Acute Ethanol Exposure Enhances Synaptic Plasticity in the Dorsal Striatum in Adult Male and Female Rats
Abstract
Background:
Acute (ex vivo) and chronic (in vivo) alcohol exposure induces neuroplastic changes in the dorsal striatum, a critical region implicated in instrumental learning.
Objective:
Sex differences are evident in alcohol reward and reinforcement, with female rats consuming higher amount of alcohol in operant paradigms compared to male rats. However, sex differences in the neuroplastic changes produced by acute alcohol in the dorsal striatum have been unexplored.
Methods:
Using electrophysiological recordings from dorsal striatal slices obtained from adult male and female rats, we investigated the effects of ex vivo ethanol exposure on synaptic transmission and synaptic plasticity. Ethanol (44 mM) enhanced basal synaptic transmission in both sexes. Ethanol also enhanced long-term potentiation in both sexes. Other measures of synaptic plasticity including paired-pulse ratio were unaltered by ethanol in both sexes.
Results:
The results suggest that alterations in synaptic plasticity induced by acute ethanol, at a concentration associated with intoxication, could play an important role in alcohol-induced experience-dependent modification of corticostriatal circuits underlying the learning of goal-directed instrumental actions and formation of habits mediating alcohol seeking and taking.
Conclusions:
Taken together, understanding the mechanism(s) underlying alcohol induced changes in corticostriatal function may lead to the development of more effective therapeutic agents to reduce habitual drinking and seeking associated with alcohol use disorders.
INTRODUCTION
Alcohol is the oldest sedative hypnotic widely and legally used and abused for its effects on memory [1–4]. Alcohol use disorder (AUD) is a chronic relapsing disorder characterized by compulsive alcohol-drinking and alcohol-seeking behaviors [5–7]. The precise molecular mechanisms by which alcohol produces its effects within the central nervous system are only now beginning to be understood [8]. Mechanistically, the effects of alcohol are diffuse, with influence on various ligand- and voltage-gated ion channels [9, 10]. Additionally, progressive neuroadaptations in striatal circuits have been reported with exposure to alcohol [8, 11], and such adaptations could be the neurobiological underpinning of moderate to severe AUDs.
The dorsal striatum is a brain region that controls movement, is implicated in mediating the formation of goal-directed responses and behavioral habits, and plays a role in the binge/intoxication phase of the addiction cycle [7, 12, 13]. Recent studies in male rats and mice have demonstrated that superfusion of ethanol (EtOH) onto acute slices or prior alcohol experience leads to disruption of synaptic plasticity in the dorsal striatum [14–17] and ventral striatum [18, 19]. Mechanistic studies have shown that acute EtOH-induced long-term depression (LTD) in the dorsal striatum is dependent on inactivation of N-methyl-D-aspartic acid receptors (NMDARs) type of glutamate receptors and activation of both dopamine D2 and cannabinoid CB1 receptors [14]. Other reports demonstrate that while acute EtOH induced an immediate depressive effect, washout after acute EtOH conditions induces long-term facilitation, particularly in the dorsal striatum; this effect requires activation of GluN2B-containing NMDARs [15]. More recent findings show that washout after acute EtOH and repeated systemic administration of EtOH followed by acute withdrawal facilitates long-term potentiation (LTP) in the dorsal striatum in a GluN2B-dependent manner [17]. Few studies have investigated whether protracted consumption of EtOH affects synaptic transmission in the dorsal striatum. Recent evidence also shows that repeated cycles of EtOH consumption alters neurotransmission in the dorsomedial striatum, evident as strengthening of glutamatergic transmission in direct pathway neurons [20]. More notable is that months of EtOH consumption also leads to reduced GABAergic neurotransmission in the dorsal striatum [21]. Furthermore, the increased synaptic transmission observed after months of EtOH consumption in the dorsomedial striatum is not restored by abstinence, indicating that these effects are long-lasting [22]. Taken together, these studies demonstrate that the effects of EtOH (acute and in vivo) and washout/withdrawal from EtOH on synaptic transmission and synaptic plasticity in male rodents in the dorsal striatum are multifaceted, and bidirectional in the case of the dorsomedial striatum [14, 17].
In this study we examined the effects of acute EtOH on long-term synaptic plasticity in the dorsomedial striatum of adult male and female rats. Female rats self-administer more EtOH, and this behavior is consistent with the previous literature in humans that demonstrate gender differences in alcohol drinking, pharmacokinetics, peak blood alcohol levels and alcohol elimination rate [23–25]. Based on these sex differences in alcohol drinking and seeking behaviors [11, 26, 27], we hypothesized that acute EtOH will produce varied effects on synaptic transmission and synaptic plasticity in the dorsal striatum in male and female rats.
MATERIAL AND METHODS
Animals
Experimental procedures were carried out in strict adherence to the National Institutes of Health Guide for the Care and Use of Laboratory Animals and approved by the Institutional Animal Care and Use Committee of VA San Diego Healthcare System. Twenty two adult Long-Evans male rats (weighing 300–350 g at the time of the experiment) and eight adult female rats (weighing 180 to 220 g at the time of the experiment; bred at the VA Vivarium), were housed two per cage in a temperature-controlled vivarium under a reverse light/dark cycle (lights off 9 : 00 am–9 : 00 pm) until study completion.
Slice preparation
Ten to twelve-week-old male and female rats were anesthetized with isoflurane and killed by rapid decapitation. Brains were quickly removed and placed in ice-cold artificial cerebrospinal fluid (ACSF) containing (in mM): NaCl 125, NaHCO3 26, KCl 4, NaHPO4 1.25, CaCl2 2, MgCl2 1 and glucose 10 bubbled with 95% oxygen and 5% CO2 [28]. Brains were trimmed on the dorsal side at an angle of approximately 140° from the horizontal plane and glued to a vibratome base (Leica VT1000S) on that side. This resulted in 440μm thick slices that included cortico-striatal projections, as tested by afferent stimulation at cortico-striatal border, and recordings in the striatum [28]. Immediately after slicing, two slices (representing 1.6 to 1.0 mm from bregma) were transferred to the interface chamber and were incubated in oxygenated ACSF at room temperature for at least 2–2.5 hours before initiating recordings. Recordings were made in the dorsomedial striatum (Fig. 1a-b), in a submersion-type recording chamber superfused with oxygenated ACSF at a rate of 2–3 ml/min at room temperature and positioned on a platform of an upright motorized microscope (Olympus BX51 WI, Scientifica) equipped with a back Illuminated sCMOS camera (Prime 95B, Photometrics) and a broad-spectrum LED illuminator (pE-300, CoolLED).
