Effect of soft and semi-rigid ankle braces on kinematic and kinetic changes of the knee and ankle joints after forward and lateral drop landing in healthy young women
Abstract
BACKGROUND:
Ankle braces are commonly used to protect ankle joints from a sprain by restricting inversion. However, the difference between a soft brace (SB) and a semi-rigid brace (SRB) regarding kinematic and kinetic changes of the lower limb joints after forward and lateral drop landing is unknown.
OBJECTIVE:
The aim of this study was to evaluate the effect of SB and SRB, on kinematic and kinetic changes after each drop landing in healthy young women.
METHODS:
Ten female adults were assessed for one leg while wearing SB, SRB or non-brace (NB). For assessing kinematic and kinetic changes after drop landing, the participant jumped and landed forward and laterally with one leg on a force platform. Knee and ankle joint angle and moment, peak ground reaction force (pGRF), time to peak GRF (TpGRF), the rate of force development (RFD) and GRF impulse (impulse) were measured.
RESULTS:
The results indicated that knee flexion angle, TpGRF, RFD, and impulse were significantly different between SRB and NB after forward drop landing. SRB demonstrated significant increases in RFD and decreases in impulse.
CONCLUSIONS:
Semi rigid brace may be beneficial in providing more restriction to the ankle joint for preventing ankle sprains during landing.
1.Introduction
The incidence rate of ankle sprains is high in athletes among junior and collegiate athletes or even nonathletes (47–60%) [9, 14, 19] and 74% of people who experienced ankle sprains develop chronic ankle instability (CAI) [2]. Valderrabano et al. [20] indicated that chronic ankle stability develops new sprains and injuries and suggest the possibility of developing osteoarthritis. Besides, a previous study reported that the level of physical activity decreases and may have a long-term adverse effect on the health condition of a person with CAI [10].
Ankle braces are mainly designed to limit the movement of the ankle joint in the frontal plane and are used to prevent ankle sprain. They are expected to reduce anterior tibial shear force and range of motion of the ankle and subtalar joints while improving the proprioception of the ankle by promoting mechanoreception and maintaining dynamic balance ability [7]. A number of studies have investigated the effect of ankle braces on postural control. Wikstrom et al. [23] and Hardy et al. [7] reported the positive effects of ankle braces on static and dynamic postural control.
The effects of Semi-Rigid Brace (SRB) and the Soft Brace (SB) vs. ‘no-brace’ (NB) on static and dynamic balance may be of importance to physical therapists, trainers and athletes. However, to the best of our knowledge, the effects of SB and SRB on kinematic and kinetic changes in the knee and ankle joint after forward and lateral drop landing have not been investigated in healthy young women whose incidence of ankle sprains is significantly higher than that of their male counterparts [6, 24].
Therefore, the aim of the present study was to clarify the effect of SB and SRB on kinematic and kinetic changes after forward and lateral drop landing in healthy young women.
2.Methods
2.1Participants
Ten healthy subjects including 15 women [age 21.8
Figure 1.
Soft brace (ZAMST FA-1; SIGMAX Devices, Inc., Japan).
