PD-1/PD-L1 pathway: Current research in breast cancer
Abstract
INTRODUCTION:
Immunotherapy has shown encouraging outcomes in breast cancer (BC) treatment in recent years. The programmed cell death ligand 1 (PD-L1) transmembrane protein is suggested to function as a co-inhibitory factor in the immune response, where it collaborates with programmed cell death protein 1 (PD-1) to stimulate apoptosis, suppress cytokine release from PD-1 positive cells, and limit the growth of PD-1 positive cells. Furthermore, in many malignancies, PD-L1 reduces the immune system’s response to neoplastic cells. These observations suggest that the PD-1/PD-L1 axis plays a vital role in cancer therapy and the regulation of cancer immune escape mechanisms. This review aimed to provide an overview of the functions of PD-1 and PD-L1 in BC cancer therapy.
METHODS:
This research design is a literature review. The style is a traditional review on topics or variables relating to the PD-1/PD-L1 pathway. A literature search was carried out using three online databases.
RESULTS:
The search using the keywords yielded a total of 248 studies. Each result was filtered again according to the inclusion and exclusion criteria, resulting in a final total of 4 studies to be included in the literature review.
CONCLUSIONS:
The combination of PD-1/PD-L1 is essential for many malignancies. According to the evidence presented, this combination presents both an opportunity and a challenge in cancer treatment. Since many solid cancers, especially BC, express high levels of PD-1/PD-L1, cancer treatment mainly involves targeted therapies.
1.Introduction
Breast cancer (BC) treatment is tailored depending on individual circumstances [1,2]. Research in oncology (genomics, transcriptomics, metabolomics, and proteomics), equipment development (diagnostic and therapeutic), and drug discovery play an important role in the treatment of BC [3,4]. Surgery remains the primary modality, and numerous innovations have evolved mastectomy techniques [4–6].
Cancer research traditionally focused on cancer cells and cancer as a gene-disease; however, extensive investigations into this disease as a systemic condition have identified cancer as a complex disease with multiple manifestations. Therefore, research has advanced from a focus on cancer cells to a holistic approach based on the function of the immune system within the host body and its environment of origin. Two hallmarks of cancer related to the immune system are chronic inflammation, which encourages tumor development, and the ability of cancer cells to evade destruction by the immune system [7]. The immune system can fail to inhibit the proliferation of cancer cells and induce further development of these cells, which is known as the immunoediting hypothesis [8]. The capacity to evade the immune system is an important component of the ten attributes of cancer cells outlined by Hanahan and Weinberg (Fig. 1) [9].
In recent years, Immunotherapy has shown encouraging outcomes in breast cancer (BC) treatment. Programmed cell death protein 1 (PD-1) promotes self-tolerance and suppresses immunological responses by preventing regulatory T cells’ apoptosis, triggering antigen-specific T cells’ apoptosis, and controlling T-cell activity. The programmed cell death ligand 1 (PD-L1) transmembrane protein is suggested to function as a co-inhibitory factor in the immune response, where it collaborates with PD-1 to stimulate apoptosis, suppress cytokine release from PD-1 positive cells, and limit the growth of PD-1 positive cells. Furthermore, in many malignancies, PD-L1 reduces the immune system’s response to neoplastic cells. These observations suggest that the PD-1/PD-L1 axis plays a vital role in cancer therapy and the regulation of cancer immune escape mechanisms. This review aimed to provide an overview of the functions of PD-1 and PD-L1 in BC with the goal of enhancing cancer therapy.
2.Methods
This research design is a literature review. The style is a traditional review on topics or variables relating to the PD-1/PD-L1 pathway. A literature search was conducted using three online databases (PubMed, Crossref, and Google Scholar). Search terms included the main keywords “programmed cell death 1 receptor” and “programmed cell death 1 ligand 1”, as well as several supporting keywords “breast cancer” and “immune system”. The articles included were those with the full text available and published in English.
Fig. 2.
PRISMA flow diagram was used in this study.
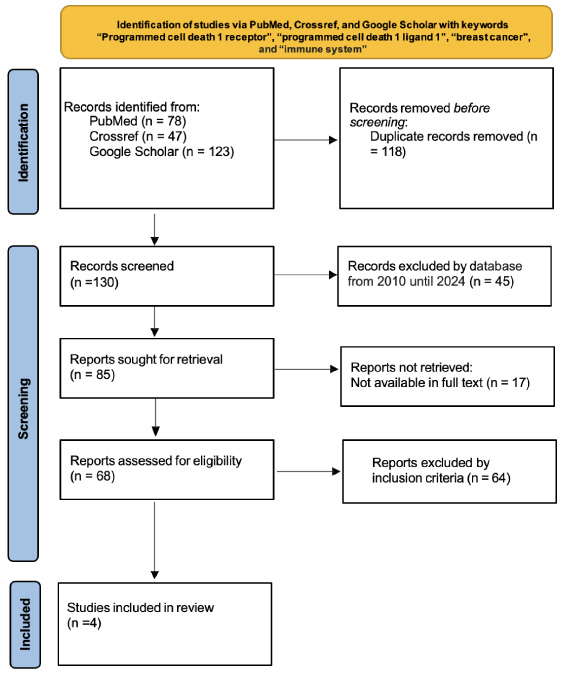
3.Results
The search using the main keywords “programmed cell death 1 receptor” and “programmed cell death 1 ligand 1”, as well as several supporting keywords previously described, yielded a total of 248 studies. Each result was screened based on the article’s title and abstract, and filtered again according to the inclusion and exclusion criteria, resulting in a final total of 4 studies to be included in the literature review (Fig. 2).
