Aquaporin-8 is a novel marker for progression of human cervical cancer cells
Abstract
BACKGROUND:
Role of aquaporin-8 (AQP8) in cervical cancer has not been fully elucidated.
OBJECTIVE:
We aim to explore the impacts of AQP8 on viability, apoptosis and metastasis in cervical cancer cells.
METHODS:
AQP8 protein expression in cervical carcinoma specimens and cell lines was detected by IHC and western blot analysis. Lentivirus-mediated transfection was used to upregulate and knockdown AQP8 in cells. Cell viability and apoptosis were assessed by CCK-8 and flow cytometry assays, respectively. Transwell experiments were conducted to investigate cell invasive and migratory capabilities. EMT-related markers were detected by western blot analysis.
RESULTS:
A strong positive of AQP8 protein expression was observed in cervical cancer tissues. Western blot analysis confirmed overexpression and knockdown of AQP8 in SiHa cells. AQP8-overexpressed SiHa cells displayed an enhanced viability, reduced apoptotic rate, increased invasive and migratory abilities. Knockdown of AQP8 inhibited the viability, promoted the apoptosis, and suppressed invasion and migration. Furthermore, AQP8 overexpression significantly upregulated vimentin and N-cadherin, and downregulated E-cadherin, which were reversed by AQP8 knockdown.
CONCLUSIONS:
AQP8 increases viability, inhibits apoptosis, and facilitates metastasis in SiHa cells. This may be associated with EMT-related markers regulated by AQP8. AQP8 could serve as a potential marker for cervical cancer progression.
1.Introduction
Aquaporins (AQPs) are a family of membrane channels that facilitate transportation of water molecules and small solutes under an osmotic gradient [1, 2]. Notably, accumulated evidences have shown that AQPs exist in numerous types of human cancer cells and are closely implicated into carcinogenesis [3, 4, 5]. AQP8 is one of the AQPs members and participates only in water metabolism [6]. Despite the recognized pathologic role of AQP8 in inflammatory diseases [7, 8], its function in carcinogenesis remains rare and controversial. Intriguingly, AQP8 is reported to serve as oncoprotein in human esophageal cancer [9] but as a suppressor in colorectal cancer [10]. Our team has previously demonstrated that AQP8 was gradually upregulated in cervix as the disease progression [11]. Nonetheless, the exact role of AQP8 in cervical tumorigenesis has not been fully elucidated up to date.
Epithelial cells often lose their polarized characteristics and gain mesenchymal features through a remodeling process, named EMT [12]. This process is a fundamental event and intimately involved in various aspects of development, including embryogenesis, wound healing, as well as cancer progression [13, 14]. During EMT, cancer cells display increased migratory and invasive phenotypes, which become a major cause of metastasis and relapse [15]. Studies have also reported the onset of EMT often leads to increased metastatic properties and drug resistance in cervical cancer [16]. However, there is a scarcity in studies that report AQPs regulating EMT process in human cancers so far.
In this study, AQP8 expression was detected in carcinoma tissues of cervix and different cell lines. Moreover, the function of AQP8 on the viability, apoptosis, and metastasis of cervical cancer cells was explored. Finally, whether AQP8 could regulate EMT process in cervical cancer cells was also investigated in this study.
2.Materials and methods
2.1Clinical samples and immunohistochemistry
A total of 10 cervical cancer and matched normal cervix samples were gleaned from the Second Affiliated Hospital of Wenzhou Medical University from Jan 2020 to August 2020. All patients included in this study did not receive any chemoradiotherapies before surgery and provided an informed consent. The approval of this study had also been obtained from the ethical committee of the Second Affiliated Hospital of Wenzhou Medical University. The pathological classification and clinical stage were gained on the basis of FIGO.
IHC staining was performed on formalin-fixed and paraffin-embedded 4
2.2Cell lines and culture
Human cervical cancer cell lines (C33A, HeLa and SiHa) were purchased from ATCC (USA). The immortalized normal cervical epithelial cell lines H8 were donated by the Institute of Immunology, Wenzhou Medical University. All cells were cultivated in DMEM (Gibco, USA), supplemented with 10% FBS (Gibco, USA) and 1% penicillin-streptomycin solution (Invitrogen, UK). Cells were maintained at 37
2.3Lentiviral transduction
The AQP8 shRNAs and control plasmids were purchased from Shanghai Jiao Tong University School of Medicine. The AQP8 sequences were amplified via PCR and inserted into the lentiviral vector pLVX-IRES-ZsGreen1 (Origene, USA). After gene sequencing, pLVX-IRES-ZsGreen1-AQP8 and the null vector, AQP8 shRNAs and the negative plasmid, together with two packing plasmids psPAX2 and pMD2.G were transduced into 293 T cells for 48 h with the help of lipofectamine 2000 (Thermo Fisher Scientific, USA). Subsequently, SiHa cells were transfected with recombinant lentiviral particles together with polybrene (8
2.4Cell viability assay
A CCK-8 kit (Dojindo, China) was used to measure viability of SiHa cells. Approximately 3
2.5Flow cytometry analysis
The Annexin V-FITC/PI Apoptosis Detection kit (BD, USA) was used to evaluate cell apoptosis. The protocol used for analysis was previously described [17]. About 5
2.6Transwell assay
The migratory and invasive abilities of cells were assessed using the 8
2.7Western blot analysis
Cells were harvested and lysed in RIPA buffer containing protease inhibitors. After centrifugation, the supernatants were collected and determined by BCA assay for total protein concentration. Electrophoresis process was performed on SDS-PAGE with equal proteins (40
Primary antibodies used in this study included mouse anti-human AQP8 (Boster, China, 1:1000), PCNA (CST, USA, 1:2000), Bcl-2 (CST, USA, 1:1000), Bax (CST, USA, 1:1000), E-cadherin (CST, USA, 1:2000), N-cadherin (CST, USA, 1:2000),
2.8Statistical analysis
Statistical analysis was performed by GraphPad Prism 8 software (GraphPad, USA). Data distribution was analyzed by the Kolmogorov-Smirnov test before comparison. Parametric or nonparametric tests were employed according to the results. Normally distributed data were displayed as the means
3.Results
3.1AQP8 is upregulated in cervical cancer tissues and cells
To understand the expression pattern of AQP8 in cervical cancer, AQP8 protein levels were first detected in normal cervix and cervical cancer tissues by immunohistochemistry. Results showed that AQP8 staining was strong positive in cancer but negative in adjacent normal samples. The representative images are shown in Fig. 1A and AQP8 localized in the cytoplasm. Cervical cancer tissues (8.3
Figure 1.