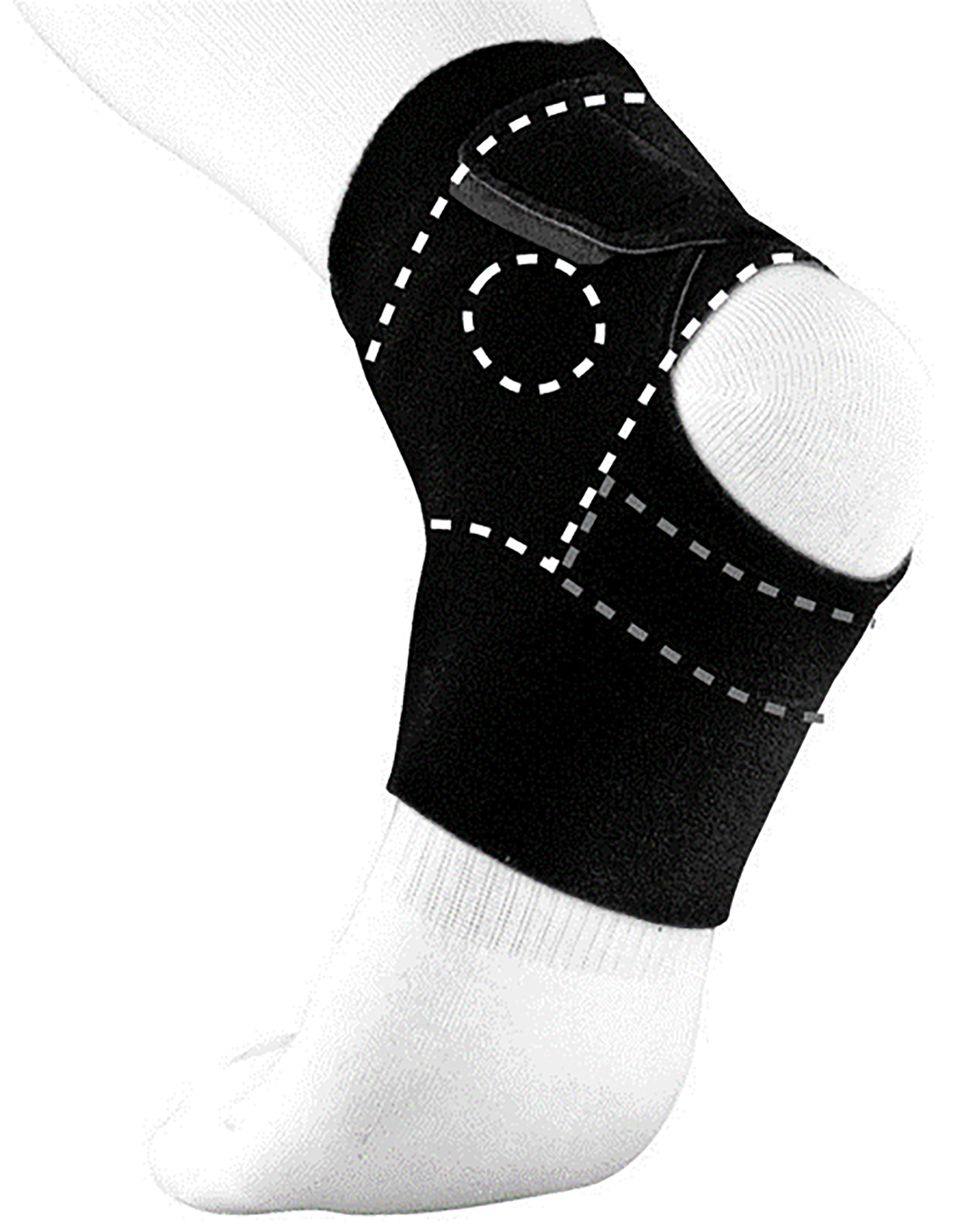
Figure 2.
Semi-rigid brace (ZAMST FA-1; SIGMAX Devices, Inc., Japan).