4.Discussion
4.1.Cancer immune system
To effectively eliminate cancer cells, the immune response must include a series of sequential and repetitive processes that establish a cycle of cancer immunity (Fig. 3). Cancer cells initially produce neoantigens that are subsequently released and captured by antigen-presenting cells (APCs). To elicit an anti-tumor response and mitigate peripheral resistance to the generated tumor antigen, a distinct signal is required during this phase. These immunogenic signals include stimuli such as inflammatory cytokines and chemical substances that are discharged by apoptotic tumor cells [11].
The processed antigen is presented to the T cells when the APCs attach to the major histocompatibility complex (MHC) molecules. The effector T cells then identify cancer-specific antigens as potentially harmful invaders, at which time the ratio between the effector and regulatory (T reg) T cells determines the type of immune response activated. Once activated, effector T cells proceed to the tumor site and interact with antigens on the tumor cells, after which they bind to these cells and kill them. As tumor cells are destroyed, the response cycle strengthens with the addition of greater numbers of tumor-specific antigens. This process is not ideal and several problems can arise with this system. For example, tumor antigens might not be detected; APCs and T cells might mistake tumor antigens for self-antigens, resulting in a T reg response rather than an anti-tumor response; and the tumor microenvironment could suppress the effector cells that are produced [11].
The cancer immunological cycle requires the coordinated action of several components, including immune stimulators that activate the immune system against cancer cells and inhibitors that regulate this activity. These factors collaborate at each step of the cycle. Stimulating factors promote immunity, while inhibitory factors monitor ongoing processes, reduce immune response activity, and/or prevent auto-immunity. Checkpoint proteins such as PD-L1, which are termed immunostats, exhibit inhibitory functions to modulate the active immune response in tumor tissues. For example, when the PD-1 receptor on the surface of T cells binds to PD-L1 expressed in cancer cells, T cell functioning is inhibited. Over-expression of PD-L1 is common in various types of cancer and is associated with a poor prognosis due to its inhibitory effect on anti-tumor responses [12].
4.2.Immunoediting
The immunoediting process elucidates the role of the immune system in the advancement and evolution of breast cancer. The immune system contributes to the prevention of cancer as well as the development, invasion, and metastasis of the disease. Currently, a number of cancer therapy approaches focus on methods to utilize the immune system more efficiently, such as the use of adaptive immunotherapy and monoclonal antibodies to prevent inhibitory signals received by T cells, such as those of anti-PD-1 [13].
The three primary stages of immunoediting are elimination, equilibrium, and escape (Fig. 4). The elimination phase involves the identification and eradication of cancer cells by the adaptive immune system. Perforin is released by cytolytic immune cells to trigger cancer cell lysis. The cancer cells that pass through the elimination stage transition into the equilibrium phase, where they exploit the negative regulatory dynamics of the immune system. During this phase, the immune response efficiently regulates the proliferation, spread, and programmed cell death (apoptosis) of the cancer cells. However, heterogeneity and genetic variation among cancer cells allow them to escape from this equilibrium, at which time immunosuppressive mechanisms are activated [14].
4.3.The effect of the immune response on tumor development
The growth and metastasis of circulating tumor cells (CTCs) involve the release of cancer cells, evasion of the immune system, and the production of distant metastases by adhesion to and exudation from blood vessels. The interactions of the immune system with CTCs are crucial for these processes. Primary tumors release thousands of tumor cells on average into the blood each day; however, the number of CTCs is rarely measured. This is because tumor cells that are released from the original immunosuppressive microenvironment become vulnerable to attacks by immune cells [14]. A diagram of the immunological escape of CTCs is presented in Fig. 5.
The immune response is highly dependent on the ability of the immune system to recognize the antigen that triggers the immune reaction. The immune response cells originate from pluripotent stem cells that differentiate through either the lymphoid pathway, which generates lymphocytes and their subsets, or the myeloid pathway, which forms phagocytic and other cells. The main immunocompetent cells are T lymphocytes, while the minor components include T helper, T cytotoxic, and T regulator cells, each of which serves a different function [15].
Immunoediting is an important part of tumor progression. The elimination of transformed cells can induce the production of tumor variants with low immunogenicity and more resistance to effector T cells in the equilibrium phase through methods such as the production of inhibitory signals. Furthermore, tumor-derived soluble factor (TDSF) can induce several mechanisms that allow for escape from immune cell attacks in the tumor microenvironment [16,17]. The failure of immune cells to eliminate primary tumor cells causes these cells to proliferate and undergo mitosis. Cell proliferation is generally associated with rapid tumor growth and more aggressive biological and clinical behavior [18], and this activity can be identified by performing a simple calculation of the mitotic index. The mitotic index is the gold standard for determining the aggressiveness and degree of differentiation of several tumors, including BC [19].
Increased tumor progression due to failure of the immune response is evident when an increase in tumor size is observed. A more significant amount of proliferation and mitosis of tumor cells results in an accelerated size increase, which can be evaluated through clinical examination and imaging. Solid cancer treatment involves both the removal of the primary tumor and the elimination of metastases; therefore, an understanding and control of metastasis is important in clinical practice. Metastases start from a single solid tumor that undergoes several stages of metastasis, leading to cancers that have spread and are challenging to cure. In each stage, the host immune system can identify the cancer cells. At the primary tumor stage, some immune cells act as pro-metastatic agents, such as T regs, which provide immunosuppression through the secretion of IL 10, thereby inhibiting the response of cytotoxic T cells and NK cells. Additional immune cells, including macrophages, participate in the process of angiogenesis, whereby M2 tumor-associated macrophages (TAMs) generate CCL-2 and IL-10 to encourage angiogenesis; boost immunosuppression by blocking DCs, T cells, and NK cells via expressing arginase; and promote invasion by using matrix metalloprotease to modify the stroma. Some immune cells act as anti-metastatic agents, such as cytotoxic NK cells, T cells, and M1 TAMs, which kill cancer cells. At the M2 circulation stage, TAMs and T reg cells act as pro-metastatic agents, whereby T reg cells reduce and suppress NK cells and T cells in the circulation, which allows metastatic cells to survive. While cytotoxic T cells and NK cells recognize and kill cancer cells, M1 TAM cells produce CXCL9, IL-6, and IL-12 to express iNOS and activate the immune system to directly destroy tumor cells through the generation of nitric oxide. At the metastatic-niche stage, mature NK cells promote metastatic niche growth when these cells have low immune capacities [19].