The expression of AQP8 in human cervical cancer tissues and cell lines. A, Immunohistochemistry analysis showed a strong positive staining of AQP8 protein in cervical cancer samples but negative in adjacent normal cervix (magnification, 200
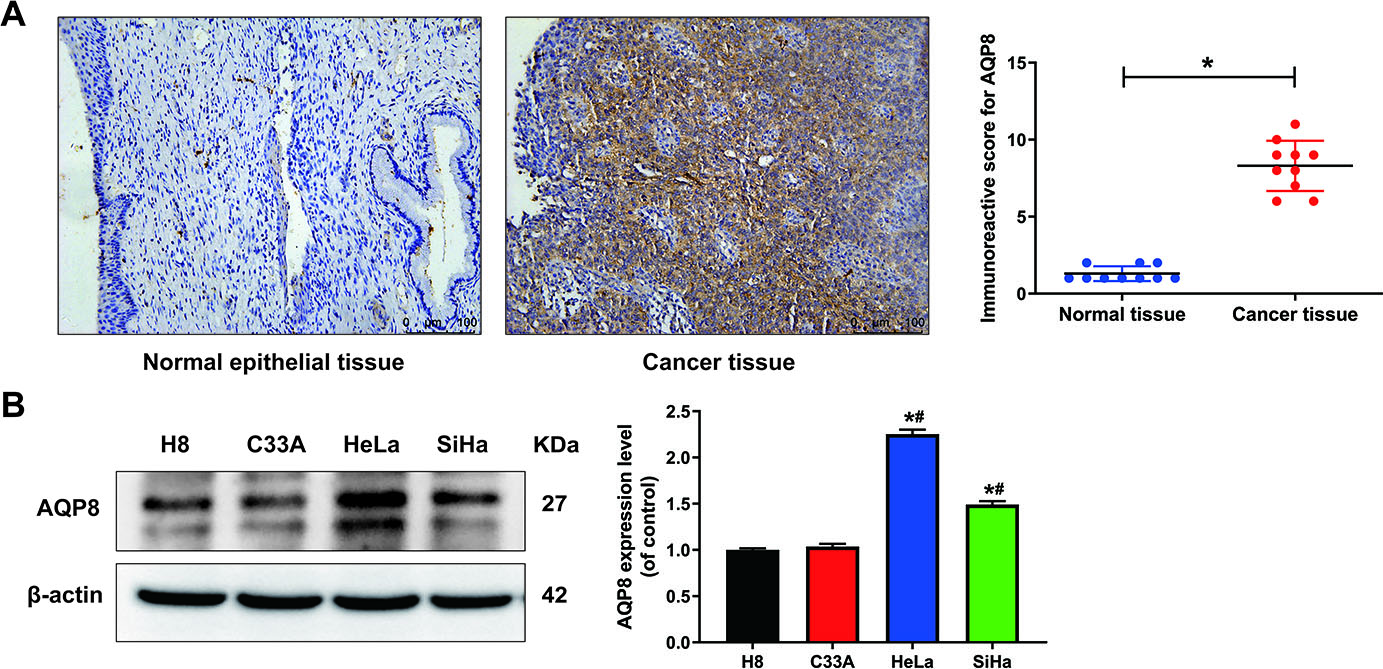
Figure 2.