Fig. 1
Basal synaptic transmission, short-term plasticity and synaptic plasticity in dorsomedial striatal slices from adult male rats with paradigm a. (a) Schematic of a coronal slice representing the area of the dorsomedial striatum in red rectangular box used for stimulation and recordings. (b) Infrared microphotograph of a 440μm thick corticostriatal slice from one adult male rat indicating the location of the stimulating electrode (SE) and recording electrode (RE). Scale bar in (b) is 100μm. Thick arrows point to the electrodes. CC: corpus callosum; DStr: dorsal striatum. (c) Input/output (I/O) curve obtained by plotting the slope of fEPSPs as a function of the stimulation intensity (from 100 to 800μA) in the dorsomedial striatum. Inset in (c) shows a representative fEPSP waveform illustrating the parameter computed in the study, including fEPSP slope (measured between the two red lines) from control (black trace) and EtOH (blue trace) treated slices. Gray arrow in the trace points to fiber volley and black arrow points to fEPSPs. (d) Paired-pulse ratio recorded from one interstimulus interval at 50 ms. Inset in (d) shows raw trace of paired-pulse with fEPSP slope measured between the two red lines from a control treated slice. Gray arrow in the trace points to fiber volley and black arrow points to fEPSPs. (e) x-y graph of time course of fEPSPs before and after HFS in all groups. Arrow in (e) at zero points to the time of HFS. Data are represented as mean±S.E.M.. n = 8 control male, Number of slices: n = 8 EtOH male. %p < 0.05 main effect of EtOH, $p < 0.05 main effect of stimulus intensity, ∧p < 0.05 main effect of time by two-way ANOVA. *p < 0.05 vs. controls by Fisher’s LSD post-hoc tests.
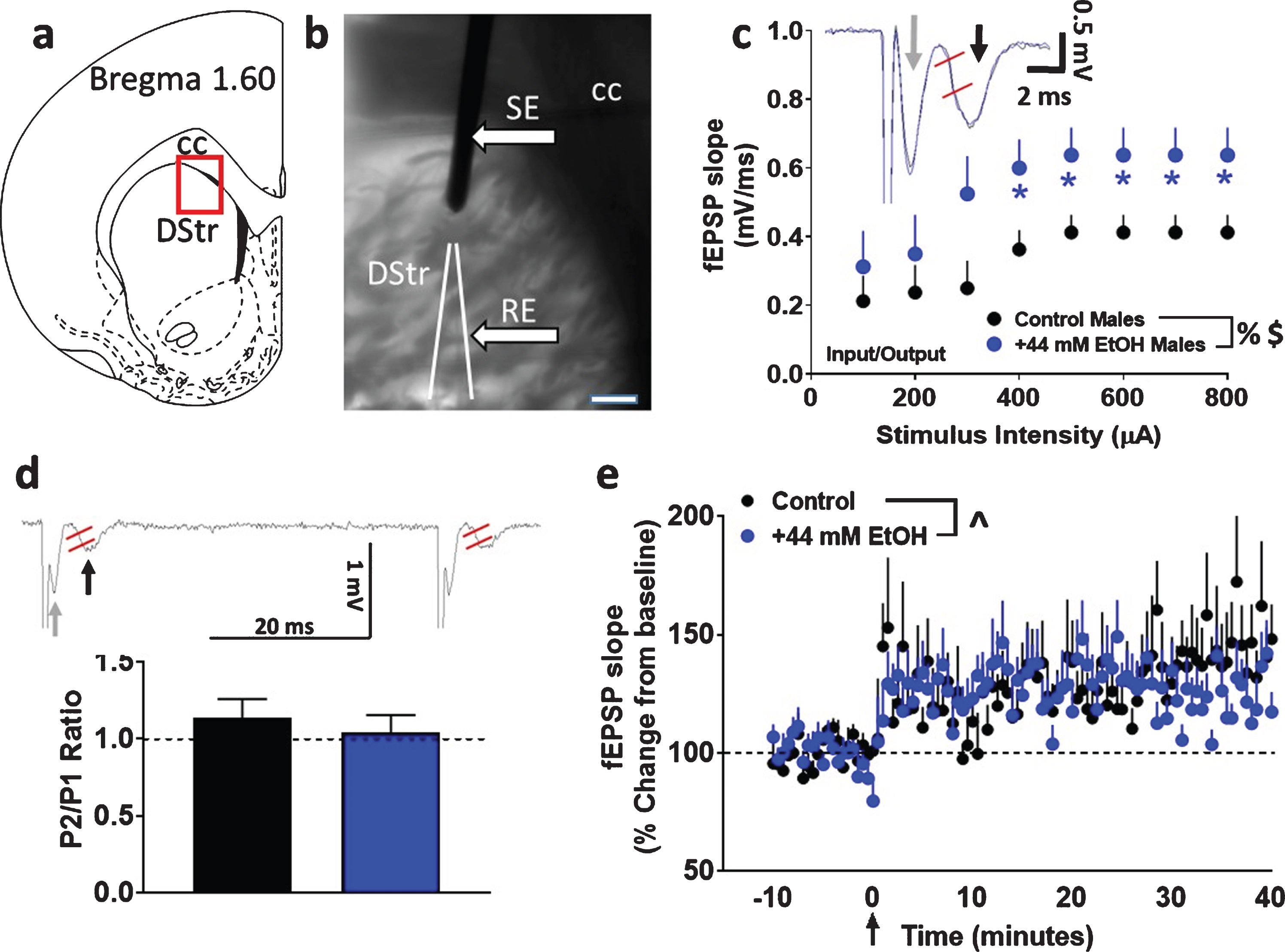
Field potential recordings
To study basal synaptic transmission, paired-pulse ratio and synaptic plasticity under control and EtOH conditions, local field potentials were recorded in acute brain slices from two (2) cohorts of rats. Cohort 1 (paradigm a) was made of male rats (n = 8) while cohort 2 (paradigm b) contained both male (n = 14) and female (n = 8) rats.
Population spikes or field excitatory postsynaptic potentials (fEPSPs) were evoked by extracellular stimulation (0.03 Hz, 0.2 milliseconds) in the dorsomedial striatum using a single silver-coated tungsten wire stimulating electrode (50μm, A-M System; Fig. 1a-b). fEPSPs were recorded using ACSF-filled patch pipettes with tip resistances of 2–4 MΩ. Pipettes were pulled from borosilicate glass capillaries (PG150T-10, 1.5 OD×1.16 ID×100 L mm, Harvard Apparatus) using a micropipette puller (PC-10, Narishige). Two to three slices per rat were used for recordings. One or two slices were recorded under control conditions (ACSF) and the other slice was recorded after superfusion of 44 mM EtOH in ACSF [29]. Slices were continuously super-perfused with EtOH for at least 30 minutes before the start of each recording session and until the end of the experiment.