In contrast, immature NK cells inhibit metastatic niche formation. At this stage, macrophages (M2) are involved in preparing the metastatic niche by inducing the local immunosuppressive environment, while M1 stimulates local immunity. In addition, cytotoxic T cells can recognize and kill cancer cells when they enter the metastatic niche.
5.PD-L1
5.1.PD-L1 structure
When T lymphocytes recognize antigens on specific cells through the identification of the major histocompatibility complex (MHC), inflammatory cytokines are secreted into the circulatory system. Immunological tolerance is promoted by programmed death-1 (PD-1; CD279) protein activation on T cells, which leads to the stimulation of programmed death-ligand 1 (PD-L1) production in tissues via the action of a specific cytokine. The user has included a number reference, suggesting the inclusion of a citation or source [20].
The inhibitory co-receptor PD-1 is found on the surface of several cell types, including B lymphocytes, CD8+ and CD4+ T cells, NK cells, and other subtypes of tumor-infiltrating lymphocytes (TILs). The user has supplied no textual description of the number reference. Given that long-term antigen exposure may induce a selective increase in PD-1 expression, T cell PD-1 expression could indicate T cell fatigue. Academic rewriting of the user’s material is not possible due to a lack of information. The type 1 transmembrane protein PD-1 is encoded by the programmed cell death protein 1 (PDCD1) gene.
Fig. 6.
Diagrammatic representation of the protein structure domains, mRNA, and PD-L1 gene [22]. Created with Biorender.com.
![Diagrammatic representation of the protein structure domains, mRNA, and PD-L1 gene [22]. Created with Biorender.com.](https://content.iospress.com:443/media/bd/2024/43-1/bd-43-1-bd249006/bd-43-bd249006-g006.jpg)
The PD-1 molecule is composed of three primary structural elements: an external immunoglobulin variable (IgV) domain, a hydrophobic transmembrane region, and several intracellular regions. The intracellular tail components include the immunoreceptor tyrosine-based switch motif (ITSM) and the immunological receptor tyrosine-based inhibitory motif (ITIM), both of which have the potential to undergo phosphorylation. The activation of immune receptor PD-1 has been suggested to require the presence of active immunoreceptor tyrosine-based switch motifs (ITSMs) for the modulation of T cell responses [21].
PD-L2 (also known as B7-DC or CD273) and PD-L1 (also known as B7-H1 or CD274) are ligands that function as co-inhibitors and comprise the PD-1 receptor. PD-L1 is a protein encoded by the CD274 gene that contains 290 amino acids and has a molecular weight of 40 kDa (Fig. 6). PD-L1 is a crucial modulator of the adaptive immune response and is potentially situated on chromosome 9 p24 [21].
The promoter region of the CD274 gene contains numerous components that respond to IFN-γ; therefore, CD274 plays a crucial role in the IFN-γ-induced upregulation of PD-L1 expression [23]. PD-L1 operates as a transmembrane protein that consists of two extracellular domains (Ig-V and Ig-C) and a single transmembrane region. PD-L1 is a concise cytoplasmic domain that transmits intracellular signals; it is encoded by the CD274 gene and consists of 7 exons (Fig. 7), each of which codes for a specific part of the protein [24].
Exon 1 encodes the intracellular and transmembrane domains, the signal sequence, and the IgC-like and IgV-like domains, while exon 7 encodes the 3’ UTR and intracellular domain. The PD-L1 gene contains seven exons (Fig. 7) and the PD-L1 protein is composed of a transmembrane domain, two extracellular domains that are similar to immunoglobulin C and immunoglobulin V, and a solitary intracellular domain [24].
5.2.PD-L1 regulation
Various cells, including tumor cells, macrophages, and T and B lymphocytes, express CD40-ligand 1 (PD-L1) [25]. However, not all tumors or malignant cells express PD-L1, despite its presence in the cytoplasm and plasma membrane of cancer cells. Figure 8A shows the many pro-inflammatory molecules, VEFG, GM-CSF, LPS, TNF-α, types I and II IFN-γ, IL-4, and IL-10 cytokines, that initiate PD-L1 expression. Among them, activated T cells release the most effective trigger, IFN-γ [21], while TGF-β, PTEN, and p53 inhibit PD-L1 expression (Fig. 8B). In contrast to PD-L1, which can be expressed in several cell types, PD-L2 expression is limited to APCs [24].
Studies have shown that PD-L1 expression is regulated by several signaling pathways, transcriptional factors, and epigenetic factors [24].