AQP8-overexpression and knockdown SiHa cell lines were constructed by lentivirus transduction. A, Stably upregulated AQP8 expression in SiHa cells were identified by western blot analysis. B, Stably downregulated AQP8 expression in SiHa cells were confirmed by western blot analysis. The data shown were the ratios of the AQP8/Tubulin and the means
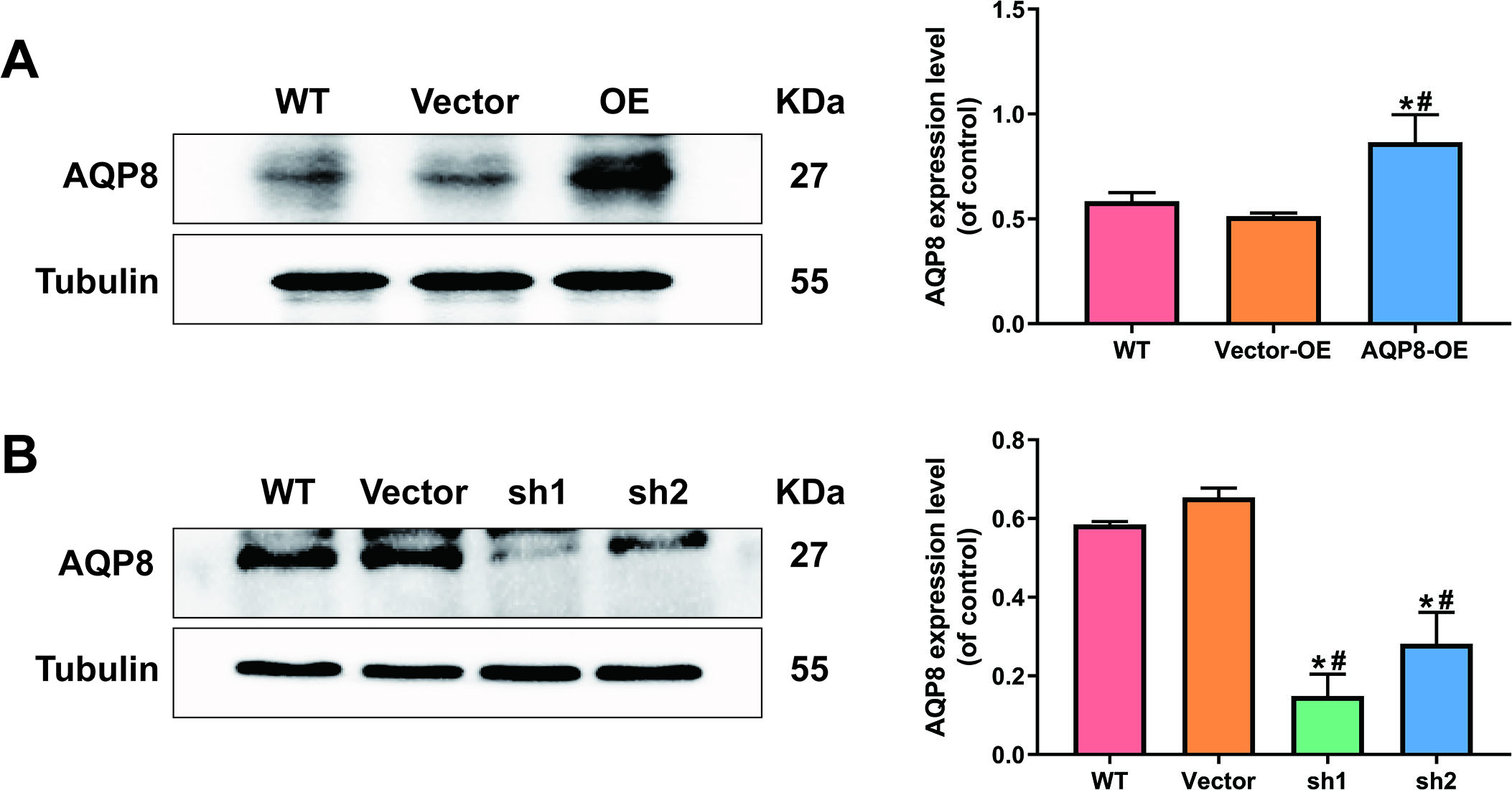
3.2AQP8 increases viability and inhibits apoptosis in cervical cancer cells
Overexpression of AQP8 in SiHa cells led to a significantly higher cell viability and lower apoptotic rate than cells with control-vector (Fig. 3A and B;
Figure 3.
AQP8 increases viability and inhibits apoptosis in SiHa cells. A and C, Cell viability of SiHa cells were determined by CCK-8 assay after AQP8 overexpression or knockdown for 0, 24, 48, 72, and 96 h. B and D, Apoptosis of SiHa cells after AQP8 overexpression or knockdown for 48 h was evaluated by flow cytometry assay. The data were shown as mean
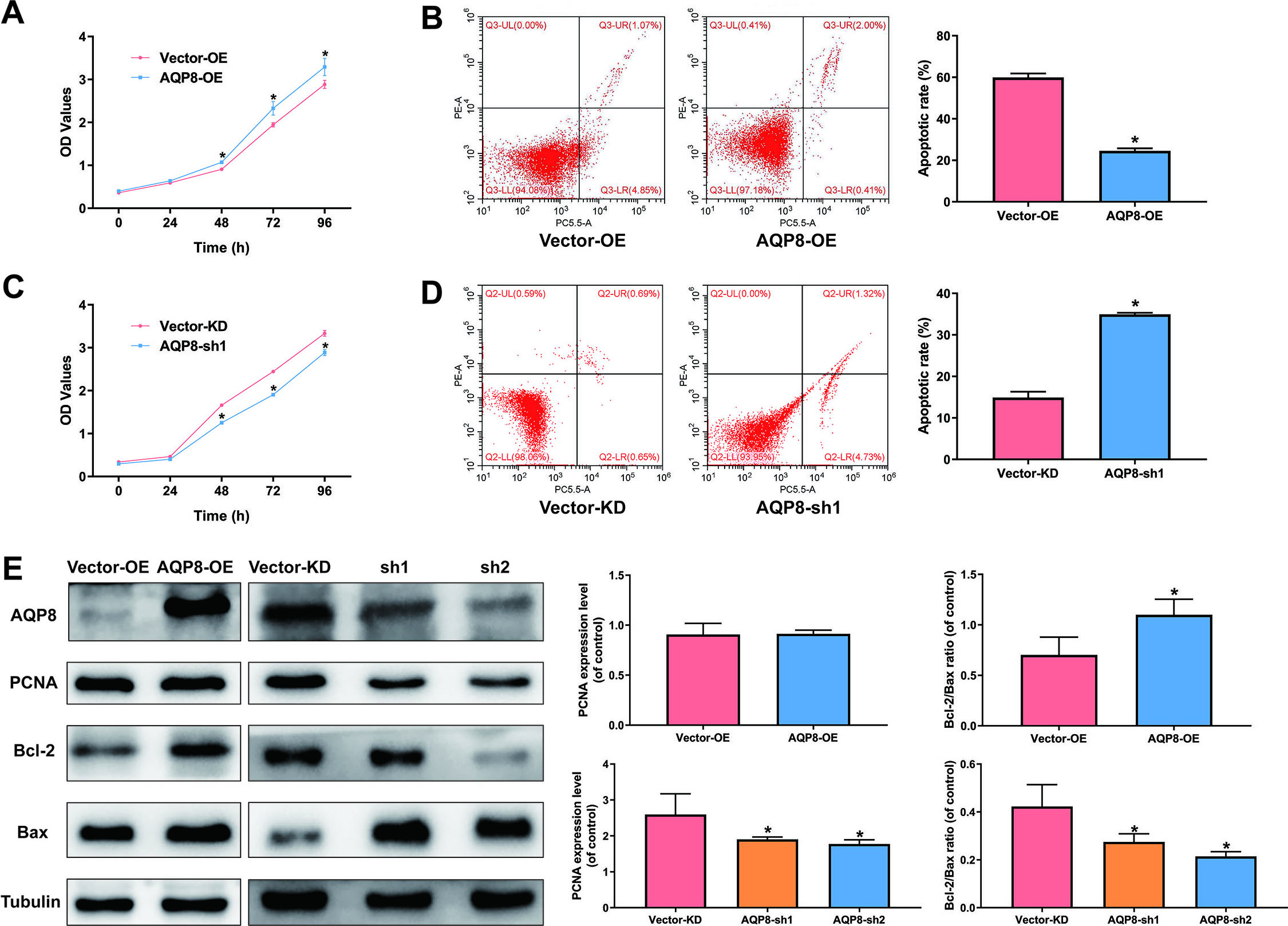
3.3AQP8 promotes invasion and migration in cervical cancer cells