Basal synaptic transmission was analyzed by generating stimulus/response curves or input/output (I/O) curves prior to each synaptic plasticity experiment. I/O curves were generated by plotting stimulus intensity (100–800μA) versus slope of fEPSPs (Fig. 1c; [30]). As indicated in Fig. 1c, fiber volley was evident in most of our recordings. However, since fiber volleys were not consistent in all of our recordings, we did not measure changes in fiber volley amplitude which represents action potential firing in pre-synaptic fibers. We only measured fEPSPs which represents action potentials (population spikes) in the dendritic region. The slope of fEPSPs were measured after the stimulus artifact and the fiber volley from the initial 2 to 5 milliseconds of the rising phase to about half-peak time of the synaptic response. We chose to assess slopes of the fEPSPs as this component of the fEPSP is considered to reflect monosynaptic activation of cortical afferents mainly of medium-sized spiny neurons, and hence is best suited to gauge monosynaptic plasticity [28, 31]. For the remainder of the experiment, the test stimulus intensity was set to elicit a fEPSP that is approximately 40–50% of the maximum response recorded during the I/O measurements. Paired-pulse ratios (P2/P1) were evaluated by evoking fEPSPs with half-maximal amplitude using paired-pulses of electrical stimulation with a constant test stimulus intensity and 50 millisecond inter-stimulus intervals (ISI) (Fig. 1d). fEPSPs at this constant test stimulus intensity were monitored for a period of 25 minutes to ensure a stable responses before induction of LTP.
For induction of synaptic plasticity or LTP in dorsal striatal synapses the following two high-frequency stimulation (HFS) paradigms were used: (paradigm a; cohort 1) three 3 sec, 100 Hz trains delivered 20 seconds apart; (paradigm b; cohort 2) four 1 sec, 100 Hz trains delivered 10 seconds apart [14, 32, 33]. For comparisons of treatment effects on fEPSP slope between slices, values for each recording were normalized to the average slope for the 10 min of baseline before HFS was initiated. Data was acquired, filtered (highpass, 0.1 Hz; lowpass 3 kHz) and amplified using a computer-controlled patch-clamp amplifier (MultiClamp 700B, Molecular Devices) and digitized using an analog to digital converter (Digidata 1550A1, Molecular Devices). Analysis of sEPSP slope was performed using pClamp10.4 software (Molecular Devices).
Statistical analyses
Effects of EtOH and sex on fEPSP slope were analyzed using a two- or three-way ANOVA using SPSS version 19 (IBM, Armonk, NY). Effects of EtOH and sex on paired-pulse ratio was analyzed using Students t test or two-way ANOVA using GraphPad Prism version 7. Post hoc analyses were conducted when a significant interaction was detected. Significance was set at p < 0.05.
RESULTS
Cohort 1 studies
Acute application of EtOH enhances basal synaptic transmission in slices
Basal synaptic transmission, measured as the I/O relationship, was significantly different in EtOH-treated male rat striatal slices (cohort 1) from those recorded in slices under control conditions. Repeated measures two-way ANOVA with superfusion of EtOH and stimulus intensity as independent variables and fEPSP slope as dependent variable did not detect a significant stimulus intensity×EtOH interaction (F (7, 98) = 1.021, p = 0.4). There was however, a main effect of EtOH (F (1, 14) = 4.575, p = 0.05) and stimulus intensity (F (7, 98) = 7.712, p < 0.001; Fig. 1c).
Short-term plasticity is not differentially expressed under control and EtOH conditions
Short-term plasticity was assessed by measuring paired-pulse ratios. Short-term changes, either facilitation or depression of synaptic strength, can be obtained by delivering paired stimuli at various inter-stimulus intervals. In the dorsal striatum, it is described that paired-pulse stimulations lead to short-term depression of fEPSPs [34, 35]. Paired-pulse ratio was calculated as the ratio of the second fEPSP slope relative to that of the first fEPSP slope after an interstimulus interval of 50 milliseconds. Unpaired t test did not detect a significant difference in paired-pulse ratio (p > 0.05; Fig. 1d), indicating that EtOH did not alter short-term plasticity.
EtOH does not alter synaptic plasticity in the dorsomedial striatum under paradigm a
Under both control and EtOH conditions, evoked fEPSPs showed significant LTP post HFS under paradigm a (control: 129±10%; EtOH: 126±9% of baseline). Two-way ANOVA with superfusion of EtOH and time after HFS as independent variables and fEPSP slope as dependent variable did not detect a significant EtOH x time interaction (F (100, 1300) = 1.2, p = 0.06) or a main effect of EtOH (F (1, 13) = 0.05, p = 0.8). There was, however, a main effect of time (F (100, 1300) = 3.501, p < 0.001; Fig. 1e). Thus, 44 mM EtOH did not alter synaptic plasticity under paradigm a.
Cohort 2 studies
Basal synaptic transmission is enhanced in slices by acute application of EtOH in both sexes
First, basal synaptic transmission was evaluated in male and female rats under control and EtOH conditions. Superfusion of 44 mM EtOH significantly enhanced basal transmission in both sexes. Three-way ANOVA with sex as between subject factors, and superfusion of EtOH and stimulus intensity as within subject factors and as independent variables and fEPSP slope as dependent variable detected a sex×EtOH×stimulus intensity interaction (F (1, 280) = 10.8, p = 0.004), however, did not detect a significant sex×EtOH interaction (F (1, 280) = 0.5, p = 0.4), EtOH×stimulus intensity interaction (F (7, 280) = 0.1, p = 0.9) or sex×stimulus intensity interaction (F (1, 280) = 0.001, p = 0.9). A main effect of EtOH (F (1, 280) = 9.6, p = 0.006) and stimulus intensity (F (1, 280) = 33.8, p < 0.001) were detected without a main effect of sex (F (1, 280) = 0.2, p = 0.6; Fig. 2a).
Fig. 2
Basal synaptic transmission and short-term plasticity in dorsomedial striatal slices from adult male and female rats. (a) Input/output (I/O) curve obtained by plotting the slope of fEPSPs as a function of the stimulation intensity (from 100 to 800μA) in the dorsomedial striatum. (b) Representative raw trace of paired-pulse from one control male (black, top trace) and one control female (gray, bottom trace) rat. Gray arrow in the top trace points to fiber volley and black arrow in the top trace points to fEPSPs. fEPSP slope was measured between the two red lines. (c) Paired-pulse ratio recorded from one interstimulus interval at 50 ms. Data are represented as mean±S.E.M.. Number of slices: n = 14 control male, n = 13 EtOH male, n = 9 control female, n = 8 EtOH female. #p < 0.05 significant interaction, %p < 0.05 main effect of EtOH, $p < 0.05 main effect of stimulus intensity by three-way ANOVA. *p < 0.05 vs. control group within each sex by Fisher’s LSD post-hoc tests.