5.2.1.Signaling pathways
A. | MAPK Pathway Activation of the mitogen-activated protein kinase (MAPK) signaling pathway can cause an increase in the level of PD-L1 expression. The MAPK pathway is an oncogenic mechanism in non-small cell lung cancer (NSCLC) that upregulates PD-L1 via the activation of the epidermal growth factor receptor (EGFR). Chemotherapeutic drug-induced PD-L1 upregulation in cancer cells may be due to MAPK pathway activation. For example, the MEK inhibitor U0126 eliminates the PD-L1 expression that is induced by paclitaxel while cisplatin concentrations trigger PD-L1 expression through MAPK activation [24]. |
B. | PI3K/Akt Pathway The phosphoinositide 3-kinase (PI3K)/Akt pathway has been implicated in PD-L1 regulation in cancer cells since it was determined that PI3K inhibitors reduce PD-L1 expression in BRAF inhibitor-resistant melanoma cells. For example, Akt inhibition eliminates the increase of PD-L1 that occurs when PTEN is depleted. The PD-L1 increases via PI3K/Akt signaling are accompanied by transcriptional upregulation as well as posttranslational processes; in colon cancer cells, stimulation of Akt causes PD-L1 protein overexpression independent of the PD-L1 mRNA expression levels. The transcriptional and posttranslational mechanisms of the ePI3K/Akt pathway regulate various cells and tissues to control PD-L1 expression. Although the downstream effector mTOR/S6 has not been shown to mediate the action of Akt on PD-L1 expression, the inhibition of Akt results in a decrease in PD-L1 expression. In contrast, PD-L1 expression is regulated by nuclear factor NF-B, which is a downstream target of Akt, whereby the transcriptional increase in PD-L1 occurs as a result of Akt activating NF-B [24]. |
C. | JAK-STAT signaling pathway The STAT pathway is activated by growth factors, IFNs, cytokines, and similar chemicals and is considered an evolutionarily conserved process [26]. This strategy provides extracellular factors with a crucial tool for controlling gene expression and represents a basic example of how cells respond to their environment and interpret signals to regulate proliferation and division [27]. Recent studies have shown that tumors manufacture PD-L1 via the JAK/STAT system, and according to Doi et al., PD-L1 expression may be blocked at both the protein and mRNA levels by using the JAK2 inhibitor AG490 [28]. These results support the suggestion that the JAK/STAT pathway regulates PD-L1 expression. In addition, the effective activation of the JAK/STAT3 signaling pathway by fibroblast growth factor receptor (FGFR)2 signaling corresponded with elevated PD-L1 expression in vitro. Xenograft models of colorectal cancer revealed that the over-expression of FGFR2 accelerated tumor development and caused PD-L1 expression to spike. One possible strategy to mitigate this impact is the use of JAK inhibitors to impede the JAK/STAT3 pathway [29]. |
D. | WNT signaling pathway Previous studies [30] have shown that dysregulated WNT signaling is closely associated with the development of tumors, the transition of cells from cancerous to malignant, and resistance to conventional cancer therapies. Aberrant WNT signaling disrupts the process of cancer immunomonitoring, resulting in immune evasion and reduced sensitivity to immunotherapies, including those of immune checkpoint inhibitors [31]. For example, in the absence of WNT ligands, non-glycosylated PD-L1 showed accelerated proteasomal degradation in breast cancer mouse models when active signaling was present along the GSK3B-β-TrCP axis. However, GSK3 blockers increased tumor eradication by reducing PDCD1 gene expression in animal melanoma models [32]. The functional interaction between WNT activity and PD-L1 expression allows triple-negative breast cancer to be treated with specific WNT inhibitors or activators to reduce or boost PD-L1 expression, respectively [33]. |
E. | NF-κB signaling pathway It has been suggested that toll-like receptors (TLRs) or IFN-γ-driven nuclear factor (NF)-κB stimulate PD-L1 gene expression. Curcumin is an NF-κB inhibitor that when combined with an anti-CTLA-4 checkpoint inhibitory drug suppresses the growth of melanoma, breast, and colon cancer cell lines. Inhibiting the survival and multiplication of tumor cells and targeting immunological checkpoints within tumors could suppress NF-κB [34], which may be involved in LMP1-induced PD-L1 expression since the caffeic acid phenethyl ester (an NF-κB inhibitor) reduced PD-L1 induction. In addition to INF-γ, the transcription factor NF-κB is a key regulator of PD-L1 expression that has been shown to successfully decrease IFN-induced PD-L1 expression while STAT3, PI3K, and MAPK inhibitors failed to achieve the same result [35]. Peng et al. [36] revealed that chemotherapy causes higher levels of PD-L1 in ovarian cancer patients due to NF-κB-mediated local immune suppression. |
5.2.2.Hedgehog signaling pathway
Recent therapeutic advancements have focused on the utilization of small molecular inhibitors, such as SMO and GLI, to modify the Hedgehog (Hh) signaling pathway. This pathway has been deemed crucial for the proliferation of substrate cells, and deviations in its functioning can result in the development of tumors [37]. Furthermore, Hh signaling could have a role in the regulation of PD-L1 production, and the inhibition of Hh signaling may elicit anti-tumor activity in lymphocytes [38]. According to Chakrabarti et al. [39], specific patients should receive a combined treatment approach that targets both the Hh signaling and immunological checkpoints since Hh signals are involved in PD-1/PD-L1 inhibition, which leads to the reversal of Gli2-induced tolerance and the expression of PD-L1 in gastric cancer.
Fig. 9.
PD-1/PD-L1 signaling reduces proliferation, survival, and cytokine production of CD8+ T cells [25]. Created with Biorender.com.
![PD-1/PD-L1 signaling reduces proliferation, survival, and cytokine production of CD8+ T cells [25]. Created with Biorender.com.](https://content.iospress.com:443/media/bd/2024/43-1/bd-43-1-bd249006/bd-43-bd249006-g009.jpg)
5.3.PD-1/PD-L1 signaling
Numerous studies have reported that immunopathology and immunological tolerance inhibitory signals are conveyed through interactions between PD-L1 and PD-1. PD-1/PD-L1 signaling can hinder the immune response through the phosphoinositide 3-kinase (PI3K), T-cell receptor (TCR), and phosphatase-1 (SHP-1) signaling pathways (Fig. 9) [23]. The effect of PD-L1 on effector T cells and PD-1 inhibits TCR signal transduction, which in turn suppresses T cell cytotoxicity. The activity of immunoreceptor tyrosine-based switch motifs (ITSM) and tyrosine-based inhibitory motifs (ITIM) may be enhanced when SHP-1 is suppressed and CD8+ T cell activity is controlled by sodium stibogluconate. The ITSM motif of PD-1 may be tyrosine phosphorylated by ITSM, which then transports SHP-2 and SHP-1 to the ITSM motif. Following this absorption, the signaling pathway prevents dendritic cells and T cells from interacting and suppressing the stop signal. Lastly, blocking TCR signal transmission inhibits MAPK and PI3K/Akt signaling. In addition, the activation of Akt and inhibition of PI3K reduce B-cell lymphoma-extra-large (Bcl-xl) expression, which in turn increases T cell apoptosis [23].