Transwell assays were performed to explore the effect of AQP8 in invasion and migration of cervical cancer cells. It was obvious that the invaded and migrated cell numbers developed from SiHa cells with AQP8 overexpression were remarkably more than cells from control groups (Fig. 4A and B;
Figure 4.
AQP8 promotes invasion and migration in SiHa cells. A and B, Invaded and migrated SiHa cells with AQP8 overexpression were detected by transwell assays. C and D, Invaded and migrated SiHa cells with AQP8 knockdown were detected by transwell assays. Representative images for cell invasion and migration on SiHa cells were observed under a microscopy (magnification, 100
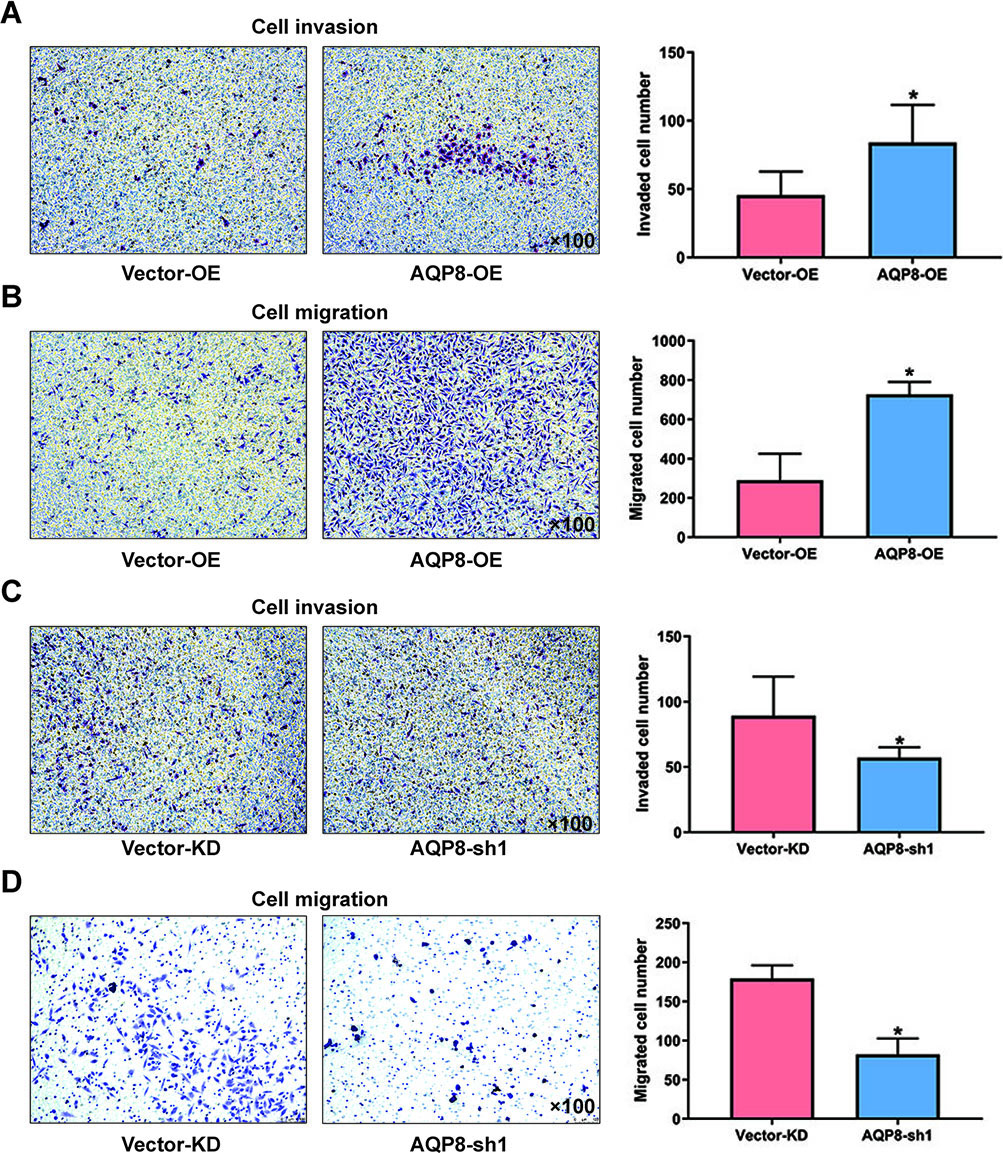
To further investigated potential mechanisms of AQP8 in regulating migration of SiHa cells, levels of migration-related proteins Ezrin and Fascin, were evaluated by western blot analysis. Nevertheless, either AQP8 overexpression or AQP8 knockdown did not alter the level of Ezrin and Fascin proteins (Fig. 5A and B;
Figure 5.