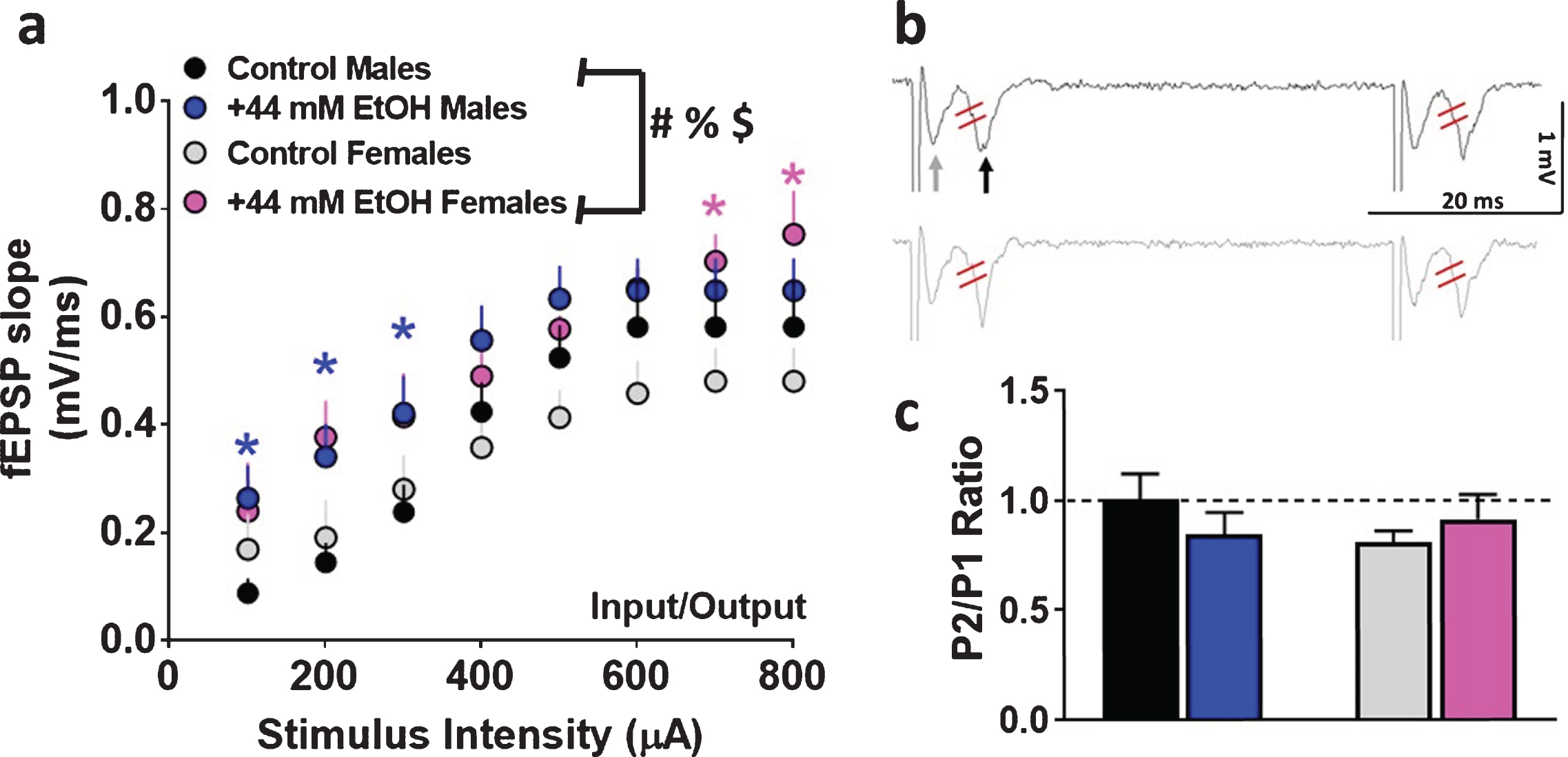
Paired-pulse ratio is not differentially expressed in males versus female rats and is not altered by EtOH in both sexes
Short-term plasticity was assessed by measuring paired-pulse ratio. Two-way ANOVA did not detect a significant interaction (F (1, 36) = 1.6, p = 0.20) or main effect of sex (F (1, 36) = 0.35, p = 0.5) or superfusion of EtOH (F (1, 36) = 0.06, p = 0.8; Fig. 2b-c), indicating that EtOH did not alter short-term plasticity in either of the sexes.
EtOH enhances LTP in the dorsomedial striatum in both sexes under paradigm b
EtOH enhanced striatal synaptic plasticity in both sexes (Fig. 3a–c). Control slices showed significant LTP post HFS (males: 114±6%; females: 112±9% of baseline). Three-way ANOVA with sex, superfusion of EtOH and time after HFS as independent variables and fEPSP slope as dependent variable did not detect a significant sex×EtOH×time interaction (F (100, 4141) = 0.19, p = 1.0), EtOH×time interaction (F (100, 4141) = 1.1, p = 0.14) or sex×time interaction (F (100, 4141) = 0.8, p = 0.8). However, there was a significant sex×EtOH interaction (F (1, 4141) = 20.2, p < 0.001). In addition, there was a main effect of EtOH (F (1, 4141) = 368.1, p < 0.0001), sex (F (1, 4141) = 9.0, p = 0.003) and time (F (100, 4141) = 2.6, p < 0.001). Post hoc analyses did not detect differences between individual time points between males and females or vehicle and EtOH treatments.
Fig. 3
Ex vivo ethanol exposure facilitates the induction of LTP in dorsomedial striatal synapses in adult male and female rats. (a-b) Representative fEPSP waveforms from males (a) and females (b) from various time points post HFS from the same recordings. Gray arrow in the first trace from males points to fiber volley and black arrow points to fEPSPs. Traces were obtained after HFS at 10, 20 and 40 minutes (indicated as open arrows in (c)) timeframe from all groups. Control males, black; EtOH males, blue; control females, gray; EtOH females, pink. Note that the slope of the fEPSPs (the second downward waveform) increases after HFS in EtOH-treated slices versus vehicle control condition in male and female rats. (c) x-y graph of time course of fEPSPs before and after HFS in all groups. Arrow in (c) at zero points to the time of HFS. (d) Average fEPSP slope of each experimental group post HFS (0 to 40 minutes). Data are represented as mean±S.E.M. Number of slices: n = 11 control male, n = 13 EtOH male, n = 8 control female, n = 8 EtOH female. #p < 0.05 significant interaction, %p < 0.05 main effect of EtOH, +p < 0.05 main effect of sex, ∧p < 0.05 main effect of time by three-way or two-way ANOVA.