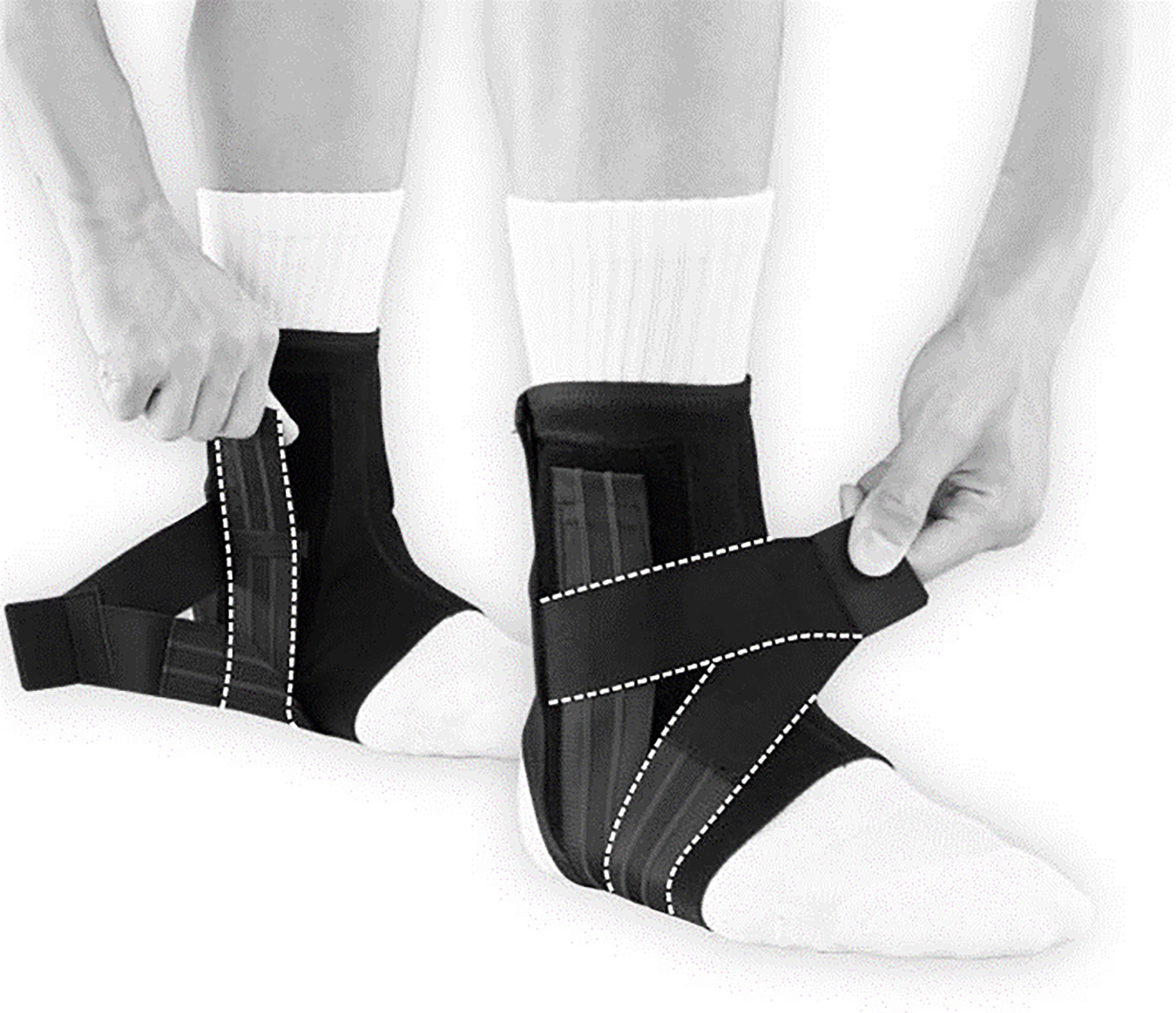
2.2Intervention
Subjects were assigned to three randomly ordered experimental conditions (NB, SB, SRB). Zamst ankle braces (Nippon Sigmax Devices, Inc., Tokyo, Japan) were used in this study. The SB (Zamst FA-1) was a nylon supporter and was designed with two layers of support for weak and swollen ankles while allowing dorsiflexion-plantar flexion and inversion-eversion (Fig. 1). An inner wrap adjusts with a hook-and-loop closure to provide compression and control of the ankle and heel area. The SRB (Zamst A1) was a nylon supporter and included mediolateral reinforcement and two straps. It is designed to resist inversion loads while allowing dorsiflexion-plantar flexion and stabilize the ankle joint (Fig. 2). The mechanical property (i.e., angle-moment relationship) of the SB and SRB in the sagittal and coronal planes was quantified using a custom motorized mechanical testing device with an inline torque sensor (Transducer Tech Inc., USA) and optical encoder [16]. The ankle brace was donned on a wooden surrogate leg with mechanical ankle and subtalar joints. The mandrel extending from the surrogate leg was attached to a mounting jig which allowed precise positioning and the mechanical joint center was aligned with the center of motor output shaft. The servo motor was controlled by a motor drive in speed mode. Data acquisition (i.e. torque and joint displacement) and motor control was done using a custom LabVIEW program interfaced with a National Instrument PCI card (National Instrument Inc., TX, USA). The braces were fit to each subject by a single investigator in order to minimize within-subject and between-subject variations. The participants performed under three brace conditions (SRB, SB, and NB) in a random order on three separate days, with an intersession interval of at least 24 hours and no more than 48 hours between tests.