AQP8 does not alter the expression level of Ezrin and Fascin proteins in SiHa cells. A, Expression of Ezrin and Fascin in SiHa cells after AQP8 overexpression were identified by western blot analysis. B, Expression of Ezrin and Fascin in SiHa cells after AQP8 knockdown were identified by western blot analysis. The data shown were the ratios of the Ezrin/GAPDH or Fascin/GAPDH. The data were shown as the means
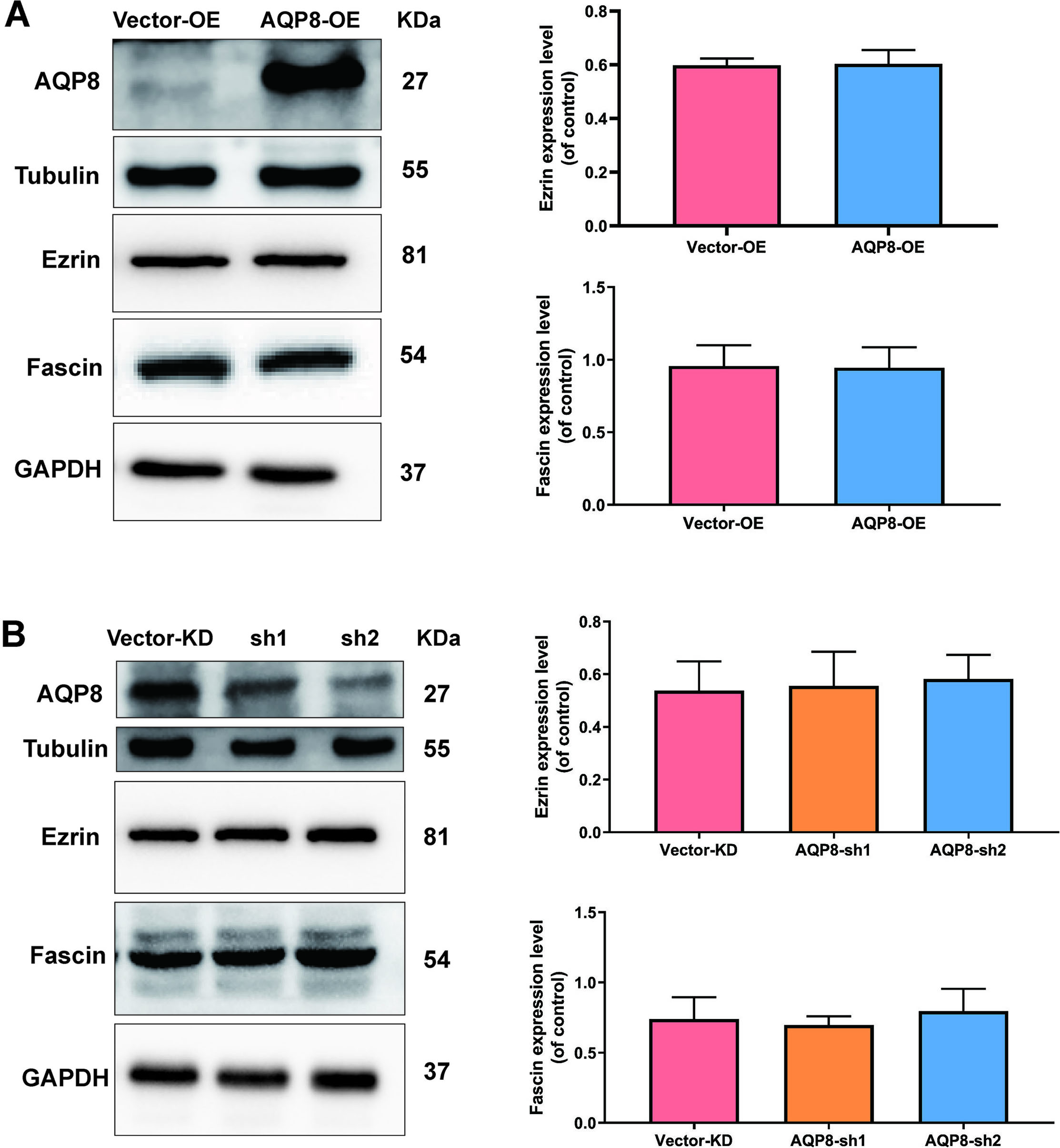
3.4AQP8 induces EMT in cervical cancer cells
To test whether the metastasis-promoting function of AQP8 in SiHa cells was due to its ability to modulate EMT process, western blot analysis was employed again to detect several classic EMT-specific markers level, including E-cadherin, N-cadherin and vimentin. Figure 6 revealed that AQP8 overexpression significantly suppressed E-cadherin expression and elevated N-cadherin and vimentin (Fig. 6A;
Figure 6.