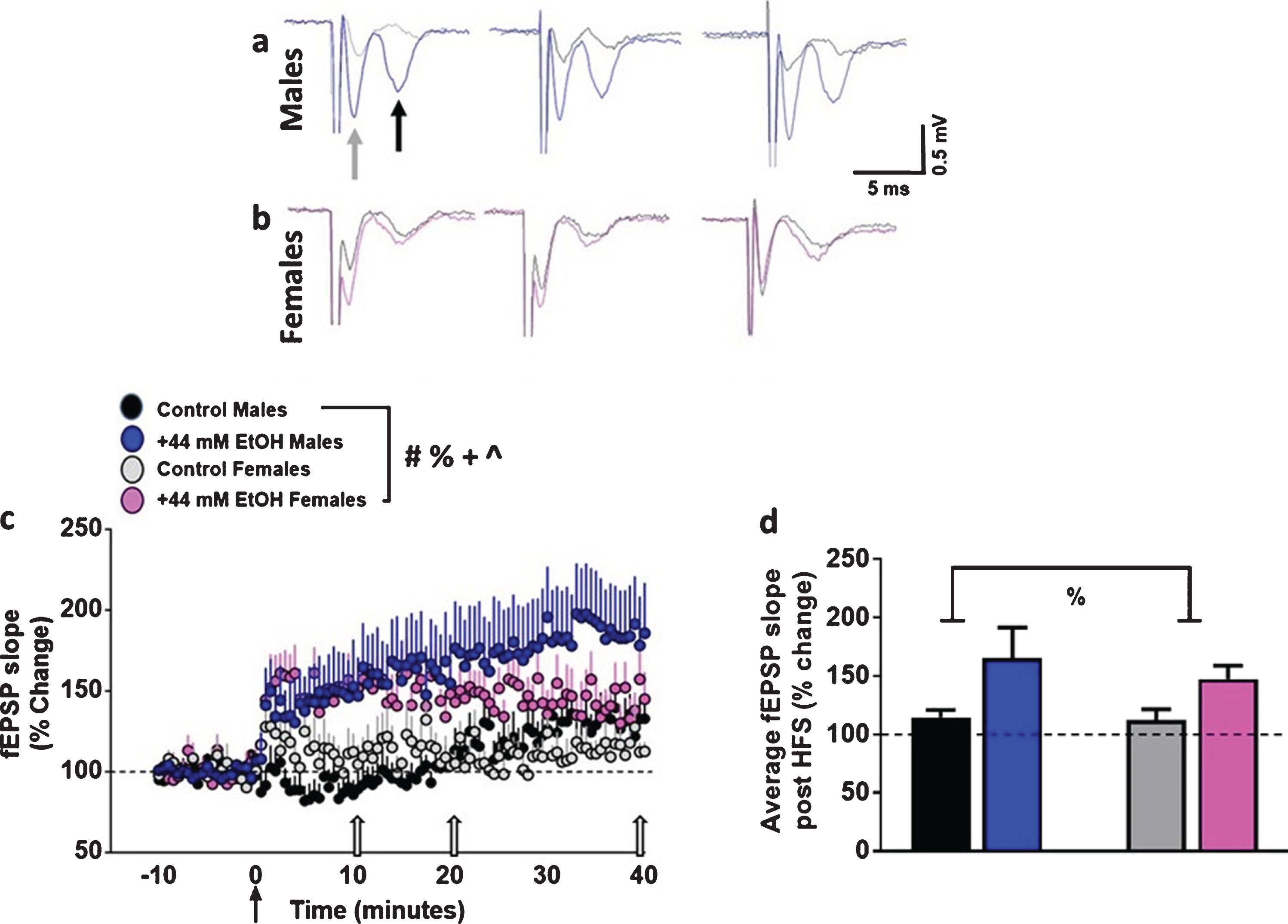
We also measured the degree of LTP for each experimental group, visualized as the average fEPSP slope of the 40 minutes of post-HFS recording period (Fig. 3d). Two-way ANOVA did not detect a sex×EtOH interaction F (1, 36) = 0.16, p = 0.6, or main effect of sex F (1, 36) = 0.2, p = 0.5. However, a main effect of EtOH was evident F (1, 36) = 5.0, p = 0.03. Thus, these findings demonstrate that 44 mM EtOH increased synaptic plasticity in both sexes.
DISCUSSION
One way to better understand EtOH’s effect on learning and memory is to study its acute and chronic effects on synaptic plasticity in the dorsomedial striatum, as this region plays critical roles in instrumental learning [36]. Notably, the dorsal striatum is also involved in the initial binge/intoxication stage of the alcohol addiction cycle [7, 37], as the loss of goal-directed control over alcohol seeking and consumption becomes evident in individuals suffering from moderate to severe AUD. A number of studies have demonstrated that EtOH alters synaptic transmission and plasticity in the dorsal striatum, and other brain regions involved in the addiction cycle [14, 15, 38–41]. However, very few have explored and compared the effects of EtOH on synaptic plasticity in the dorsal striatum in adult male and female rats.
With regard to sex differences, in the chronic intermittent ethanol vapor inhalation model of alcohol dependence, female rats show greater blood alcohol levels compared with males when maintained at similar drip-rate/body weight ratio in the vapor chambers [42]. This is consistent with previous literature in humans and rodents that demonstrate sex differences in pharmacokinetics, peak blood alcohol levels and alcohol elimination rate [23–25, 27, 43]. While these studies are notable, it is unclear still whether the acute effects of EtOH on LTP induction in male rats can be generalized to female rats [14, 17]. We therefore investigated the effects of superfusion of EtOH on basal synaptic transmission and synaptic plasticity (HFS induced LTP) in the dorsomedial striatum in adult male and female rats.
We first tested whether LTP can be induced in adult male rats in the dorsal medial striatum, as most of the previous work has been performed in preadolescent to young adult rats [14, 17, 44, 45]. We used two paradigms that have been shown to induce LTP in the striatum [28, 33, 44, 45]. We demonstrate LTP induction in the dorsomedial striatum using both paradigms in adult male rats. However, we only observed a significant effect of EtOH on LTP induction with paradigm b in adult male rats, and therefore investigated whether paradigm b would produce similar effects in adult female rats. The distinct effect of EtOH on the two LTP induction protocols could be associated with the differential cellular mechanisms underlying LTP induction with each protocol [33, 34, 46–51]. For example, LTP induction by paradigm a is dependent on elevation of intracellular calcium mediated by L-type high-voltage activated calcium channels, and the magnitude of LTP is attributable to the potentiation of the GABAA receptors and not NMDARs. However, LTP induction by paradigm b is dependent on elevation of intracellular calcium mediated by NMDARs, and the magnitude of LTP is attributable to the potentiation of NMDARs and dopamine D1 receptors. Therefore it is tempting to speculate that EtOH-induced enhancement of LTP with paradigm b could be via NMDA or D1 receptor mechanisms.