PD-L1 blocks the Ras/MEK/ERK and PI3K/Akt pathways, which prevents the activation of T cells and the operation of transcription factors that are critical for T cell survival. The interaction between PD-1 and PD-L1 could inhibit the expression of T-bet and GATA-3. The GATA-3 transcription factor is involved in Th2 cell production, while the T-bet transcription factor could enhance T-cell proliferation by binding to the T-box region [23].
However, it is unclear whether all types of cancer use the exact mechanism of PD-L1 signaling since some cancer types differ in their prognoses, possibly due to the involvement of different PD-L1 mechanisms [25].
6.PD-1/PD-L1 function in cancer
6.1.Breast cancer
PD expression has been correlated with epithelial-mesenchymal transition (EMT) in human breast cancer stem cells (BCSCs). The observed increase in PD-L1 expression in MCF-7 and BT-549 tumorspheres when compared to the cell line may be partly attributed to demethylation inside the PD-L1 promoter region. In addition, the overexpression of histone acetylase in the tumor can accelerate PD-L1 transcription. The distribution of slow histones in the PD-L1 promoter region is less evident in the tumor than it is in the cell line [40]. These results indicate that PD-L1 has an important function in BCSCs.
Recent pathological investigations have determined that multiple subtypes of estrogen receptor (ER) α-negative BC contained increased levels of PD-L1. Liu et al. revealed a significant discrepancy in the mean expression of PD-L1 mRNA between breast cancer cell lines that were negative for ERα and those that were positive for Erα. Therefore, the expression of PD-L1 in ER-negative (MDA-MB-231 and BT549) and ER-positive breast cancer cell lines (MCF7 and T47D) was examined. The protein and mRNA levels of PD-L1 in the T47D and MCF7 cells were significantly lower than those in the MDA-MB-231 and BT549 cells, according to RT-qPCR and western blot studies [41]). Recent studies have shown that PD-L1 suppresses the development of cancer cells and that doxorubicin-induced and spontaneous death in BC cells are increased when PD-L1 is silenced [42].
7.Inhibitors of PD-1/PD-L1 in cancer
Cancer progression may be significantly slowed with the use of PD-1/PD-L1 targeted therapies. A few critical regimens for enhancing cancer treatment are outlined in Fig. 10.
1. | Pembrolizumab Joseph etal.revealed that the therapeutic effectiveness of pembrolizumab in lung metastases was significantly higher (62%) than its effectiveness in managing liver metastases (22%) [44]. The one-year overall survival rates for people with lung and liver metastases were 89% and 53%, respectively. Patients diagnosed with metastatic squamous cell carcinoma (SCCHN) and/or recurrences in the head and neck region had limited overall survival rates, often not exceeding six months. The use of anti-PD-1 checkpoint drugs in immunotherapy has increased survival rates and tumor responses. Pembrolizumab had a response rate of 18% and a median OS of 6 to 8 months in treated metastatic and recurrent patients in the single-arm phase II KEY- NOTE-055 trials and phase Ib KEYNOTE-012 trials [45]. Furthermore, HER2-positive, trastuzumab-resistant, and PD-L1-positive BC patients have been treated with pembrolizumab with trastuzumab [45]. |
2. | Nivolumab The completely human monoclonal antibody nivolumab can inhibit the interaction between PD-L1 and PD-1. Zhao etal.described a case in which a patient with advanced liver carcinosarcoma had partial remission after apatinib and nivolumab therapy [46]. However, there was a notable rise in the level of grade 3 aminotransferase throughout the therapy. Therefore, prudence should be used when contemplating combination treatment and a comprehensive evaluation of potential risks should be undertaken before endorsing its implementation. The results of the CheckMate 275 phase 2 clinical trial suggest that individuals diagnosed with advanced urothelial carcinoma who have shown resistance to platinum-based chemotherapy in prior therapeutic interventions may have favorable outcomes with nivolumab [47]. Immunotherapy has a number of advantages over the few chemotherapy choices available after platinum-based chemotherapy, one of which is the increased endurance of its therapeutic effects. Long-term survival rates in other trials corroborate this, including the phase 3 CheckMate 057 (n =582) and CheckMate 017 (n =272) trials. The effectiveness of docetaxel and nivolumab in treating advanced non-squamous NSCLC that has previously been treated was compared in these studies [48]. The predicted 3-year overall survival rate for docetaxel was 8% in the combined CheckMate 057 and 017 trial groups, whereas the rate was 17% for the nivolumab group for patients with non-squamous NSCLC, the predicted 2-year OS rates with docetaxel were 8%, whereas for squamous NSCLC, the rates were 16% and 29%, for docetaxel and nivolumab, respectively. |