2.3Test protocol
To compare the effect of each condition, we measured kinematic and kinetic changes after forward and lateral drop landing under SB, SRB and NB conditions. The participants were instructed to perform the following actions: landing in the forward and lateral (90
2.4Data collection
Kinematic data were recorded by 3-dimensional motion capture systems Vicon (Vicon motion systems, UK) with 16 cameras at a sampling rate of 100 Hz. A force plate (AMTI, USA) was used to measure the ground reaction force as kinetic data at a sampling rate of 1000 Hz. The instance of contacts on the force plate were defined as when the vertical ground reaction force was above 10 N [16]. Infrared reflective markers were applied to the subject according to the Plug-in Gait lower limb model. Marker data were post processed using Vicon Nexus 1.8.5 (Vicon Motion Systems, UK). Each subject had 16 reflective surface markers taped to specific anatomical landmarks on the pelvis, thigh, shank and shoe using hypoallergenic tape. Markers were placed on the right and left anterior superior iliac spine and posterior superior iliac spine, right and left lateral epicondyle of the knee, over the lower lateral 1/3 surface of the right and left thigh, right and left lateral malleolus along an imaginary line that passes through the transmalleolar axis, over the lower lateral 1/3 surface of the right and left shank (to determine the alignment of the ankle flexion axis), right and left over