AQP8 induces expression of EMT-related markers in SiHa cells. A, Expression of E-cadherin, N-cadherin and vimentin in SiHa cells after AQP8 overexpression were identified by western blot analysis. B, Expression of E-cadherin, N-cadherin and Vimentin in SiHa cells after AQP8 knockdown were identified by western blot analysis. The data shown were the ratios of the E-cadherin/Tubulin, N-cadherin/Vinculin, N-cadherin/GAPDH, Vimentin/Vinculin or Vimentin/GAPDH. The data were shown as the means
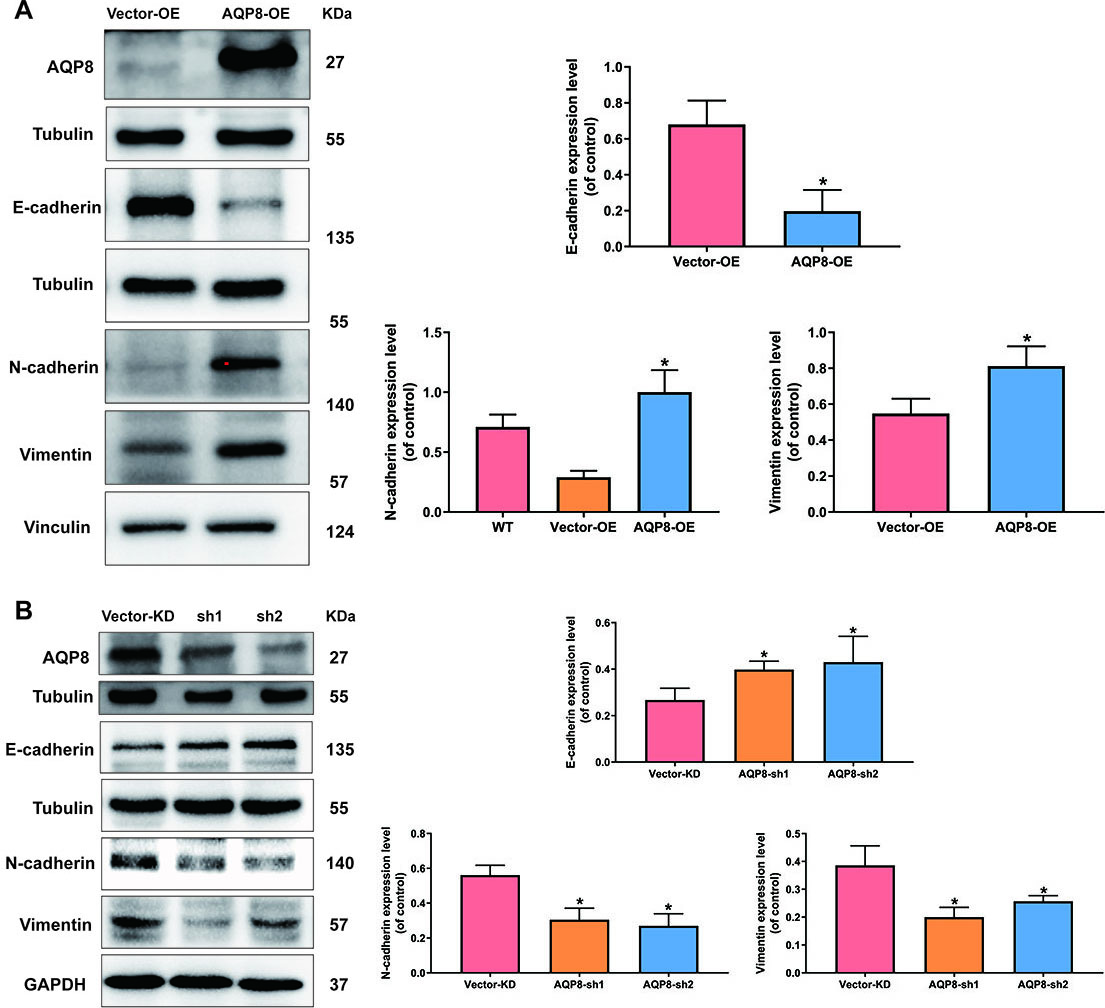
4.Discussion
Patterns of upregulation of specific classes of AQPs have been repeated for clusters of cancers [3, 18, 19]. In contrast to the common AQPs subtypes such as AQP1, AQP3, AQP5, and AQP9, AQP8 seems to serve as a tumor suppressor in human colorectal cancer [10]. However, the role of AQP8 in most other types of human cancers such as cervical cancer is poorly understood. Herein, we thoroughly investigated the function of AQP8 in viability, apoptosis, invasion, migration and EMT in cervical cancer cells.
In the current study, IHC analysis first revealed that AQP8 staining was strong positive in cervical cancer specimens but weak positive in adjacent normal cervical tissues. High expression level of AQP8 was further confirmed in different cervical cancer cell lines by western blot analysis. SiHa cells showed a moderate expression pattern of AQP8, as compared with other cell lines. Thus, SiHa cells was constructed with AQP8 overexpression and knockdown in the following experiments.
