbrooke’s cognitiv examination (ACE) in the differential diagnosis of early dementias versus affective disorder. Am J Geriatr Psychiatry 13: , 218–226. |
[20] | Torralva T , Roca M , Gleichgerrcht E , Bekinschtein T , Manes F ((2009) ) A neuropsychological battery to detect specific executive and social cognitive impairments in early frontotemporal dementia. Brain 132: , 1299–1309. |
[21] | Folstein MF , Folstein SE , McHugh PR ((1975) ) Mini-mental state: A practical method for grading the cognitive state of patients for the clinician. J Psychiatr Res 12: , 189–198. |
[22] | Rascovsky K , Hodges JR , Knopman D , Mendez MF , Kramer JH , Neuhaus J , Hillis AE ((2011) ) Sensitivity of revised diagnostic criteria for the behavioural variant of frontotemporal dementia. Brain 134: , 2456–2477. |
[23] | Gorno-Tempini ML , Hillis AE , Weintraub S , Kertesz A , Mendez M , Cappa SEEA , Manes F ((2011) ) Classification of primary progressive aphasia and its variants. Neurology 76: , 1006–1014. |
[24] | Warren JD , Jonathan DR , Martin NR ((2013) ) Frontotemporal dementia. BMJ 347: , f4827. |
[25] | Kessels RP , Van Zandvoort MJ , Postma A , Kappelle LJ , De Haan EH ((2000) ) The Corsi block-tapping task: Standardization and normativedata. App Neuropsychol 7: , 252–258. |
[26] | Benbadis SR , Lin K ((2008) ) Errors in EEG interpretation and misdiagnosis of epilepsy. Eur Neurol 59: , 267–271. |
[27] | de Curtis M , Jefferys JG , Avoli M ((2012) ) Interictal epileptiform discharges in partial epilepsy: Complex neurobiological mechanisms based on experimental and clinical evidence. In Jasper’s Basic Mechanisms of Epilepsies, 4th edition, Noebels JL, Avoli M, Rogawski MA, Olsen RW, Delgado-Escueta AV, eds. National Center for Biotechnology Information (US), Bethesda, MD. |
[28] | Belcastro V , Costa C , Galletti F , Pisani F , Calabresi P , Parnetti L ((2007) ) Levetiracetam monotherapy in Alzheimer patients with late-onset seizures: A prospective observational study. Eur J Neurol 14: , 1176–1178. |
[29] | Cumbo E , Ligori LD ((2010) ) Levetiracetam, lamotrigine, and phenobarbital in patients with epileptic seizures and Alzheimer’s disease. Epilepsy Behav 17: , 461–466. |
[30] | Neary D , Snowden J , Mann D ((2005) ) Frontotemporal dementia. Lancet Neurol 4: , 771–780. |
[31] | Treiman DM , Walker M ((2006) ) Treatment of seizure emergencies: Convulsive and non-convulsive status epilepticus. Epilepsy Res 68: , 77–82. |
[32] | NICE-SCIE, Dementia ((2006) ) Supporting People with Dementia and their Careers in Health and Social Care (NICE Clinical Guideline 42). London: National Institute for Health and Clinical Excellence and Social Care Institute for Excellence. |
[33] | Smith S ((2005) ) EEG in the diagnosis, classification, and management of patients with epilepsy. J Neurol Neurosurg Psychiatry 76: (Suppl 2), ii2–ii7. |
[34] | Nilsson D , Fohlen M , Jalin C , Dorfmuller G , Bulteau C , Delalande O ((2009) ) Foramen ovale electrodes in the preoperative evaluation of temporal lobe epilepsy in children. Epilepsia 50: , 2085–2096. |
[35] | Horvath A , Szucs A , Barcs G , Kamondi A ((2016) ) The value of long-term EEG in the diagnosis of epilepsy in Alzheimer’s disease. J Neurol Stroke 4: , 125–131. |
[36] | Vossel KA , Beagle AJ , Rabinovici GD , Shu H , Lee SE , Naasan G , Seeley WW ((2013) ) Seizures and epileptiform activity in the early stages of Alzheimer disease. JAMA Neurol 70: , 1158–1166. |
[37] | Wieser HG , Elger CE , Stodieck SRG ((1985) ) The ‘foramen ovale electrode’: A new recording method for the preoperative evaluation of patients suffering from mesio-basal temporal lobe epilepsy. Electroencephalogr Clin Neurophysiol 61: , 314–322. |
[38] | Peris-Celda M , Graziano F , Russo V , Mericle RA , Ulm AJ ((2013) ) Foramen ovale puncture, lesioning accuracy, and avoiding complications: Microsurgical anatomy study with clinical implications: Laboratory investigation. J Neurosurg 119: , 1176–1193. |
[39] | Fernandez Torre JL , Alarcon G , Binnie CD , Polkey CE ((1999) ) Comparison of sphenoidal, foramen ovale and anterior temporal placements for detecting interictal epileptiform discharges in presurgical assessment for temporal lobe epilepsy. Clin Neurophysiol 110: , 895. |
[40] | Clemens Z , Janszky J , Szűcs A , Békésy M , Clemens B , Halász P ((2003) ) Interictal epileptic spiking during sleep and wakefulness in mesial temporal lobe epilepsy: A comparative study of scalp and foramen ovale electrodes. Epilepsia 44: , 186–192. |
[41] | Sheth SA , Aronson JP , Shafi MM , Phillips H , Velez-Ruiz N , Walcott BP , Eskandar EN ((2014) ) Utility of foramen ovale electrodes in mesial temporal lobe epilepsy. Epilepsia 55: , 713–724. |
[42] | Liu XY , Shi T , Yin WN , Ren ZY , Deng YL , Chen SD ((2016) ) Interictal epileptiform discharges were associated with poorer cognitive performance in adult epileptic patients. Epilepsy Res 128: , 1–5. |
[43] | Oka Y ((2013) ) Sleep problems in dementia. Rinshoshinkeigaku= Clinical Neurol 54: , 994–996. |
[44] | McCarter SJ , Louis EKS , Boeve BF ((2016) ) Sleep disturbances in frontotemporal dementia. Curr Neurol Neurosci Rep 16: , 85–93. |
[45] | Maestri M , Carnicelli L , Economou NT , Bonakis A , Paparrigopoulos T , Papageorgiou ST , Bonuccelli U ((2015) ) NREM sleep transient events in fronto-temporal dementia: Beyond sleep stage architecture. Arch Ital Biol 153: , 226–236. |
[46] | Bonakis A , Economou NT , Paparrigopoulos T , Bonanni E , Maestri M , Carnicelli L , Papageorgiou SG ((2014) ) Sleep in frontotemporal dementia is equally or possibly more disrupted, and at an earlier stage, when compared to sleep in Alzheimer’s disease. J Alzheimers Dis 38: , 85–91. |
[47] | Naylor E , Penev PD , Orbeta L , Janssen I , Ortiz R , Colecchia EF , Zee PC ((2000) ) Daily social and physical activity increases slow-wave sleep and daytime neuropsychological performance in the elderly. Sleep 23: , 1–9. |
[48] | James SP , Mendelson WB ((2004) ) The use of trazodone as a hypnotic: A critical review. J Clin Psychiatry 65: , 752–755. |
[49] | Cipriani G , Lucetti C , Danti S , Nuti A ((2015) ) Sleep disturbances and dementia. Psychogeriatrics 15: , 65–74. |