the second metatarsal head, and placed on the calcaneus at the same height above the plantar surface of the foot as the toe maker [21]. The global reference system of the laboratory was established so the anteroposterior axis was in the y-axis direction, the mediolateral axis was the x-axis, and the vertical axis was the z-axis.
Figure 3.
Angle-moment relationship of the SB and SRB in the sagittal and coronal plane.
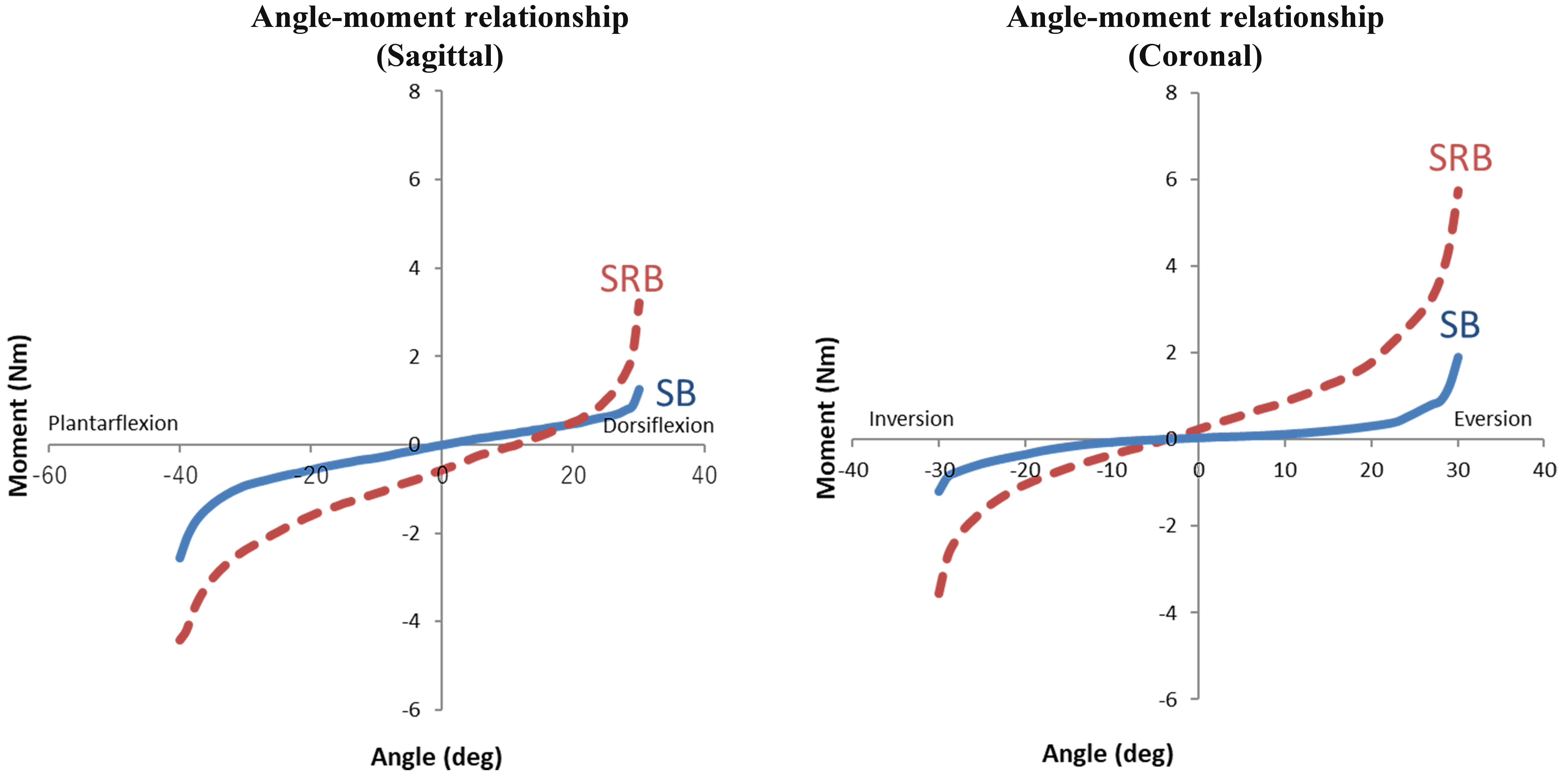
The data of knee and ankle joints angle, ground reaction force (GRF), moment and GRF impulse (impulse) were filtered using a zero-lag, fourth-order, low-pass Butterworth filter with a cutoff frequency of 20 Hz. Angular displacements, force and moment of the knee and ankle joints were analyzed as each movement from initial contact to peak angle. Ankle and knee joint kinematic and kinetic data were averaged over three trials for each subject. Angle displacement (
2.5Statistical analysis
A repeated-measures 1 (time)
3.Results
3.1Angle-moment relationship of SB and SRB
The angle-moment relationship of the SB and SRB in the sagittal and coronal planes is shown in Fig. 3. In both planes, SRB demonstrated more resistance than SB throughout the range.
3.2Kinematic and kinetic data
Knee flexion and valgus angle, ankle dorsiflexion and inversion angle in each brace condition and direction of drop landing are shown in Table 1. KFM, KVM, APM and AIM are shown in Table 2. pGRF, pGRF, RFD, and impulse are shown in Table 3.