Due to various tumorigenic factors, a loss of normal cell growth at the genetic level is frequently occurred in tumor development. A growing body of evidence have indicated that AQPs play a vital role in cell proliferation by different mechanisms [20]. Our study revealed that AQP8 upregulation significantly enhanced viability and inhibited apoptosis in SiHa cells, while these effects were reversed by AQP8 knockdown. Moreover, western blot analysis confirmed the pro-growth and anti-apoptosis function of AQP8 in SiHa cells. Specifically, AQP8 upregulated the expression of proliferation-related protein PCNA and reduced the Bcl-2/Bax ratio that was closely related to anti-apoptosis. Indeed, tumor growth relies on various metabolic processes including water molecules that can be specifically transported by AQPs [4, 21]. However, it is reported that the AQPs-mediated cell growth is not completely dependent on their water permeability. In this scenario, impairment of AQP8 permeability to H
Metastasis is the most frequent cause of death for cancerous patients [24]. Studies from the last two decades have demonstrated AQPs-facilitated rapid osmolality and cell volume changes are indispensable for cancer cell migration [25, 26, 27, 28]. Specifically, this change may involve water influx into lamellipodia at the front edge of migrating cells, which is triggered by AQPs [18]. Our results confirmed the function of AQP8 in promoting invasion and migration of SiHa cells, which was impaired by AQP8 knockdown. Interestingly, western blot analysis showed no alterations in Ezrin and Fascin proteins, irrespective of AQP8 upregulation or downregulation. This finding indicated that these molecules did not contribute to AQP8-dependent SiHa cells migration.
EMT process is an embryonic program that loosens cell-cell adherence complexes and endows cells with enhanced metastatic properties [29]. Notably, EMT is critically involved in cervical cancer progression and cancer cells that undergo EMT are more aggressive and invasive [16]. Herein, levels of EMT-related central markers were detected in the present study, including E-cadherin, N-cadherin and vimentin. Results found that expression of the epithelial marker E-cadherin was significantly reduced after AQP8 overexpression and rescued by AQP8 knockdown in SiHa cells. Moreover, the two mesenchymal-specific markers N-cadherin and vimentin were upregulated when AQP8 was overexpressed and downregulated after AQP8 knockdown in SiHa cells. These results suggested that AQP8-activated EMT process was a novel hallmark that led to invasion and migration in SiHa cells. However, it is unknown whether a crosstalk exists between water/ion channel functions of AQP8 and EMT process.
5.Conclusion
In conclusion, this study found that AQP-8 may serve as a potential marker for cervical cancer progression. Furthermore, these is a need for new experiments designed specifically to address more potential mechanisms under the AQP8-mediated cervical cancer tumorigenesis.
Abbreviation
7-AAD, 7-amino-actinomycin D; ANOVA, analysis of variance; AQP8, aquaporin-8; AQP8-KD, AQP8 knockdown; AQP8-OE, AQP8 overexpression; AQPs, aquaporins; ATCC, American Type Culture Collection; BCA, bicinchoninic acid; CCK-8, cell counting kit-8; DMEM, Dulbecco’s Modified Eagle Medium; ECL, efficient chemiluminescence; EMT, epithelial-mesenchymal transition; FBS, fetal bovine serum; FIGO, International Federation of Gynecology and Obstetrics; FITC, fluorescein isothiocyanate isomer; HPV, human papilloma virus; HRP, horseradish peroxidase; IHC, immunohistochemistry; PBS, phosphate buffer saline; PE, phycoerythrin; PI, propidium iodide; PVDF, polyvinylidene fluoride; RIPA, radio-immunoprecipitation assay; SDS-PAGE, sodium dodecyl sulfate-polyacrylamide gel electrophoresis; SEM, standard error of mean; shRNA, short hairpin RNA; WT, wild type.
Author contributions
Conception: WBL, YZS, JAZ, and XQZ.
Interpretation or analysis of data: WBL, YZS, CYP, and JHY.
Preparation of the manuscript: WBL and YZS.
Revision for important intellectual content: JAZ and XQZ.
Supervision: XQZ.
Acknowledgments
We thank the Institute of Immunology, Wenzhou Medical University for donating the immortalized normal cervical epithelial cell lines H8. We also thank Prof. Luzhe Sun and his group for donating the packaging plasmids psPAX2 and pMD2.G used in this work. This study was supported by grants from Gynecology and Obstetrics of Integrated Traditional and Western Medicine of Zhejiang Province (2017-XK-A42) and Taizhou Science and Technology (20ywb02). The study sponsors had no involvement in the collection, analysis, or interpretation of data or in the writing of the manuscript.
Conflict of interest
None.