3. | JQ1 Liu etal.studied the effects of JQ1 on PD-L1 protein and mRNA expression in various cancer cell lines and primary culture cells derived from renal cell carcinomas. The cancer cell lines included a variety of malignancies, including prostate, lung, and liver tumors [49]. Those authors reported that JQ1 had an inhibitory impact on cell growth that was dependent on the dosage administered. A reduction in the expression of PD-L1 was seen in primary cultures of lung, liver, prostate, and kidney cancer cell lines after treatment with JQ1. In addition, a decrease was observed in PD-L2 levels in the cells treated with JQ1. Therefore, JQ1 appears to have the ability to regulate the PD-1/PD-L1 axis [43]. |
4. | Cemiplimab The use of PD-1 inhibitors has produced positive outcomes in the treatment of advanced cutaneous squamous cell carcinoma (cSCC). Cemiplimab is a monoclonal antibody that can bind to programmed death receptor-1 (PD-1), which inhibits its interaction with both PD-L2 and PD-L1. In September 2018, the Food and Drug Administration (FDA) authorized Cemiplimab for the treatment of patients with locally advanced metastatic colorectal cancer who are ineligible for radiation therapy or surgery [50]. |
5. | Atezolizumab Atezolizumab is a monoclonal antibody that has undergone humanization and was designed to selectively inhibit interactions between PD-L1 and its receptors, B7.1 and PD-1. This compound augments the immunological response facilitated by T cells against neoplastic conditions [51]). Atezolizumab monotherapy has emerged as a potential treatment for metastatic NSCLC and UC. Recently, the IMmotion 151 phase 3 study compared sunitinib with bevacizumab (anti-VEFG) and atezolizumab as possible first-line treatments for colorectal cancer. Bevacizumab/atezolizumab achieved one of its co-primary goals, in that it showed better investigator-assessed PFS in patients with advanced colorectal cancer when compared to sunitinib and ≥1% of tumor-infiltrating immune cells expressed PD-L1, according to immunohistochemistry [52]. |
6. | Avelumab The monoclonal human IgG1 antibody avelumab inhibits the PD-L1 receptor. A phase 1b study (JAVELIN Solid Tumor) revealed a decrease in PD-L1 expression in tumor and immune cells in patients with recurrent or metastatic NSCLC who had previously undergone therapy. Results from a phase 1b, non-randomized trial encompassing 55 patients with advanced renal cell carcinoma showed that the combination of axitinib and avelumab was effective. Specifically, 58% of patients had successful responses and 78% of those attained illness control [53]. |
8.Conclusion
The combination of PD-1/PD-L1 is essential for many malignancies; therefore, more research in this area is needed. According to the evidence presented, this combination presents both an opportunity and a challenge in the treatment of cancer. Since many solid cancers, especially BC, express high levels of PD-1/PD-L1, cancer treatment mainly involves targeted therapies. The promising findings of immunotherapy suggest that this treatment may increase the life span of specific patients. PD-1/PD-L1 immunotherapy blockades could be the next logical progression for various cancer types; however, many factors are yet to be determined, including dosage, durability, efficacy, and safety.
Acknowledgements
None.
Ethic committee
Not applicable.
Conflict of interest
Each author declares that he or she has no commercial associations (e.g., consultancies, stock ownership, equity interest, patent/licensing arrangement etc.) that might pose a conflict of interest in connection with the submitted article.
Data availability statement
The data presented in this study are available on request from the corresponding author.
Funding
This article review received no external funding.
Authors contribution
SAS, MF, and AP study design; SAS, MF, and AP databases search and articles selection; All authors participate in the debate and consensus on the articles to be included. SAS, MF, and AP manuscript writing. NS, ES, FI, and IFT proof-reading of the manuscript.
References
[1] | Berberabe T, Tailoring therapy in breast cancer proves to be an elusive goal, Target Ther Oncol, 12: (9): 53–55, (2023) . |
[2] | Coates AS, , Winer EP, , Goldhirsch A , Tailoring therapies—improving the management of early breast cancer: St Gallen International Expert Consensus on the Primary Therapy of Early Breast Cancer 2015, Ann Oncol, 26: (8): 1533–1546, (2015) . doi:10.1093/annonc/mdv221. |
[3] | Kwon YW, , Jo H-S, , Bae S , Application of proteomics in cancer: Recent trends and approaches for biomarkers discovery, Front Med, 8: : 747333, (2021) . doi:10.3389/fmed.2021.747333. |
[4] | Prihantono P, , Rusli R, , Christeven R, , Faruk M, Cancer incidence and mortality in a tertiary hospital in Indonesia: An 18-year data review, Ethiop J Health Sci, 33: (3): 515–522, (2023) . doi:10.4314/ejhs.v33i3.15. |
[5] | Keelan S, , Flanagan M, , Hill ADK, Evolving trends in surgical management of breast cancer: An analysis of 30 years of practice changing papers, Front Oncol, 11: : 622621, (2021) . doi:10.3389/fonc.2021.622621. |