Table 1
Values for knee flexion and valgus, ankle dorsiflexion and inversion angle according to ankle brace condition in each direction
Variable | Condition | Effect size | Observed power | |||
---|---|---|---|---|---|---|
NB | SB | SRB | ||||
Knee flexion angle (KF) ( | ||||||
Forward | 48.1 | 46.6 | 51.1 | NB | 0.19 | 0.28 |
Lateral | 48.1 | 51.3 | 50.3 | NB | 0.33 | 0.58 |
Knee valgus angle (KV) ( | ||||||
Forward | 5.38 | 6.11 | 6.66 | n.s. | 0.07 | 0.13 |
Lateral | 5.05 | 6.58 | 7.41 | n.s. | 0.20 | 0.37 |
Ankle dorsiflexion angle (AD) ( | ||||||
Forward | 26.2 | 26.3 | 24.3 | n.s. | 0.16 | 0.30 |
Lateral | 28.2 | 28.8 | 24.6 | SB | 0.38 | 0.68 |
Ankle inversion angle (AI) ( | ||||||
Forward | 4.42 | 4.31 | 3.67 | n.s. | 0.10 | 0.18 |
Lateral | 3.49 | 3.82 | 1.26 | n.s. | 0.26 | 0.37 |
Note. *Effect size was calculated using the formula
Table 2
Values for ankle dorsiflexor and inversion moment according to ankle brace condition in each direction
Variable | Condition | Effect size | Observed power | |||
---|---|---|---|---|---|---|
NB | SB | SRB | ||||
Knee flexor moment (KFM) (N | ||||||
Forward | 1.85 | 2.13 | 2.25 | n.s. | 0.21 | 0.30 |
Lateral | 1.67 | 1.73 | 1.87 | n.s. | 0.08 | 0.15 |
Knee valgus moment (KVM) (N | ||||||
Forward | 1.34 | 1.30 | 1.26 | n.s. | 0.01 | 0.06 |
Lateral | 1.19 | 1.22 | 1.12 | n.s. | 0.12 | 0.22 |
Ankle plantar flexor moment (APM) (N | ||||||
Forward | 2.35 | 2.42 | 2.23 | n.s. | 0.10 | 0.17 |
Lateral | 2.55 | 2.63 | 2.25 | NB, SB | 0.31 | 0.56 |
Ankle inversion moment (AIM) (N | ||||||
Forward | 0.80 | 0.07 | 0.07 | n.s. | 0.15 | 0.28 |
Lateral | 0.23 | 0.22 | 0.23 | n.s. | 0.02 | 0.07 |
Note. *Effect size was calculated using the formula
Table 3
Values for pGRF, TpGRF, RFD, impulse according to ankle brace condition in each direction
Variable | Condition | Effect size | Observed power | |||
---|---|---|---|---|---|---|
NB | SB | SRB | ||||
Peak vertical GRF (pGRF) (N/kg) | ||||||
Forward | 32.3 | 31.8 | 32.0 | n.s. | 0.03 | 0.08 |
Lateral | 30.7 | 31.6 | 31.2 | n.s. | 0.12 | 0.22 |
Time to peak vertical GRF (TpGRF) (s) | ||||||
Forward | 69.5 | 66.4 | 63.4 | NB | 0.30 | 0.52 |
Lateral | 84.6 | 82.3 | 79.3 | NB | 0.42 | 0.41 |
The rate of force development (RFD) (N/s) | ||||||
Forward | 0.52 | 0.60 | 0.60 | NB | 0.41 | 0.40 |
Lateral | 0.39 | 0.44 | 0.45 | NB | 0.43 | 0.43 |
Impulse (N/kg | ||||||
Forward | 1.08 | 0.99 | 0.93 | NB, SB | 0.34 | 0.55 |
Lateral | 1.27 | 1.23 | 1.18 | n.s. | 0.16 | 0.28 |
Note. *Effect size was calculated using the formula
The results indicate that knee flexion angle (
4.Discussion
The aim of this study was to clarify the effects of the SB, SRB, and NB interventions on kinematics and kinetics of the lower limb joints after forward and lateral drop landing in healthy young women. The mechanical testing of the SB and SRB demonstrated that SRB provide more resistance than SB throughout the range in the sagittal and coronal planes. This outcome suggests that SRB provided more restriction to the ankle joints than SB during the tests. Knee flexion angle after forward landing with SRB was significantly larger compared with NB, and ankle dorsiflexion angle after lateral landing was significantly lower compared with SB and demonstrated a tendency for decreasing compared with NB. Venesky et al. [22] have indicated that a restriction of the ankle movement could possibly increase the loading at the knee joint. Simpson et al. [18] reported that this mechanical restriction by ankle brace was expected to directly influence the ankle joint kinematics and indirectly influence the knee joint kinematics.
In this study, knee and ankle moments after forward landing showed no significant difference among the three conditions. However, ankle plantar flexor moment after lateral landing was significantly lower with SRB compared with SB and NB. Therefore, due to its higher rigidity SRB restricted the ankle range of motion better than SB, but there is a possibility of increasing the flexion angle of the knee as a compensatory strategy and decreasing ankle plantar moment in drop landing.