Sex differences have been detected in dopaminergic and glutamatergic synaptic transmission in the dorsal and ventral striatum with female rats having greater neurotransmission compared to males [52–55]. These neuromodulatory events could underlie sex-dependent differences in basal synaptic transmission observed in the striatum in adult rats, where striatal medium-sized spiny neurons (MSNs) in female rats show higher neuronal excitability [56]. The observed sex differences in neurotransmission in adult rats have been extended in the human striatum, where postmortem tissue analysis indicated higher dendritic spine density of MSNs (a neuroanatomical correlate of excitatory synapse number) in female subjects compared with males [57].
We first compared basal synaptic transmission in adult male and female rats, and report that basal synaptic transmission, measured as I/O curves, does not differ between males and females. Notably, basal synaptic transmission was enhanced by superfusion of 44 mM EtOH in both sexes, indicating that acute EtOH enhances basal excitatory drive in the dorsomedial synapses in both adult male and female rats. This result, however, cannot be generalized to all regions of the brain, as acute effects of EtOH on synaptic transmission in the central nucleus of the amygdala are sex specific [58]. Next, we examined short-term plasticity by studying paired-pulse ratio in dorsomedial striatal synapses. A change in paired-pulse ratio supports either facilitation or depression of synaptic strength, and it is thought to reflect activity-dependent changes of transmitter release from presynaptic terminals [59]. Paired-pulse facilitation (PPF, where paired-pulse ratio is >1), is inversely related to transmitter release such that enhanced probability of transmitter release is associated with a reduction of PPF, whereas decreased probability of transmitter release is associated with an increase in PPF [59]. We report that paired-pulse ratio did not differ in the dorsomedial striatal synapses in both male and female rats, and was not altered by acute EtOH in either sex, supporting previous reports in preadolescent male rats [15, 17]. Therefore, our results highlight the fact that acute EtOH spares presynaptic sites in the dorsomedial striatal synapses, and suggests that EtOH-mediated enhancement of basal synaptic transmission probably occurs through postsynaptic events. This finding, however, is distinct from EtOH-induced effects on paired-pulse ratio in the hippocampus. For example, acute EtOH decreases presynaptic glutamate release in hippocampal CA1 synapses; visualized as increased paired-pulse facilitation. This effect on glutamate release is mediated via presynaptic calcium channels [60, 61]. Thus, the overall effect of EtOH on short-term plasticity seems to vary considerably from one brain region to another. Future mechanistic studies are warranted to determine the brain region specific differences in EtOH modulation of short-term plasticity.
Finally, we examined sex differences in the synaptic plasticity induced by HFS in the dorsomedial striatal synapses and EtOH’s effect on this phenomena. Our results demonstrate an overall effect of sex on LTP in the dorsal striatum, however, further analysis did not detect sex specific effects on the magnitude of LTP under vehicle and EtOH conditions. Therefore, our results demonstrate that the magnitude of HFS-induced LTP in the dorsal striatum is not sex specific and that EtOH enhanced HFS-induced LTP in both sexes. Previous work in preadolescent rats have demonstrated that EtOH reduces HFS-induced LTP in the dorsomedial striatum when EtOH was continuously superfused prior to, during and after HFS [14]. In same-age male rats, EtOH washout enhances HFS-induced LTP in the dorsomedial striatum when EtOH superfusion is discontinued prior to HFS [17]. Our results add to these findings and show that EtOH’s effect on HFS-induced LTP in the dorsomedial striatum of adult male rats is distinct from its effects in the preadolescent brain. Comparing the results from our findings and those from others, we believe that EtOH can modulate synaptic plasticity bidirectionally in the preadolescent versus adult brain. We also report that the enhanced synaptic plasticity by EtOH in the dorsomedial striatum is observed in adult female rats. The finding that the acute effect of EtOH on enhanced synaptic plasticity in the dorsomedial striatum is not sex specific, suggests that this process does not underlie the difference in baseline EtOH consumption between adult male and female rats. However, elucidating EtOH-induced changes in dorsostriatal synaptic plasticity in adult male and female rats following chronic drinking will be an important future pursuit.
Recent mechanistic studies have convincingly shown that excitatory NMDARs in the brain are important sites for EtOH’s actions [62–64]. Concurrently, NMDARs play an important role in learning and memory, and provide a unique role in maintaining and regulating synaptic plasticity [65–67]. Therefore, it appears that EtOH’s actions via the NMDARs could be facilitating the EtOH-induced deficits in synaptic plasticity and learning and memory functions [8, 64, 68, 69]. Based on the available literature, we can speculate that post-synaptic mechanisms contributed to EtOH-induced increases in LTP in the dorsomedial striatum, including activation of GluN2B containing NMDA receptors and insertion of AMPA receptors into post-synaptic sites [15, 17, 18]. Future mechanistic studies are needed to determine the cellular mechanism(s) underlying the enhanced plasticity by acute EtOH in adult animals, and whether the alterations in plasticity by acute EtOH persist in animals that have chronically experienced EtOH in vivo in both sexes.
ACKNOWLEDGMENTS
Funds from the Department of Veterans Affairs (BX003304 to CDM), National Institute on Drug Abuse and National Institute on Alcoholism and Alcohol Abuse (AA020098 and DA034140 to CDM, AA009986 and AA010761 to JJW) supported the study. The authors thank Mr. Wulfran Trenet and Mrs. Rocio Erandi Heyer Osorno for their assistance with animal breeding and maintenance. The authors also thank Drs. Jeffrey Tang (Molecular Devices), and Jeffry Isaacson (Department of Neurosciences, UCSD) for assistance with LTP studies.
Declarations of interest: The authors declare no conflict of interest. The funders had no role in the design of the study; in the collection, analyses, or interpretation of data; in the writing of the manuscript, and in the decision to publish the results.