[6] | Freeman MD, , Gopman JM, , Salzberg CA, The evolution of mastectomy surgical technique: From mutilation to medicine, Gland Surg, 7: (3): 308–315, (2018) . doi:10.21037/gs.2017.09.07. |
[7] | Finn OJ, Immuno-oncology: Understanding the function and dysfunction of the immune system in cancer, Ann Oncol Off J Eur Soc Med Oncol, 23: (8): 6–9, (2012) . doi:10.1093/annonc/mds256. |
[8] | Jacqueline C, , Bourfia Y, , Hbid H, , Sorci G, , Thomas F, , Roche B, Interactions between immune challenges and cancer cells proliferation: Timing does matter! Evol Med public Heal, 2016: (1): 299–311, (2016) . doi:10.1093/emph/eow025. |
[9] | Hanahan D, , Weinberg RA, Hallmarks of cancer: The next generation, Cell, 144: (5): 646–674, (2011) . doi:10.1016/j.cell.2011.02.013. |
[10] | Hanahan D, Hallmarks of cancer: New dimensions, Cancer Discov, 12: (1): 31–46, (2022) . doi:10.1158/2159-8290.CD-21-1059. |
[11] | Chen DS, , Mellman I, Oncology meets immunology: The cancer-immunity cycle, Immunity, 39: (1): 1–10, (2013) . doi:10.1016/j.immuni.2013.07.012. |
[12] | Disis ML, Immune regulation of cancer, J Clin Oncol Off J Am Soc Clin Oncol, 28: (29): 4531–4538, (2010) . doi:10.1200/JCO.2009.27.2146. |
[13] | Pandya PH, , Murray ME, , Pollok KE, , Renbarger JL, The immune system in cancer pathogenesis: Potential therapeutic approaches, J Immunol Res, 2016: : 4273943, (2016) . doi:10.1155/2016/4273943. |
[14] | Zhong X, , Zhang H, , Zhu Y , Circulating tumor cells in cancer patients: Developments and clinical applications for immunotherapy, Mol Cancer, 19: (1): 15, (2020) . doi:10.1186/s12943-020-1141-9. |
[15] | Kresno SB. Imunologi: Diagnosis Dan Prosedur Laboratorium. . 5th ed. (Jakarta), Badan Penerbit Fakultas Kedokteran Universitas Indonesia; 1–33. (2010) . |
[16] | Kim R, , Emi M, , Tanabe K, Cancer immunoediting from immune surveillance to immune escape, Immunology, 121: (1): 1–14, (2007) . doi:10.1111/j.1365-2567.2007.02587.x. |
[17] | Schreiber RD, , Old LJ, , Smyth MJ, Cancer immunoediting: Integrating immunity’s roles in cancer suppression and promotion, Science, 331: (6024): 1565–1570, (2011) . doi:10.1126/science.1203486. |
[18] | Bartoš V, , Adamicová K, , Kullová M, , Péč M, Immunohistochemical evaluation of proliferative activity (Ki-67 index) in different histological types of cutaneous basal cell carcinoma, Biologia (Bratisl), 67: (3): 610–615, (2012) . doi:10.2478/s11756-012-0035-8. |
[19] | Devianti L, , Asri A, Peningkatan Ekspresi Ki67 Tidak Berhubungan dengan Para-meter Prognostik Histopatologik Karsinoma Payudara Invasif di Sumatera Barat, Indones J Pathol, 21: (3): 1–6, (2012) . [Indonesia]. |
[20] | Alsaab HO, , Sau S, , Alzhrani R , PD-1 and PD-L1 checkpoint signaling inhibition for cancer immunotherapy: Mechanism, combinations, and clinical outcome, Front Pharmacol, 8: : 561, (2017) . doi:10.3389/fphar.2017.00561. |
[21] | He J, , Hu Y, , Hu M, , Li B, Development of PD-1/PD-L1 pathway in tumor immune microenvironment and treatment for non-small cell lung cancer, Sci Rep, 5: (1): 13110, (2015) . doi:10.1038/srep13110. |
[22] | Fabrizio FP, , Trombetta D, , Rossi A, , Sparaneo A, , Castellana S, , Muscarella LA, Gene code CD274/PD-L1: From molecular basis toward cancer immunotherapy, Ther Adv Med Oncol, 10: : 1758835918815598, (2018) . doi:10.1177/1758835918815598. |
[23] | Guo L, , Lin Y, , Kwok HF, The function and regulation of PD-L1 in immunotherapy, ADMET DMPK, 5: (3): 159, (2017) . doi:10.5599/admet.5.3.442. |
[24] | Chen J, , Jiang CC, , Jin L, , Zhang XD, Regulation of PD-L1: A novel role of pro-survival signalling in cancer, Ann Oncol Off J Eur Soc Med Oncol, 27: (3): 409–416, (2016) . doi:10.1093/annonc/mdv615. |
[25] | Yu J, , Wang X, , Teng F, , Kong L, PD-L1 expression in human cancers and its association with clinical outcomes, Onco Targets Ther, 9: : 5023–5039, (2016) . doi:10.2147/OTT.S105862. |
[26] | Banerjee S, , Biehl A, , Gadina M, , Hasni S, , Schwartz DM, JAK-STAT signaling as a target for inflammatory and autoimmune diseases: Current and future prospects, Drugs, 77: (5): 521–546, (2017) . doi:10.1007/s40265-017-0701-9. |
[27] | Groner B, , von Manstein V, Jak Stat signaling and cancer: Opportunities, benefits and side effects of targeted inhibition, Mol Cell Endocrinol, 451: : 1–14, (2017) . doi:10.1016/j.mce.2017.05.033. |
[28] | Doi T, , Ishikawa T, , Okayama T , The JAK/STAT pathway is involved in the upregulation of PD-L1 expression in pancreatic cancer cell lines, Oncol Rep, 37: (3): 1545–1554, (2017) . doi:10.3892/or.2017.5399. |
[29] | Li P, , Huang T, , Zou Q , FGFR2 promotes expression of PD-L1 in colorectal cancer via the JAK/STAT3 signaling pathway, J Immunol, 202: (10): 3065–3075, (2019) . doi:10.4049/jimmunol.1801199. |
[30] | Harb J, , Lin P-J, , Hao J, Recent development of Wnt signaling pathway inhibitors for cancer therapeutics, Curr Oncol Rep, 21: (2): 12, (2019) . doi:10.1007/s11912-019-0763-9. |