The TpGRF was significantly shorter while the RFD was significantly higher with the SRB than with the NB after forward and lateral drop landing. Bates et al. [3] reported that shorter TpGRF, rapid impulse load, and increased RFD may contribute to increasing the loading within a joint of lower extremity. SRB demonstrated significant decreases in impulse compared to SB and NB after drop landing. Evaluating impulse based on the relationship of the impulse and momentum clarifies the ability of the performer to change the momentum of the center of mass [15], considering that change in momentum is equal to impulse as showed by impulse-momentum theorem. Nordin et al. [12, 13] reported that GRF impulse indicates the effects of both the pre-contact strategy and the response to ground contact. Majumdar et al. [11] also reported that the increases in impulse indicates that the displacement of the center of gravity of the body is increasing which might lead to higher risk of injury. Based on these reports, although SRB may decrease displacement of the center of gravity of landing compared with SB and NB, it is suggested that SRB may increase the load on ankle and knee joint. However, it is more likely that factors other than structural support play an important role to restrict appropriate range of motion of ankle joint after landing. Castro et al. [4] reported similar results regarding the loading of impact forces in basketball players. These measurements will be made in a future study. Increased joint loading in the lower extremity, following landing, may be associated with the presence of sports injuries and trauma to the lower extremities [17].
Some limitations in the study need to be considered. First, we analyzed a relatively small sample size and only healthy women. An increased number of subjects of both sexes will allow us to perform an inter-gender comparison. Second, the implication/s of this study in the clinical setting, particularly with respect to chronic ankle instability could not be assessed. Third, this study only investigated the acute effect of ankle braces but the long-term effects of the braces must be addressed. Lastly, we did not investigate the change in muscle activation for each condition. For example, with co-activation of agonist and antagonist muscles, it will be hard to tease out their individual contribution toward resultant joint moment and it is not surprising that joint reaction force might be underestimated without taking into account the muscle force.
5.Conclusions
We demonstrated that impulse of SRB after forward drop landing was significantly lower with the SRB than with the SB and NB after forward landing while TpGRF was lower and RFD was higher with the SRB than with the NB after forward and lateral landing. These findings indicate that the SRB may increase the loading within joints of the lower extremity in healthy young women. In addition, SRB restricted the range of motion of the ankle joint more than the SB or NB. These findings suggest that SRB may be beneficial in providing more restriction to the ankle joint protecting this joint against sprains occurring during forward and lateral landing. However, these positive effects may come at the expense of secondary disorders such as fatigue and pain in the knee, hip and lumbar region. Athletic trainers, physical therapists, and athletes should be aware of these implications when making a decision regarding the type of bracing, if, and when, applied.
Conflict of interest
None to report.
References
[1] | Abdel-Rahman IA, Mohamed MD. Biomechanical indicators of jump height among varied techniques of vertical jump. Am J Sports Sci (2016) ; 4: (5): 77-83. |
[2] | Anandacoomarasamy A, Barnsley L. Long term outcomes of inversion ankle injuries. Br J Sports Med (2005) ; 39: (3): e14. |
[3] | Bates NA, Ford KR, Myer GD, Hewett TE. Timing differences in the generation of ground reaction forces between the initial and secondary landing phases of the drop vertical jump. Clin Biomech (2013) ; 28: (7): 796-799. |