REFERENCES
[1] | Spear LP . Effects of adolescent alcohol consumption on the brain and behaviour. Nat Rev Neurosci. (2018) ;19: (4):197–214. |
[2] | Sullivan EV . Contributions to Understanding the Neuropsychology of Alcoholism: An INS Legacy. J Int Neuropsychol Soc. (2017) ;23: (9-10):843–859. |
[3] | Van Skike CE , Goodlett C , Matthews DB . Acute alcohol and cognition: Remembering what it causes us to forget. Alcohol. (2019) ;79: :105–125. |
[4] | Waszkiewicz N . et al Neurobiological Effects of Binge Drinking Help in Its Detection and Differential Diagnosis from Alcohol Dependence. Dis Markers. (2018) ;2018: :5623683. |
[5] | Sliedrecht W . et al Alcohol use disorder relapse factors: A systematic review. Psychiatry Res. (2019) ;278: :97–115. |
[6] | Kravitz AV . et al Cortico-striatal circuits:Novel therapeutic targets for substance use disorders. Brain Res. (2015) ;1628: (Pt A):186–98. |
[7] | Koob GF , Volkow ND . Neurobiology of addiction: a neurocircuitry analysis. Lancet Psychiatry. (2016) ;3: (8):760–73. |
[8] | Lovinger DM , Abrahao KP . Synaptic plasticity mechanisms common to learning and alcohol use disorder. Learn Mem. (2018) ;25: (9):425–434. |
[9] | Lovinger DM . Alcohols and neurotransmitter gated ion channels: past, present and future. Naunyn Schmiedebergs Arch Pharmacol. (1997) ;356: (3):267–82. |
[10] | Woodward JJ . Ethanol and NMDA receptor signaling. Crit Rev Neurobiol. (2000) ;14: (1):69–89. |
[11] | Abrahao KP , Salinas AG , Lovinger DM . Alcohol and the Brain: NeuronalMolecular Targets, Synapses, and Circuits. Neuron. (2017) ;96: (6):1223–1238. |
[12] | Graybiel AM , et al , The basal ganglia and adaptive motor control. Science. (1994) ;265: (5180):1826–31. |
[13] | Yin HH , Knowlton BJ . The role of the basal ganglia in habit formation. Nat Rev Neurosci. (2006) ;7: (6):464–76. |
[14] | Yin HH . et al Ethanol reverses the direction of long-term synaptic plasticity in the dorsomedial striatum. Eur J Neurosci. (2007) ;25: (11):3226–32. |
[15] | Wang J . et al Ethanol induces long-term facilitation of NR2B-NMDA receptor activity in the dorsal striatum: implications for alcohol drinking behavior. J Neurosci. (2007) ;27: (13):3593–602. |
[16] | Wang J . et al Alcohol Elicits Functional and Structural Plasticity Selectively in Dopamine D1 Receptor-Expressing Neurons of the Dorsomedial Striatum. J Neurosci. (2015) ;35: (33):11634–43. |
[17] | Wang J . et al Ethanol-mediated facilitation of AMPA receptor function in the dorsomedial striatum: implications for alcohol drinking behavior. J Neurosci. (2012) ;32: (43):15124–32. |
[18] | Jeanes ZM , Buske TR , Morrisett RA . In vivo chronic intermittent ethanol exposure reverses the polarity of synaptic plasticity in the nucleus accumbens shell. J Pharmacol Exp Ther. (2011) ;336: (1):155–64. |
[19] | Renteria R . et al Selective alterations of NMDAR function and plasticity in D1 and D2 medium spiny neurons in the nucleus accumbens shell following chronic intermittent ethanol exposure. Neuropharmacology. (2017) ;112: (Pt A):164–171. |
[20] | Cheng Y . et al Distinct Synaptic Strengthening of the Striatal Direct and Indirect Pathways Drives Alcohol Consumption. Biol Psychiatry. (2017) ;81: (11):918–929. |
[21] | Adermark L . et al Region-specific depression of striatal activity in Wistar rat by modest ethanol consumption over a ten-month period. Alcohol. (2013) ;47: (4):289–98. |
[22] | Lagstrom O . et al Voluntary Ethanol Intake Produces Subregion-Specific Neuroadaptations in Striatal and Cortical Areas of Wistar Rats. Alcohol Clin Exp Res. (2019) ;43: (5):803–811. |
[23] | Thomasson HR . Gender differences in alcohol metabolism. Physiological responses to ethanol. Recent Dev Alcohol. (1995) ;12: :163–79. |
[24] | Rivier C . Female rats release more corticosterone than males in response to alcohol: influence of circulating sex steroids and possible consequences for blood alcohol levels. Alcohol Clin Exp Res. (1993) ;17: (4):854–9. |
[25] | Rivier C , Rivest S , Vale W . Alcohol-induced inhibition of LH secretion in intact and gonadectomized male and female rats: possible mechanisms. Alcohol Clin Exp Res. (1992) ;16: (5):935–41. |
[26] | Erol A , Karpyak VM . Sex and gender-related differences in alcohol use and its consequences: Contemporary knowledge and future research considerations. Drug Alcohol Depend. (2015) ;156: :1–13. |
[27] | Priddy BM . et al Sex, strain, and estrous cycle influences on alcohol drinking in rats. Pharmacol Biochem Behav. (2017) ;152: :61–67. |
[28] | Kohling R . et al Increased excitability in cortico-striatal synaptic pathway in a model of paroxysmal dystonia. Neurobiol Dis. (2004) ;16: (1):236–45. |
[29] | Roberto M . et al Acute and chronic ethanol alter glutamatergic transmission in rat central amygdala: an in vitro and in vivo analysis. J Neurosci. (2004) ;24: (7):1594–603. |
[30] | Petersen RP . et al Electrophysiological identification of medial and lateral perforant path inputs to the dentate gyrus. Neuroscience. (2013) ;252: :154–68. |
[31] | Flagmeyer I , Haas HL , Stevens DR . Adenosine A1 receptor-mediated depression of corticostriatal and thalamostriatal glutamatergic synaptic potentials in vitro. Brain Res. (1997) ;778: (1):178–85. |
[32] | Avchalumov Y . et al Persistent changes of corticostriatal plasticity in dt(sz) mutant hamsters after age-dependent remission of dystonia. Neuroscience. (2013) ;250: :60–9. |
[33] | Bonsi P . et al Coordinate high-frequency pattern of stimulation and calcium levels control the induction of LTP in striatal cholinergic interneurons. Learn Mem. (2004) ;11: (6):755–60. |
[34] | Partridge JG , Tang KC , Lovinger DM . Regional and postnatal heterogeneity of activity-dependent long-term changes in synaptic efficacy in the dorsal striatum. J Neurophysiol. (2000) ;84: (3):1422–9. |