References
[1] | G.M. Preston, T.P. Carroll, W.B. Guggino and P. Agre, Appearance of water channels in Xenopus oocytes expressing red cell CHIP28 protein, Science 256: ((1992) ), 385–387. |
[2] | P. Agre, Aquaporin water channels (nobel lecture), Angew Chem Int Ed Engl 43: ((2004) ), 4278–4290. |
[3] | B. Aikman, A. de Almeida, S.M. Meier-Menches and A. Casini, Aquaporins in cancer development: Opportunities for bioinorganic chemistry to contribute novel chemical probes and therapeutic agents, Metallomics 10: ((2018) ), 696–712. |
[4] | D. Ribatti, G. Ranieri, T. Annese and B. Nico, Aquaporins in cancer, Biochim Biophys Acta 1840: ((2014) ), 1550–1553. |
[5] | A.S. Verkman, M.O. Anderson and M.C. Papadopoulos, Aquaporins: Important but elusive drug targets, Nat Rev Drug Discov 13: ((2014) ), 259–277. |
[6] | T. Walz, Y. Fujiyoshi and A. Engel, The AQP structure and functional implications, Handb Exp Pharmacol ((2009) ), 31–56. |
[7] | G. Zhao, J. Li, J. Wang, X. Shen and J. Sun, Aquaporin 3 and 8 are down-regulated in TNBS-induced rat colitis, Biochem Biophys Res Commun 443: ((2014) ), 161–166. |
[8] | J.Q. Wang, L. Zhang, X.G. Tao, L. Wei, B. Liu, L.L. Huang and Y.G. Chen, Tetramethylpyrazine upregulates the aquaporin 8 expression of hepatocellular mitochondria in septic rats, J Surg Res 185: ((2013) ), 286–293. |
[9] | H. Chang, Y.H. Shi, T.K. Talaf and C. Lin, Aquaporin-8 mediates human esophageal cancer Eca-109 cell migration via the EGFR-Erk1/2 pathway, Int J Clin Exp Pathol 7: ((2014) ), 7663–7671. |
[10] | Q. Wu, Z.F. Yang, K.J. Wang, X.Y. Feng, Z.J. Lv, Y. Li and Z.X. Jian, AQP8 inhibits colorectal cancer growth and metastasis by down-regulating PI3K/AKT signaling and PCDH7 expression, Am J Cancer Res 8: ((2018) ), 266–279. |
[11] | Q. Shen, W. Lin, H. Luo, C. Zhao, H. Cheng, W. Jiang and X. Zhu, Differential expression of aquaporins in cervical precursor lesions and invasive cervical cancer, Reprod Sci 23: ((2016) ), 1551–1558. |
[12] | J.P. Thiery, H. Acloque, R.Y. Huang and M.A. Nieto, Epithelial-mesenchymal transitions in development and disease, Cell 139: ((2009) ), 871–890. |
[13] | R. Kalluri, EMT: When epithelial cells decide to become mesenchymal-like cells, J Clin Invest 119: ((2009) ), 1417–1419. |
[14] | B. De Craene and G. Berx, Regulatory networks defining EMT during cancer initiation and progression, Nat Rev Cancer 13: ((2013) ), 97–110. |
[15] | I. Pastushenko and C. Blanpain, EMT transition states during tumor progression and metastasis, Trends Cell Biol 29: ((2019) ), 212–226. |
[16] | R. Qureshi, H. Arora and M.A. Rizvi, EMT in cervical cancer: Its role in tumour progression and response to therapy, Cancer Lett 356: ((2015) ), 321–331. |
[17] | M. Chetry, Y. Song, C. Pan, R. Li, J. Zhang and X. Zhu, Effects of galectin-1 on biological behavior in cervical cancer, J Cancer 11: ((2020) ), 1584–1595. |
[18] | A.S. Verkman, M. Hara-Chikuma and M.C. Papadopoulos, Aquaporins – new players in cancer biology, J Mol Med (Berl) 86: ((2008) ), 523–529. |
[19] | G.P. Nagaraju, R. Basha, B. Rajitha, O.B. Alese, A. Alam, S. Pattnaik and B. El-Rayes, Aquaporins: Their role in gastrointestinal malignancies, Cancer Lett 373: ((2016) ), 12–18. |
[20] | A. Galan-Cobo, R. Ramirez-Lorca and M. Echevarria, Role of aquaporins in cell proliferation: What else beyond water permeability? Channels (Austin) 10: ((2016) ), 185–201. |
[21] | A.J. Levine and A.M. Puzio-Kuter, The control of the metabolic switch in cancers by oncogenes and tumor suppressor genes, Science 330: ((2010) ), 1340–1344. |
[22] | I. Medrano-Fernandez, S. Bestetti, M. Bertolotti, G.P. Bienert, C. Bottino, U. Laforenza, A. Rubartelli and R. Sitia, Stress regulates aquaporin-8 permeability to impact cell growth and survival, Antioxid Redox Signal 24: ((2016) ), 1031–1044. |
[23] | M.J. Marchissio, D.E. Frances, C.E. Carnovale and R.A. Marinelli, Mitochondrial aquaporin-8 knockdown in human hepatoma HepG2 cells causes ROS-induced mitochondrial depolarization and loss of viability, Toxicol Appl Pharmacol 264: ((2012) ), 246–254. |
[24] | C.L. Chaffer and R.A. Weinberg, A perspective on cancer cell metastasis, Science 331: ((2011) ), 1559–1564. |
[25] | M.C. Papadopoulos, S. Saadoun and A.S. Verkman, Aquaporins and cell migration, Pflugers Arch 456: ((2008) ), 693–700. |
[26] | K. Morishita, K. Watanabe and H. Ichijo, Cell volume regulation in cancer cell migration driven by osmotic water flow, Cancer Sci 110: ((2019) ), 2337–2347. |
[27] | E. McCoy and H. Sontheimer, Expression and function of water channels (aquaporins) in migrating malignant astrocytes, Glia 55: ((2007) ), 1034–1043. |
[28] | J. Hu and A.S. Verkman, Increased migration and metastatic potential of tumor cells expressing aquaporin water channels, FASEB J 20: ((2006) ), 1892–1894. |
[29] | M. Suarez-Carmona, J. Lesage, D. Cataldo and C. Gilles, EMT and inflammation: Inseparable actors of cancer progression, Mol Oncol 11: ((2017) ), 805–823. |