[4] | Castro A, Goethel MF, Gáspari AF, Crozara LF, Gonçalves M. Ankle brace attenuates the medial-lateral ground reaction force during basketball rebound jump. Rev Bras Med Esporte (2017) ; 23: (3): 232-236. |
[5] | Decker MJ, Torry MR, Noonan TJ, Riviere A, Sterett WI. Landing adaptations after ACL reconstruction. Med Sci Sports Exerc (2002) ; 34: (9): 1408-1413. |
[6] | Doherty C, Delahunt E, Caulfield B, Hertel J, Ryan J, Bleakley C. The incidence and prevalence of ankle sprain injury: A systematic review and meta-analysis of prospective epidemiological studies. Sports Med (2014) ; 44: (1): 123-140. |
[7] | Hardy L, Huxel K, Brucker J, Nesser T. Prophylactic ankle braces and star excursion balance measures in healthy volunteers. J Athl Train (2008) ; 43: (4): 347-351. |
[8] | Hicks KM, Onambélé GL, Winwood K, Morse CI. Muscle damage following maximal eccentric knee extensions in males and females. PLoS One (2016) ; 11: (3): e0150848. |
[9] | Hiller CE, Nightingale EJ, Raymond J, Kilbreath SL, Burns J, Black DA, Refshauge KM. Prevalence and impact of chronic musculoskeletal ankle disorders in the community. Arch Phys Med Rehabil (2012) ; 93: (10): 1801-1807. |
[10] | Hubbard-Turner T, Turner MJ. Physical activity levels in college students with chronic ankle instability. J Athl Train (2015) ; 50: (7): 742-747. |
[11] | Majumdar D, Pal MS, Pramanik A, Majumdar D. Kinetic changes in gait during low magnitude military load carriage. Ergonomics (2013) ; 56: (12): 1917-1927. |
[12] | Nordin AD, Dufek JS, James CR, Bates BT. Classifying performer strategies in drop landing activities. J Sports Sci Med (2016) ; 35: (8): 1858-1863. |
[13] | Nordin AD, Dufek JS. Neuromechanical synergies in single-leg landing reveal changes in movement control. Hum Mov Sci (2016) ; 49: : 66-78. |
[14] | Pasanen K, Ekola T, Vasankari T, Kannus P, Heinonen A, Kujala UM, Parkkari J. High ankle injury rate in adolescent basketball: A 3-year prospective follow-up study. Scand J Med Sci Sports (2017) ; 27: (6): 643-649. |
[15] | Robertson G, Caldwell G, Hamill J, Kamen G, Whittlesey S. Research Methods in Biomechanics. 2nd edition. Champaign: Human Kinetics; (2014) . |
[16] | Segura A, Piazza SJ. Mechanics of ambulation with standard and spring-loaded crutches. Arch Phys Med Rehabil (2007) ; 88: (9): 1159-1163. |
[17] | Sell TC, Pederson JJ, Abt JP, Nagai T, Deluzio J, Wirt MD, Lephart SM. The addition of body armor diminishes dynamic postural stability in military soldiers. Mil Med (2013) ; 178: (1): 76-81. |
[18] | Simpson KJ, Yom JP, Fu YC, Arnett SW, O’Rourke S, Brown CN. Does wearing a prophylactic ankle brace during drop landings affect lower extremity kinematics and ground reaction forces? J Appl Biomech (2013) ; 29: (2): 205-213. |
[19] | Tanen L, Docherty C, Van Der Pol B, Simon J, Schrader J. Prevalence of chornic ankle instability in high school and division I athletes. Foot Ankle Spec (2013) ; 7: (1): 37-44. |
[20] | Valderrabano V, Hintermann B, Horisberger M, Fung TS. Ligamentous posttraumatic ankle osteoarthritis. Am J Sports Sci (2016) ; 34: (4): 612-620. |
[21] | Vanwanseele B, Stuelcken M, Greene A, Smith R. The effect of external ankle support on knee and ankle joint movement and loading in netball players. J Sci Med Sport (2014) ; 17: (5): 511-515. |
[22] | Venesky K, Docherty CL, Dapena J, Schrader J. Prophylactic ankle braces and knee varus-valgus and internal-external rotation torque. J Athl Train (2006) ; 41: (3): 239-244. |
[23] | Wikstrom EA, Arrigenna MA, Tillman MD, Borsa PA. Dynamic postural stability in subjects with braced, functionally unstable ankles. J Athl Train (2006) ; 41: (3): 245-250. |
[24] | Zahradnik D, Jandacka D, Uchytil J, Farana R, Hamill J. Lower extremity mechanics during landing after a volleyball block as a risk factor for anterior cruciate ligament injury. Phys Ther Sport (2015) ; 16: (1): 53-58. |