[31] | Galluzzi L, , Spranger S, , Fuchs E, , López-Soto A, WNT signaling in cancer immunosurveillance, Trends Cell Biol, 29: (1): 44–65, (2019) . doi:10.1016/j.tcb.2018.08.005. |
[32] | Taylor A, , Rothstein D, , Rudd CE, Small-molecule inhibition of PD-1 transcription is an effective alternative to antibody blockade in cancer therapy, Cancer Res, 78: (3): 706–717, (2018) . doi:10.1158/0008-5472.CAN-17-0491. |
[33] | Castagnoli L, , Cancila V, , Cordoba-Romero SL , WNT signaling modulates PD-L1 expression in the stem cell compartment of triple-negative breast cancer, Oncogene, 38: (21): 4047–4060, (2019) . doi:10.1038/s41388-019-0700-2. |
[34] | Lim W, , Jeong M, , Bazer FW, , Song G, Curcumin suppresses proliferation and migration and induces apoptosis on human placental choriocarcinoma cells via ERK1/2 and SAPK/JNK MAPK signaling pathways, Biol Reprod, 95: (4): 83, (2016) . doi:10.1095/biolreprod.116.141630. |
[35] | Bi X-W, , Wang H, , Zhang W-W , PD-L1 is upregulated by EBV-driven LMP1 through NF-κB pathway and correlates with poor prognosis in natural killer/T-cell lymphoma, J Hematol Oncol, 9: (1): 109, (2016) . doi:10.1186/s13045-016-0341-7. |
[36] | Peng J, , Hamanishi J, , Matsumura N , Chemotherapy induces programmed cell death-ligand 1 overexpression via the nuclear factor-κB to foster an immunosuppressive tumor microenvironment in ovarian cancer, Cancer Res, 75: (23): 5034–5045, (2015) . doi:10.1158/0008-5472.CAN-14-3098. |
[37] | Wu F, , Zhang Y, , Sun B, , McMahon AP, , Wang Y, Hedgehog signaling: From basic biology to cancer therapy, Cell Chem Biol, 24: (3): 252–280, (2017) . doi:10.1016/j.chembiol.2017.02.010. |
[38] | Martin AM, , Nirschl CJ, , Polanczyk MJ , PD-L1 expression in medulloblastoma: An evaluation by subgroup, Oncotarget, 9: (27): 19177–19191, (2018) . doi:10.18632/oncotarget.24951. |
[39] | Chakrabarti J, , Holokai L, , Syu L , Hedgehog signaling induces PD-L1 expression and tumor cell proliferation in gastric cancer, Oncotarget, 9: (100): 37439–37457, (2018) . doi:10.18632/oncotarget.26473. |
[40] | Darvin P, , Sasidharan Nair V, , Elkord E, PD-L1 expression in human breast cancer stem cells is epigenetically regulated through posttranslational histone modifications, J Oncol, 2019: : 3958908, (2019) . doi:10.1155/2019/3958908. |
[41] | Liu L, , Shen Y, , Zhu X , ERα is a negative regulator of PD-L1 gene transcription in breast cancer, Biochem Biophys Res Commun, 505: (1): 157–161, (2018) . doi:10.1016/j.bbrc.2018.09.005. |
[42] | Liu S, , Chen S, , Yuan W , PD-1/PD-L1 interaction up-regulates MDR1/P-gp expression in breast cancer cells via PI3K/AKT and MAPK/ERK pathways, Oncotarget, 8: (59): 99901–99912, (2017) . doi:10.18632/oncotarget.21914. |
[43] | Han Y, , Liu D, , Li L, PD-1/PD-L1 pathway: Current researches in cancer, Am J Cancer Res, 10: (3): 727–742, (2020) . PIMD: 32266087. |
[44] | Joseph RW, , Elassaiss-Schaap J, , Kefford R , Baseline tumor size is an independent prognostic factor for overall survival in patients with melanoma treated with pembrolizumab, Clin Cancer Res an Off J Am Assoc Cancer Res, 24: (20): 4960–4967, (2018) . doi:10.1158/1078-0432.CCR-17-2386. |
[45] | Pelster MS, , Mott F, , Lewin J, Pembrolizumab-induced mucositis in a patient with recurrent hypopharynx squamous cell cancer, Laryngoscope, 130: (4): E140–E143, (2020) . doi:10.1002/lary.28038. |
[46] | Zhao L, , Yang Y, , Gao Q, Efficacy and safety of nivolumab plus apatinib in advanced liver carcinosarcoma: A case report, Immunotherapy, 11: (8): 651–656, (2019) . doi:10.2217/imt-2018-0214. |
[47] | Hussain SA, , Birtle A, , Crabb S , From clinical trials to real-life clinical practice: The role of immunotherapy with PD-1/PD-L1 inhibitors in advanced urothelial carcinoma, Eur Urol Oncol, 1: (6): 486–500, (2018) . doi:10.1016/j.euo.2018.05.011. |
[48] | Schiwitza A, , Schildhaus H-U, , Zwerger B , Monitoring efficacy of checkpoint inhibitor therapy in patients with non-small-cell lung cancer, Immunotherapy, 11: (9): 769–782, (2019) . doi:10.2217/imt-2019-0039. |
[49] | Liu K, , Zhou Z, , Gao H , JQ1, a BET-bromodomain inhibitor, inhibits human cancer growth and suppresses PD-L1 expression, Cell Biol Int, 43: (6): 642–650, (2019) . doi:10.1002/cbin.11139. |
[50] | Ogata D, , Tsuchida T, Systemic immunotherapy for advanced cutaneous squamous cell carcinoma, Curr Treat Options Oncol, 20: (4): 30, (2019) . doi:10.1007/s11864-019-0629-2. |
[51] | Crist M, , Balar A, Atezolizumab in invasive and metastatic urothelial carcinoma, Expert Rev Clin Pharmacol, 10: (12): 1295–1301, (2017) . doi:10.1080/17512433.2017.1389275. |
[52] | Atkins MB, , Tannir NM, Current and emerging therapies for first-line treatment of metastatic clear cell renal cell carcinoma, Cancer Treat Rev, 70: : 127–137, (2018) . doi:10.1016/j.ctrv.2018.07.009. |
[53] | Lantuejoul S, , Damotte D, , Hofman V, , Adam J, Programmed death ligand 1 immunohistochemistry in non-small cell lung carcinoma, J Thorac Dis, 11: (1): S89–S101, (2019) . doi:10.21037/jtd.2018.12.103. |