[35] | Mori A . et al Two distinct glutamatergic synaptic inputs to striatal medium spiny neurones of neonatal rats and paired-pulse depression. J Physiol. (1994) ;476: (2):217–28. |
[36] | Balleine BW , Liljeholm M , Ostlund SB . The integrative function of the basal ganglia in instrumental conditioning. Behav Brain Res. (2009) ;199: (1):43–52. |
[37] | Koob GF . et al Neurobiological mechanisms in the transition from drug use to drug dependence. Neurosci Biobehav Rev. (2004) ;27: (8):739–49. |
[38] | Kroener S . et al Chronic alcohol exposure alters behavioral and synaptic plasticity of the rodent prefrontal cortex. PLoS ONE. (2012) ;7: (5):e37541. |
[39] | Lovinger DM , White G , Weight FF . NMDA receptor-mediated synaptic excitation selectively inhibited by ethanol in hippocampal slice from adult rat. J Neurosci. (1990) ;10: (4):1372–9. |
[40] | Carpenter-Hyland EP , Woodward JJ , Chandler LJ . Chronic ethanol induces synaptic but not extrasynaptic targeting of NMDA receptors. J Neurosci. (2004) ;24: (36):7859–68. |
[41] | Nimitvilai S . et al Chronic Intermittent Ethanol Exposure Enhances the Excitability and Synaptic Plasticity of Lateral Orbitofrontal Cortex Neurons and Induces a Tolerance to the Acute Inhibitory Actions of Ethanol. Neuropsychopharmacology. (2016) ;41: (4):1112–27. |
[42] | Fannon MJ . et al Hippocampal neural progenitor cells play a distinct role in fear memory retrieval in male and female CIE rats. Neuropharmacology. (2018) ;143: :239–249. |
[43] | Becker JB , Koob GF . Sex Differences in Animal Models: Focus on Addiction. Pharmacol Rev. (2016) ;68: (2):242–63. |
[44] | Calabresi P . et al Long-term synaptic depression in the striatum: physiological and pharmacological characterization. J Neurosci. (1992) ;12: (11):4224–33. |
[45] | Fino E , Glowinski J , Venance L . Bidirectional activity-dependent plasticity at corticostriatal synapses. J Neurosci. (2005) ;25: (49):11279–87. |
[46] | Lisman J . Long-term potentiation: outstanding questions and attempted synthesis. Philos Trans R Soc Lond B Biol Sci. (2003) ;358: (1432):829–42. |
[47] | Makino Y . et al Enhanced synaptic plasticity in mice with phosphomimetic mutation of the GluA1 AMPA receptor. Proc Natl Acad Sci U S A. (2011) ;108: (20):8450–5. |
[48] | Nguyen PV , Kandel ER . Brief theta-burst stimulation induces a transcription-dependent late phase of LTP requiring cAMP in area CA1 of the mouse hippocampus. Learn Mem. (1997) ;4: (2):230–43. |
[49] | Huang YY , Pittenger C , Kandel ER . A form of long-lasting, learning-related synaptic plasticity in the hippocampus induced by heterosynaptic low-frequency pairing. Proc Natl Acad Sci U S A. (2004) ;101: (3):859–64. |
[50] | Bonsi P . et al Stimulus frequency, calcium levels and striatal synaptic plasticity. Neuroreport. (2003) ;14: (3):419–22. |
[51] | Kerr JN , Wickens JR . Dopamine D-1/D-5 receptor activation is required for long-term potentiation in the rat neostriatum in vitro. J Neurophysiol. (2001) ;85: (1):117–24. |
[52] | Becker JB . Gender differences in dopaminergic function in striatum and nucleus accumbens. Pharmacol Biochem Behav. (1999) ;64: (4):803–12. |
[53] | Cao J . et al Sex Differences in Medium Spiny Neuron Excitability and Glutamatergic Synaptic Input: Heterogeneity Across Striatal Regions and Evidence for Estradiol-Dependent Sexual Differentiation. Front Endocrinol (Lausanne). (2018) ;9: :173. |
[54] | Cao J , Dorris DM , Meitzen J . Neonatal Masculinization Blocks Increased Excitatory Synaptic Input in Female Rat Nucleus Accumbens Core. Endocrinology. (2016) ;157: (8):3181–96. |
[55] | Di Paolo T . Modulation of brain dopamine transmission by sex steroids. Rev Neurosci. (1994) ;5: (1):27–41. |
[56] | Wissman AM . et al Sex differences and effects of cocaine on excitatory synapses in the nucleus accumbens. Neuropharmacology. (2011) ;61: (1-2):217–27. |
[57] | Sazdanovic M . et al Sexual dimorphism of medium-sized neurons with spines in human nucleus accumbens. Arch Biol Sci. (2013) ;65: :1149–1155. |
[58] | Kirson D . et al CB1 and ethanol effects on glutamatergic transmission in the central amygdala of male and female msP and Wistar rats. Addict Biol. (2018) ;23: (2):676–688. |
[59] | Zucker RS . Calcium- and activity-dependent synaptic plasticity. Curr Opin Neurobiol. (1999) ;9: (3):305–13. |
[60] | Hendricson AW , Sibbald JR , Morrisett RA . Ethanol alters the frequency, amplitude, and decay kinetics of Sr2+-supported, asynchronous NMDAR mEPSCs in rat hippocampal slices. J Neurophysiol. (2004) ;91: (6):2568–77. |
[61] | Maldve RE . et al Ethanol selectively inhibits enhanced vesicular release at excitatory synapses: real-time visualization in intact hippocampal slices. Alcohol Clin Exp Res. (2004) ;28: (1):143–52. |
[62] | Crews FT . et al Effects of ethanol on ion channels. Int Rev Neurobiol. (1996) ;39: :283–367. |
[63] | Diamond I , Gordon AS . Cellular and molecular neuroscience of alcoholism. Physiol Rev. (1997) ;77: (1):1–20. |
[64] | Morisot N , Ron D . Alcohol-dependent molecular adaptations of the NMDA receptor system. Genes Brain Behav. (2017) ;16: (1):139–148. |
[65] | Baez MV , Cercato MC , Jerusalinsky DA . NMDA Receptor Subunits Change after Synaptic Plasticity Induction and Learning and Memory Acquisition. Neural Plast. (2018) ;2018: :5093048. |
[66] | Hansen KB . et al Structure, function, and allosteric modulation of NMDA receptors. J Gen Physiol. (2018) ;150: (8):1081–1105. |
[67] | Wang H , Peng RY . Basic roles of key molecules connected with NMDAR signaling pathway on regulating learning and memory and synaptic plasticity. Mil Med Res. (2016) ;3: (1):26. |
[68] | Lovinger DM , Roberto M . Synaptic effects induced by alcohol. Curr Top Behav Neurosci. (2013) ;13: :31–86. |
[69] | Zorumski CF , Mennerick S , Izumi Y . Acute and chronic effects of ethanol on learning-related synaptic plasticity. Alcohol. (2014) ;48: (1):1–17. |